#engineer hydrocarbon
Explore tagged Tumblr posts
Text
The Science Behind Mix Hydrocarbon Oil: A Comprehensive Guide
In the realm of industrial lubricants and fuels, mix hydrocarbon oil plays a crucial role. Its versatility and effectiveness make it a key player in various applications, from engine lubrication to industrial processes. In this comprehensive guide, we'll explore the science behind hydrocarbon oils, including their composition, properties, applications and environmental considerations.

What is Mix Hydrocarbon Oil?
Mix hydrocarbon oil is derived from the refining of crude oil, and it consists of a blend of different hydrocarbons. These hydrocarbons can be categorized into two main types: aliphatic and aromatic.
Aliphatic hydrocarbons include straight-chain (paraffin) and branched (iso-paraffins) hydrocarbons. They are typically less reactive and have higher stability.
Aromatic hydrocarbons are cyclic compounds that contain one or more benzene rings. They tend to have unique properties, such as higher boiling points and greater stability under heat, making them useful in certain applications.
Composition
The composition of mix hydrocarbon oil can vary significantly based on its intended use and the source of the crude oil. The refining process includes distillation, cracking, and treating to separate and convert various hydrocarbon components into usable products. Additives are often included to enhance performance characteristics, such as:
Detergents: Help keep engines clean by preventing the accumulation of deposits.
Anti-wear agents: minimize friction and wear on metal surfaces.
Viscosity index improvers: Ensure the oil retains its thickness across varying temperatures.
Properties
The performance of mix hydrocarbon oil is largely dictated by their physical and chemical properties, which include:
Viscosity: A quantification of a fluid's ability to resist movement. The viscosity of oil can change with temperature; thus, oils with a high viscosity index are preferable in applications subject to temperature fluctuations.
Flash Point: The minimum temperature at which the vapors above the oil can catch fire. A higher flash point indicates better safety during handling and use.
Pour Point: The lowest temperature at which the oil will still flow. This is essential for use in low-temperature conditions.
Oxidation Stability: The ability of oil to resist degradation when exposed to oxygen at high temperatures, ensuring longer service life.
Applications
Mix hydrocarbon oilfind applications in a wide variety of industries. Below are several of the typical applications:
Automotive Industry: In the automotive sector, mix hydrocarbon oil is primarily used as engine oils. They provide lubrication to reduce friction between moving parts, dissipate heat, and keep the engine clean. The formulation of these oils is critical, as they must withstand high temperatures and pressures.
Industrial Machinery: Hydraulic oils, gear oils, and compressor oils are also formulated from hydrocarbon oils. Their ability to reduce wear and tear makes them essential in manufacturing and heavy machinery operations.
Marine Applications: In marine settings, hydrocarbon oils are used in both diesel engines and lubricants for equipment operating under harsh conditions. Their stability and resistance to corrosion are particularly important in this sector.
Aviation: Aviation lubricants often utilize hydrocarbon oils due to their excellent high-temperature performance and stability, which are vital for aircraft engines operating at high altitudes and speeds.
Environmental Considerations
As with any petroleum-derived product, mix hydrocarbon oil come with environmental concerns. The extraction, refining, and use of these oils can lead to pollution and other ecological impacts. Here are some key considerations:
Pollution: Oil spills, whether from tankers or drilling operations, can have devastating effects on marine and terrestrial ecosystems. The use of hydrocarbon oils must be managed to minimize the risk of spills and leaks.
Biodegradability: hydrocarbon oils are generally not biodegradable, leading to concerns about their persistence in the environment. The development of biodegradable alternatives is an ongoing area of research, as industries seek more sustainable options.
Regulations: Governments worldwide are imposing stricter regulations on oil usage, focusing on minimizing environmental impact. This includes regulations on emissions from oil combustion and requirements for spill preparedness and response.
Future Trends
The future of mix hydrocarbon oil will likely be influenced by several factors:
Synthetic Oils: The rise of synthetic oils, which can offer superior performance and reduced environmental impact, may change the landscape of lubricant formulations.
Renewable Sources: The shift toward bio-based oils and renewable resources could offer alternatives to traditional hydrocarbon oils, potentially reducing reliance on fossil fuels.
Recycling and Reuse: Advances in recycling technologies may allow for more effective recovery and reuse of oils, minimizing waste and environmental impact.
Conclusion
Mix hydrocarbon oil is indispensable in various sectors due to their unique properties and versatility. Understanding their composition, properties, and applications is vital for anyone working in industries that rely on these oils. As environmental concerns and technological advancements shape the future of lubrication and fuels, ongoing research and innovation will play a crucial role in developing sustainable solutions while meeting the demands of modern industry.
0 notes
Text
Table 4.4 gives data for a 125 cc high performance motorcycle engine operating in three formats – as a standard production engine; with optimization of the carburettor and output gas scavenging conditions; and, finally, with the addition of a catalyst to the exhaust system.

"Environmental Chemistry: A Global Perspective", 4e - Gary W. VanLoon & Stephen J. Duffy
#book quotes#environmental chemistry#nonfiction#textbook#optimization#catalyst#carbon monoxide#nitric oxide#nitrogen dioxide#hydrocarbons#125cc#production engine#optimized engine#engine with catalyst#swiss standards#high performance#carburetor#exhaust system
0 notes
Text
Nevertheless, it should be clear that one feature of the design – namely, the simultaneous introduction of fuel and release of exhaust gases – can lead to problems of loss of unburned hydrocarbons.

