#mitochondrial therapy
Explore tagged Tumblr posts
Text
youtube
#Spinal cord injury#neuroregeneration#nanomedicine#mitochondrial therapy#silica nanoparticles#dual-responsive nanoparticles#targeted drug delivery#neuroprotection#regenerative medicine#oxidative stress#inflammation control#apoptosis inhibition#cellular repair#tissue regeneration#advanced therapeutics#biomaterials#neuroscience research#precision medicine#theranostics#medical breakthrough.#Youtube
2 notes
·
View notes
Text
Mitochondrial Dysfunction in the Pathogenesis of Parkinson’s Disease
Introduction
Parkinson's disease (PD) is a progressive neurodegenerative disorder primarily affecting motor function due to the selective degeneration of dopaminergic neurons in the substantia nigra pars compacta. The pathogenesis of PD is multifactorial, with emerging evidence pointing to mitochondrial dysfunction as a pivotal event in the onset and progression of the disease. This article provides a comprehensive technical analysis of the role of mitochondrial dysfunction in PD, focusing on key molecular mechanisms, genetic factors, and potential therapeutic strategies.
Mitochondria and Their Cellular Roles
Mitochondria are essential organelles that generate the majority of the cell's ATP via oxidative phosphorylation in the electron transport chain (ETC). In addition to their role in energy production, mitochondria are involved in maintaining cellular homeostasis by regulating calcium signaling, apoptosis, and reactive oxygen species (ROS) production. The proper functioning of mitochondria is crucial for neurons, particularly dopaminergic neurons, which have a high metabolic demand.
Mitochondrial Dysfunction and Parkinson's Disease Pathogenesis
Mitochondrial dysfunction in PD primarily manifests through alterations in mitochondrial bioenergetics, increased oxidative stress, defective mitophagy, and calcium dysregulation. These abnormalities converge on exacerbating neuronal injury, particularly in dopaminergic neurons.
1. Impaired Mitochondrial Complex I Activity
One of the hallmark features of mitochondrial dysfunction in PD is the impairment of mitochondrial complex I, the first enzyme complex in the ETC. Complex I is responsible for transferring electrons from NADH to ubiquinone, a critical step in ATP synthesis. Studies consistently show that PD patients exhibit significant reductions in complex I activity in the substantia nigra, which leads to defective ATP production. This mitochondrial dysfunction results in energy deficits, rendering dopaminergic neurons more susceptible to stressors.
Inhibition of complex I activity is not limited to genetic mutations; environmental toxins such as rotenone and paraquat, which inhibit complex I, have been implicated in Parkinsonian syndromes. Furthermore, complex I dysfunction increases the production of ROS, exacerbating oxidative stress in neurons and contributing to mitochondrial damage.
2. Oxidative Stress and ROS Generation
Mitochondria are both the primary source and target of ROS. The process of oxidative phosphorylation inevitably generates ROS as byproducts, particularly superoxide anion, hydrogen peroxide, and hydroxyl radicals. Under normal conditions, ROS are detoxified by endogenous antioxidant systems. However, in PD, mitochondrial dysfunction leads to an imbalance between ROS production and the cell’s antioxidant defenses.
The substantia nigra, which is particularly vulnerable in PD, is exposed to elevated ROS levels due to the high metabolic rate of dopaminergic neurons and the catabolism of dopamine, which generates additional ROS via the action of monoamine oxidase (MAO). Accumulation of ROS results in lipid peroxidation, protein misfolding, and mitochondrial DNA (mtDNA) mutations, all of which contribute to neuronal death and the progression of Parkinson’s pathology.
3. Mitophagy and Dysfunctional Quality Control Mechanisms
Mitophagy, a selective autophagic process that removes damaged or dysfunctional mitochondria, is crucial for maintaining mitochondrial quality and function. In PD, mitophagy is impaired, leading to the accumulation of damaged mitochondria within neurons. The PINK1-parkin pathway plays a pivotal role in the initiation of mitophagy. PINK1, a mitochondrial kinase, accumulates on depolarized mitochondria and recruits the E3 ubiquitin ligase parkin, which ubiquitinates outer mitochondrial membrane proteins to tag them for autophagic degradation.
Mutations in the PINK1 and parkin genes, which are associated with autosomal recessive forms of PD, disrupt this process and contribute to the accumulation of dysfunctional mitochondria. This failure to remove damaged mitochondria exacerbates oxidative stress and promotes the activation of apoptotic signaling pathways. As mitochondrial dysfunction progresses, neuronal survival becomes increasingly compromised, accelerating disease progression.
4. Calcium Homeostasis and Mitochondrial Regulation
Mitochondria play a critical role in buffering cytosolic calcium levels. Neurons, due to their high metabolic activity, are particularly dependent on mitochondrial calcium buffering to prevent cytotoxic calcium overload. However, in PD, mitochondrial dysfunction leads to impaired calcium handling, resulting in an increase in cytosolic calcium concentrations.
Elevated calcium levels activate a variety of calcium-dependent enzymes, such as calpains and phospholipases, that further damage cellular structures. Additionally, excessive calcium in mitochondria can activate the mitochondrial permeability transition pore (mPTP), leading to mitochondrial depolarization, the release of pro-apoptotic factors such as cytochrome c, and eventual cell death.
Genetic Factors in Mitochondrial Dysfunction in PD
Genetic mutations that directly affect mitochondrial function have been identified in familial forms of PD. These mutations often impair mitochondrial dynamics, quality control, and bioenergetics, contributing to the pathogenesis of the disease.
PINK1 and Parkin Mutations: Mutations in the PINK1 gene and the parkin gene, both involved in the regulation of mitophagy, lead to impaired mitochondrial quality control. PINK1, a serine/threonine kinase, normally accumulates on damaged mitochondria and recruits parkin to initiate mitophagy. Loss of PINK1 or parkin function results in the accumulation of dysfunctional mitochondria, contributing to neuronal degeneration.
LRRK2 Mutations: The LRRK2 gene encodes a large protein kinase involved in multiple cellular processes, including mitochondrial dynamics and autophagy. Mutations in LRRK2 are the most common genetic cause of PD, particularly in late-onset forms. LRRK2 is implicated in the regulation of mitochondrial fission and fusion, processes that control mitochondrial morphology and function. Dysregulation of these processes leads to the fragmentation of mitochondria, impaired mitochondrial function, and increased susceptibility to oxidative stress.
Alpha-Synuclein and Mitochondrial Interaction: Alpha-synuclein, the protein most notably associated with Lewy body formation in PD, has also been shown to interact with mitochondrial membranes. Aggregation of alpha-synuclein disrupts mitochondrial dynamics, leading to decreased mitochondrial respiration and increased ROS production. This interaction exacerbates mitochondrial dysfunction and accelerates neurodegeneration.
Environmental Toxins and Mitochondrial Dysfunction
Environmental exposures, particularly to pesticides like rotenone and paraquat, have been shown to inhibit mitochondrial complex I, leading to oxidative stress and mitochondrial dysfunction. These toxins induce PD-like symptoms in animal models, supporting the hypothesis that environmental factors contribute to the pathogenesis of the disease.
Therapeutic Approaches Targeting Mitochondrial Dysfunction
Given the central role of mitochondrial dysfunction in PD, therapeutic strategies aimed at restoring mitochondrial function are being actively explored. These include:
Antioxidant Therapies: Antioxidants such as coenzyme Q10 (CoQ10) have been proposed to alleviate oxidative stress by scavenging ROS. CoQ10 functions as an electron carrier in the ETC and may help restore mitochondrial bioenergetics in PD. Clinical trials, however, have shown mixed results, necessitating further research.
Gene Therapy: Gene therapy approaches aimed at correcting genetic defects that impair mitochondrial function are under investigation. For example, restoring PINK1 or parkin function in neurons may enhance mitophagy and mitigate mitochondrial damage.
