#Biotechnology Advances
Explore tagged Tumblr posts
Text
Emerging Technologies of 2024: What's New and What's Next
Unveiling the Tech Wonders of Today and Tomorrow As we step further into 2024, the technological landscape continues to evolve at an unprecedented pace. Innovations once relegated to the realm of science fiction are now becoming realities, reshaping our lives in profound ways. In this article, we’ll explore some of the most groundbreaking technologies emerging this year and what we can…
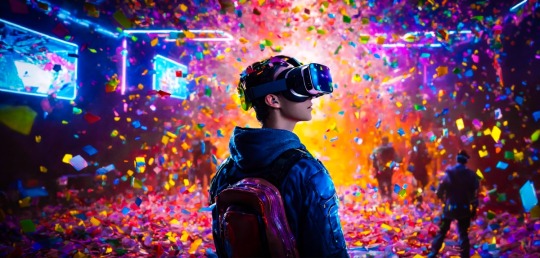
View On WordPress
#2024 Technology#5G Networks#AI Innovations#Augmented Reality#Biotechnology Advances#Emerging Technologies#Future Tech Trends#Internet of Things#Quantum Computing#Tech Industry Insights
0 notes
Text

69 notes
·
View notes
Note
girl what yoshiden fic is your favorite? i need recs.
anon you're lucky i'm in a magnanimous nicey mood, i was gonna gatekeep this fic till the end of time but here. i think there was a point in time where i would read this every single day just to study the writing... definitely one of the strangest things i've ever read. it feels exactly like a fever dream. the deep mundane insanity of an all-consuming crush... the author is so amazingly skilled and they have a few other fics i absolutely adore including this other yoshiden fic which is really more of a denji character study than it is a ship fic.
i've read other things in their tag and enjoyed them enough though not enough to recommend. once i'm in a yoshiden mood again i'll go through more of the tag. if you happen to read doujin, i highly suggest you read this one. though fair warning i'm super into eroguro type manga/doujin so if you get squicked out easily i'd skip it <3
#i don't think it's that bad but if ur not used to that kind of stuff it might be intense#idk tho i don't really have a gauge for these things anymore skdhfsf#that other fic by the author is an omegaverse fic WHICH I DIDN'T KNOW until i read a comment that mentioned it#like it was just that good & seamless that i didn't notice. i scrolled back up and there it was the fucking. abo dynamics tag#it might have been one of the first abo fics that i'd ever read in its entirety? i was telling my friend about it#i was like 'he kept taking these pills? maybe some advanced antidepressant biotechnology idk' CONSIDERING WHAT HE GOES THRU IN THE SERIES#that was when i learned what heat suppressant pills were#anyway have fun!!#please come back and tell me what you think <3
4 notes
·
View notes
Text
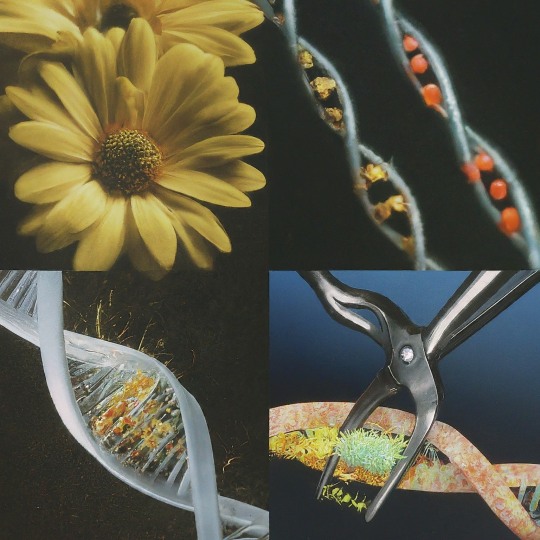
A snip, a splice : Power of rDNA Technology
Deoxyribonucleic acid (DNA), the blueprint of life, holds the secrets to the intricate workings of every living organism. But what if we could manipulate this blueprint, adding, removing, or tweaking its code? This revolutionary concept forms the core of recombinant DNA (rDNA) technology, a powerful tool that has transformed biology and medicine.
The story starts in the early 1970s with two brilliant scientists; Stanley Cohen at Stanford University and Herbert Boyer at the University of California, San Francisco. Cohen, a microbiologist, had been studying plasmids – small circular DNA molecules found in bacteria. Boyer, a biochemist, was an expert on restriction enzymes – molecular scissors that could cut DNA at specific sequences. Their collaboration proved groundbreaking. They envisioned combining these tools to create the first ever recombinant DNA molecule. Cohen provided the plasmids, which would act as vectors to carry foreign DNA into host cells. Boyer, on the other hand, used restriction enzymes to cut both the plasmid and the desired foreign DNA, allowing them to be pieced together. Through meticulous experimentation, they successfully created the first recombinant DNA molecule, forever altering the course of biology.
Cohen and Boyer's work wouldn't have been possible without the earlier discoveries of restriction enzymes. These "molecular scissors" were independently identified by three separate research groups in the 1960s. Werner Arber in Switzerland, along with Hamilton Smith and Daniel Nathans in the US, unraveled the role of restriction enzymes in bacterial defense mechanisms. These enzymes helped bacteria defend against invading viruses by cutting up their foreign DNA. Recognizing the potential of these "genetic scalpels," the groundwork was laid for their application in rDNA technology.
Here's a simplified breakdown of the rDNA process:
Isolation of DNA: The journey starts with isolating DNA from a donor organism.
Cleavage with Restriction Enzymes: Specific enzymes cut the DNA at defined sequences.
Selection of Vector: A carrier molecule (often a plasmid) is chosen to transport the recombinant DNA.
Ligation: The DNA fragments and vector are stitched together using DNA ligase, an enzyme.
Transformation: The recombinant DNA enters a host cell (usually bacteria or yeast).
Selection and Expression: The transformed cells are selected, and the gene of interest is expressed, leading to the desired protein production.
Since its inception, rDNA technology has played a pivotal role in several groundbreaking advancements. Let's take a whirlwind tour through some of the most significant moments in R-DNA history:
1978: Birth of Insulin on the Factory Floor: Scientists achieved a feat of genetic engineering by using R-DNA to produce human insulin in bacteria. This marked a turning point for diabetics, offering a readily available and more consistent source of this life-saving hormone.