"Environmental Chemistry: A Global Perspective", 4e - Gary W. VanLoon & Stephen J. Duffy
0 notes
Text


‘Flying at over Mach 3 is a thermal problem. Everything is too hot, including any air you slow down to interact with the vehicle. You are trying to make the vehicle (and the pilots inside) survive for hours in a pizza oven, while they are getting cozy with two 500 million BTU/hour flamethrowers,’ Iain McClatchie, an aviation and turbine engine expert
Quora.
‘When you look at a graph like this, your first impression might be that the vehicle is this glowing hot thing slicing through the icy -52 C air at 80,000 feet. So naturally, you think of the air as cooling the airplane down.
NO…Not so much. The air has to change to the vehicle’s speed to touch the vehicle, and that requires work. That work heats the air. At Mach 3.2, the stagnation temperature of the air is 740 F, which is hotter than every (labelled) point on the above graph! (The nacelles around the engine afterburners, unlabelled, are in fact hotter than the air around them
Basically, the shocks from the airplane heat the air around it, but the vehicle itself cools the air in contact with it down. Once the airplane passes by, all that disturbed air tumbles to a stop, leaving a path of hot air through the upper atmosphere.
‘So back to life in the pizza oven. The basic solution is (a) leave most of the airframe hot and make it out of stuff like titanium and stainless steel that are strong when hot, and (b) start with a large amount of cold fuel, and then dump heat from critical areas into the fuel before burning it. When decoupling from an aerial tanker, half the SR-71’s weight was fuel.
‘A special type of kerosene fuel, JP-7, was developed for the SR-71 to be good as a heat sink. It boils away at 285 C at 1 atmosphere pressure, which is the upper end of the kerosene range. When the plane tanked up at 30,000 feet, the kerosene might start below 0 C. At speed, it would be used to cool the avionics and cockpit, and by the time it arrived at the engine it would get up to 177 C. It was then used as hydraulic fluid for the various engine actuators, primarily the variable geometry nozzle. By the time it got to the fuel injectors it had gotten up to 316 C (but wasn’t boiling because it was at several atmospheres of pressure). At cruise the burner cans were at 330 kPa (about 3.3x the pressure at sea level), so the fuel still didn’t boil as it left the nozzles but the droplets would have evaporated very quickly.’
McClatchie continues;
‘JP-7 is mostly a mix of hydrocarbons centered around C12H26 (dodecane). The graph above shows the vapor pressure of dodecane as a function of reciprocal absolute temperature. That makes it a bit hard to read. 0.0024, for instance, is 417 Kelvin which is 143 Celsius. Liquids start to boil when their vapor pressure is greater than the ambient pressure. I’ve labelled the boiling point of dodecane at 2900 Pa, which is the absolute pressure at 80,000 feet, and 13000 Pa, which is the minimum absolute pressure in the SR-71 fuel tanks. Note that the dodecane component of JP-7 starts to boil at 162 C at sea level… quite a bit less than the advertised 285 C which is actually when the stuff boils away completely.
‘The flash point of JP-7 is 60 C. The fuel was held in tanks whose walls were formed of the skin of the vehicle. Since fuel vapor against the top skin of the vehicle would be well over 60 C during cruise, if air was allowed in any ignition source in the tank would cause a deflagration and destruction of the vehicle. Instead, nitrogen gas from a 260-liter liquid nitrogen dewar was used to pressurize the tanks. This would have mostly been an issue during descent, when the ambient pressure rose and extra gas was needed to fill the tank ullage space. Click on the full article to read more.
Written by Linda Sheffield
@Habubrats71 via X
#sr 71#sr71#sr 71 blackbird#blackbird#aircraft#usaf#lockheed aviation#skunkworks#aviation#mach3+#habu#reconnaissance#cold war aircraft
93 notes
·
View notes
Text
I feel really sorry for whales on account of the whole "driving them to near extinction" thing but in retrospect it's extremely funny to me that we figured out "create an entire industry out of extremely dangerous hunting of a limited number of giant sea mammals" before mastering the whole "hydrocarbons that shoot right out of the fucking ground in unimaginable quantities" thing. There were entire factories of steam engines running machines powered by coal mined from the ground but they were lubricated entirely with foul smelling cetacean juice instead of like, fossil fuel oil that literally shoots out of the ground.
90 notes
·
View notes
Text
Electrons, not molecules
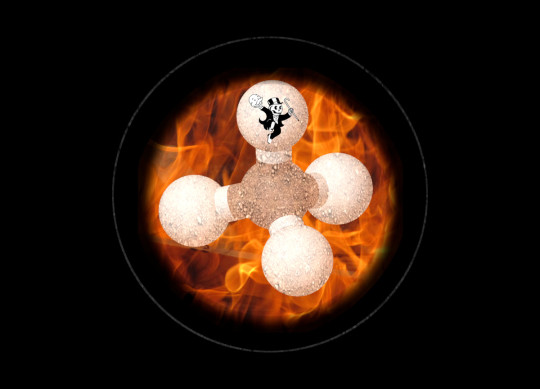
I'm on tour with my new, nationally bestselling novel The Bezzle! Catch me in TUCSON (Mar 9-10), then SAN FRANCISCO (Mar 13), Anaheim, and more!
