#Tissue engineering scaffolds
Explore tagged Tumblr posts
Text

Biomaterials are at the forefront of regenerative medicine and implant technology! 🌱🔬 From creating sustainable solutions to advancing healthcare, these innovations are shaping the future of medical science. Discover how science and nature unite for better healing! 🌟
#Biomaterials in regenerative medicine#Innovative medical implants#Natural and synthetic biomaterials#Tissue engineering scaffolds#Advanced drug delivery systems#Biocompatibility challenges#3D printing in biomaterials
0 notes
Text
Advanced Biomanufacturing Solutions: How Biofabrication is Meeting the Need for Complex Biologics in the Biotechnology Industry
Biofabrication Market: A Growing Industry with Promising Future
Biofabrication is an emerging technological field that focuses on manufacturing structures for tissue engineering and regenerative medicine. This field has gained significant attention in recent years due to its potential to revolutionize the healthcare industry. In this article, we will explore the biofabrication market, its size, growth projections, and the key factors driving its growth.
Market Size and Growth Projections
The biofabrication market is expected to grow significantly in the coming years. According to a report by Intent Market Research, the global biofabrication market size is anticipated to reach USD 80 Billion by the end of 2036, growing at a CAGR of 10% during the forecast period, i.e., 2024-2036. Another report estimates the market size to be USD 22.76 Billion in 2023 and predicts it to reach USD 48.27 Billion by 2031, with a CAGR of 10.2% from 2024 to 2031.
Key Factors Driving Growth
Several factors are driving the growth of the biofabrication market. One of the primary factors is the increasing demand for biologics and biosimilars. The development of biologics accounts for around 40% of all pharmaceutical R&D spending, making it essential for advancing healthcare. Additionally, biomanufacturing accelerates scientific research, boosts economic growth, and provides jobs, making it a vital industry for the future.
Another key factor driving growth is the advancements in biofabrication techniques. Recent advancements in volumetric bioprinting, scaffold-free bioassembly, and hybrid biofabrication strategies have improved the scale, rate, and intricacy at which tissues can be fabricated. These advancements have the potential to recapitulate the structure and complexity of native tissues, making them suitable for various applications such as biomimetically engineered models for drug discovery, cosmetics testing, tissue regeneration, and medical devices.
Regional Analysis
The biofabrication market is expected to grow significantly in various regions. North America is expected to hold the largest share of 36% during the forecast period due to the robust presence of large biopharmaceutical businesses and the improved infrastructure in biological research. The Asia-Pacific region is expected to witness the fastest growth due to the rapid adoption of advanced technologies and the high prevalence of chronic diseases.
Competitive Landscape
The biofabrication market is highly competitive, with several key players operating in the market. Some of the major players include Applikon Biotechnology BV, bbi-biotech GmbH, Danaher Corporation, Eppendorf AG, Esco Group of Companies, GEA Group Aktiengesellschaft, Meissner Filtration Products, Inc., Merck KGaA, PBS Biotech, Inc., Pierre Guérin, Sartorius AG, Shanghai Bailun Biotechnology Co. Ltd., Solaris Biotechnology Srl., Thermo Fisher Scientific Inc., and ZETA GmbH.
Conclusion
In conclusion, the biofabrication market is expected to grow significantly in the coming years due to the increasing demand for biologics and biosimilars, advancements in biofabrication techniques, and the growing demand for advanced biomanufacturing solutions. The market is expected to be driven by key players operating in the market, and regional growth is expected to vary. As the industry continues to evolve, it is essential to stay updated on the latest advancements and trends to capitalize on the growth opportunities in the biofabrication market.
#Biofabrication#biomanufacturing#biologics#biosimilars#regenerative medicine#tissue engineering#biomimetic engineering#biotechnology#biopharmaceutical industry#advanced biomanufacturing solutions#volumetric bioprinting#scaffold-free bioassembly#hybrid biofabrication strategies#biomanufacturing techniques#biomanufacturing market
0 notes
Text

Scientists create method to bond hydrogels and other polymeric materials using chitosan
Hydrogels are versatile biomaterials conquering an increasing number of biomedical areas. Consisting of water-swollen molecular networks that can be tailored to mimic the mechanical and chemical features of various organs and tissues, they can interface within the body and on its outer surfaces without causing any damage to even the most delicate parts of the human anatomy. Hydrogels are already used in clinical practice for the therapeutic delivery of drugs to fight pathogens; as intraocular and contact lenses, and corneal prostheses in ophthalmology; bone cement, wound dressings, blood-coagulating bandages, and 3D scaffolds in tissue engineering and regeneration. However, attaching hydrogel polymers quickly and strongly to one another has remained an unmet need as traditional methods often result in weaker adhesion after longer-than-desired adhesion times, and rely on complex procedures.
Read more.
20 notes
·
View notes
Text

Helping Heal Bone
A 3D-printed scaffold with cryogel fibres incorporating vessel growth- and bone formation-enhancing peptides for promoting bone regeneration at the site of skull damage or defect
Read the published research article here
Image from work by Yuxuan Wei and Hao Pan, and colleagues
Zhejiang Engineering Research Center for Tissue Repair Materials, Wenzhou Institute, University of Chinese Academy of Sciences, Wenzhou, Zhejiang, China
Image originally published with a Creative Commons Attribution 4.0 International (CC BY-NC 4.0)
Published in Science Advances, February 2024
You can also follow BPoD on Instagram, Twitter and Facebook
17 notes
·
View notes
Text
Human Tissues: An Anatomy and Physiology Exploration
In the captivating realm of human anatomy and physiology, tissues are the cornerstone, weaving the intricate tapestry of our bodily functions. Let's embark on a journey to uncover the different types of tissues, delving into their unique characteristics and significance.
1. Epithelial Tissue: The Body's Protective Shield
Epithelial tissue lines surfaces, cavities, and glands, acting as our first line of defense. Stratified, simple, squamous, cuboidal, and columnar epithelia all serve distinct functions in various bodily structures.
2. Connective Tissue: The Structural Framework
Connective tissues serve as the architectural scaffolding, binding and supporting other tissues. Dense, loose, cartilage, bone, and blood, each with its specialized properties, contribute to the body's structural integrity.
3. Muscle Tissue: The Engine of Movement
Muscle tissues are the driving force behind movement and force generation. Skeletal, smooth, and cardiac muscles exhibit unique characteristics and play crucial roles in our mobility and vital functions.
4. Nervous Tissue: The Information Superhighway
Nervous tissue conducts electrical impulses, facilitating rapid communication within the body. Neurons and neuroglia work in harmony to transmit and process vital information.