1980s: Gene Wars and the Rise of GMOs: The 1980s saw the development of genetically modified organisms (GMOs). Plants were engineered with genes for insect resistance or herbicide tolerance, sparking debates about the safety and ethics of this technology. R-DNA research continues to be at the forefront of discussions regarding genetically modified foods.
1990s: The Human Genome Project Sets Sail: This ambitious international project aimed to sequence the entire human genome. R-DNA techniques played a crucial role in deciphering the 3 billion letters of our genetic code, opening doors for personalized medicine and a deeper understanding of human health and disease.
2000s: Gene Therapy Takes Center Stage: The first successful gene therapy trials for inherited diseases like severe combined immunodeficiency (SCID) took place. R-DNA technology offered a glimmer of hope for treating genetic disorders by introducing healthy genes to replace defective ones.
2010s and Beyond: CRISPR Takes Over: The emergence of CRISPR-Cas9, a revolutionary gene editing tool based on R-DNA principles, has ushered in a new era of genetic manipulation. With unprecedented precision, scientists can now edit genes in various organisms, holding immense potential for gene therapy, crop improvement, and even the eradication of diseases.
But with great power comes great responsibility, and R-DNA raises a host of ethical concerns.Tinkering with the building blocks of life carries the risk of unintended consequences. Engineered genes could escape and disrupt ecosystems, or modified organisms could have unforeseen health effects. The ability to edit human genes opens the door to designer babies, raising questions about social equity and the potential misuse of the technology for eugenics.