When hydrocarbon barons do their damndest to torch the Earth with fossil fuels, they call us dreamers. They insist that there's a hard-nosed reality – humanity needs energy – and they're the ones who live in it, while we live in the fairy land where the world can run on sunshine and virtuous thoughts. Without them making the tough decisions, we'd all be starving in the frigid dark.
Here's the thing: they're full of shit.
Mostly.
Humanity does need energy if we're going to avoid starving in the frigid dark, but that energy doesn't have to come from fossil fuels. Indeed, in the long-term, it can't. Even if you're a rootin' tootin, coal-rollin' climate denier, there's a hard-nosed reality you can't deny: if we keep using fossil fuels, they will someday run out. Remember "peak oil" panic? Fossil fuels are finite, and the future of the human race needn't be. We need more.
Thankfully, we have it. Despite what you may have heard, renewables are more than up to the task. Indeed, it's hard to overstate just how much renewable energy is available to us, here at the bottom of our gravity well. I failed to properly appreciate it until I read Deb Chachra's brilliant 2023 book, How Infrastructure Works:
https://pluralistic.net/2023/10/17/care-work/#charismatic-megaprojects
Chachra, an engineering prof and materials scientist, offers a mind-altering reframing of the question of energy: we have a material problem, not an energy problem. If we could capture a mere 0.4% of the sun's rays that strike the Earth, we could give every person on the planet the energy budget of a Canadian (like an American, only colder).
Energy isn't just wildly abundant, though: it's also continuously replenished. For most of human history, we've treated energy as scarce, eking out marginal gains in energy efficiency – even as we treated materials as disposable, using them once and consigning them to a midden or a landfill. That's completely backwards. We get a fresh shipment of energy every time the sun (or the moon) comes up over the horizon. By contrast, new consignments of material are almost unheard of – the few odd ounces of meteoric ore that survive entry through Earth's atmosphere.
A soi-dissant adult concerned with the very serious business of ensuring our species isn't doomed to the freezing, starving darkness of an energy-deprived future would think about nothing save for this fact and its implications. They'd be trying to figure out how to humanely and responsibly gather the materials needed for the harvest, storage and distribution of this nearly limitless and absolutely free energy.
In other words, that Very Serious, Hard-Nosed Grown-Up should be concerned with using as few molecules as possible to harvest as many electrons as possible. They'd be working on things like turning disused coal-mines into giant gravity batteries:
https://www.euronews.com/green/2024/02/06/this-disused-mine-in-finland-is-being-turned-into-a-gravity-battery-to-store-renewable-ene
Not figuring out how to dig or flush more long-dead corpses out of the Earth's mantle to feed them into a furnace. That is a profoundly unserious response to the human need for energy. It's caveman shit: "Ugh, me burn black sticky gunk, make cave warm, cough cough cough."
Enter Exxon CEO Darren Woods, whose interview with Fortune's Michal Lev-Ram and editor Alan Murray contains this telling quote: "we basically focus our technology on transforming molecules and they happen to be hydrogen and carbon molecules":
https://fortune.com/2024/02/28/leadership-next-exxonmobil-ceo-darren-woods/
As Bill McKibben writes, this is a tell. A company that's in the molecule business is not in the electron business. For all that Woods postures about being a clear-eyed realist beating back the fantasies of solarpunk-addled greenies, Woods does not want a future where we have all our energy needs met:
https://billmckibben.substack.com/p/the-most-epic-and-literal-gaslighting
That's because the only way to get that future is to shift from molecules – whose supply can be owned and therefore sold by Exxon – to electrons, which that commie bastard sun just hands out for free to every person on our planet's surface, despite the obvious moral hazard of all those free lunches. As Woods told Fortune, when it comes to renewables, "we don’t see the ability to generate above-average returns for our shareholders."
Woods dresses this up in high-minded seriousness kabuki, saying that Exxon is continuing to invest in burning rotting corpses because our feckless species "waited too long to open the aperture on the solution sets terms of what we need as a society." In other words, it's just too late for solar. Keep shoveling those corpses into the furnace, they're all that stands between you and the freezing, starving dark.
Now, this is self-serving nonsense. The problem of renewables isn't that it's too late – it's that they don't "generate above-average returns for our shareholders" (that part, however, is gospel truth).
But let's stipulate that Woods sincerely believes that it is too late. It's pretty goddamned rich of this genocidal, eminently guillotineable monster to just drop that in the conversation without mentioning the role his company played in getting us to this juncture. After all, #ExxonKnew. 40 years ago, Exxon's internal research predicted climate change, connected climate change to its own profits, and predicted how bad it would be today.
Those predictions were spookily accurate and the company took them to heart, leaping into action. For 40 years, the company has been building its offshore drilling platforms higher and higher in anticipation of rising seas and superstorms – and over that same period, Exxon has spent millions lobbying and sowing disinformation to make sure that the rest of us don't take the emergency as seriously as they are, lest we switch from molecules to electrons.
Exxon knew, and Exxon lied. McKibben quotes Woods' predecessor Lee Raymond, speaking in the runup to the Kyoto Treaty negotiations: "It is highly unlikely that the temperature in the middle of the next century will be significantly affected whether policies are enacted now or 20 years from now."
When Woods says we need to keep shoveling corpses into the furnace because we "waited too long to open the aperture on the solution sets terms of what we need as a society," he means that his company lied to us in order to convince us to wait too long.