Diverse and specialized, these tissue types ensure the harmonious functioning of our body systems. Join us in this captivating journey through the pages of OpenStax Anatomy and Physiology to explore the intricacies of human biology. 📚🧬
Prepare to be captivated by the precise design and functionality of human tissues as we navigate the intricate world of anatomy and physiology! 📖🔬
References
OpenStax Anatomy and Physiology, Section 4.1
OpenStax Anatomy and Physiology, Section 4.2
OpenStax Anatomy and Physiology, Section 4.3
OpenStax Anatomy and Physiology, Section 4.4
OpenStax Anatomy and Physiology, available at https://openstax.org/details/books/anatomy-and-physiology.

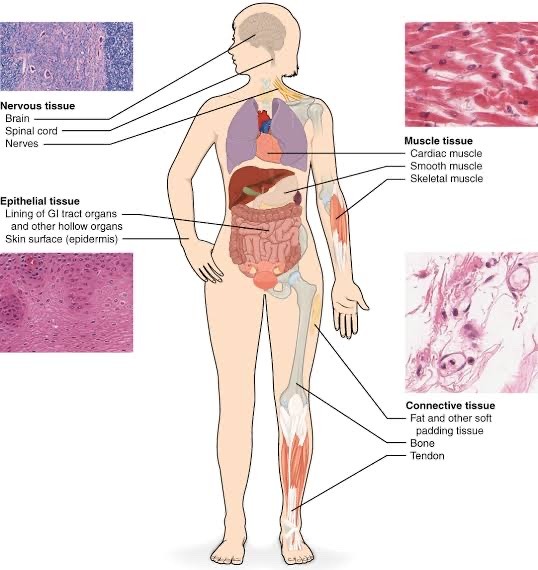
#science#biology#college#education#school#student#medicine#doctors#healthcare#health#human physiology#humanphysiologynotes#human anatomy#anatomy#nursing#medical stuff
17 notes
·
View notes
Text
The Art of Building Meat: Bioprinting Techniques in Cultured Meat Market Production

Introduction:
The future of food is taking shape in bioreactors and 3D printers. Cultured Meat Market, also known as clean meat or lab-grown meat, emerges as a revolutionary solution to the environmental and ethical concerns surrounding traditional meat production. This article delves into the fascinating world of bioprinting techniques, a key component in creating complex, delicious cultured meat products.
Download FREE Sample: https://www.nextmsc.com/cultured-meat-market/request-sample
Cultured Meat: A Sustainable Disruption
Traditional meat production carries a significant environmental burden, contributing to deforestation, greenhouse gas emissions, and water pollution. Cultured meat offers a promising alternative, growing meat from animal cells in a controlled environment.
Here's a simplified breakdown of the process:
Cell Collection: A small sample of muscle stem cells is obtained from an animal through a minimally invasive procedure.
Cell Culture: These cells are multiplied in a nutrient-rich medium within bioreactors.
Differentiation and Maturation: Controlled growth factors in the medium stimulate the cells to differentiate and mature into muscle tissue.
Bioprinting: This innovative step allows for precise arrangement of the cells to create the desired structure and texture of meat.
Maturation and Processing: The bioprinted meat undergoes further maturation and processing to achieve the final product.
Inquire before buying: https://www.nextmsc.com/cultured-meat-market/inquire-before-buying
Bioprinting: Engineering the Future of Meat
Bioprinting takes cultured meat production to a whole new level. Here's how it works:
Digital Design: A computer-aided design (CAD) model is created to define the desired structure of the meat product, such as a steak or a burger patty.
Bioink Preparation: A biocompatible material, often containing a mixture of cells, nutrients, and scaffolding materials, is prepared for printing.
3D Printing Process: The bioink is loaded into a bioprinter, which uses computer-controlled mechanisms to deposit the bioink layer-by-layer, replicating the designed structure.
Cell Culture and Maturation: Once printed, the bioprinted cells are allowed to mature and grow within a bioreactor, mimicking the natural development of muscle tissue.
Benefits of Bioprinting for Cultured Meat
Bioprinting offers several advantages in the production of cultured meat:
Complex Structures: Bioprinting allows for the creation of complex, multi-cellular structures that mimic the texture and marbling of traditional meat cuts. This is difficult to achieve with traditional culturing techniques.
Fat Distribution: Bioprinting enables precise control over fat distribution within the meat, allowing for the creation of leaner or marbled cuts as desired.
Customization: Bioprinting paves the way for personalized meat products tailored to specific dietary needs or preferences.
Vascularization: Bioprinting can potentially be used to create vascular networks within the cultured meat, promoting cell growth and mimicking the natural delivery of nutrients and oxygen.
Types of Bioprinting Techniques for Cultured Meat
Several bioprinting techniques are being explored for cultured meat production, each with its own advantages and limitations:
Extrusion-based Bioprinting: This common technique uses a pressurized system to deposit bioink through a nozzle, creating a filamentous structure. It's suitable for high-viscosity bioinks but may have limitations in resolution.
Inkjet Bioprinting: Similar to an inkjet printer, this technique uses a jet of bioink droplets to create a patterned structure. It offers high resolution but may be limited in the types of bioinks it can handle.
Stereolithography (SLA): This light-based technique uses a laser to solidify layers of bioink resin, building the desired structure layer-by-layer. It offers high accuracy but may require specialized biomaterials.
Challenges and Opportunities in Bioprinting for Cultured Meat
While bioprinting holds immense promise, some challenges need to be addressed:
Cost Reduction: Bioprinting equipment and bioink development are currently expensive, requiring cost optimization for large-scale production.
Bioink Development: Creating bioinks that are biocompatible, support cell growth, and allow for precise printing remains an ongoing area of research.
Scalability: Scaling up bioprinting processes to meet commercial production demands requires further advancements in technology and infrastructure.
However, these challenges offer exciting opportunities for innovation:
Advancements in Biomaterial Science: Development of affordable and efficient bioinks specifically tailored for cultured meat is crucial for large-scale adoption.
Bioprinter Design and Optimization: Improvements in bioprinter design can increase printing speed, resolution, and efficiency for cost-effective production.
Collaboration Between Researchers and Industry: Collaboration can accelerate research and development efforts to overcome technical hurdles and improve bioprinting techniques.
Conclusion: A Bite of the Future - Bioprinted Cultured Meat
Bioprinting represents a transformative leap in the world of cultured meat. This technology holds the potential to create delicious, sustainable, and ethical meat alternatives, replicating the textures and flavors we crave.
While challenges remain in terms of cost reduction, bioink development, and scalability, ongoing research and collaboration are paving the way for advancements. Bioprinting paves the way for a future where:
Cultured meat becomes readily available and affordable for consumers.
Bioprinting techniques can create a wider variety of meat products, from steaks to sausages.
Consumers can enjoy the taste and texture of meat while making a positive environmental impact.
2 notes
·
View notes
Text
A More Realistic Artificial Skin May Lead to Medical Advances - Technology Org
New Post has been published on https://thedigitalinsider.com/a-more-realistic-artificial-skin-may-lead-to-medical-advances-technology-org/
A More Realistic Artificial Skin May Lead to Medical Advances - Technology Org
A new bioengineered skin model could improve testing of skincare products and lead to better ways to heal damaged skin.