Mitochondrial Replacement Therapy: Mitochondrial replacement or mitochondrial transplantation holds promise as a therapeutic strategy for restoring mitochondrial function in PD. Early-stage studies are exploring the feasibility of mitochondrial transplantation into dopaminergic neurons to restore cellular function.
Exercise and Lifestyle Interventions: Regular physical exercise has been shown to stimulate mitochondrial biogenesis and improve mitochondrial function. Exercise-induced upregulation of mitochondrial regulators such as PGC-1α may provide neuroprotective benefits in PD by enhancing mitochondrial turnover and reducing oxidative damage.
Conclusion
Mitochondrial dysfunction is a central event in the pathogenesis of Parkinson's disease, contributing to the degeneration of dopaminergic neurons through mechanisms such as impaired mitochondrial complex I activity, oxidative stress, defective mitophagy, and disrupted calcium homeostasis. Genetic mutations in key mitochondrial regulators such as PINK1, parkin, and LRRK2 exacerbate these defects, while environmental toxins further contribute to mitochondrial damage. Targeting mitochondrial dysfunction through antioxidant therapies, gene therapy, and lifestyle interventions holds promise for mitigating the progression of Parkinson's disease. Understanding the intricate molecular mechanisms linking mitochondrial dysfunction to neurodegeneration in PD will be crucial for developing effective therapeutic strategies.

#Mitochondrial dysfunction#Parkinson’s disease (PD)#Dopaminergic neurons#Substantia nigra#Complex I activity#Oxidative stress#Reactive oxygen species (ROS)#Mitophagy#PINK1#Parkin#Mitochondrial DNA (mtDNA)#Calcium homeostasis#Mitochondrial permeability transition pore(mPTP)#LRRK2#Alpha-synuclein#Mitochondrial dynamics#Genetic mutations in PD#Environmental toxins#Rotenone#Paraquat#Coenzyme Q10 (CoQ10)#Antioxidant therapy#Gene therapy#Mitochondrial replacement therapy#Exercise and mitochondrial biogenesis#Neurodegeneration#Parkinsonian syndromes#Mitochondrial quality control#Mitochondrial fission and fusion#Neuroprotective therapies
0 notes
Text

@strawberryswitchblader One of the problems surrounding Long Covid as a diagnosis is that it encompasses an overly broad variety of post-acute sequelae. You have people experiencing everything from scarring on the lungs, liver and kidney damage, to loss of smell. Then there are those who develop dysautonomic conditions like POTS or who are later diagnosed with ME/CFS and experience Post-Exertional Malaise. There is also a very large (perhaps even the majority) group of persons who will experience a prolonged but temporary period of post-viral fatigue; these are the people who recover gradually on their own, generally within a timeframe of six to eight months. It's not really exercise that leads to their recovery, they would have recovered on their own, and may even have recovered more quickly through a program of radical rest. My beautiful girlfriend is dealing with some post-viral fatigue right now after having gotten sick with mononucleosis this past summer. It's been a real struggle for her dealing with it, but she's also not experiencing PEM, so I'm confident she'll fully recover.
Many of the people who make claims about recovering from "chronic fatigue syndrome" through exercise therapy or some psychological treatment are in this post-viral fatigue category and mistaking correlation for causation and forgetting that the plural of anecdote is not data. The data overwhelmingly supports the notion that for patients experiencing PEM, graded exercise leads to a worsened disease state and a potentially permanently lowered baseline. Before I was diagnosed it's precisely how I inadvertently powerlifted, nightwalked and gradschooled myself into becoming housebound.
And having lived with ME at varying degrees of severity going on twenty-seven years now, I gotta say, it's very boring resting all the time. You get antsy fast. If all it took to get better was walking a bit more every day, I'd jump at the chance, but exercise doesn't really do much for chronic CD8+ T cell exhaustion, or hypofusion causing excess calcium and sodium buildup in skeletal muscles leading to mitochondrial damage. There was a paper that came out just a few months ago that published the results of analyzing blood samples from nearly 1500 ME/CFS patients and 130,000 healthy controls, and they discovered hundreds of biomarkers which indicated everything from insulin resistance to poor blood oxygenation, mitochondrial dysfunction, and systemic chronic inflammation. You can't fix any of that with exercise.
It's all a mess, there really needs to be stricter research diagnostic criteria, and better delineation between the various subtypes. It would clear up so much confusion, but that's also why there haven't been tighter criteria. Exercise and therapy makes for a very inexpensive treatment, one that insurance companies are far more willing to back than experimental anti-viral treatments or IVIg therapy, and in some countries the disability allowances for psychological conditions is less than for physical conditions. If you keep it ambiguous if Long Covid or ME/CFS or fibromyalgia or POTS are physical or psychological diseases, well you save austerity governments a few bucks there too.
#chronic illness#me/cfs#long covid#sorry for using your tags as a jumping off point for an essay. i'm glad your mom recovered.
591 notes
·
View notes
Text
Also preserved on our archive
Some interesting science analyzed
BY BROOKS LEITNER
Imagine lying back in an enclosed chamber where you bask for 90 minutes in a sea of pure oxygen at pressures two to three times that felt at sea level. This is the world of hyperbaric oxygen therapy (HBOT), a technology that’s been around for decades and is now being explored as a possible treatment for Long COVID.
"The silence on the inside is deafening at first,” says John M.,* who has undergone dozens of HBOT treatments for his persistent Long COVID symptoms. Fortunately, there is a television outside the chamber in view, and it is easy to communicate with the provider if needed. While the potential protocol is still being refined, patients may undergo up to 40 HBOT sessions to address some of the most problematic, lingering symptoms of this complex condition.
HBOT is a therapeutic process that has been widely used to treat such conditions as decompression sickness in scuba divers, carbon monoxide poisoning, and diabetic foot ulcers. In HBOT, the body is exposed to 100% oxygen, a significant increase from the 21% oxygen concentration we typically breathe. The therapy takes place in an enclosed chamber where the air pressure is elevated above normal levels. The combination of high-pressure and high-oxygen conditions enhances the amount of oxygen that can reach the body's tissues. The hope is that this therapy can provide the same relief and healing to people with Long COVID that it does for those with other conditions.
According to John M., HBOT was the first treatment that helped with his sleep and reduced his heart palpitations. “At one point after hospitalization, my Long COVID symptoms were so bad that I could barely walk or talk. HBOT was a great tool that really assisted with my recovery,” he said. John added that he hopes the medical community will achieve a better understanding of how HBOT can help relieve suffering for patients with Long COVID and that more research will increase access to this innovative therapy.
Does HBOT improve Long COVID symptoms? One key observation from the work of Inderjit Singh, MBChB, an assistant professor at Yale School of Medicine (YSM) specializing in pulmonary, critical care, and sleep medicine, is that Long COVID patients often experience debilitating fatigue. Based on Dr. Singh’s previous Long COVID research, the exhaustion is thought to be linked to the muscles’ inability to efficiently extract and utilize oxygen.
To picture how HBOT might work, you can think of your muscles as engines sputtering, struggling to get the fuel they need. If oxygen is the gas that fuels the muscles, it’s as if you are trying to complete your daily routine while the gas tank is running on “empty.” By aiming to directly address this oxygen utilization impairment, HBOT may be a potential solution.
A systematic review by researchers at the China Medical University Hospital noted that HBOT could tackle another major factor in the Long COVID puzzle: oxidative stress. This relates to the body's struggle to maintain balance when harmful molecules, known as free radicals, run amok, causing chronic inflammation.
Research co-authored by Sandra K. Wainwright, MD, medical director of the Center for Hyperbaric Medicine and Wound Healing at Greenwich Hospital in Connecticut, suggests that HBOT, with its high-oxygen environment, might dampen this chronic inflammation by improving mitochondrial activity and decreasing production of harmful molecules. Other potential benefits of HBOT in the treatment of Long COVID may include restoration of oxygen to oxygen-starved tissues, reduced production of inflammatory cytokines, and increased mobilization of hematopoietic stem cells—primary cells that transform into red blood cells, white blood cells, and platelets.