When Woods – and his fellow enemies of humanity in the C-suites of Chevron and other corpse-torching giants – was sending the arson billions to his shareholders, he held back a healthy share to fund this deceit. He colluded with the likes of Joe Manchin ("[D-POLLUTION]" -McKibben) to fill the Inflation Reduction Act with gifts for molecules. The point of fantasies like "direct air carbon-capture" is to extend the economic life of molecule businesses, by tricking us into thinking that we can keep sending billions to Exxon without suffocating in its waste-product.
These lies aren't up for debate. Back in 2021, Greenpeace tricked Exxon's top DC lobbyist Keith McCoy into thinking that he was on a Zoom call with a corporate recruiter and asked him about his work for Exxon, and McCoy spilled the beans:
https://pluralistic.net/2021/07/01/basilisk-tamers/#exxonknew
He confessed to everything: funding fake grassroots groups and falsifying the science – he even names the senators who took his bribes. McCoy singled out Manchin for special praise, calling him "a kingmaker" and boasting about the "standing weekly calls" Exxon had with Manchin's office.
Exxon's response to this nine-minute confession was to insist that their most senior American lobbyist "wasn't involved at all in forming policy positions."
McKibben points to the forthcoming book The Price Is Wrong, by Brett Christophers, which explains how the neoclassical economics establishment's beloved "price signals" will continue to lead us into the furnace:
https://www.versobooks.com/products/3069-the-price-is-wrong
The crux of that book is:
We cannot expect markets and the private sector to solve the climate crisis while the profits that are their lifeblood remain unappetizing.
Nearly 100 years ago, Upton Sinclair wrote, "It is difficult to get a man to understand something, when his salary depends on his not understanding it." Today, we can say that it's impossible to get an oil executive to understand that humanity needs electrons, not molecules, because his shareholders' obscene wealth depends on it.

Name your price for 18 of my DRM-free ebooks and support the Electronic Frontier Foundation with the Humble Cory Doctorow Bundle.
#pluralistic#bill mckibben#exxon#exxonknew#solarpunk#climate#climate emergency#climate crisis#gaslighting#guillotine watch#Darren Woods#incentives matter
239 notes
·
View notes
Note
WHY HYDROGEN IS AWESOME
1. No direct CO2 emissions
No carbon dioxide (CO2) is produced when using hydrogen. When it reacts with oxygen, hydrogen generates only electricity, water and heat. And since it does not contain any carbon, no CO2 is created.
This means that by switching from fossil fuels to hydrogen in engines, gas turbines, boilers and fuel cells, the creation of power and heat can be done without direct CO2 emissions. Plant owners can switch to hydrogen technologies like the hydrogen gas turbine from Mitsubishi Power, a power solutions brand of Mitsubishi Heavy Industries (MHI), by converting to hydrogen co-firing, and soon 100% hydrogen firing.
2. High energy density
Hydrogen combusts quickly and at high temperature. When it is combined with oxygen and ignites, it forms water and releases heat.
As our knowledge of how to safely handle the flammability of hydrogen has developed, this has unlocked hydrogen’s potential as a resource for our long-term energy needs, as cleaner fuels can now be made with hydrogen that are highly efficient.
3. Plentiful and versatile
Hydrogen is the most abundant element in the universe and is all around us, mainly in the form of water (H2O) and fossil fuels, otherwise known as hydrocarbons. But it is rare to find pure hydrogen in nature as a gas �� typical levels are less than one part per million by volume. To make pure hydrogen, therefore, it must be produced either from fossil fuels, biomass or water.
Most of the hydrogen in use today is produced using a thermal process. This utilizes high temperatures to produce steam, which is in turn mixed with hydrocarbons to produce hydrogen. But it is increasingly being made using solar-driven, electrolytic or even biological processes.
4. Storage potential
The amount of energy produced from renewable energy sources such as solar and wind power tends to fluctuate because of weather conditions. Combining energy storage solutions with renewables mitigates the intermittent nature of renewable energy production, and hydrogen is a proven provider of effective storage.
Converting renewable energy to hydrogen via electrolysis allows it to be stored and used at a later date, while also stabilizing the energy grid by providing a source of energy “on tap”. Better still, the hydrogen can be stored for long periods without significant losses.
5. An industrial fuel
Hydrogen can also be used as a fuel to power energy-intensive industrial processes, such as metal processing and glass manufacturing. Heavy industry has a challenging decarbonization journey ahead of it, accounting for nearly 40% of the world’s final energy use in 2021.
Hydrogen is likely to be an important tool for replacing fossil fuels in hard-to-abate industry, many processes of which are difficult to be electrified. Initiatives are already underway - steel manufacturer ArcelorMittal is developing industrial-scale production and use of Direct Reduced Iron (DRI) made with 100% hydrogen.
- @hydrogentruck
Hydra, I wouldn't understand this on a normal day. I'm runnin' on two hours of sleep at the minute - I'm convinced this is written in French.
11 notes
·
View notes
Text

The largest tanker on Earth is the Hellespont_Alhambra
It is capable of carrying 441,893 tons of crude oil, which is equivalent to the production of some countries. Its crew consists of only 37 people.....
Deadweight tonnage is 441,893 tons,
Total length is 380 meters,
Width is 68 meters.
Draft is 24.5 meters, and it can carry up to 3.2 million barrels of crude oil.