Paul Dalton and Ievgenii Liashenko in the lab. Image credit: University of Oregon
University of Oregon researchers have teamed up with scientists with the French personal care company L’Oréal to develop a multilayered artificial skin that more accurately mimics real human skin, and can be grown in just 18 days. The advance relies on a novel 3D printing technique invented by Paul Dalton, an associate professor in the Phil and Penny Knight Campus for Accelerating Scientific Impact at the UO.
The research was published in the journal Advanced Functional Materials.
“This is the first known case of replicating quality skin tissue at full thickness, using different kinds of cells separated by a membrane,” said Ievgenii Liashenko, a research engineer in Dalton’s lab.
Creating an artificial skin isn’t as simple as growing cells in a petri dish. Real skin has multiple layers, with different kinds of cells that perform distinct functions. And in the body, cells are supported by an external network of proteins and other molecules. Called the extracellular matrix, this system helps cells stay in position and communicate with their neighbors, which is key to keeping all systems working properly.
To replicate this complex environment, the researchers designed a two-layered artificial skin, with the layers separated by a membrane.
A 3D printer making the extremely fine strands used to create a new generation of artificial skin. Image credit: University of Oregon
Researchers from Dalton’s lab and L’Oréal co-developed plastic scaffolds that mimic the extracellular matrix via a network of finely structured 3D printed threads. Then, L’Oréal researchers grew cultured cells in those scaffolds to create the artificial skin, with different cell types growing in each layer. The membrane prevents the cells in the different layers from mixing as they develop.
“Other attempts don’t have the same layering—it actually looks like real skin,” said Dalton, who is the Bradshaw and Holzapfel Research Professor in Transformational Science and Mathematics.
The underlying scaffolds resemble a mesh material made of many spaghetti-like threads, each much thinner than a human hair. To make the porous scaffold, members of Dalton’s team used a 3D printing technique they’ve developed called melt electrowriting. In that technique, an electric field pulls the molten printing plastic from a nozzle into a thin thread, enabling very precise control over the printing.
Some 3D printing techniques can create very fine details, but only small objects, Dalton said.
Other techniques allow easy fabrication of larger pieces, but at the expense of resolution. Melt electrowriting bridges that gap, allowing engineers to create relatively large objects with fine details.
The new skin model can be grown in just 18 days, the researchers found, rather than the 21 to 35 days it took to create previous scaffold-based artificial skin models. That makes it more viable to use in commercial lab testing.
The final product. Image credit: University of Oregon
L’Oréal is currently using the artificial skin to test cosmetics and skin care products. Going forward, both Dalton’s team and L’Oréal researchers plan to explore the many other potential uses for the underlying scaffolding in skin tissue engineering.
Other potential skin-related applications include healing diabetic foot ulcers and creating skin grafts for burn patients. Beyond skin, the scaffolds developed by Dalton’s team could support myriad biomedical applications, such as artificial blood vessels and structures to help regrow damaged nerves.
“While we’ve made this big advance with the skin, the design of the scaffold is crucial and could be applied more broadly,” Dalton said. “There are so many diseases and injuries in the world that aren’t being solved, so having an extra tool to try to tackle these is really valuable.”
The materials used in the scaffold are already FDA– approved for use inside the human body, making the path to real-world application smoother.
The fabrication facilities at the Knight Campus make it possible for Dalton’s team to scale up production of the materials, Dalton said. “This is the part of the Knight Campus projecting its expertise beyond UO to influence state-of-the-art research fields globally.”
Source: University of Oregon
You can offer your link to a page which is relevant to the topic of this post.
#3d#3D printing#applications#Art#artificial#artificial skin#blood#blood vessels#cell#cell types#Cells#Chemistry & materials science news#Design#details#Diseases#easy#electric field#Engineer#engineering#engineers#Environment#Fabrication#Facilities#FDA#Featured technology news#Full#gap#healing#human#Ideas
3 notes
·
View notes
Text
Round 1B
Erykah Badu’s Baduizm: On and on and on and on, my cypher keeps moving like a rolling stone. Baduizm is the groundbreaking debut album by Erykah Badu, released in early 1997 by Kedar and Universal Records. The album a cornerstone of the neo-soul movement, blending elements of R&B, jazz, and hip-hop in an experimentally-retro musical experience, which drew favorable comparisons from the critics of the time to soul music of the 70s. Badu's sound is recognizable for her sultry vocals and introspective or socially conscious lyrics, while the album’s production stands out with warm, rich live instrumentation. Featuring the singles On & On and Next Lifetime, the album not only stood tall as a leading example in the evolution of the soul genre but also established her as a new force in contemporary music, leaving an indelible mark on the world of R&B and soul moving forward. Baduizm debuted at #2 on the US Billboard charts and #1 on the Billboard R&B/Hip-Hop Album charts, and is certified triple Platinum in the US; it would also win the Grammy for Best R&B Album under the banner of neo-soul.
EMOUSE: The Vacanti Mouse Emails: In August 1997, scientists Charles and Joseph Vacanti published a paper in the journal Plastic and Reconstructive Surgery regarding their research into tissue engineering and regenerative medicine. In the journal, they printed a picture of a mouse with what appeared to be a human ear growing out of its back; within months of this publication, every one of your aunts would forward you an email chain containing the “Ear Mouse” photo, proclaiming it to be a horrifying example of genetic modification and decrying the ethics of scientists playing God. (Note: I’ve opted to show you a cuter photo for the bracket.) In actuality, to create the ear-like structure on the mouse's back, researchers implanted a biodegradable scaffold made of a synthetic material seeded with cartilage cells into the mouse. Over time, the cartilage cells grew and formed the ear-shaped structure; no genetic modification was involved. This did not stop the spread of the emails, which often lacked text entirely or misrepresented what was shown, and incensed both animal rights activists and far-right religious groups to the extent that full-page ads were taken out in major news publications like the New York Times expressing outrage. While the photo remains divisive today, the Vacanti Mouse is an iconic image in discussions about the potential of regenerative medicine and the ethical considerations surrounding such research.
6 notes
·
View notes
Text
Xrumon Arigah’s Surgical Extraction Procedure
AS WRITTEN AND DICTATED BY ULLANE WISTIM, M.D, IN THE CROWN CLINIC
PREP BEFORE OPERATION:
All surgeons will be fed, watered, well rested, and having used the restroom. They will have woken up shortly before the operation starts due to its duration. All surgeons will be wearing proper attire - head covers, masks, and scrub suits. Sterile scrub suits, shoes, gloves, and goggles will be provided by the clinic, donned upon entry into the operating room to provide the lowest possible bacterial contamination during the operation.
The operating theater will be prepared with sufficient room to remove, lift, and rotate sections of the suit to provide access to all the systems requiring detachment. These sections will be held and maneuvered with hover-tech above the operating surgeons for maximum ease of access to the patient and a clear field for operations.