Who Controls the Tools? Access to R-DNA technology could be restricted to wealthy nations or corporations, exacerbating existing inequalities. Biosecurity is also a concern, as the technology could be misused for bioterrorism. Creating entirely new organisms forces us to confront what it means to be "natural." Should we modify plants and animals for human benefit, or preserve their original forms? R-DNA technology is a powerful tool, and we must have open discussions about its ethical implications. Scientists, policymakers, and the public all need to be involved in shaping the future of this technology. As we move forward, open dialogue and collaboration between scientists, policymakers, and the public are crucial to ensure the safe and ethical application of this powerful technology.
The journey of rDNA technology is a testament to human ingenuity and its potential to reshape our world. From decoding the secrets of life to creating solutions for healthcare, agriculture, and beyond, rDNA technology continues to evolve, promising a future filled with exciting possibilities.
#science sculpt#life science#science#molecular biology#biology#biotechnology#artists on tumblr#dna#double helix#genetics#recombinant#genetic engineering#insulin#research#education#learning#academics#scientific research#scientific illustration#medical science#scifi#daily dose of science#scientific advancements#scientific tools#medical school
17 notes
·
View notes
Text
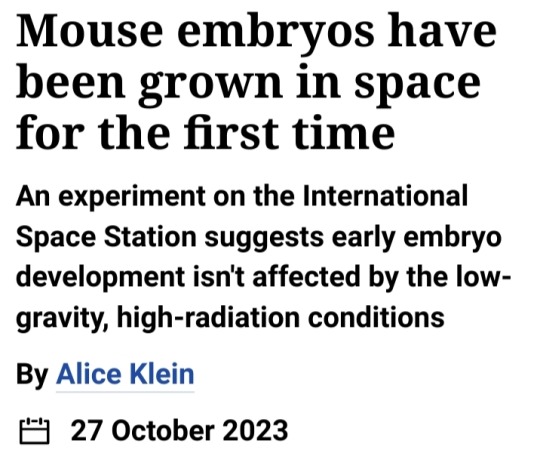
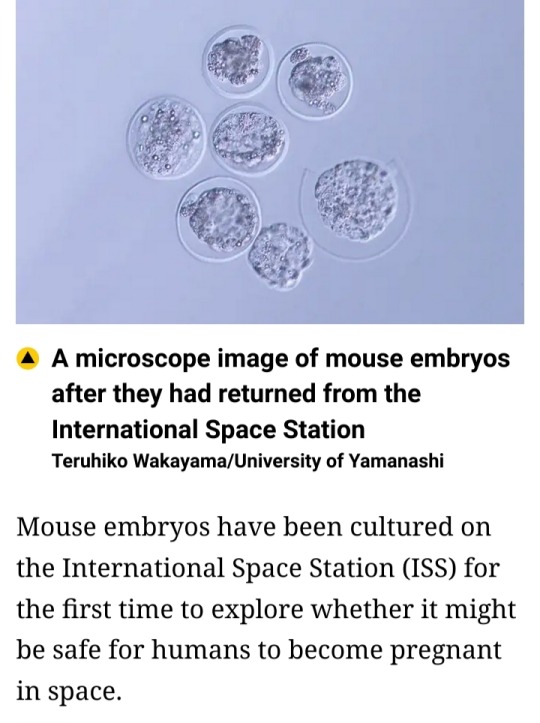
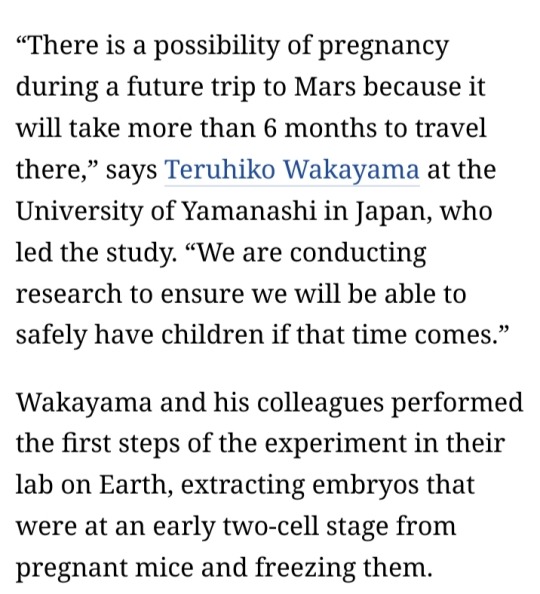
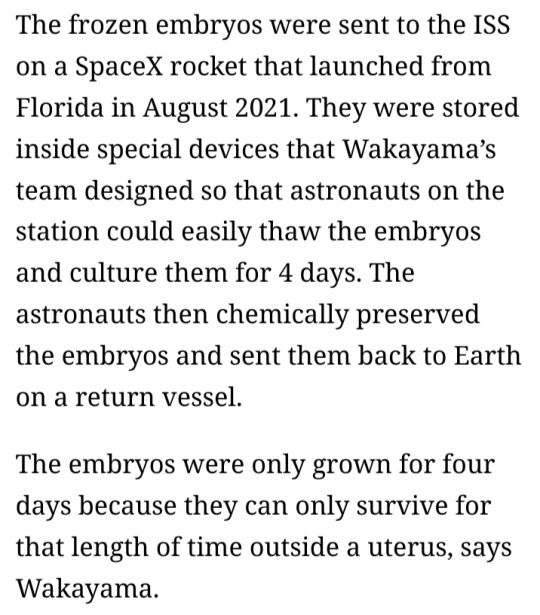
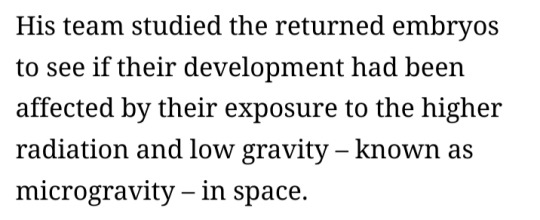
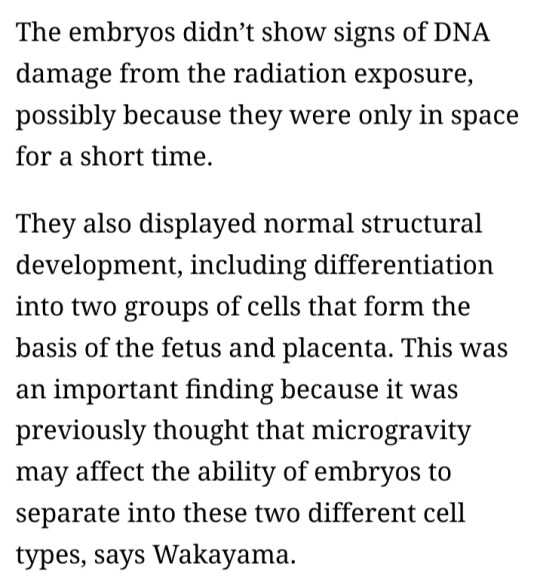
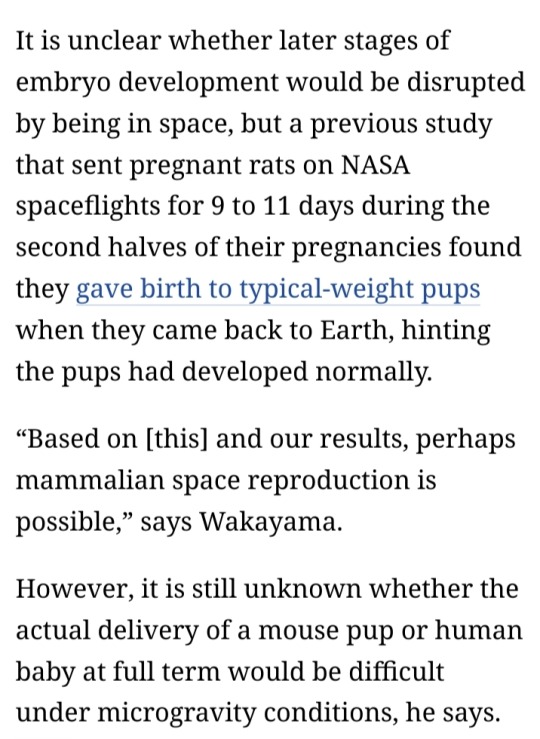
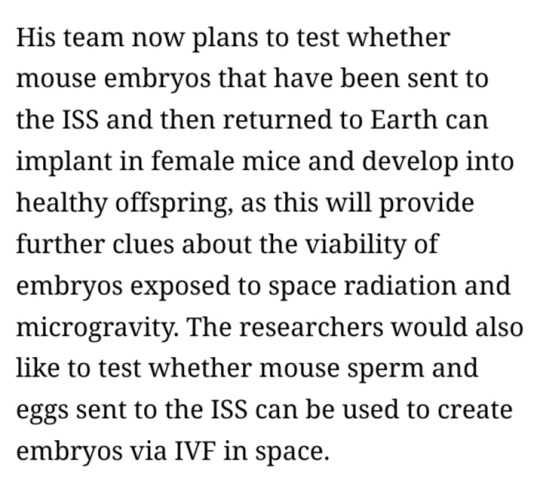
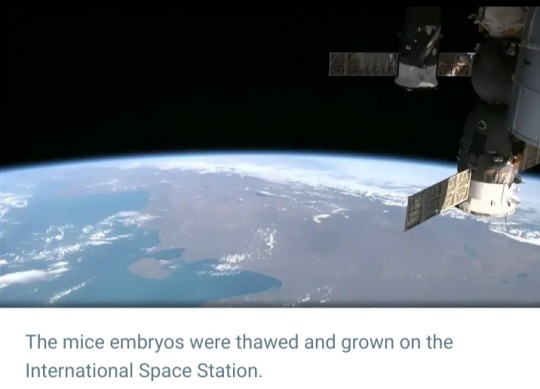
#International Space Station (ISS)#ISS#mouse embryos#Teruhiko Wakayama#University of Yamanashi#Japan#SpaceX#frozen embryos#microgravity#space#research#experiment#space radiation#astronauts#embryo development#Japan Aerospace Space Agency (JAXA)#JAXA#Advanced Biotechnology Centre#space exploration#colonization mission#NASA#gravity
2 notes
·
View notes
Text
The White Biotechnology Market is projected to reach $672.92 Billion by 2034 from $317.21 Billion in 2024, growing at a CAGR of 7.97% during the forecast period 2025-2034.