The ship is powered by an HSD-Sulzer 9RTA84T-D engine, which produces 36,900 kW at 76 rpm, allowing it to achieve a service speed of 16.5 knots when loaded and 17.5 knots in ballast. Classified by both Lloyd’s Register (LR) and the American Bureau of Shipping (ABS), the Hellespont Alhambra features a double hull for enhanced safety. Ballast tanks are emptied to reduce corrosion, and a unique white paint system above the waterline helps reflect sunlight, keeping cargo cooler and reducing hydrocarbon emissions.
17 notes
·
View notes
Note
💌
hey :3 lemme get to the ones I couldn't yesterday!
and you are one more of my extremely cool mutuals!! you've got that perfect combo of really technical interests and wry surreal gay jokes that I can't get enough of :p
but can I also say something about your name!! Anomalocaris canadensis is a really fucking cool animal. we looked at some weird spiral shapes in a bit of rock and applied our imaginations and build our computer simulations and write our comparative morphology papers and in the end we can say that there was very probably an animal that looked something quite like this:
and that 499 million years after the last of these animals died, some weird apes came along and did their best to reconstruct its form. by contrast I feel like the horizon for my last coherent effect on the world might be measured in the hundreds of years at the absolute outside. pretty good going, Anomalocaris!
and, this is a tangent of a tangent, but I love that palaeontology exists. It's one of the most purely 'knowledge for its own sake' sciences, like astronomy. In contrast to physics, biology, etc., which all lead to useful things that let us build bridges or nuclear missiles, cure diseases or engineer fatter chickens or exert other forms of power over the world for good or ill, it's very hard to achieve power from knowing about long dead animals and plants that used to live. Sure, fossil fuels - but you don't need to know much about Carboniferous rainforests to make use of the hydrocarbon sludge they turned into.
But palaeontologists are purely in it for the creatures (and plants and bacteria and all sorts of other beings), and the stories we can tell using what we know about those creatures about how we got here. In that sense, it's kind of an art form - but in contrast to fantasy artists making up creatures from culturally received images, this tradition involves a feedback loop between our imagination and the evidence we can gather from fossils, living animals, etc. etc. This model of Anomalocaris canadensis is pretty, but it's considered wrong, because we have been able to infer a bunch of extra stuff about the real animal, which Wikipedia editors helpfully summarise in this block of jargon:
Lacking head sclerites (evident in A. canadensis); Lacking neck flaps (3 pairs in Anomalocaris); stout frontal appendages with endites lacking auxiliary spines (frontal appendages laterally flatten with endites of intermediate podomeres possess auxiliary spines in A. canadensis).
And despite its lack of direct application to any form of engineering, humans have put the resources into palaeontology to get a really quite intricate knowledge of some fraction of the life that has existed on this planet. Look at this radial cladogram:

might as well be a solomonic pentagram for all the practical good it does, but it's beautiful. the cheeky little 'you are here'!
so from one really dorky academia URL to another, shoutout from canonical momentum to Anomalocaris canadensis :p
anyway returning to the 'saying nice things about mutuals' game, you ask lots of really interesting questions that I have a lot of fun engaging with! I had a great time with that chat about energy we had a little while back. so high fives across the wired ^_^
7 notes
·
View notes
Text

In a step toward solar fuels, durable artificial photosynthesis setup chains two carbons together
A key step toward reusing CO2 to make sustainable fuels is chaining carbon atoms together, and an artificial photosynthesis system developed at the University of Michigan can bind two of them into hydrocarbons with field-leading performance. The system produces ethylene with efficiency, yield and longevity well above other artificial photosynthesis systems. Ethylene is a hydrocarbon typically used in plastics, so one direct application of the system would be to harvest carbon dioxide that would otherwise be vented into the atmosphere for making plastics. "The performance, or the activity and stability, is about five to six times better than what is typically reported for solar energy or light-driven carbon dioxide reduction to ethylene," said Zetian Mi, professor of electrical and computer engineering at the University of Michigan and corresponding author of the study in Nature Synthesis.
Read more.
#Materials Science#Science#Solar power#Artificial photosynthesis#Carbon dioxide#Materials processing#University of Michigan
11 notes
·
View notes
Text
Carrying out this kind of test using 7.3 kW engines burning gasoline, the exhaust was found to have the composition shown in Table 4.3.

"Environmental Chemistry: A Global Perspective", 4e - Gary W. VanLoon & Stephen J. Duffy
#book quotes#environmental chemistry#nonfiction#textbook#experiment#test#engine#gasoline#exhaust#two stroke engine#four stroke engine#carbon monoxide#nitric oxide#nitrogen dioxide#hydrocarbons#greenhouse gas emissions
0 notes
Text
Some Spider Silk Facts
The strongest spider silk is produced by Darwin’s Bark Spider, which is twice as strong as any gossamer recorded before. It has a tensile strength of up to 520 megajoules per cubic metre.
Gossamer is stronger than steel and kevlar, and it has been suggested that a single pencil-width strand of the stuff could stop a Boeing 747 in its tracks.
The reason we can break such a strong material is because it is 20 times thinner than a human hair, usually measuring just 0.003mm across.
There are seven types of silk produced by a spider of Araneus Diadematus: dragline/major ampullate silk (which forms the basic structure of a web and also the web the spider itself dangles from), minor ampullate silk (which forms the auxiliary spiral in the centre of a web), flagelliform silk (which forms the core fibres of the ‘capture spiral’) , aggregate silk (forming the aqueous coating on a web), cylindriform silk (tough outer silk of an egg sac), aciniform silk (soft inner silk of an egg sac also used for swathing prey) and pyriform silk (which is used as a sort of cement for joining and attaching different parts of the web).