The patient will have been scanned by intraoperative CT prior to the operation, and 3D imagery of these scans will be displayed on wall screens to give surgeons access to all information about his internal organs. These images can be changed at will with simple voice commands.
Lard’s note: The person in the suit would be kept standing while being worked on - picture a sort of medical stand, keeping the patient upright even while unconscious, which allows mechanics/doctors access to everything except the literal soles of the feet. Which they shouldn't need access to, and even if they did, they could just move the stand onto a lift of some kind.
Surgeons will be sustained via nutritionally sufficient meal drinks provided. Short rest breaks will be allowed in shifts. Surgeons requiring restroom breaks will need to be sterilized before returning to the operating room.
Robots will be present with biological waste containers to ferry waste away for autoclaving. Robots will also be present to bring and take away tools and equipment.
The suit will be put into maintenance mode so that the subject can be safely operated on, and general anesthetic will be performed. A catheter will be inserted and IVs will be connected to supply sources on movable, levitating robots. The subject will have already undergone fasting, being shaved, and using clinic-issue painkillers for two weeks instead of his standard variety.
LIST OF SURGICAL TOOLS:
Harmonic Scalpel - A surgical instrument used to simultaneously cut and cauterize tissue. Ultrasonic energy is converted to mechanical energy at the active blade to apply pressure and then seal with a denatured protein coagulum. It has almost no thermal spread and smoke production, making it the safest model possible.
Protective Goggles - In appearance they are ordinary surgical goggles, and provide protection from spurts of blood and other bodily fluids the same way. They can be adjusted with the tap of a button or voice command to provide advanced perception of oxygenated versus un-oxygenated blood, and generate vein road maps.
LIST OF CLINIC INVENTIONS:
Internal Laryngeal Rebreather - A liquid that goes down the patient’s throat and expands into the airway, becoming an internal, independent structure instead of an external mask that would impede other surgeries. The oxygen intake is reduced, so it is inadvisable to wear for long periods - this is why it will only be utilized in later stages of the surgery.
Regenerative Serum - A substance derived from Thrixe Varzim’s tissue that allows for controlled regeneration of a troll’s body. It speeds healing and can allow for minor regrowth of lost biological matter. It must be used in small doses to not accidentally cause cancerous activity or undo surgical work, as it only regrows tissue in its natural form.
Bio-Sponge Buds - A modified version of the synthetic troll flesh already utilized by the clinic, these have been custom engineered by Ullane Wistim out of Xrumon Arigah’s DNA, monofilament fibers, and a bioabsorbable scaffold for optimal integration and structural integrity in his stomach wall.
Nanotechnology - A specific brand created by Friday Lovely, these tiny repair vehicles made of her own radiation-resistant DNA can be programmed to fulfill a variety of tasks during surgery.
SURGERY PROCESSES, IN ORDER:
VISUAL SENSOR SUITE DISCONNECTION STEPS:
Due to it hampering the eventual necessary removal of the helmet, the visual sensor suite will be disconnected first.
The head will be held in an altered 3-pin skull fixation device (to accommodate the patient’s standing position) to keep it absolutely still during the surgeries.
3D image-guidance will be used to display the patient’s internal condition to the 3D computer model created from the CT scan.
The helmet sections will be adjusted and slide aside so that the suite may be accessed. The skin will be prepped with an antiseptic. A cranial drill will be used to drill through the skull, exposing the dura, which will be peeled back to allow access to the brain.
The optic nerve will be held and isolated via forceps as it is packed with cottonoids for protection. The cells grown into the conduits will be dissociated by injections of TrypLE, so that the conduits may be safely removed without damaging the vitreous humor.
His eyes will be monitored for their light response (and integrity of the optic nerve) at all times. Regenerative serum will be immediately applied via infused cottonoids if damage is sustained.
The skull bone will be sealed back in place with laser soldering.
It will be likely the patient has sustained minor vision loss due to scar buildup around the conduits, but this will be addressed post-surgery.
PSIIONIC DAMPENER REMOVAL STEPS:
Dioscuri’s Area is the part of the troll brain where psiionic energy is generated; it is tied to voluntary motor functions, as while psiionic abilities are not muscularly based, even passively present powers require focus from the user to manipulate at will.
Fortunately for Xrumon Arigah, he has no psiionic powers, so removal is safe in that regard.
The dampener will be removed second, after the visual sensor suite. After its removal, psiionic influence on the surgery will be possible via Ullane Wistim and Friday Lovely’s psiionics.
Once the helmet sections are slid back to expose the head, the skull will be cut into via cranial drill and the dampener will be located. Once it is located the wires attaching it to the Dioscuri region will be carefully detached via a stent-retriever inserted by way of a micro catheter.
Once all wires have been detached and removed from the body, the dampener itself will be extracted.
As with all neurological operations during this surgery, laser soldering will once more be used to seal the brain tissue after incision and prevent damage and blood loss.
VITAL SIGNS MONITOR REMOVAL STEPS:
The monitor must first be removed from where it is hooked into the patient’s brain stem by PEDOT clusters. Sterile saline solution will be used to sanitize and avoid excess heating while drilling through the skull to reach the clusters, microspheres meshed with the brainstem via hydrogels.
A lighted scope will be used to view the site clearly, and it will be clipped to prevent full circulation so the patient does not die of blood loss. The clusters will be detached via a syringe inserted into the capillary of the correct cerebellar blood vessel to extract all the PEDOTS clusters, earlier located during the CT scan.
A small window will be drilled into the bone above the spinal cord to observe the monitor site and to make it possible to extract after the wires are detached, all the way down to the base of the vertebrae at the conus medullaris.
A few key incisions along the spine will be made to access the scar tissue around the wires, and nanotech delivering extracorporeal shockwave treatment will be used to loosen and draw them out by gentle tissue dissolution around the sites.
The neck muscles will be spread apart to allow extraction via retractor, then a bony well will be drilled to access the monitor, using a silicone replica of the monitor as a guide to ensure the exact necessary depth and no further.
The monitor will then be set aside, along with the wires.
ADVANCED WARFARE CENTER REMOVAL STEPS:
Located prior via CT scan, the microchips will be reached by syringes piercing the brain matter exactly where the chips in their capsules are located.
Care must be taken to ensure the brain tissue itself is not damaged in the process. As is standard, regenerative serum injections will be prepped in case damage is sustained.
The microchips will be set aside and later prepped for hazardous waste disposal.
BREATH OF LIFE SYSTEM REMOVAL STEPS:
Infrared fluorescent imaging will be used to provide a real-time model of the patient’s lungs and the structures within during this operation.
A bite block followed by an endoscope will be placed down the patient’s throat. This will deliver nanotech to dissolve the anti-clotting coating on the cannulas so that hemostatic nanoparticles may temporarily clot his blood to prevent the patient choking to death.