HBOT for Long COVID: Current and ongoing research Several small-scale reports have indicated that HBOT is safe for patients with Long COVID.
To address this question, a trial that followed the gold standard of modern medical research—a randomized, placebo-controlled, double-blind design—assigned 73 Long COVID patients to either receive 40 sessions of HBOT or a placebo of only 21% oxygen. The study observed positive changes in attention, sleep quality, pain symptoms, and energy levels among participants receiving HBOT. In a longitudinal follow-up study published in Scientific Reports, the authors at the Tel Aviv University found that clinical improvements persisted even one year after the last HBOT session was concluded. In a second study, the same authors focused on heart function, measured by an echocardiogram, and found a significant reduction in heart strain, known as global longitudinal strain, in patients who received HBOT.
In another study, 10 patients with Long COVID underwent 10 HBOT treatments over 12 consecutive days. Testing showed statistically significant improvement in fatigue and cognitive function. Meanwhile, an ongoing trial at the Karolinska Institute in Sweden has reported interim safety results wherein almost half of the Long COVID patients in the trial reported cough or chest discomfort during treatment. However, it was unclear whether HBOT exacerbated this symptom or if this adverse effect was due to the effort of participation by patients suffering from more severe Long COVID symptoms.
Is HBOT currently available as a treatment for Long COVID? For HBOT to become a mainstream treatment option for Long COVID, several critical priorities must be addressed. First, there is currently no established method for tailoring HBOT dosages to individual patients, so researchers must learn more about the specific features or symptoms that indicate potential benefits from HBOT. At the same time, we need to identify factors that may be associated with any adverse outcomes of HBOT. And finally, it’s important to determine how long these potentially beneficial effects last in a larger cohort. Will just a few HBOT trials be enough to restore patients to their baseline health, or will HBOT become a recurring component of their annual treatment regimen?
For now, HBOT remains an experimental therapy—and as such is not covered by insurance. This is a huge issue for patients because the therapy is expensive. According to Dr. Wainwright, a six-week course of therapy can run around $60,000. That’s a lot to pay for a therapy that’s still being studied. In the current completed studies, different treatment frequencies and intensities have been used, but it’s unclear how the treatment conditions affect the patient’s outcome.
“I have had some patients notice improvements after only 10 or 15 treatments, whereas some others need up to 45 treatments before they notice a difference,” notes Dr. Wainwright. “I think that HBOT is offering some promising results in many patients, but it is probably a strong adjunctive treatment to the other spectrum of things Long COVID patients should be doing, like participating in an exercise, rehab, and nutritional program.”
Dr. Singh notes that “a major challenge for research is the heterogeneity of Long COVID. It is hard to determine which symptoms to treat and enroll patients into trials based on them.”
Perhaps treatments that target multiple issues caused by Long COVID, like HBOT, may help overcome this challenge.
*Not his real name.
Brooks Leitner is an MD/PhD candidate at Yale School of Medicine.
The last word from Lisa Sanders, MD: Hyperbaric oxygen therapy (HBOT) is just one of the many existing treatments that are being looked at to treat Long COVID. We see this with many new diseases—trying to use a treatment that is effective in one set of diseases to treat another. And there is reason for optimism: We know that HBOT can deliver high levels of oxygen to tissues in need of oxygen. That’s why it’s used to treat soft tissue wounds. If reduced oxygen uptake is the cause of the devastating fatigue caused by Long COVID, as is suggested by many studies, then perhaps a better delivery system will help at least some patients.
Studies referenced:
bmcinfectdis.biomedcentral.com/articles/10.1186/s12879-023-08002-8
www.ncbi.nlm.nih.gov/pmc/articles/PMC8806311/
www.nature.com/articles/s41598-024-53091-3
www.nature.com/articles/s41598-022-15565-0
www.frontiersin.org/journals/medicine/articles/10.3389/fmed.2024.1354088/full
www.ncbi.nlm.nih.gov/pmc/articles/PMC11051078/#:~:text=Proposed%20Mechanism%20of%20HBOT%20o
#long covid#hbottherapy#HBOT#hyperbaric oxygen therapy#mask up#covid#pandemic#wear a mask#public health#covid 19#still coviding#wear a respirator#coronavirus#sars cov 2
37 notes
·
View notes
Text
things that people who are stop recommending to CFS/ME/SEID/long COVID with PEM and CF (called "PEM w/ CF" henceforth) patients immediately:
Exercise. This includes yoga, pilates, aquatherapy, and other "gentle" exercises. If it works against gravity or another resistance, it's not good for treating PEM w/ CF. The deconditioning theory was debunked years ago, so don't bring that shit here.
B-12. It's important to get tested for a B-12 deficiency as the symptoms can be similar to PEM w/ CF, but once it's ruled out added B-12 can have side effects. The main and most studied one is insomnia, but anecdotal reports (including my own) also often mention racing heart rate and jitters. Since these side effects use up energy, it's better for people with PEM w/ CF to err on the side of caution and avoid excess B-12 intake. Also it's pretty much the first thing anyone suggests so unless someone is newly ill they've likely already tried it and are sick of hearing about it.
Caffeine and other stimulants. While these can make you feel energized temporarily, it doesn't actually increase the amount of energy in your body. Assuming we're running on the theory of PEM w/ CF being a deficiency of ATP caused by mitochondrial issues, fatigue isn't a state of mind. It's our bodies telling us we're running out of fuel to keep them going. Also many of us have a co-morbid tachycardia condition and a flair up of that condition can cause a flair up of fatigue.
Medication and other treatments designed for mental illnesses. While having a chronic illness can cause mental illness, especially if you live in an ableist country with failed disability aid services, mental illness does not cause PEM w/ CF. Treatment for mental illness won't actually treat the symptoms of PEM w/ CF.
Alternative medicine. Many alternative medicine treatments are actively dangerous and the idea that there's "no harm in trying" because "it's natural anyway" is false. Stop recommending ozone therapy (potentially deadly and not useful in concentrations not strong enough to kill you), stop recommended chiropractors (can result in a stroke), stop recommending essential oils (no approved medical use beyond basic relaxation and concentrated nature can make them dangerous), you get the picture. Alternative medicine preys on desperate suffering people to sell them ineffective, potentially dangerous "treatments", don't do their advertising for them.
PEM w/ CF patients feel free to add on treatments that are commonly recommended but unscientific/uneffective, others be respectful and don't derail.
#cfs#chronic fatigue syndrome#me/cfs#cfs/me#seid#long covid#cpunk#cripplepunk#cripple punk#actually disabled
94 notes
·
View notes
Text
Moon Missions
What’s going on with the moon?
The United States recently had a solar eclipse on April 8th, 2024, and some might be surprised to learn that the moon is, in fact, affected by solar radiation. The charged particles emitted by the sun, called the solar wind, reach the moon with no interruption from its atmosphere, as it has none. It also has no global magnetic field, another layer of protection that Earth does have, in comparison.
The moon does, however, have small areas of magnetic fields. We can see this because these areas remain lighter in photos whereas chemical reactions from radiation darken the unprotected areas.