#White Biotechnology Market#White Biotechnology Report#White Biotechnology Industry#Advanced Materials#BISResearch
0 notes
Text
Infectious Disease Diagnostics in India: Addressing Emerging Threats
In recent years, India’s healthcare system has witnessed a remarkable transformation, particularly in the area of infectious disease diagnostics. The rising burden of communicable diseases such as tuberculosis, dengue, malaria, hepatitis, and emerging viral outbreaks like COVID-19 has pushed the country’s medical and scientific community to adopt more accurate and timely diagnostic solutions. At the forefront of this progress is the biotechnology diagnostics sector in India, which integrates innovative tools like molecular testing, genetic sequencing, and advanced laboratory infrastructure to tackle both existing and emerging health threats.
The Need for Precision in Infectious Disease Diagnostics
India’s diverse geography and dense population make it highly susceptible to infectious disease outbreaks. Early detection and precise diagnosis are essential not just for effective treatment but also for breaking the chain of transmission. Traditional diagnostic methods such as microscopy and culture techniques are time-consuming and sometimes less reliable. This is where biotechnology labs in India step in to revolutionize the approach by offering faster, more sensitive, and accurate solutions.
Rise of Molecular Diagnostics in India
Molecular diagnostics labs in India are playing a pivotal role in identifying pathogens at the genetic level. Techniques such as Polymerase Chain Reaction (PCR), Real-Time PCR (RT-PCR), and Nucleic Acid Amplification Tests (NAATs) allow for the early detection of viruses and bacteria, even in low concentrations. These technologies not only speed up the diagnostic process but also enhance specificity and sensitivity, which is crucial for diseases with overlapping symptoms.
As a result, hospitals, public health organizations, and private diagnostic centers are increasingly integrating molecular testing into their routine infectious disease screening protocols.
Genetic Testing Labs and Their Contribution
Another breakthrough in disease diagnostics comes from the emergence of genetic testing labs in India. These labs delve deeper into the human genome and microbial DNA to understand susceptibility to infections, monitor pathogen mutations, and even detect resistance to treatments. For example, genetic tests are now being used to assess drug-resistant tuberculosis (DR-TB) and HIV genotyping, helping clinicians design personalized treatment strategies.
Moreover, advanced genetic testing services in India are aiding epidemiological surveillance by tracking disease-causing organisms’ mutation patterns. This is particularly important in managing pandemic threats where the pathogen is rapidly evolving.
The Role of DNA Sequencing Services
The advancement of DNA sequencing services in India has opened new doors for clinical diagnostics. Next-generation sequencing (NGS), whole-genome sequencing, and metagenomic sequencing are some of the tools helping researchers and clinicians identify rare pathogens and understand disease outbreaks at a molecular level. These technologies are vital during unexplained disease outbreaks where standard tests fail to provide answers.
Private biotech companies and government research institutes are collaborating to expand sequencing capabilities and build a strong disease surveillance network across the country.
Biotechnology Labs in India: Bridging Innovation and Implementation
The backbone of these technological advancements is a growing network of biotechnology labs in India. Equipped with cutting-edge tools, these labs are not just diagnosing diseases but also conducting critical research to develop new assays, discover biomarkers, and enhance point-of-care diagnostic tools.
India’s biotech ecosystem includes startups, research institutes, and academic collaborations that are continually developing innovative diagnostic kits tailored to the country’s unique needs. These include rapid antigen tests, CRISPR-based diagnostics, and portable molecular testing devices that can be deployed in rural and remote areas.
Bangalore – India’s Biotechnology Capital
When we speak of biotechnology in India, Bangalore undeniably stands out. Often dubbed the "Silicon Valley of India," Bangalore is also the country’s biotechnology capital. The city is home to a concentration of biotechnology companies, research hubs, and diagnostic startups that are making significant contributions to global health.
A typical biotechnology company in Bangalore engages in a wide range of activities, from infectious disease research to developing cutting-edge diagnostic tools and collaborating on global pandemic preparedness initiatives. These companies play a central role in translating lab-based research into market-ready solutions that can be scaled across the country.
Government Initiatives and Public-Private Partnerships
The Indian government has recognized the importance of rapid diagnostics in combating infectious diseases and has launched several initiatives under schemes like National Health Mission (NHM) and Ayushman Bharat. Investments are being made in expanding diagnostic infrastructure, training healthcare workers, and encouraging public-private partnerships to reach underserved populations.
Furthermore, collaboration between Indian biotech firms and international organizations such as WHO and CDC has helped in creating a robust disease surveillance and response framework.
The Road Ahead
As India continues to tackle a wide spectrum of infectious diseases, the importance of fast, reliable, and scalable diagnostics cannot be overstated. The combination of biotechnology diagnostics in India, molecular diagnostics labs, DNA sequencing services, and genetic testing labs is enabling a future where diagnosis is no longer reactive but proactive.
The integration of artificial intelligence and digital platforms with diagnostics is further set to enhance the capabilities of biotechnology labs in India, making them more accessible and efficient. Bangalore’s thriving biotech community will continue to be a major driver in this progress, pushing India toward becoming a global leader in infectious disease diagnostics.
Conclusion
India is at a critical juncture in public health. With infectious diseases posing both traditional and novel challenges, the role of biotechnology, genetic testing, and molecular diagnostics becomes indispensable. Through cutting-edge biotechnology diagnostics in India, a robust network of biotechnology labs, and the innovation-driven spirit of biotechnology companies in Bangalore, the country is well-equipped to address current and future health threats with confidence and precision.