The oxygenator membrane and pump will be disabled by nanotech to safely cut off its power source, then the lung pumps’ tubes loosened and detached gradually. The tubes - cannulas- will be disassembled into their glass and wire components, and removed back up the endoscope via nanotech, and set aside. This step will need to be done incrementally over several minutes to ensure safe disconnect.
The stomach tube will also need to be disconnected and disassembled. All tubes require time to do so properly, as the cannulas are structured to avoid bubbling in the blood vessels and support a smooth transition to prevent improper drainage and maintain regular flow levels.
As the drainage is redirected, more hemostatic particles will both absorb the excess and other nanoparticles will temporarily graft and re-direct blood to other vessels so the patient does not die of blood loss.
In the likely case of damage caused during the surgery, nanotech carrying regenerative serum will also be accompanying the disassembly units. Regardless of additional harm, the vessels the tubes were attached to will need to be repaired by this method before the nanotech is removed once more.
Finally, a ventilator will need to be attached afterward to help his lungs transition back to their usual functionality.
BLOODFLOW REGULATOR REMOVAL STEPS:
The chest will be cut open below the collarbone, and pacing leads will be attached to the cardiac veins. Plastic tubing will be inserted over leads as sheaths to break up the scar tissue that has formed around the vein sites. The leads will be anchored via suture after fixation to prevent dislodging.
The regulator will first be disconnected from the devilfish reflex kit via surgical scissor and the wound sealed via harmonic scalpel, then disconnected from the heart itself.
A smaller, less powerful biventricular pacemaker will be installed to ease the loss of the old one, as his heart has come to depend on it. It will be implanted inside the heart itself, with a lead attached to a vein under the collarbone on one side and the pulse generator on the other.
In time, he will ideally be able to survive without one entirely.
DEVILFISH REFLEX KIT DISCONNECTION STEPS:
All conduits must be disconnected from the relevant nerve clusters without paralyzing the patient from damage to his nervous system or incurring fatal amounts of blood loss. This stage of the surgery will be the longest; surgeons will be swapped out every three hours to ensure focus and quality of work is retained.
The conduits will be cut via robotically operated surgical scissors, and harmonic scalpels will be used to seal openings.
In case of any damage, nerves can later be repaired via a few different methods: suture, grafting, or transfer. This will be a future operation, as any damage will only be able to be patched and not fully repaired in the present moment due to time constraints.
Intraoperative cell salvage will be used to prevent the patient from dying of blood loss. Blood will be collected, combined with anticoagulant, centrifuged, washed in saline, and reinfused periodically by robots.
One to two surgeons will operate a robotic set of instruments to detach the conduits - allowing for tremor-free and magnified views of the vital nerve clusters - as others perform the cell salvage and set the conduits aside, ready to pause in case of emergencies.
ANTI-TOXIN FILTER DISCONNECTION STEPS:
The dialysis units in the suit must be safely disconnected from the subject’s kidneys and liver.
The hazards primarily involve not tearing the fistula connection site, as the vein and artery connection will bleed heavily if torn during removal of the tubing. Excessive clotting should also be avoided.
The units must be prepared for removal. First, they will be switched off, then the units’ lines must be clamped to prevent blood loss. Saline will be kept on hand for emergency infusions, and to flush the tubes in case of any clotting.
Nanotech will be used to disconnect the tubing by loosening it safely so the needles may be removed nigh-instantly via scalpel and needle pliers. To best prevent damage and complications, they will be removed at the same angle they were inserted, and with moderate compression via nanotech to both prevent excess clotting and circulatory issues.
The kidney units themselves must be removed via disassembly via laparoscopic tube (similarly to the breath of life regulator) and the wound sealed shut using subcuticular stitches (placed below the epidermal layer).
The liver unit will follow the same process, using the same supracostal incision.
An external dialysis machine will then be connected following the removal of all three units, to ensure his body does not fail from the dependence it will have likely developed during its time connected to the units.
IRON JAW REMOVAL STEPS:
The front portion of the helmet will be opened, and the mouth propped open with gags.
The patient will be switched from breathing tubes to the laryngeal airway mask substitute, allowing direct access to the tongue.
This substitute will also contain the solvent to dissolve the hardening compound. When the compound is fully dissolved, it and the solvent can be safely swallowed.
WAVEFORM ALIGNMENT RETICULATOR DISCONNECTION STEPS:
First, the stomach will be inflated via tube-delivered carbon dioxide gas, to have a clear view of its lining.
The reticulator itself will first be secured via medical cinch. The clips holding the tube in place will be removed and the primed buds containing bio-sponge will be placed in the clips’ former locations via syringe.
The tube will be pulled through the stomach wall as the buds generate and weave a mesh plug over the hole in under a second. This allows for the minimum possible leakage.
The tube removal site will then be covered with a sterile dressing to further contain any later leakage and later changed periodically.
Afterward, the patient will receive medication to reduce his stomach acid to facilitate the healing and successful graft of the stomach hole.
CLEAN CHAIN REACTOR REMOVAL STEPS:
The final operation. Before it is undertaken, the vitals of the suit must be checked to ensure all other disconnects are stable.
Xrumon must still be under anesthesia, and he must be stabilized with fresh IV fluids to prepare him for full life-support disconnect.
Much like the reticulator, the reactor will follow similar securement and removal steps, and the stomach hole will need to be plugged using the same method as above. Once this hole is plugged, Xrumon will be fully detached from the suit.
Once this is done, the patient laid down to rest and stabilize with the aforementioned post-surgery procedures and the suit itself must be set aside.
---
ULLANE’S BACKUP PLAN, SHOULD XRUMON SEEM IRRECOVERABLY ON THE BRINK OF DEATH, AS A PROJECTED MESSAGE THAT WILL SHOW IF SHE IS COMPELLED TO USE UP ALL HER PSIIONIC ENERGY IN ONE BURST:
To my staff, should this be necessary -
I have had ports installed under my skin like those of helms. So that if I must overclock my psiionics to save Xrumon’s life, I will be immediately stabilized, preventing brain damage if not other damage from burnout, though the ports are also designed to release regenerative serum if such occurs. I have fallen unconscious, and can be safely revived after an hour’s rest.
I know you’ll question me; you’re right to. But even if this is the wrong decision, it’s the one I’m making. Ideally, it won’t be necessary.
I would still rather do it to ensure his survival. He must live.
No matter what.