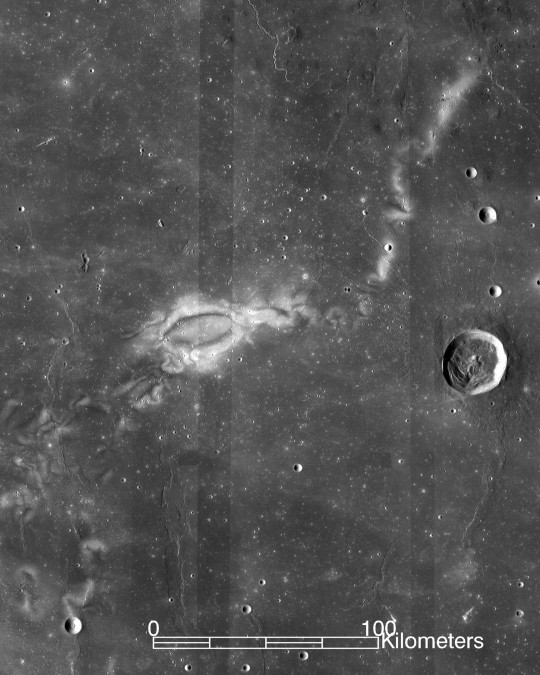
Fortunately, most of these charged particles cannot pass through the hulls of space stations, so astronauts are safe in orbit. Cosmic rays, made of stronger and faster-moving particles, are more dangerous. Even on Earth, under the atmosphere and magnetosphere, cosmic radiation reaches humans, though not enough to be considered damaging to our health.
A lander and rover launched in 2018 delivered the first measurements of radiation levels on the moon 4. Based on those data, astronauts on the moon can be exposed to up to 150 times higher radiation levels than on Earth.
Radiation is a leading reason for the pause in lunar landing missions. It raises risks of cataracts, heart diseases, radiation illness, cancer, and other ailments. Longer missions, of course, would heavily exacerbate these radiation doses.
Other Health Concerns
Cosmic rays contain High-Energy (HZE) ions. In different exposure such as from nuclear accidents or irradiation therapy, HZE ions have been found to cause dysregulation in the mitochondria and damage to DNA. Because of this, prolonged exposure is linked to health effects often associated with aging, such as hippocampus synapse loss and metabolic disruption caused by damage to mitochondrial DNA.
Long-duration space flights have also been linked to cardiovascular disorders. For astronauts on the Apollo missions, heart attack was “the second leading cause of death” 8. For additional space flights outside of Earth’s magnetosphere, astronauts also had a higher mortality rate due to cardiovascular diseases.
In a previous article, we discussed the relationship between circadian rhythms and health. These rhythms are another thing that space travel can impact, causing sleep and mental health disturbances in astronauts 9.
While various studies are investigating the conditions of these health risks, a current NASA mission is specifically investigating radiation protection.
Long-term Mission
NASA plans on eventually returning to human-manned missions to the moon.
First, they have to address the issues discussed above.
In November of 2022, Artemis I launched with two manikins bearing radiation detectors. From this mission, NASA was able to confirm the success of the intended trajectory, launch of ground systems, and the Orion spacecraft. The radiation results from this mission are still being analyzed.
The Artemis missions are intended to explore more of the moon than ever before, and lay groundwork for eventual missions to Mars.
Artemis II will not launch any earlier than September of 2025. It is planned to last ten days, consist of a 4-person crew, and be a lunar flyby to ensure the proper functioning of the spacecraft’s systems.
It has seemed for years that lunar exploration has halted. Manned missions have indeed been paused, for good reasons. Ensuring the safety of astronauts is a priority, and they face serious health risks even when missions go as expected. But NASA intends to continue exploring space, the moon, and Mars. The current Artemis missions are discovering improved, new ways to ensure the safety of astronauts while making scientific progress.
Additional Resources
1. https://science.nasa.gov/moon/solar-wind/
2. https://phys.org/news/2012-01-solar-flares-astronauts.html
3. https://arxiv.org/ftp/arxiv/papers/1211/1211.3962.pdf
4. https://link.springer.com/article/10.1007/s11214-020-00725-3
5.https://www.nasa.gov/missions/artemis/orion/orion-passengers-on-artemis-i-to-test-radiation-vest-for-deep-space-missions/
6.https://www.smithsonianmag.com/science-nature/how-space-radiation-threatens-lunar-exploration-180981415/
7.https://www.nasa.gov/humans-in-space/analysis-confirms-successful-artemis-i-moon-mission-reviews-continue-2/
8.https://www.frontiersin.org/journals/physiology/articles/10.3389/fphys.2020.00955/full
9. https://www.ncbi.nlm.nih.gov/pmc/articles/PMC9818606/
#radiation#moon#moon mission#nasa#nasa photos#article#research#solar wind#solar radiation#cosmic rays#cosmic radiation#space exploration#science#space#Work has been horribly busy so the next article might not be out on the usual schedule
19 notes
·
View notes
Text
i've been collecting resources and studies about myalgic encephalomyelitis for upcoming doctors appointments, and since there's a lot of misinformation about ME out there i thought i'd make a post with links and information that might be helpful for other ME patients, or just generally educational!
for anyone who doesn't know, myalgic encephalomyelitis is a debilitating multi system chronic illness. it's most characteristic symptom is post exertional malaise/symptom exacerbation (meaning symptoms getting significantly worse after exertion with prolonged recovery periods - see the diagnostic criteria linked below for more explanation). it has previously been called chronic fatigue syndrome, a name associated with claims of it being a psychosomatic condition rather than a medical one despite evidence to the contrary (here's a page with some information about the history of ME, and see the pathology section further down for evidence of ME being a physical disease). ME is also often comorbid with conditions like POTS (or other forms of orthostatic intolerance and dysautonomia), MCAS, and Small Fibre Neuropathy, and it's not uncommon for people who have long covid to develop ME. but yea! here's some links to resources i've gathered.
general resources/overviews:
Chronic Fatigue Syndrome Myalgic Encephalomyelitis Primer For Clinical Practitioners 2014 Edition
Diagnosis and Management of Myalgic Encephalomyelitis - ME Action
Initiating Care of a Patient With Myalgic Encephalomyelitis/Chronic Fatigue Syndrome (ME/CFS)
Medical considerations when treating urgently ill patients with underlying myalgic encephalomyelitis/chronic fatigue syndrome (ME/CFS)
Caring for the Patient with Severe or Very Severe Myalgic Encephalomyelitis/Chronic Fatigue Syndrome
Three Cases of Severe ME/CFS in Adults
diagnostics:
Myalgic Encephalomyelitis: International Consensus Criteria <- just the criteria
Myalgic encephalomyelitis: International Consensus Criteria <- criteria with explanation of how and why it was developed
TESTING RECOMMENDATIONS FOR SUSPECTED ME/CFS US ME/CFS Clinician Coalition
there are multiple ME severity scales, and exact definitions of what constitutes mild/moderate/severe/very severe vary a bit, but here is one: M.E. Disability Scale, another one is the ME/CFS Disability Rating Scale from ME Association, however the pdf on their website costs so i also have a pdf that i made with the text
pathology:
Brainstem volume changes in myalgic encephalomyelitis/chronic fatigue syndrome and long COVID patients
Decreased oxygen extraction during cardiopulmonary exercise test in patients with chronic fatigue syndrome
Developing a blood cell-based diagnostic test for myalgic encephalomyelitis/chronic fatigue syndrome using peripheral blood mononuclear cells
Human Herpesvirus-6 Reactivation, Mitochondrial Fragmentation, and the Coordination of Antiviral and Metabolic Phenotypes in Myalgic Encephalomyelitis/Chronic Fatigue Syndrome
Mitochondrial complex activity in permeabilised cells of chronic fatigue syndrome patients using two cell types
Muscle sodium content in patients with Myalgic Encephalomyelitis/Chronic Fatigue Syndrome
Redox imbalance links COVID-19 and myalgic encephalomyelitis/chronic fatigue syndrome
The use of oxygen as a possible screening biomarker for the diagnosis of chronic fatigue
Tissue specific signature of HHV-6 infection in ME/CFS
treatment (both helpful and harmful):
ME/CFS TREATMENT RECOMMENDATIONS US ME/CFS Clinician Coalition
Low-dose naltrexone in the treatment of myalgic encephalomyelitis/chronic fatigue syndrome (ME/ CFS)
Potential Therapeutic Benefit of Low Dose Naltrexone in Myalgic Encephalomyelitis/Chronic Fatigue Syndrome: Role of Transient Receptor Potential Melastatin 3 Ion Channels in Pathophysiology and Treatment
Back to the Future? Immunoglobulin Therapy for Myalgic Encephalomyelitis/Chronic Fatigue Syndrome
Evidence Against Exercise for people with PEM/PESE in Long COVID and ME/CFS
PACE trial claims for recovery in myalgic encephalomyelitis/chronic fatigue syndrome – true or false? It’s time for an independent review of the methodology and results
Treatment harms to patients with ME/CFS
#more than okay to reblog!#🐛#myalgic encephalomyelitis#me/cfs#chronic fatigue syndrome#chronic illness#disability#actually disabled#long covid
143 notes
·
View notes
Text
Sounds like the local team is going to have us reapply and then appeal the older denials so we might get paid for at least the work my husband is doing while we wait for the appeals to finally get approved. The guy who is confused about the denial is the director of eligibility here and ironically enough the same guy I saw about ketamine therapy last year and has experience with mitochondrial disease.