#biotechnology#biotechnology lab#dna sequencing#advanced genetic testing#molecular biology#molecular diagnostics
0 notes
Text
Revolutionary Anti-Aging Drug for Dogs Launching by 2026
Revolutionary Anti-Aging Drug for Dogs Launching by 2026 - #DogHealth #AgingDogs #LOY001 #PetWellness #VeterinaryScience #DogLovers #AntiAging #PetCare #HealthyPets #DogLife #CognitiveHealth #PetOwnership #ScienceForPets
The Future of Canine Longevity Imagine a world where your dog ages gracefully—where those energetic zoomies, enthusiastic tail wags, and joyous leaps don’t diminish with the passage of time. All dog lovers understand the heartache of witnessing their loyal companion’s decline, as the once-vibrant energy wanes, replaced by aching joints and graying fur. For centuries, we have accepted the notion…
#aging in dogs#anti-aging drug#biotechnology#canine longevity#Dog Health#dog lifespan#ethical implications#health advancements#humane treatment#IGF-1#longevity research#LOY-001#Pet Care#pet ownership#veterinary medicine
0 notes
Text
Navigating the Era of Mental Transparency
#EmpoweredJourney#Resilience#SelfAwareness#AI advancements#authenticity#biotechnology#collective thought#emotional intelligence#empathy#Hafsa Reasoner#human interactions#mental communication#mental transparency#Mindfulness#online connections#open minds#overcoming challenges#personal boundaries#Personal growth#psychological exploitation#radical compassion#Self-Awareness#self-judgment#social justice#transformative change
1 note
·
View note
Text
Brainoware: The Hybrid Neuromorphic System for a Brighter Tomorrow
A glimpse into the double-edged nature of Brain Organoid Reservoir Computing, with the pros/cons of this biological computing approach From a young age, I was captivated by the mysteries of science and the promise of technology, wondering how they could shape our understanding of the world. I was fortunate to receive STEM education early on in a specialized school, where my creativity and…
#Artificial Intelligence (AI) in Healthcare#Biocomputing and Drug Testing#Biocomputing Applications#Biodigital Transformation#Biological Computing#Brain Organoid Reservoir Computing (BORC)#Brain-Machine Interfaces#Brainoware Research#Brainoware: The Hybrid Neuromorphic System#Cognitive Science Advances#Ethics in Biotechnology#Future of Drug Testing#Healthcare Transformation#Hybrid Neuromorphic Systems#Literature Reviews by Dr Mehmet Yildiz#Medicine 3.0#Mental Health and Behavioral Science#Neurocomputing and Neurobiology Advances#NeuroHIV and cognitive decline#neuroinflammation#Neurological Disorder Research#Neuroplasticity and Learning#neurorehabilitation#Neuroscience Innovations#Organoid Intelligence#Understanding neural circuitry
1 note
·
View note
Text
Transhumanism: The Future of Human Enhancement
Transhumanism envisions a future where humanity can transcend its current physical and mental limitations through the use of advanced technologies.
Transhumanism and the Connection Between Human Consciousness and Artificial Intelligence Transhumanism envisions a future where humanity can transcend its current physical and mental limitations through the use of advanced technologies. The central belief of transhumanism is that humanity not only can but should pursue certain objectives to shape its future. However, there are differing…
#ARTICLES#artificial intelligence#biotechnological advancement#cognitive existence#Collections#digital system#disease eradication#enhancement#general artificial intelligence#genetic modification#human consciousness#human evolution#immortality#life extension#machine intelligence#mind uploading#Rodrigo Granda#singularity#strong AI#suffering alleviation#technological progress#transhumanism
0 notes
Text
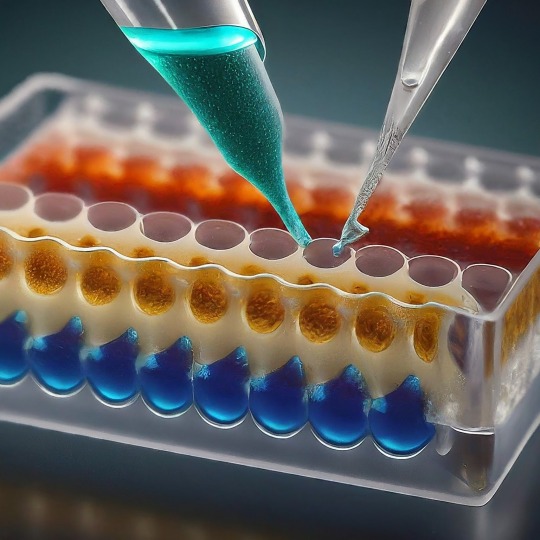
ELISA: A Powerful Tool for Detecting the Invisible
ELISA, or Enzyme-Linked Immunosorbent Assay, has become a cornerstone of medical diagnostics and biological research. This versatile technique allows scientists to detect and quantify minute amounts of target molecules, such as proteins, antibodies, and even viruses, with remarkable accuracy. In this blog, we'll delve into the world of ELISA, exploring its various types, its applications, and the exciting future directions this technology holds.
At its core, ELISA relies on the exquisite specificity of antibodies. Antibodies are highly specialized proteins produced by the immune system in response to foreign invaders. Each antibody can bind to a unique structure, called an antigen, on a specific molecule. In an ELISA, scientists leverage this binding property to create a sensitive detection system.
The 1960s witnessed a surge in interest in immunoassays, techniques that utilize the specificity of antibodies to detect target molecules. One such technique, radioimmunoassay (RIA), developed by Rosalyn Yalow and Solomon Berson, revolutionized medical diagnostics. RIA used radioactively labeled antibodies to detect antigens, offering high sensitivity. However, concerns regarding the safety of radioactive materials fueled the search for a safer alternative. The year 1971 marked a turning point. Independently, Eva Engvall and Peter Perlmann published their work on a novel technique – the enzyme-linked immunosorbent assay (ELISA). ELISA replaced radioactive labels with enzymes, eliminating the safety concerns associated with RIA. Like RIA, ELISA harnessed the specific binding between antibodies and antigens. However, it employed enzymes that could generate a detectable signal, such as a color change, upon interacting with a substrate. This innovation paved the way for a safer and more user-friendly diagnostic tool.
The basic ELISA protocol involves immobilizing the target antigen on a solid surface like a plate well. Then, a sample containing the molecule of interest (e.g., a suspected virus) is introduced. If the target molecule is present, it will bind to the immobilized antigen. Next, an antibody specific to the target molecule, linked to an enzyme, is introduced. This "detection antibody" binds to the target molecule already attached to the antigen. Finally, a substrate specific to the enzyme is added. This antigen-antibody binding is visualized using an enzyme linked to a reporter molecule. When the enzyme encounters its substrate, a detectable signal is produced, such as a color change or luminescence. The intensity of this signal is directly proportional to the amount of antigen present in the sample, allowing for quantification. The beauty of ELISA lies in its adaptability. Several variations exist, each tailored for specific detection needs.