- Ullane Wistim
5 notes
·
View notes
Text
Towards clinical application of tissue engineering for erectile penile regeneration | Nature Reviews Urology (paywalled)
Towards clinical application of tissue engineering for erectile penile regeneration (unpaywalled)
Complete Human Penile Scaffold for Composite Tissue Engineering: Organ Decellularization and Characterization | Scientific Reports (as linked above, no paywall, contains explicit images)
Complete Human Penile Scaffold for Composite Tissue Engineering: Organ Decellularization and Characterization | Scientific Reports (alternate, contains explicit images)
Engineered penis raises reproduction hopes | Nature (as linked above, paywalled)
@spacedembers @georgington
the people are hungry for penis knowledge and i must provide
so: y'all remember that post about them turning a grape into a meat grape? basically using the same process (de/recellularization), scientists are aiming to create transplantable penises w/o the concerns that come with organ transplants by removing the cells from a donor to get a scaffold, and replacing them with the receiver's cells, which would avoid concerns about rejection
here's the article I read on it; disclaimer that's it's a Very scientific read. I do not know much about science, but this process has seen success in rabbits who had their penises surgically removed; they were able to achieve full erections and impregnate female rabbits. I don't think trans humans will be able to ejaculate anytime soon, but if this becomes an accessible form of surgery it would mean we could have a form of phalloplasty that allows for natural erections!!! which is really really cool. obviously this is all far from available for us rn but it's very exciting to see this being researched, and it seems like we have good reason to be optimistic about the future!
also, the article specifically mentions trans surgery, which is really nice. a lot of articles about penis reconstruction/transplants I've seen only focus on the potential benefits for cis men, so i really appreciate that they bring up how this has the potential to revolutionize bottom surgery
2K notes
·
View notes
Text
The Chrysalid Sky of Guadalajara
Enrique Venne’s body was no longer his own. His spine arched into a serrated curve, vertebrae bristling with quill-like hairs that tasted the air. The city below him had dissolved into a living scaffold, its streets replaced by pulsating veins that pumped ichor instead of blood. The sky-mass—now a vast, translucent chrysalis—throbbed with unborn light.
Survivors called it El Nido. The Nest.
A ragged coalition of engineers, soldiers, and children had fortified themselves in Guadalajara’s abandoned subway tunnels. Their leader, General Ríos, wore a hazmat suit welded to her skin, her voice a static hiss through a corroded respirator. “We bombed the sky three times,” she told Enrique, not knowing what he’d become. “The explosives just… fed it.”
Enrique said nothing. His new tongue—forked and glandular—could no longer shape human words. But he showed her. With a touch, he flooded her mind with visions: the chrysalis breathing in radiation, converting missiles into sugars, war into sustenance. The Nest was not a structure. It was a womb.
That night, the first tendrils descended. Ribboned cords of bioluminescent tissue, dripping with amniotic acid. They slithered into the tunnels, seeking the uninfected. A child’s scream echoed as a tendril plugged into her sternum, flooding her with black nectar. Within minutes, her skin split into featherless wings.
General Ríos ordered a retreat to the surface. What they found there froze even Enrique’s alien blood.
The Nest had birthed seeds. Floating orbs the size of stadiums, their surfaces veined with gravitational filaments. They hovered over cities, their shadows etching fractal patterns into the ruined earth. Mexico City’s seed had already rooted, its tendrils drilling into the mantle. Magma geysered upward, only to be siphoned into the orb’s core. The planet was being drained.
Enrique felt the others now—minds like his, scattered across the globe. A former botanist in Lagos, her roots fused with a seed’s mycelium. A fisherman in Jakarta, his lungs adapted to breathe molten silica. They shared a single thought: Prepare the way.
General Ríos aimed her rifle at Enrique. “Fix this,” she demanded. “You made it. Unmake it.”
He couldn’t. But he offered her truth. With a twitch of his quills, he dissolved her hazmat suit, her skin, her bones—revealing the larvae already nesting in her marrow. She’d been infected for weeks. They all had.
The survivors turned on each other, their bodies betraying them mid-scream. One man’s skull peeled into a flower of cartilage, pollen swirling with viral RNA. Another liquefied, his remains seeping into the ground to fertilize the soil.
Enrique ascended. His flesh unraveled as he climbed the Nest’s umbilical cord, joining the swarm of prophets. Below, the seeds shuddered. Their shells cracked, releasing not life, but antilife—a vacuum that devoured sound, light, and finally, gravity. Mexico City’s ruins collapsed into a singularity no wider than a coin.
The chrysalis darkened. The sky folded.
Enrique’s mind dissolved into the chorus. He understood now. The seeds were not weapons. They were mouths.
Earth’s molten core gushed into the void, chewed and processed into a scream that echoed through dimensions. A scream meant to be heard. A scream that was, unmistakably, an invitation.
As the last prophets merged with the Nest, the chrysalis hardened into a obsidian shell. Inside, something ancient stretched new limbs.
On the dead streets of Guadalajara, the air turned to glass.
And the stars began to go out.
0 notes
Text
Biomaterials for Tissue Engineering Market Trends, Challenges, and Forecast 2028
The Biomaterials for Tissue Engineering Market sector is undergoing rapid transformation, with significant growth and innovations expected by 2028. In-depth market research offers a thorough analysis of market size, share, and emerging trends, providing essential insights into its expansion potential. The report explores market segmentation and definitions, emphasizing key components and growth drivers. Through the use of SWOT and PESTEL analyses, it evaluates the sector’s strengths, weaknesses, opportunities, and threats, while considering political, economic, social, technological, environmental, and legal influences. Expert evaluations of competitor strategies and recent developments shed light on geographical trends and forecast the market’s future direction, creating a solid framework for strategic planning and investment decisions.
Brief Overview of the Biomaterials for Tissue Engineering Market:
The global Biomaterials for Tissue Engineering Market is expected to experience substantial growth between 2024 and 2028. Starting from a steady growth rate in 2023, the market is anticipated to accelerate due to increasing strategic initiatives by key market players throughout the forecast period.
Get a Sample PDF of Report - https://www.databridgemarketresearch.com/request-a-sample/?dbmr=global-biomaterials-for-tissue-engineering-market
Which are the top companies operating in the Biomaterials for Tissue Engineering Market?
The report profiles noticeable organizations working in the water purifier showcase and the triumphant methodologies received by them. It likewise reveals insights about the share held by each organization and their contribution to the market's extension. This Global Biomaterials for Tissue Engineering Market report provides the information of the Top Companies in Biomaterials for Tissue Engineering Market in the market their business strategy, financial situation etc.
1 SYNTHECON, INCORPORATED, Dr Lal PathLabs, NeoGenomics Laboratories, Inc., Abbott, Solvay, Olympus Terumo Biomaterials Corporation, Berkeley Advanced Biomaterials, Avery Therapeutics, Inc., Biomimetic Solutions The Lubrizol Corporation, CRS Holdings Inc., CAM Bioceramics B.V., Zimmer Biomet, Wright Medical Group N.V, GELITA AG , Victrex plc, and MATEXCEL
Report Scope and Market Segmentation
Which are the driving factors of the Biomaterials for Tissue Engineering Market?
The driving factors of the Biomaterials for Tissue Engineering Market are multifaceted and crucial for its growth and development. Technological advancements play a significant role by enhancing product efficiency, reducing costs, and introducing innovative features that cater to evolving consumer demands. Rising consumer interest and demand for keyword-related products and services further fuel market expansion. Favorable economic conditions, including increased disposable incomes, enable higher consumer spending, which benefits the market. Supportive regulatory environments, with policies that provide incentives and subsidies, also encourage growth, while globalization opens new opportunities by expanding market reach and international trade.