#the ketamine thing ended up being 'well shit i dont think it would help but you can TRY when you're not trying to get pregnant'#and is a good guy
13 notes
·
View notes
Text
This place make my mind and body happy!!! @upgradelabsriverton 😁 The therapies here are designed to tame inflammation and promote healing on a cellular level. This is beneficial for anyone with inflammation, or athletes needing recovery. I often point my clients to these therapies because of their science-backed benefits. Here are some I enjoyed today....
RED LIGHT THERAPY - improves cellular function and health by enhancing mitochondrial function and improving the production of ATP (cells energy source)
LYMPHATIC MASSAGE (the big squeeze) - stimulates the lymphatic system responsible for transporting white blood cells to help fight infection and strengthen immune system
CRYOTHERAPY (cold therapy) - lowers oxidative stress (free radicals) and inflammation by reducing the production and release of pro-inflammatory substances (eg. cytokines) while increasing the release of anti-inflammatory substances (noradrenaline)
If this Hawaiian can do cryotherapy, so can you! Thank you Tom, Prosper & Nate 🤙🏽
#biohacking#cellhealth#inflammation#antiinflammatory#health#Healthy#longevity#healthyliving#healthylifestyle#recovery#cognitive#brain health
2 notes
·
View notes
Text
Dr. Thomas Seyfried: Cancer as a Mitochondrial Metabolic Disease
youtube
1 - Cancer is a type of metabolic disease: It is not a genetic disease!
2 - A reliance on substrate level phosphorylation for energy is the metabolic hallmark of all or nearly all cancers.
3 - The simultaneous restriction of glucose and glutamine can manage GBM and most other cancers.
4 - Press-Pulse metabolic therapy is a non-toxic, cost effective strategy for the management and possible resolution of all types of cancer!
Cancer can be addressed with a combination of calorie restriction and keto diet.
5 notes
·
View notes
Text
youtube
#Mitochondria-targeted therapy#photoredox catalysis#pyroptosis#tumor therapy#cancer treatment#oxidative stress#mitochondrial disruption#light-activated therapy#immune activation#cancer immunotherapy#programmed cell death#oncology breakthrough#cancer research#targeted cancer therapy#precision oncology#tumor targeting#photodynamic therapy#innovative oncology#cell death mechanisms#clinical translation.#Youtube
0 notes
Text
Mitochondrial Dysfunction in Cardiovascular Disease
Introduction
Mitochondria are essential organelles responsible for the production of cellular energy in the form of adenosine triphosphate (ATP) through oxidative phosphorylation (OXPHOS). The heart, due to its continuous contractile activity, has a high energy demand and is critically dependent on mitochondrial function for normal physiological and pathological processes. Mitochondrial dysfunction has emerged as a central mechanism in the pathogenesis of cardiovascular diseases (CVDs), including ischemic heart disease, heart failure, hypertension, and arrhythmias. This technical overview discusses the molecular mechanisms of mitochondrial dysfunction in cardiovascular disease, its impact on cellular and organ function, and the potential therapeutic strategies to mitigate mitochondrial-related pathophysiology in CVDs.
Mitochondrial Function in Cardiovascular Cells
Mitochondria are highly dynamic organelles that perform several key functions crucial for the health of cardiovascular cells. They are involved in:
ATP Production via Oxidative Phosphorylation: In the mitochondria, energy production is driven by the electron transport chain (ETC), which is composed of complex I-IV embedded in the inner mitochondrial membrane. Electrons derived from NADH and FADH2 produced during the citric acid cycle are transferred through these complexes to ultimately reduce oxygen to water at complex IV. This electron transfer drives proton pumps that create an electrochemical gradient (proton motive force) across the inner mitochondrial membrane, which is utilized by ATP synthase (complex V) to produce ATP.
Calcium Homeostasis: Mitochondria play a crucial role in buffering intracellular calcium concentrations. They take up calcium from the cytoplasm in response to cellular signaling and help maintain cellular homeostasis by storing calcium in the matrix and releasing it when required for cellular signaling. Dysregulation of mitochondrial calcium handling can lead to pathophysiological conditions such as mitochondrial permeability transition (MPT) and cell death.
Reactive Oxygen Species (ROS) Production: Mitochondria are the primary source of ROS due to the incomplete reduction of oxygen molecules during electron transport in the ETC. Under normal conditions, low levels of ROS act as signaling molecules. However, excessive ROS generation due to mitochondrial dysfunction can cause oxidative stress, which damages cellular components such as lipids, proteins, and mitochondrial DNA (mtDNA), contributing to the pathogenesis of cardiovascular diseases.
Apoptosis and Cell Death: Mitochondria are central regulators of apoptosis. The release of pro-apoptotic factors such as cytochrome c from the mitochondrial intermembrane space into the cytoplasm triggers caspase activation, leading to programmed cell death. Mitochondrial dysfunction in cardiovascular tissues can lead to inappropriate cell death, contributing to the progression of CVDs.
Molecular Mechanisms of Mitochondrial Dysfunction in Cardiovascular Disease
Mitochondrial dysfunction in cardiovascular disease can result from several factors, including oxidative damage, altered mitochondrial dynamics, mutations in mitochondrial DNA, and defects in mitochondrial signaling. Below are the primary molecular mechanisms contributing to mitochondrial dysfunction in cardiovascular pathologies:
1. Oxidative Stress and ROS Accumulation
Excessive ROS generation is a hallmark of mitochondrial dysfunction and a major contributor to cardiovascular disease progression. Under normal conditions, the ETC produces ROS as a byproduct of electron transfer; however, under pathological conditions such as ischemia, hypoxia, or heart failure, there is an increase in mitochondrial ROS production. This increase is due to the altered electron flow through the ETC, particularly at complex I and III, which results in the incomplete reduction of oxygen.
The accumulation of ROS causes oxidative damage to mitochondrial lipids, proteins, and mtDNA. For instance, lipid peroxidation of mitochondrial membranes leads to membrane destabilization and disruption of mitochondrial function. ROS also modify proteins involved in mitochondrial dynamics and bioenergetics, impairing the capacity of mitochondria to generate ATP. Furthermore, oxidative damage to mtDNA leads to mutations that compromise the mitochondrial respiratory chain complexes, creating a vicious cycle of mitochondrial dysfunction.
2. Mitochondrial Permeability Transition (MPT) and Calcium Overload
Mitochondrial permeability transition is a critical event in mitochondrial dysfunction. The opening of the mitochondrial permeability transition pore (mPTP) occurs when the inner mitochondrial membrane becomes permeable to ions and small molecules, disrupting the electrochemical gradient required for ATP production. Under pathological conditions such as ischemia-reperfusion injury, excessive ROS and calcium overload activate the mPTP, leading to mitochondrial swelling, loss of membrane potential, and the release of pro-apoptotic factors (e.g., cytochrome c), triggering cell death.