These little architects have seven different silk glands, as a result, all of which are employed by the spinnerets at the spider’s rear end.
Gossamer is made up of a blend of different proteins linked together in a chain: it consists of proteins rich in nonpolar (example; fats, oils, gasoline and petrol) and hydrophobic (example; oils, waxes and steroids) amino acids like glycine (C₂H₅NO₂ - white solid) and alanine (C3H7NO2 - white powder) but no (or very little) tryptophan (C11H12N2O2).
Glycine is a compound our bodies use to make protein. It is an antioxidant, anti-inflammatory, cryoprotective and immunomodulatory in peripheral and nervous tissues.
Alanine is an alpha amino acid also used to make proteins. It is a hydrocarbon. Hydrocarbons are divided into two classes in biochemistry: aromatic compounds and aliphatic compounds (from the Greek word ‘aleiphar’ - fat/oil). Alanine falls into the latter category. Another example of an aliphatic compound is squalene, which is found in shark livers and the stomach oil of birds.
So spidersilk seems to be mainly made up of carbon, hydrogen, nitrogen and oxygen, with more hydrogen and carbon than any other element, making it an aliphatic hydrocarbon based substance. (I think. I’m not a scientist, I’m just making an educated guess.)
So why have we not spun our own clothes / harvested spidersilk? Multiple reasons.
The main reason being that spiders can’t be farmed like silkworms due to the fact that they will cannibalise each other in close proximity. The silk is so fine that it would take harvesting from 400+ spiders to make a single yard of silk. And the silk also hardens when exposed to air which makes it difficult to work with.
This silk hardens as it passes through the spider’s spinnerets. Also, the problem with trying to genetically engineer spidersilk ourselves is that we can only partially replicate its chemical makeup.

Also here’s the heckin chungus of a spider in question, with the world’s strongest web:

He’s buff and he knows it. Proud chonky fella. He’s cute. 😭🥺
15 notes
·
View notes
Text
Transforming Wood Waste for Sustainable Manufacturing - Technology Org
New Post has been published on https://thedigitalinsider.com/transforming-wood-waste-for-sustainable-manufacturing-technology-org/
Transforming Wood Waste for Sustainable Manufacturing - Technology Org
Lignin, a complex organic polymer, is one of the main components of wood, providing structural support and rigidity to make trees strong enough to withstand the elements. When transforming wood into paper, lignin is a key ingredient that must be removed and often becomes waste.
Marcus Foston (left) and collaborators are exploring how to use lignin, a common waste product of paper pulping, as a source of renewable alteratives to petroleum-derived chemicals. Image credit: Jerry Naunheim Jr./Washington University
Marcus Foston, an associate professor of energy, environmental and chemical engineering in the McKelvey School of Engineering at Washington University in St. Louis, is exploring how to add value to lignin by breaking it down into small molecules that are structurally similar to oxygenated hydrocarbons. These renewable chemicals are key components in many industrial processes and products, but they are traditionally sourced from nonrenewable petroleum.
Foston’s study of lignin disassembly, done in collaboration with Sai Venkatesh Pingali, a neutron scattering scientist at Oak Ridge National Laboratory (ONRL), was published Jan. 17 in the journal Sustainable Chemistry & Engineering.
“Lignin’s structure actually looks a lot like what we get from petroleum,” said Foston, who is also director of WashU’s Synthetic Biology Manufacturing of Advanced Materials Research Center (SMARC). “In current manufacturing processes, we spend time making petroleum look like the elements of lignin. Instead, I’m using a catalyst to break lignin down more easily and in such a way that it produces specific chemicals. Once we can produce chemical from lignin in a form we want, then we can make more efficient use of lignin, which is an abundant byproduct of pulping wood into paper.”
With collaborators at ORNL, Foston used neutron scattering to study how lignin interacts with solvents and catalysts during its disassembly under reaction conditions, including high temperature and pressure. ORNL’s advanced facilities allowed researchers to observe the reaction process in real time to improve their catalyst and further streamline reaction systems for lignin depolymerization. This direct, molecular-level view is critical, Foston said, to figure out how the catalyst and lignin behave in solution and to ensure the lignin doesn’t recondense into a polymer with bonds scientists can’t easily break.
“In this study, we’re specifically thinking about how we can take the large amount of lignin that gets produced during biofuel or paper production and use it to make renewable chemicals that replace some of the chemicals we currently get from petroleum,” Foston said. “More broadly, the same depolymerization principles we’re exploring with lignin could be used in other applications. For example, the same lessons from this study apply to plastic waste scenarios, where one approach is to deconstruct plastic waste into small molecules that could be used to make plastic or other useful products.”
“Ultimately, we want to take a bunch of chemicals that are coming from petroleum and figure out how we can make those renewably,” Foston added. “Everything we’re learning about lignin will apply to other spaces as well.”
Source: Washington University in St. Louis
You can offer your link to a page which is relevant to the topic of this post.