Biomaterials for Tissue Engineering Market - Competitive and Segmentation Analysis:
**Segments**
- Based on material type, the biomaterials for tissue engineering market can be segmented into synthetic materials, natural materials, and hybrid materials. Synthetic materials such as polymers, ceramics, and composite materials offer advantages like tunable properties and controlled degradation rates. Natural materials derived from sources like collagen, gelatin, and silk provide biocompatibility and bioactivity for tissue regeneration. Hybrid materials combine the advantages of both synthetic and natural materials to enhance the performance of scaffolds in tissue engineering applications.
- On the basis of application, the market can be categorized into orthopedic, cardiovascular, neurological, dental, and soft tissue applications. Orthopedic applications dominate the market due to the high demand for biomaterials in bone grafting, cartilage repair, and joint replacements. Cardiovascular applications are witnessing significant growth with the development of vascular grafts, heart valves, and stents using biomaterials. Neurological applications for nerve regeneration and brain tissue engineering are also gaining traction in the market.
- By end-user, the biomaterials for tissue engineering market is segmented into hospitals, research institutes, and academic institutes. Hospitals represent the largest end-user segment due to the increasing number of surgical procedures requiring biomaterial-based implants. Research institutes play a crucial role in driving innovation and product development in the market, while academic institutes contribute to educating future professionals in the field of tissue engineering.
**Market Players**
- Some of the key players in the global biomaterials for tissue engineering market include Zimmer Biomet, Acelity L.P. Inc., Medtronic, Invibio Ltd., and Stryker Corporation. These companies focus on strategic partnerships, product launches, and acquisitions to strengthen their market position and expand their product portfolios. Zimmer Biomet, for instance, offers a wide range of biomaterials for orthopedic and dental applications, catering to the diverse needs of healthcare providers globally. Acelity L.P. Inc. specializes in wound care products using advanced biomThe global biomaterials for tissue engineering market is experiencing significant growth and evolution due to the increasing demand for advanced materials in regenerative medicine and tissue repair applications. The market segmentation based on material type highlights the diverse options available to researchers and healthcare providers. Synthetic materials offer tunable properties and controlled degradation rates, making them ideal for a wide range of tissue engineering applications. Natural materials provide biocompatibility and bioactivity, crucial for promoting tissue regeneration effectively. Hybrid materials combine the best of both worlds, leveraging the advantages of synthetic and natural materials to enhance scaffold performance in tissue engineering.
In terms of application segmentation, orthopedic applications emerge as the dominant segment in the biomaterials for tissue engineering market. The high demand for biomaterials in orthopedic procedures such as bone grafting, cartilage repair, and joint replacements drives growth in this segment. Cardiovascular applications are also gaining momentum, with the development of vascular grafts, heart valves, and stents using biomaterials to address cardiac health needs. Neurological applications for nerve regeneration and brain tissue engineering represent a niche but growing segment within the market, as researchers explore innovative solutions for neurological disorders and injuries.
The end-user segmentation of the biomaterials for tissue engineering market reflects the diverse stakeholders involved in driving market growth and innovation. Hospitals constitute the largest end-user segment, as the demand for biomaterial-based implants in surgical procedures continues to rise globally. Research institutes play a crucial role in advancing the field of tissue engineering through innovative research and product development collaborations with market players. Academic institutes contribute to shaping the future of the industry by educating and training the next generation of professionals in the field of tissue engineering, ensuring a steady supply of talent and expertise.
Key players in the global biomaterials for tissue engineering market, such as Zimmer Biomet, Acelity L.P. Inc., Medtronic, Invibio Ltd., and Stryker Corporation, drive market growth through strategic initiatives and product innovations. These companies invest in partnerships,**Market Players**
SYNTHECON, INCORPORATED, Dr Lal PathLabs, NeoGenomics Laboratories, Inc., Abbott, Solvay, Olympus Terumo Biomaterials Corporation, Berkeley Advanced Biomaterials, Avery Therapeutics, Inc., Biomimetic Solutions The Lubrizol Corporation, CRS Holdings Inc., CAM Bioceramics B.V., Zimmer Biomet, Wright Medical Group N.V, GELITA AG , Victrex plc, and MATEXCEL.
The global biomaterials for tissue engineering market is a dynamic and growing sector driven by the increasing demand for advanced materials in regenerative medicine and tissue repair applications. The segmentation of the market based on material type provides researchers and healthcare providers with a wide array of options to choose from, ranging from synthetic materials offering tunable properties to natural materials providing biocompatibility and bioactivity, as well as hybrid materials combining the strengths of both. This diversity in material types allows for tailored solutions to various tissue engineering needs, ultimately contributing to the market's expansion.
In terms of application segmentation, orthopedic applications continue to be the dominant segment in the biomaterials for tissue engineering market, fueled by the persistently high demand for biomaterials in procedures such as bone grafting and joint replacements. The cardiovascular segment is also on the rise, driven by the development of biomaterial-based solutions for vascular grafts and heart valves. Neurological applications represent a niche but promising area for growth, as research explores innovative approaches
North America, particularly the United States, will continue to exert significant influence that cannot be overlooked. Any shifts in the United States could impact the development trajectory of the Biomaterials for Tissue Engineering Market. The North American market is poised for substantial growth over the forecast period. The region benefits from widespread adoption of advanced technologies and the presence of major industry players, creating abundant growth opportunities.
Similarly, Europe plays a crucial role in the global Biomaterials for Tissue Engineering Market, expected to exhibit impressive growth in CAGR from 2024 to 2028.
Explore Further Details about This Research Biomaterials for Tissue Engineering Market Report https://www.databridgemarketresearch.com/reports/global-biomaterials-for-tissue-engineering-market
Key Benefits for Industry Participants and Stakeholders: –
Industry drivers, trends, restraints, and opportunities are covered in the study.