Calcium overload plays a significant role in mitochondrial dysfunction. During stress conditions like ischemia, excessive intracellular calcium is taken up by mitochondria, causing mitochondrial matrix expansion and rupture of the mitochondrial membrane. This exacerbates cellular injury and promotes cell death pathways in the myocardium, contributing to myocardial infarction and heart failure.
3. Mitochondrial Dynamics Dysregulation
Mitochondrial dynamics refer to the continuous processes of mitochondrial fusion and fission that maintain mitochondrial quality and function. In response to cellular stress, mitochondria can undergo fission to segregate damaged components or fusion to promote functional compensation. Mitochondrial fission is regulated by dynamin-related protein 1 (DRP1), while fusion is mediated by mitofusins (MFN1 and MFN2) and optic atrophy 1 (OPA1). In cardiovascular diseases, this dynamic balance is often disrupted, leading to mitochondrial fragmentation, reduced mitochondrial function, and increased susceptibility to apoptosis.
In heart failure, for example, the upregulation of DRP1 and downregulation of fusion proteins contribute to mitochondrial fragmentation, reduced ATP production, and elevated ROS levels. This dysfunction is exacerbated by altered signaling pathways, including those associated with autophagy (mitophagy), which is responsible for removing damaged mitochondria. Dysfunctional mitophagy further impairs mitochondrial quality control, worsening cardiac injury.
4. Mitochondrial DNA Mutations
Mitochondrial DNA is more prone to mutations than nuclear DNA due to its proximity to the ETC and lack of protective histones. In cardiovascular diseases, mutations in mtDNA contribute to defective mitochondrial function. For example, mutations in genes encoding subunits of the OXPHOS complexes (such as ATP6, ND1, or CYTB) lead to impaired ATP synthesis and defective mitochondrial bioenergetics, contributing to myocardial ischemia and heart failure.
Mitochondrial mutations may also affect the regulation of ROS production and the activation of apoptotic pathways, accelerating tissue damage and organ dysfunction.
Therapeutic Approaches Targeting Mitochondrial Dysfunction in Cardiovascular Disease
Given the critical role of mitochondria in cardiovascular disease, several therapeutic strategies have been developed to target mitochondrial dysfunction and restore normal mitochondrial function. These include:
1. Mitochondrial Antioxidants
Mitochondrial-targeted antioxidants, such as MitoQ, MitoTEMPO, and SkQ1, have been developed to specifically target ROS within mitochondria. These compounds aim to reduce oxidative stress, limit mitochondrial damage, and improve mitochondrial function. Clinical studies are ongoing to assess the efficacy of these antioxidants in reducing myocardial injury and improving outcomes in heart failure and ischemic heart disease.
2. Mitochondrial Biogenesis Activation
Stimulating mitochondrial biogenesis to increase the number of functional mitochondria is another potential therapeutic strategy. Activators of peroxisome proliferator-activated receptor gamma coactivator 1-alpha (PGC-1α), a key regulator of mitochondrial biogenesis, are being investigated as potential treatments for heart failure. Exercise training is a natural way to activate PGC-1α and increase mitochondrial function, which has been shown to improve cardiac outcomes in patients with heart failure.
3. MPTP Inhibition
Inhibitors of the mPTP, such as cyclosporine A, have been studied for their potential to prevent ischemia-reperfusion injury by inhibiting pore opening. By preserving mitochondrial integrity, these inhibitors may help reduce myocardial damage and improve survival after myocardial infarction.
4. Gene Therapy and Mitochondrial Transplantation
Gene therapy approaches, including the use of CRISPR/Cas9 to correct mitochondrial DNA mutations, hold promise in treating mitochondrial diseases. Additionally, mitochondrial transplantation, where healthy mitochondria are delivered to damaged cardiac cells, is an emerging area of research, with the potential to restore mitochondrial function and improve heart function in patients with severe myocardial injury.
Conclusion
Mitochondrial dysfunction plays a central role in the pathogenesis of cardiovascular diseases, contributing to impaired ATP production, increased ROS production, and cell death. Understanding the molecular mechanisms underlying mitochondrial dysfunction provides critical insights into the development of novel therapeutic strategies. Approaches targeting mitochondrial biogenesis, oxidative stress, mitochondrial dynamics, and mPTP inhibition offer promising avenues for the treatment of cardiovascular diseases and could lead to more effective management of conditions such as heart failure, ischemic heart disease, and hypertension. However, further research and clinical trials are needed to fully elucidate the potential of these therapeutic strategies in improving cardiovascular health.
#Mitochondria#Cardiovascular Disease (CVD)#Mitochondrial Dysfunction#Oxidative Phosphorylation#ATP Production#Reactive Oxygen Species (ROS)#Mitochondrial DNA (mtDNA)#Mitochondrial Permeability Transition (MPT)#Calcium Homeostasis#Heart Failure#Ischemic Heart Disease#Hypertension#Mitochondrial Dynamics#Mitochondrial Fission and Fusion#Mitochondrial Biogenesis#Mitochondrial Antioxidants#Mitochondrial Targeted Antioxidants#MPTP Inhibitors#Gene Therapy#Mitochondrial Transplantation#Electrochemical Gradient#Mitochondrial Fragmentation#Cytochrome C#Cell Death (Apoptosis)#Peroxisome Proliferator-Activated#Receptor Gamma Coactivator 1-alpha (PGC-1α)
0 notes
Text
Meet the Amatruda Lab!
James Amatruda, MD, PhD

www.chla.org
Dr. James Amatruda is the Head of Basic and Translational Research for the Cancer and Blood Disease Institute at Children’s Hospital Los Angeles. He’s the inaugural holder of the Dr. Kenneth O. Williams Chair in Cancer Research. Dr. Amatruda is a Professor of Pediatrics and Medicine for the Keck School of Medicine of USC. He attends on the Solid Tumor oncology service at CHLA.
Dr. Amatruda received his MD and PhD from Washington University School of Medicine. He completed his internship and residency in Internal Medicine from Brigham and Women’s Hospital. He was a Visiting Fellow at the Institute of Cell Biology in Consiglio Nazionale delle Ricerche in Rome and completed his Medical Oncology fellowship at Dana-Farber/Partners Cancer Care in Boston, Massachusetts.
When not in the lab, Dr. Amatruda enjoys running, reading, music-making and exploring around Los Angeles.
Ashley Jean, MD
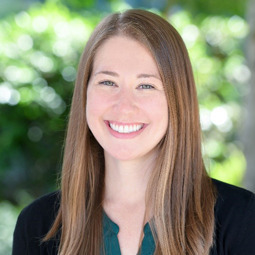
www.chla.org
Dr. Ashley Jean is a Clinical Fellow in the Amatruda Lab. Dr. Jean graduated from Tufts Medical School in Boston and completed her Pediatric Residency at Maine Medical Center. Dr. Jean started her Pediatric Fellowship at Children’s Hospital Los Angeles in 2019.
Her research focuses on pediatric Ewing Sarcoma. She is currently studying the TAK1 pathway in the tumor genesis of this condition.
Dr. Jean likes to spend her free time outdoors. She enjoys activities such as hiking, paddle boarding and snowboarding.
Christopher Kuo, MD

www.chla.org
Dr. Christopher Kuo is a Clinical Fellow in the Amatruda Lab. Dr. Kuo received his Medical Degree from Rush University and completed his Pediatric Residency from Children’s Hospital Los Angeles. Dr. Kuo started his Pediatric Fellowship at Children’s Hospital Los Angeles in 2020.
His research interest is in osteosarcoma. He is currently working on a project that involves the investigation of the tumor microenvironment of Ewing sarcoma.
Dr. Kuo’s hobbies include breakdancing, swimming and going to coffee shops.