The Four Main ELISA Formats are:
Direct ELISA: Simplicity at its finest. In this format, the antigen is directly coated onto the ELISA plate. A labeled antibody specific to the antigen is then introduced, binding directly to its target. After washing away unbound molecules, the enzyme linked to the antibody generates a signal upon addition of the substrate. Direct ELISA offers a rapid and straightforward approach, but sensitivity can be lower compared to other formats due to the lack of amplification.
Indirect ELISA: Unveiling the Power of Amplification. Similar to the direct ELISA, the antigen is first coated onto the plate. However, instead of a labeled primary antibody, an unlabeled one specific to the antigen is used. This is followed by the introduction of a labeled secondary antibody that recognizes the species (e.g., mouse, rabbit) of the primary antibody. This two-step approach acts as an amplification strategy, significantly enhancing the signal compared to the direct ELISA. However, the presence of an extra incubation step and the potential for cross-reactivity with the secondary antibody add complexity.
Sandwich ELISA: Capturing the Antigen Between Two Antibodies. Here, the capture antibody, specific for one region of the antigen, is pre-coated onto the ELISA plate. The sample containing the antigen is then introduced, allowing it to be "sandwiched" between the capture antibody and a detection antibody specific for a different region of the same antigen. A labeled secondary antibody or a labeled detection antibody itself can then be used to generate the signal. Sandwich ELISA boasts high sensitivity due to the double-antibody recognition and is often the preferred format for quantifying analytes.
Competitive ELISA: A Race for Binding Sites. In this format, the antigen competes with a labeled antigen (usually a known amount) for binding sites on a capture antibody pre-coated onto the plate. The more antigen present in the sample, the less labeled antigen can bind to the capture antibody. Following a washing step, the amount of bound labeled antigen is measured, providing an inverse relationship between the signal and the concentration of antigen in the sample. Competitive ELISA is particularly useful for studying small molecules that may be difficult to directly conjugate to an enzyme.
ELISA's Reach: From Diagnostics to Research. The applications of ELISA are as vast as they are impressive. Let's delve into some key areas where ELISA plays a vital role:
Unveiling the Mysteries of Disease: Diagnostics: ELISA is a cornerstone of diagnosing infectious diseases like HIV, Hepatitis, and Lyme disease. It detects antibodies produced by the body in response to the invading pathogen, providing valuable information for early detection and treatment. Monitoring Autoimmune Diseases: ELISA helps monitor autoimmune diseases like rheumatoid arthritis and lupus by measuring specific antibodies associated with these conditions. Cancer Screening: Certain cancers can be detected by identifying tumor markers, proteins elevated in the blood of cancer patients. ELISA assays are being developed to detect these markers for early cancer screening.
Safeguarding Food Quality: Allergen Detection: Food allergies can be life-threatening. ELISA ensures food safety by enabling the detection of allergens like peanuts, gluten, and milk in food products, protecting consumers with allergies. Monitoring Foodborne Pathogens: ELISA can identify harmful bacteria, viruses, and toxins in food, preventing outbreaks of foodborne illnesses.
Environmental Monitoring: Pollutant Detection: ELISA can detect pollutants like pesticides and herbicides in water and soil samples, contributing to environmental protection efforts. Microbial Analysis: This technique can be used to identify and quantify specific microbes in environmental samples, providing insights into ecosystem health.
Research and Development: ELISA plays a crucial role in various research fields: Drug Discovery: It helps researchers assess the effectiveness of new drugs by measuring drug-target interactions and monitoring drug levels in the body. Vaccine Development: ELISA is instrumental in developing vaccines by evaluating immune responses to vaccine candidates. Basic Research: Scientists use ELISA to study various biological processes by detecting and quantifying specific molecules involved in these processes.
Despite its established role, ELISA is evolving alongside technological advancements. New multiplex platforms allow for the simultaneous detection of various targets in a single sample, boosting efficiency in biomarker discovery and disease analysis. Automation streamlines workflows minimizes errors, and increases throughput, making high-throughput screening feasible in drug development and clinical settings. Miniaturization and portable devices enable rapid on-site diagnostics, providing healthcare professionals with real-time data for quicker interventions. Additionally, ongoing research is improving assay sensitivity, reducing background noise, and expanding detection limits, allowing for the identification of trace analytes and early disease biomarkers with greater accuracy than ever before. Integration of ELISA with emerging technologies such as microfluidics, nanotechnology, and artificial intelligence holds promise for enhancing assay performance, scalability, and data analysis capabilities.
These advancements hold promise for even wider applications of ELISA in the future. ELISA has revolutionized our ability to detect and quantify biological molecules. Its versatility, accuracy, and adaptability make it an invaluable tool across various scientific disciplines. As research continues to refine and innovate ELISA techniques, we can expect even more exciting possibilities to emerge in the years to come. ELISA's future is bright, promising to play a pivotal role in unraveling the mysteries of the biological world and improving human health.
#science sculpt#life science#molecular biology#science#biology#artists on tumblr#ELISA#immunology#immunotherapy#diagnostic management software#diagnosticimaging#history of immunology#scientific advancements#biotechnology#scientific research#scientific equipment#scientific instruments#techniques in biotechnology#scientific illustration#lab equipment#sciencenature#laboratory#lab skills#molecular diagnostics market
11 notes
·
View notes
Text
Revolutionary Tech Innovations Shaping Our Future
Introduction
Technology is advancing rapidly, revolutionizing our lifestyles, workplaces, and interactions with the world. From artificial intelligence (AI) and quantum computing to renewable energy and biotechnology, revolutionary tech innovations are shaping our future in unprecedented ways. In this blog post, we will explore some of the most groundbreaking technological advancements and their potential to reshape our lives. Read to continue
#Innovation Insights#TagsAI advancements#AI in education#AI in healthcare#AR applications#augmented reality#biotech in healthcare#biotechnology breakthroughs#future of technology#IoT applications#IoT in smart cities#quantum computing#quantum computing benefits#renewable energy future#renewable energy technology#revolutionary tech innovations#smart cities#tech advancements#virtual reality#Technology#Science#business tech#Adobe cloud#Trends#Nvidia Drive#Analysis#Tech news#Science updates#Digital advancements#Tech trends
1 note
·
View note
Text
Advanced Biomanufacturing Solutions: How Biofabrication is Meeting the Need for Complex Biologics in the Biotechnology Industry
Biofabrication Market: A Growing Industry with Promising Future
Biofabrication is an emerging technological field that focuses on manufacturing structures for tissue engineering and regenerative medicine. This field has gained significant attention in recent years due to its potential to revolutionize the healthcare industry. In this article, we will explore the biofabrication market, its size, growth projections, and the key factors driving its growth.