#advanced materials#amp#applications#approach#Biology#catalyst#catalysts#chemical#Chemical engineering#chemicals#chemistry#Collaboration#energy#engineering#Environmental#Facilities#form#Fundamental physics news#high temperature#how#how to#hydrocarbons#it#learning#Link#Manufacturing#materials#molecules#neutron#Oak Ridge National Laboratory
1 note
·
View note
Text
Oh yeah, about that persistent anemia that the new endo pretty much flipped over...
Yeah, I developed some suspicions of my own there, and it took maybe 2 seconds of searching to turn up plenty of info about PPIs directly causing iron absorption issues too. 🙄 Besides the other minerals I already knew about. They're pretty well known for that, leading to bone density problems for some people. Most likely also the reason my sodium and potassium levels have kept coming back just under the acceptable range too. You fuck around with the balance of digestive secretions and pH, it may have some unintended effects!
I've been stuck on high-dose PPIs ever since that big DKA crash in 2020 damaged my digestive system. They've actually kept me on the maximum dosages approved for adult humans, but I've ended up cutting it back some myself to the point that it's just higher than usual. (Partly to hopefully also cut back somewhat on potential side effects like this, but also thanks to repeated pharmacy supply issues with formats I can reasonably swallow with my now fucked-up esophagus!)
Never had any notable reflux issues before, but with that damage there's nothing really keeping the stomach acid down where it belongs. With evidence of that from some truly unpleasant testing where they dangle sensors down your throat. And of course they're trying to keep more acid from doing more damage. Some of that effect if I don't have the meds is probably from rebound acid overproduction, but if I don't take them for whatever reason now it gets ugly within a few days!
So yeah, I do seem to be stuck on the PPIs for the forseeable future now, whether I like it or not. It's just good to know that I do need to make sure to take in extra of certain minerals on a regular basis to try and compensate for that.
Am I surprised that two endos now, and the GP I got referred to for more tests over it have just completely overlooked well-known effects of meds they knew I was taking regularly, and which are readily found immediately by plugging "PPIs iron" into your search engine of choice? Not remotely.
Nope, they'd prefer to mutter direly about rare liver diseases and all kinds of less likely possibilities--and order a zillion blood tests. At least they DO seem to like to order various testing here when something looks off instead of just ignoring it, I will give them that. Guessing they are not under the same kind of pennypenching pressure that kinda got me needing the freaking PPIs at all.
PPIs are more within primary care's wheelhouse than endocrinology's. But. the gastroenterology guy who has been prescribing the stuff for a good while now ALSO did not put together that the borderline low electrolytes he was concerned about in the context of anesthesia might have some connection there before I brought it up as a possibility. To his credit, he also did not act like I must be on crack mentioning it, or seem to take it as some weird challenge to His Authority. But, the guy--who does come across as smart and competent enough--had obviously never considered this as a possibility.
This shit may be anything but unexpected by now. But, it really does sort of bring home just how bad at least 95% of clinicians seem to be at diagnostic pattern matching, in general.
(I mean, working with patterns like this is just one of the things my own brain has Always Just Done. Getting it to fucking shut up sometimes when it's not being useful, and channeling it into actually productive avenues are the bigger challenge. Seems to be another on the less shitty side of those neuroweird superpowers. But, some of these otherwise intelligent people are just so damn terrible at it by just about any standards. At the risk of sounding like some kind of condescending prick.)
11 notes
·
View notes
Text
1981 Pontiac Trans Am Turbo V-8

1st generation of Firebirds ran from 1967 to 1969, very short lived but it made a generation impact setting up many more gutsy Firebirds combined with rugged good looks that lasted for four generations, with models in production from 1967 through 2002. These 1st Pontiac Firebirds would evolve into the most iconic muscle cars to ever hit the market during that time period.
These first Firebirds came with a 3.8 L6 engine that offered 220 horsepower. It hit a top speed of 114 mph and go 0-60 in just over 10 seconds. Optional was an upgraded 6.6-liter V8 engine that packed 340 horsepower and torque of 240 lb.ft. These Firebirds got off to a slow start which prompted Pontiac to release in 1969 a Trans Am Performance and Appearance Package and cost $725. Its name was inspired by the Name Trans American racing series nationwide fitted with a 60-inch airfoil across the rear deck.
Along with the polar white background were blue racing stripes The base engine that came with the Trans Am was a 400-cid V-8 engine that had a brake horsepower of 335. Other performance advantages Trans Ams gave buyers included a one-inch front stabilizer bar, a heavy-duty suspension system, variable-ratio power steering and seven-inch-wide rims featuring Polyglas F70xl4s.
1970 models saw four different engines and three transmissions but only tepid power. Pontiac quickly put out a stronger 6.6-liter Ram Air IV motor. In 1971, buyers had access to a 7.5-liter engine. Though it looked like the sky was the limit for the Firebird’s.
By 1974 regulations relating to limiting carbon monoxide and hydrocarbon also made it so ignition timing took longer, and cams weren’t as aggressive. Choked so bad, the optional V8 engine would put out 200 hp. It started with a 6.6L V8 engine and progressed to a 7.5 L V8 engine, but by the end of their run were down to their top engine being a 4.9 L Pontiac turbo V8.
The third generation of Firebirds from 1982 to 1992 would be a strong one with movie roles.
3 notes
·
View notes
Text

Air Travel – A Faster Car?