Adam Marentes, MSc., PhD Candidate

www.chla.org
Adam Marentes is a Graduate Student Researcher in the Amatruda Lab. Adam received his Bachelor of Science in Neuroscience from the University of California, Riverside. He then completed his Master of Science from California Polytechnic University Pomona. Adam is currently attending University of Southern California Keck School of Medicine to earn his PhD in Cancer Biology and Genomics.
Adam’s research focus is in mitochondrial DNA variants in Ewing Sarcoma. He is currently working on a collaboration that involves editing mitochondrial DNA in cancer cell lines in zebrafish.
Adam enjoys baking, playing video games with his fiancé and catching a show at the local comedy club.
Tanya Mosesian, MHA

www.chla.org
Tanya Mosesian received her Bachelor of Science in Public Health from California State University of Northridge. She then completed her Master of Health Administration at the University of Southern California.
Tanya is Project Associate for the Amatruda Lab. She provides on-site support for all administrative matters and project facilitation.
Tanya enjoys spending time with her family and friends. She likes to play tennis and hike during the weekends.
Elena Vasileva, PhD, MSc.
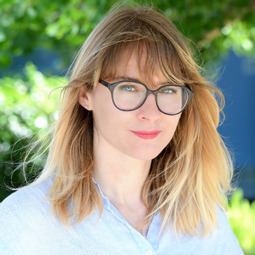
www.chla.org
Dr. Elena Vasileva is a post-doctoral fellow in the Amatruda Lab. Dr. Vasileva received her Bachelor of Science and Master of Science from Peter the Great St. Petersburg Polytechnic University in Applied Mathematics and Physics. She received her PhD in Molecular Biology from the Institute of Cytology, Russian Academy of Sciences.
Dr. Vasileva is interested in studying the molecular mechanisms of cancer development and progression. She has developed an inducible zebrafish model of EWS-FLI driven Ewing Sarcoma as a platform for biologic discovery and preclinical testing of novel therapies.
Dr. Vasileva enjoys running and hiking in Los Angeles.
Mona Wu, PhD
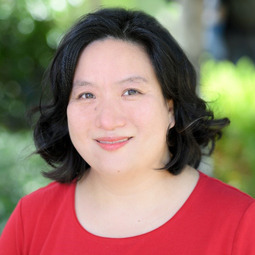
www.chla.org
Dr. Mona Wu is a post-doctoral fellow in the Amatruda Lab. Dr. Wu received her Bachelor of Science from the University of British Columbia, a Master of Science from Université de Montréal, and a PhD from McGill University.
Dr. Wu is interested in understanding the cell of origin for pediatric neoplasms because she believes that this knowledge could lead to better early biomarkers and more effective treatment. She is particularly interested in understanding how aberrant ncRNA (especially miRNAs) may play a role in pediatric disease.
Dr. Wu likes reading and visiting different libraries. She enjoys “foodie-related” activities including trying restaurants, cooking, baking and watching (far too many) cooking shows.
5 notes
·
View notes
Link
Recurring DNA variants in EDS that merit evaluation in COVID19 resistance include those impacting connective tissue elements--51 in COL5 (joint), 29 in COL1/2/9/11 (bone), 13 in COL3 (vessel), and 18 in FBN1 (vessel-heart)--or neural function--93 in mitochondrial DNA, 28 in COL6/12, 16 in SCN9A/10A/11A, 14 in POLG, and 11 in genes associated with porphyria.
Although initial analyses of connective tissue disorders focused on tethering proteins like collagens [1–7], its necessary role in protist to metazoan transitions [14] requires that connecting tissue be medium [15–21] and message [12, 22–28]. The result in complex organisms that combine internal homeostasis with external movement is a diversity of constraining and connecting structures [skin, joint, skeleton—15-21] permeated by wired [nerve 12-13, 22-25], moving [muscle 26-28] and circulating [heart-vessel 7] parts. All of these tissues including many blood components have a common developmental origin [mesoderm, mesenchyme--29] that reflects an evolutionary drive for cell connection.
The inevitable consequence of this integrated anatomy is a reciprocal relationship between the systems that constrain/contain body or blood [20,21] and the nervous system that coordinates their functions [22,23]. Disposition to tissue laxity will not only cause wear-and-tear osteoarthritis and skeletal bends from gravity [deformations like scoliosis, 4-8] but also will provoke adrenergic response to restore cerebral circulation deprived by vessel distensibility and lower body blood pooling [9–12]. Repeated adrenergic stimulation, evident even in those with minimal or benign joint hypermobility [9], produces the brain fog, stress response, and chronic fatigue of postural orthostatic tachycardia syndrome [30–32], the reactive allergic [32–33], immune [23,34], and inflammatory [35] symptoms of mast cell activation [32–33], and, through cholinergic suppression, the irregularity, reflux, and swallowing difficulties of irritable bowel syndrome [36].
Moving beyond the joint hypermobility and skin elasticity emphasized by dermatologists Ehlers and Danlos [11] are S1 Table assessments of neuromuscular symptoms like migraines or poor balance that affect a respective 60% or 61% of EDS females, 96% of all patients having at least one of 12 neuromuscular findings by history. Equally frequent are the brain fog (83%) or chronic fatigue (87%) of postural orthostatic tachycardia syndrome (POTS) in EDS females, the bowel irregularity (82%) or bloating-reflux (79%) of irritable bowel syndrome (IBS), and the rashes (42%) or asthma-dyspnea (49%) of mast cell activation syndrome (MACS) in S1 Table. All but 3 of the 1261 patients had 1 of these dysautonomia findings and the average among females and males of all ages was 12 of the 20 findings.
The neuromuscular and dysautonomia findings are inextricably linked to those of joint-skin-vessel laxity as shown in the Appendix, 483 females over 10.5 years who presented with joint pain having similar numbers of joint 5.8 to 5.1 of 10, skin (2.5 each of 10), or dysautonomia findings (12.2 to 13.1 of 20) as the 358 who presented with postural orthostatic tachycardia syndrome (POTS). Underappreciation of these neuromuscular and autonomic problems and their exclusion from consensus findings [66] is a major reason for the diagnostic delays for males (7 years, 21-14) and females (13 years, 30-17) in Table 1, columns 2-3 rows 4-5)
Flexible glia, dura and vertebrae allow descent of the lower brain to produce Chiari deformation in 14% of women [70] plus back pain from spinal disc herniation or degeneration in 42% [13].
Equally flexible pelvic ligaments (exaggerated in women for parturition) lead to pelvic congestion [71–73] and urogenital problems (menorrhagia—67%, endometriosis—33%) that when counted give women an average 1.5-point excess in total history scores (S2 Table). Inflicting circulatory imbalance is the increased distensibility of vessels that leads to lower body blood pooling (57% of EDS women have foot discoloration upon standing), the reactive adrenergic stimulation producing stress-related psychiatric symptoms [74] that combine with those from pain [75–76] and inflammation [77–78]. Some of these changes including rare joint contractures [79] were undoubtedly related to aging as 74 EDS females and 6 males were over 50 years old.
Comprehensive assessment of dysautonomia findings in EDS [9-10, 30-33] is crucial for diagnosis and for correlating its cardiovascular, immune, and inflammatory changes with the gene changes in S2 and S3 Tables. Holistic ascertainment renders EDS types as subtle variations on a theme or spectrum…
Grouping of genes by impact on peripheral nerve (Np) does seem appropriate since the consistent phenotype of Charcot-Marie-Tooth disease with its classic steppage and foot-drop gait is associated with 7 genes and their 22 variants including LMNA and AARS1 in Fig. 3.