Market Size and Growth Projections
The biofabrication market is expected to grow significantly in the coming years. According to a report by Intent Market Research, the global biofabrication market size is anticipated to reach USD 80 Billion by the end of 2036, growing at a CAGR of 10% during the forecast period, i.e., 2024-2036. Another report estimates the market size to be USD 22.76 Billion in 2023 and predicts it to reach USD 48.27 Billion by 2031, with a CAGR of 10.2% from 2024 to 2031.
Key Factors Driving Growth
Several factors are driving the growth of the biofabrication market. One of the primary factors is the increasing demand for biologics and biosimilars. The development of biologics accounts for around 40% of all pharmaceutical R&D spending, making it essential for advancing healthcare. Additionally, biomanufacturing accelerates scientific research, boosts economic growth, and provides jobs, making it a vital industry for the future.
Another key factor driving growth is the advancements in biofabrication techniques. Recent advancements in volumetric bioprinting, scaffold-free bioassembly, and hybrid biofabrication strategies have improved the scale, rate, and intricacy at which tissues can be fabricated. These advancements have the potential to recapitulate the structure and complexity of native tissues, making them suitable for various applications such as biomimetically engineered models for drug discovery, cosmetics testing, tissue regeneration, and medical devices.
Regional Analysis
The biofabrication market is expected to grow significantly in various regions. North America is expected to hold the largest share of 36% during the forecast period due to the robust presence of large biopharmaceutical businesses and the improved infrastructure in biological research. The Asia-Pacific region is expected to witness the fastest growth due to the rapid adoption of advanced technologies and the high prevalence of chronic diseases.
Competitive Landscape
The biofabrication market is highly competitive, with several key players operating in the market. Some of the major players include Applikon Biotechnology BV, bbi-biotech GmbH, Danaher Corporation, Eppendorf AG, Esco Group of Companies, GEA Group Aktiengesellschaft, Meissner Filtration Products, Inc., Merck KGaA, PBS Biotech, Inc., Pierre Guérin, Sartorius AG, Shanghai Bailun Biotechnology Co. Ltd., Solaris Biotechnology Srl., Thermo Fisher Scientific Inc., and ZETA GmbH.
Conclusion
In conclusion, the biofabrication market is expected to grow significantly in the coming years due to the increasing demand for biologics and biosimilars, advancements in biofabrication techniques, and the growing demand for advanced biomanufacturing solutions. The market is expected to be driven by key players operating in the market, and regional growth is expected to vary. As the industry continues to evolve, it is essential to stay updated on the latest advancements and trends to capitalize on the growth opportunities in the biofabrication market.
#Biofabrication#biomanufacturing#biologics#biosimilars#regenerative medicine#tissue engineering#biomimetic engineering#biotechnology#biopharmaceutical industry#advanced biomanufacturing solutions#volumetric bioprinting#scaffold-free bioassembly#hybrid biofabrication strategies#biomanufacturing techniques#biomanufacturing market
0 notes
Text
Sci-Fi to Reality: Mind-Blowing Innovations Transforming Our World
Science fiction has long been a source of inspiration for technological innovation, with many once-fanciful ideas now becoming reality. From space travel to artificial intelligence, the line between science fiction and reality is becoming increasingly blurred. In this article, we'll explore some of the most mind-blowing innovations that were once the stuff of science fiction but are now transforming our world.
Space Travel and Exploration
One of the most iconic images of science fiction is that of humans traveling through space, exploring distant planets and galaxies. While we may not yet have achieved the level of space travel depicted in movies and books, significant strides have been made in recent years. Private companies like SpaceX and Blue Origin are pioneering reusable rocket technology, making space travel more accessible and affordable. Meanwhile, robotic missions to Mars and beyond are expanding our understanding of the universe and laying the groundwork for future manned missions.
Artificial Intelligence (AI) and Robotics
Artificial intelligence (AI) and robotics are transforming nearly every aspect of our lives, from the way we work to how we interact with technology. AI-powered assistants like Siri and Alexa have become ubiquitous, helping us navigate our daily lives and access information with ease. In industries like healthcare, AI is revolutionizing diagnosis and treatment, while in manufacturing, robots are increasing efficiency and productivity. As AI continues to advance, the possibilities for its application are virtually limitless.
Virtual and Augmented Reality
Virtual reality (VR) and augmented reality (AR) are no longer just the stuff of science fiction—they are increasingly becoming part of our everyday lives. VR technology allows us to immerse ourselves in virtual worlds, whether for entertainment, education, or training. AR, on the other hand, overlays digital information onto the real world, enhancing our perception of reality. From gaming and design to healthcare and education, VR and AR are transforming how we interact with the world around us.
Biotechnology and Genetics
Advances in biotechnology and genetics are revolutionizing healthcare and agriculture, offering new ways to treat diseases and improve crop yields. CRISPR gene editing technology, for example, allows scientists to precisely edit the genetic code of organisms, opening up new possibilities for treating genetic diseases and developing genetically modified crops. In healthcare, personalized medicine is becoming a reality, with treatments tailored to individual genetic profiles.
Conclusion
The innovations that were once the realm of science fiction are now becoming reality, transforming our world in ways we never thought possible. From space travel and artificial intelligence to virtual reality and biotechnology, these advancements are reshaping how we live, work, and interact with the world around us. As we continue to push the boundaries of what is possible, the future promises to be even more exciting and trans-formative.