Like the automobile, air travel appears to offer humans benefits anarchists cherish: the freedom to travel widely, to experience new cultures and relate to more people, to develop culturally and spiritually, to walk a mile in other people’s shoes, to find a place for ourselves in a world going mad. But modern air travel, because of its huge volume and vast infrastructural needs, is one of the most uniquely polluting activities humans have ever invented. Acres of concrete. Vast areas taken up by terminals, facilities, access roads, warehouses, hotels and shopping malls. The noise of take-off and landing and the misery caused by the increasing number of night-time flights. The destruction of habitats as runways are extended and new terminals built. The unremitting damage to the atmosphere, especially the troposphere (where weather systems form), which is vital for human life on Earth. Greenhouse gas emissions from the world’s aircraft fleet currently account for 4% of all global warming from human activity. By 2050, emissions from air travel may be contributing 15% of predicted climate change if left unchecked.
The convenience of air travel and the rise of the no-frills carrier obscures the extent of the problem. Passenger-kilometres flown from the UK increased from 125 billion in 1990 to 260 billion in 2000. Government forecasts predict that, without controls, British airports will be serving over 1bn passengers a year by 2050. Air traffic worldwide is predicted to increase six times by 2050 and we will be burning at least three times as much fuel as at present. The problem is bad and is going to get a lot worse over the next 30–40 years.
During flight, aircraft engines emit carbon dioxide, oxides of nitrogen and sulphur, water vapour, hydrocarbons, sulphur particles and soot. Your share of a return flight to Florida pumps more carbon dioxide into the atmosphere than a whole year’s driving. These emissions alter the chemical composition of the atmosphere in a variety of ways: they have a massive and widely-acknowledged impact on climate change. Air travel causes large-scale reduction in ozone levels in the stratosphere and increased UV radiation at ground level, i.e. increased risks of skin cancer. Activity at airports cause changes to the troposphere for hundreds of kilometres downwind and greatly reduces local air quality. When all planes in the USA were grounded after 9/11, the nights got warmer and the days colder, evidence that air travel causes global warming right now.
Air travel is big business, and getting bigger: airports expanding, regional hubs, new terminals, the associated sprawl of commercial development, government subsidies for each new generation of planes, the bidding wars for landing rights. Air travel is subsidised by £9bn a year in Britain alone, because there is no tax on kerosene or VAT charged on fares. In 1944, the great powers decided that to rebuild a shattered world and reward the aircraft companies building military aircraft to win the war, air travel would not be taxed. But who knew then what the environmental effects would be or just how big air travel would get? Sixty years have gone by and we are staring over the abyss.
The working class bears the brunt of the destructive effects of air travel and airport development, yet the poorest 10% of us never fly. The rise of charter flights to Ibiza and no-frills flights to Prague – which carry a surprising number of the comfortable middle class (75% or travellers are from the ABC social classes) cannot alter the basic facts. Our health, sanity, quality of life and future are all at risk. Governments say air travel is good and cannot be restricted or curbed. Business says demand for air travel – a demand created and fuelled by big business – means that airports must expand and the volume of flights must increase. We must build bigger planes that are more fuel efficient. Why? We shouldn’t forget either the point where big business and the state truly meet: in the 1990s, military aircraft consumed one third of the fuel used by all planes and produce proportionately more emissions of climate-changing pollution. Who’s going to regulate them?
There is no latent demand for more air travel and bigger airports, it is being stoked by hidden subsidies and government collusion with big business to do nothing to halt runaway growth. Government studies pointing to a ‘need’ for new airports and runways are always based on taxation regimes and subsidies remaining the same: one study found that if fuel were taxed at 46p a litre and VAT phased in, passenger numbers would rise so slowly that existing runways could cope till 2030 and beyond. At the same time, if we invested in high-speed rail links huge amounts of air travel across Europe could be avoided.
People often say that technological improvements will reduce environmental impacts. But air travel is increasing so much that no amount of technological advance will reduce damage, merely slow the rate of environmental destruction. By 2010, increased CO2 emissions by the aviation industry will totally negate all other climate-change protection policies and regimes: we are being asked to change our ways and tighten our belts just so the rich can go on flying. There is no technological fix, only a massive reduction in the amount or air travel will do. That will only come about by us choosing not to travel, by a massive change in culture and social relations. This is what an anarchist society of the future, a sustainable society for all to share and enjoy, is all about. Our society is on a truly human scale, where we live, travel and interact in ways that meet our human needs without damaging others and destroying the environment.
There is beauty all around us if we choose to see it. There is something in every human face. There are places of interest and leisure within hundreds of miles, why travel thousands? If communities can be re-engineered to be self-sustaining – and they can be – will we need to travel thousands of miles to do business, to negotiate deals, exchange ideas, meet people? If we need to work only a few hours a day and tasks get done when they can, not when someone says they must, will we need to travel at hundreds of miles an hour to do them? Did people invent the internet just so we could buy on-line? The massive amount of air travel today and in the future isn’t good for people or the planet and isn’t necessary for society to function or people to live rich lives of experience and pleasure. It is necessary for the airline industries and those who are running things: the politicians, bureaucrats, generals and businessmen who enjoy looking down on us.
#anarcho-communism#anarcho-primitivism#anti-capitalism#capitalism#class#class struggle#climate crisis#colonialism#deep ecology#ecology#global warming#green#Green anarchism#imperialism#industrialization#industrial revolution#industrial society#industry#mutual aid#overpopulation#poverty#social ecology#anarchism#anarchy#anarchist society#practical anarchy#practical anarchism#resistance#autonomy#revolution
2 notes
·
View notes