Symptoms common to EDS and long COVID are shown at the top of Fig 5 using the analogy to Tolkien’s Ents: A tissue laxity-dysautonomia entome is imagined with a converging network of contributing genes at the bottom (roots) and a diverging network of symptoms at the top (branching canopy), the two connected through major pathophysiologic mechanisms like articulo-autonomic dysplasia (flowing channels of phloem or sap in the trunk). Peripheral genes with less impact on the central mechanism will have less disruptive variations in affected patients while those like COL3A1(M120180) will act as nodes in these gene networks and cause more numerous and severe symptoms.
The large percentage ranges for symptoms in both patient groups reflects the heterogeneity of patient ascertainment (clinic, online, retrospective in EDS, different hospitalized-outpatient cohorts, post-infection times for COVID19), and the subjective nature of reported findings. All symptoms, ordered by percentage in COVID19 patients, are more frequent in EDS although ranges are a bit more compatible. Symptoms of autonomic imbalance (brain fog, chronic fatigue, asthma-dyspnea, sleep difficulties, and tachycardia) are common in both EDS and long COVID19 (Fig 5), compatible with a prior hypothesis [42]. Asthma is a consequence of mast-cell activation [32–33], the other four of postural orthostatic tachycardia syndrome [30–31, 39].
Less common in long COVID19 than EDS are IBS symptoms and those orthostatic hypotension like syncope and dizziness. Neurologic symptoms like difficulty walking-poor balance, muscle weakness, myalgia, and frequent headaches occur in both as does joint pain that is common in EDS, post-infectious, or autoimmune illnesses (Fig 5).
This clinical genetic study of EDS relates its quantified finding pattern to underlying articulo-autonomic dysplasia mechanisms and the multiple gene variants found by NextGen DNA sequencing. Major results are the connected tissue laxity-neural symptoms of EDS, their relation to disparate nuclear and mitochondrial genes, and the similarities of these relationships to those of acute or long COVID19. The study illustrates the potential strengths and limitations of genomic analysis as summarized below.
Many genes like FLNC in Table 4 are associated with cardiomyopathy and muscle weakness, reflecting overlap of proteins in cardiac, skeletal, and probably intestinal smooth muscle (possibly contributing to the 92% of EDs patients with bowel dysmotility in S1 Table). Further study of musculoskeletal and mitochondrial dysfunction [140–141] in EDS and acute/long COVID19 could justify trials of promising dietary [31], physical therapy [18, 142] and exercise [75, 121, 143] protocols in both disorders. Important objectives regarding long COVID19 are to associate symptom frequencies and outcome measures with defined post-infection time periods, then determine whether the genes influencing COVID19 infectious (S5 Table) also influence the duration and disability of its post-infectious phases.
1 note
·
View note
Text
An interesting perspective article about Long Covid
Should we be fighting gene damage instead of individual symptoms?
The pathogenesis of long COVID (LC) still presents many areas of uncertainty. This leads to difficulties in finding an effective specific therapy. We hypothesize that the key to LC pathogenesis lies in the presence of chronic functional damage to the main anti-inflammatory mechanisms of our body: the three reflexes mediated by the vagus nerve, the hypothalamic-pituitary-adrenal (HPA) hormonal axis, and the mitochondrial redox status. We will illustrate that this neuro-endocrine-metabolic axis is closely interconnected and how the SARS-CoV-2 can damage it at all stages through direct, immune-inflammatory, epigenetic damage mechanisms, as well as through the reactivation of neurotropic viruses. According to our theory, the direct mitochondrial damage carried out by the virus, which replicates within these organelles, and the cellular oxidative imbalance, cannot be countered in patients who develop LC. This is because their anti-inflammatory mechanisms are inconsistent due to reduced vagal tone and direct damage to the endocrine glands of the HPA axis. We will illustrate how acetylcholine (ACh) and cortisol, with its cytoplasmatic and cellular receptors respectively, are fundamental players in the LC process. Both Ach and cortisol play multifaceted and synergistic roles in reducing inflammation. They achieve this by modulating the activity of innate and cell-mediated immunity, attenuating endothelial and platelet activation, and modulating mitochondrial function, which is crucial for cellular energy production and anti-inflammatory mechanisms. In our opinion, it is essential to study the sensitivity of the glucocorticoids receptor in people who develop LC and whether SARS-CoV-2 can cause long-term epigenetic variations in its expression and function.
#mask up#public health#wear a mask#pandemic#wear a respirator#covid#still coviding#covid 19#coronavirus#sars cov 2#long covid
17 notes
·
View notes
Text
In addition to mitochondrial disorder, which is likely behind a whole host of medical diseases, ME/CFS/Long Covid also has damage to the lymph system which causes it to flow backwards instead of forwards. This is why you react like you're constantly having an allergic reaction, and why exercise makes things worse, because your body is pumping stuff like lactic acid deeper into your muscles instead of out. As for why your heartbeat is so high, you are exhausted because you're full of chemicals so you're basically doing everything off of adrenaline instead of using your body's natural hormone system. So:
Reduce your toxin load. Change your diet, your toiletries, your laundry soap — whatever you're reactive to, and be aware that you're going to develop weird sensitivities and allergies because your immune system is overexposed to stuff. Meat, strawberries, plastic bottles, be hyper aware of what you're using.
See an acupuncturist. Most acupuncture sites are either directly on lymph nodes or lymph branches, and puncturing or even putting pressure on those sites can downregulate your immune reactions and can increase how fast your lymph heals by bringing reconstructive cells to the area (the same mechanism doctors use when administering glucose to torn muscles).
Go to a massage therapist trained in Manual Lymph Drainage (I'm biased for Vodder, even if they're a little culty) once to three times a week. Any kind of massage will help with lymph drainage, but in order to see improvement, you need light pressure specifically designed to move lymph in the right direction, which then creates a vortex effect that can keep the lymph moving in the right direction for up to a week but more realistically a couple of days.
Buy a body brush and do some self MLD at home. Dry brush your skin inside out (medial to lateral) and tips to heart. NOTE: DO NOT DO THIS IF YOU HAVE LYMPH NODES REMOVED; IT CAN CAUSE LYMPHEDEMA. You'll need to see a specialist for information on how to cross watersheds.
Try (just try) a ketogenic or intermittent fasting diet, which has been shown to heal mitochondria, but be wary of allergens, which restrictive and substitutionary diets can exacerbate.
Doing things where you sweat can reduce some toxins (such as heavy metals and excessive hormones (and you are basically living , but not things like chemicals), and since your skin is where most of your toxins are living because of how your body is currently pumping lymph, sweating can help immensely with detox and getting your adrenaline down. Steam rooms, saunas, and other heat therapies are your best choice because exercising is not it fam.
Avoid ice baths and other cold therapies until your lymph system regrows (10-20 years is typical, but you can accelerate it with MLD treatments). This is because you are compensating for your chronic illness by pushing through with adrenaline. If you downregulate your adrenals, as is the result of cold therapies, you will likely be more exhausted because you don't have your primary driving factor.
Avoid exercise, unless immediately following MLD, as exercise will stimulate lymph in the wrong direction. Also, I wouldn't recommend contrast bathing (cold to hot to cold to hot treatments) for this same reason; despite it being a useful treatment for edema, you are a special condition.
Basically, there really isn't anything you can do to fix it fast, but you can fix it faster and manage your condition until it heals. And then deal with the inevitable adrenal dysfunction.
Full Transcript at the link; 3-minute listen.
Quote:
By taking biopsies from long COVID patients before and after exercising, scientists in the Netherlands constructed a startling picture of widespread abnormalities in muscle tissue that may explain this severe reaction to physical activity.
Among the most striking findings were clear signs that the cellular power plants, the mitochondria, are compromised and the tissue starved for energy.
"We saw this immediately and it's very profound," says Braeden Charlton, one of the study's authors at Vrije University in Amsterdam.
The tissue samples from long COVID patients also revealed severe muscle damage, a disturbed immune response, and a buildup of microclots.
"This is a very real disease," says Charlton. "We see this at basically every parameter that we measure."
30K notes
·
View notes