#technology#biotechnology#science fiction#artificial intelligence#vr technology#rocket technology#technological advancements#technological innovation#ar and vr
0 notes
Text
Useful Tips for Becoming a Successful Agriculture Investor
Agriculture investment refers to the allocation of financial resources, capital, or assets into various aspects of the agricultural sector with the expectation of generating a return on investment (ROI). This could mean investing monies in agriculture land for sale such as coconut land for sale in Sri Lanka, or other types of investments. It involves deploying funds in activities and projects related to agriculture for the purpose of profit, income generation, or long-term wealth creation. Agriculture investment can take many forms, including:
Farmland Acquisition: Purchasing agricultural land for the cultivation of crops or the raising of livestock. This can involve both large-scale and small-scale farming operations.
Infrastructure Development: Investing in the construction and improvement of infrastructure such as irrigation systems, roads, storage facilities, and processing plants to enhance agricultural productivity and efficiency.
Technological Advancements: Funding the development and adoption of agricultural technologies, such as precision agriculture, automation, and biotechnology, to improve crop yields and reduce operational costs.
Agribusiness Ventures: Investing in agribusinesses, such as food processing, distribution, and marketing, that are part of the agricultural value chain.
Research and Development: Supporting research initiatives related to agriculture to develop new crop varieties, pest-resistant strains, and sustainable farming practices.
Input Supply: Investing in the production and distribution of agricultural inputs like seeds, fertilisers, pesticides, and machinery.
Commodity Trading: Speculating on the future prices of agricultural commodities, such as grains, oilseeds, and livestock, through commodity markets or futures contracts.
Sustainable Agriculture: Funding practices and projects aimed at sustainable and environmentally responsible farming methods, which can include organic farming, agroforestry, and conservation efforts.
Rural Development: Supporting initiatives that improve the overall economic and social well-being of rural communities, often through investments in education, healthcare, and infrastructure.
Venture Capital and Start-ups: Investing in start-ups and companies focused on innovations in agriculture, such as vertical farming, aquaculture, or agricultural technology (AgTech).
Agriculture investment is important for food security, economic development, and job creation in many regions. However, it also comes with risks related to weather conditions, commodity price fluctuations, and market dynamics. Investors often conduct thorough research and risk assessments before committing their resources to agricultural ventures. Additionally, they may need to consider factors like government policies, environmental regulations, and social impacts on their investment decisions in the agricultural sector.
How to become a successful agriculture investor
Becoming a successful agriculture investor requires a combination of financial acumen, agricultural knowledge, and a strategic approach to investment. Here are some steps to help you become a successful agriculture investor:
Educate Yourself: Gain a strong understanding of the agricultural sector, including the different sub-sectors (crops, livestock, agribusiness, etc.). Stay updated on industry trends, market conditions, and emerging technologies.
Set Clear Investment Goals: Define your investment objectives, whether it is long-term wealth creation, income generation, or diversification of your investment portfolio.
Risk Assessment: Understand and assess the risks associated with agriculture investments, such as weather-related risks, market volatility, and regulatory changes, whether you are looking at land for sale or any other type of investment.
Develop a Diversified Portfolio: Diversify your investments across different agricultural sectors and geographic regions to spread risk.
Market Research: Conduct thorough market research to identify promising investment opportunities and potential demand for agricultural products.
Build a Network: Establish connections with farmers, agricultural experts, government agencies, and industry stakeholders who can provide insights and opportunities.
Financial Planning: Create a budget and financial plan that outlines your investment capital, expected returns, and cash flow requirements.
Select the Right Investment Type: Choose the type of agriculture investment that aligns with your goals, whether it is farmland, agribusiness ventures, or agricultural technology.
Due Diligence: Conduct comprehensive due diligence on potential investments, including assessing the quality of farmland, the financial health of agribusinesses, and the technology's potential for scalability and profitability.
Sustainable Practices: Consider investments in sustainable and environmentally responsible agriculture practices, as they are gaining importance in the industry.
Risk Management: Implement risk management strategies, such as insurance, to protect your investments from unforeseen events like natural disasters or crop failures.
Continuous Learning: Stay informed about changes in the agricultural industry and adapt your investment strategy accordingly.
Legal and Regulatory Compliance: Understand and comply with local, national, and international regulations and tax laws that may impact your agriculture investments.
Monitor and Adjust: Regularly review the performance of your investments and be prepared to make adjustments or exit underperforming ones.
Long-Term Perspective: Agriculture investments often require a long-term perspective, so be patient and avoid making impulsive decisions based on short-term market fluctuations.
Seek Professional Advice: Consult with financial advisors, agricultural experts, and legal professionals to ensure that your investments are structured and managed effectively.
Successful agriculture investment often involves a mix of financial expertise, industry knowledge, and a willingness to adapt to changing conditions. It is important to approach agriculture investment with a well-thought-out strategy, and to be prepared for both opportunities and challenges in this sector.
#Agriculture investment refers to the allocation of financial resources#capital#or assets into various aspects of the agricultural sector with the expectation of generating a return on investment (ROI). This could mean#or other types of investments. It involves deploying funds in activities and projects related to agriculture for the purpose of profit#income generation#or long-term wealth creation. Agriculture investment can take many forms#including:#●#Farmland Acquisition: Purchasing agricultural land for the cultivation of crops or the raising of livestock. This can involve both large-sc#Infrastructure Development: Investing in the construction and improvement of infrastructure such as irrigation systems#roads#storage facilities#and processing plants to enhance agricultural productivity and efficiency.#Technological Advancements: Funding the development and adoption of agricultural technologies#such as precision agriculture#automation#and biotechnology#to improve crop yields and reduce operational costs.#Agribusiness Ventures: Investing in agribusinesses#such as food processing#distribution#and marketing#that are part of the agricultural value chain.#Research and Development: Supporting research initiatives related to agriculture to develop new crop varieties#pest-resistant strains#and sustainable farming practices.#Input Supply: Investing in the production and distribution of agricultural inputs like seeds#fertilisers#pesticides#and machinery.
1 note
·
View note