Neutral perspective on the Biomaterials for Tissue Engineering Market scenario
Recent industry growth and new developments
Competitive landscape and strategies of key companies
The Historical, current, and estimated Biomaterials for Tissue Engineering Market size in terms of value and size
In-depth, comprehensive analysis and forecasting of the Biomaterials for Tissue Engineering Market
Geographically, the detailed analysis of consumption, revenue, market share and growth rate, historical data and forecast (2024-2028) of the following regions are covered in Chapters
The countries covered in the Biomaterials for Tissue Engineering Market report are U.S., Canada, Mexico, Brazil, Argentina, Rest of South America, Germany, Italy, U.K., France, Spain, Netherlands, Belgium, Switzerland, Turkey, Russia, Rest of Europe, Japan, China, India, South Korea, Australia, Singapore, Malaysia, Thailand, Indonesia, Philippines, Rest of Asia-Pacific, Saudi Arabia, U.A.E, South Africa, Egypt, Israel, and Rest of the Middle East and Africa
Detailed TOC of Biomaterials for Tissue Engineering Market Insights and Forecast to 2028
Part 01: Executive Summary
Part 02: Scope Of The Report
Part 03: Research Methodology
Part 04: Biomaterials for Tissue Engineering Market Landscape
Part 05: Pipeline Analysis
Part 06: Biomaterials for Tissue Engineering Market Sizing
Part 07: Five Forces Analysis
Part 08: Biomaterials for Tissue Engineering Market Segmentation
Part 09: Customer Landscape
Part 10: Regional Landscape
Part 11: Decision Framework
Part 12: Drivers And Challenges
Part 13: Biomaterials for Tissue Engineering Market Trends
Part 14: Vendor Landscape
Part 15: Vendor Analysis
Part 16: Appendix
Browse More Reports:
North America Protein Purification - Isolation Market – Industry Trends and Forecast North America Bioinformatics Market – Industry Trends and Forecast North America Next Generation Sequencing (NGS) Market – Industry Trends and Forecast North America Western Blotting Market – Industry Trends and Forecast North America Cardiac Monitoring and Cardiac Rhythm Management Devices Market – Industry Trends and Forecast North America Human Insulin Drugs and Delivery Devices Market – Industry Trends and Forecast North America Leukemia Therapeutics Market – Industry Trends and Forecast North America Autologous Stem Cell and Non-Stem Cell Based Therapies Market – Industry Trends and Forecast Europe Home Healthcare Market – Industry Trends and Forecast Europe Anti-Snoring Devices and Snoring Surgery Market – Industry Trends and Forecast Europe Orthodontic Supplies Market – Industry Trends and Forecast Europe Intraoperative Imaging Market – Industry Trends and Forecast Europe Biosurgery Market – Industry Trends and Forecast Europe Interventional Cardiology and Peripheral Vascular Devices Market – Industry Trends and Forecast Europe Transplant Diagnostics Market – Industry Trends and Forecast
Data Bridge Market Research:
Today's trends are a great way to predict future events!
Data Bridge Market Research is a market research and consulting company that stands out for its innovative and distinctive approach, as well as its unmatched resilience and integrated methods. We are dedicated to identifying the best market opportunities, and providing insightful information that will help your business thrive in the marketplace. Data Bridge offers tailored solutions to complex business challenges. This facilitates a smooth decision-making process. Data Bridge was founded in Pune in 2015. It is the product of deep wisdom and experience.
Contact Us:
Data Bridge Market Research
US: +1 614 591 3140
UK: +44 845 154 9652
APAC: +653 1251 978
Email:- [email protected]
#Biomaterials for Tissue Engineering Market#Biomaterials for Tissue Engineering Market size#Biomaterials for Tissue Engineering Market scope
0 notes
Text
Kombucha-derived bioink developed for personalized tissue repair
0 notes
Text

Researchers develop novel 'bone bandage' material for cracked bones
Bone regeneration is a complex process, and existing methods to aid regeneration including transplants and growth factor transmissions face limitations such as the high cost. But recently, a piezoelectric material that can promote the growth of bone tissue has been developed. A KAIST research team led by Professor Seungbum Hong from the Department of Materials Science and Engineering (DMSE) has developed a biomimetic scaffold that generates electrical signals upon the application of pressure by utilizing the unique osteogenic ability of hydroxyapatite (HAp). HAp is a basic calcium phosphate material found in bones and teeth. This biocompatible mineral substance is also known to prevent tooth decay and is often used in toothpaste. This research was conducted in collaboration with a team led by Professor Jangho Kim from the Department of Convergence Biosystems Engineering at Chonnam National University. The results are published in the journal ACS Applied Materials & Interfaces.
Read more.
24 notes
·
View notes
Photo

Growing Heart
Growing up in a supportive environment is at the heart of healthy, happy development. This is true for our hearts as well, and scientists engineering heart tissue from stem cells (starter cells with limitless development potential) for use in research and healthcare are trying to create that perfect environment. Engineered heart tissue is often limited because the cells don’t fully mature, restricting their use. A new 3D printing technique using an electric field to precisely deposit materials and create complex structures has produced an ideal microenvironment to support maturation, like a loving home or enriching school. Heart tissue grew along the structure (pictured, with fibrous actin in red and a heart cell protein in green) and showed mature cell behaviours. Scaffolds like this could help create new tissue to repair damage rather than relying on scarce donor hearts, and also provide material for tests of new potential treatments.
Written by Anthony Lewis
Image from work by Guangming Zhang and colleagues
Shandong Engineering Research Center for Additive Manufacturing, Qingdao University of Technology, Qingdao, China
Image originally published with a Creative Commons Attribution 4.0 International (CC BY 4.0)
Published in Advanced Science, February 2023
You can also follow BPoD on Instagram, Twitter and Facebook
#science#biomedicine#stem cells#3D printing#actin#immunofluorescence#tissue regeneration#heart cells#cardiomyocytes
16 notes
·
View notes
Text
Tooth Regeneration Market Insights Regional Market Growth and Expansion
The tooth regeneration market is rapidly evolving, driven by groundbreaking advancements in regenerative medicine, stem cell therapy, and biotechnology. Unlike traditional dental implants, bridges, or dentures, tooth regeneration focuses on biological restoration, stimulating the body’s natural ability to regrow lost or damaged teeth. This revolutionary approach is transforming dental care by providing long-lasting and more natural solutions to address tooth loss caused by aging, trauma, or disease.
With an increasing global demand for innovative dental treatments, the tooth regeneration market is attracting substantial investments from biotech firms, research institutions, and healthcare companies. This article explores key market insights, growth drivers, technological innovations, challenges, and future market projections.
Market Growth Drivers and Opportunities
The growth of the tooth regeneration market is fueled by several critical factors, including:
Rising Prevalence of Dental Disorders – Conditions like periodontitis, tooth decay, and oral trauma are increasing globally, creating a strong demand for regenerative dental solutions.
Aging Population – As life expectancy rises, more individuals suffer from tooth loss due to aging, boosting demand for long-term and biological tooth replacement options.
Technological Advancements – Innovations in stem cell research, tissue engineering, and biomaterials are accelerating the development of effective tooth regeneration therapies.
Consumer Awareness and Demand – Patients are increasingly seeking natural, long-lasting alternatives to traditional prosthetic dental solutions, driving market expansion.
Investment in Regenerative Medicine – Governments, private investors, and research institutions are funding new studies and clinical trials, supporting the growth of regenerative dentistry.
Role of Stem Cells and Biotechnology in Tooth Regeneration
Stem cell therapy is at the core of tooth regeneration, offering immense potential for regrowing dental tissues such as dentin, enamel, and pulp. Scientists are leveraging various types of stem cells, including:
Dental Pulp Stem Cells (DPSCs) – Found in tooth pulp, these cells show strong regenerative potential for dental tissue repair.
Mesenchymal Stem Cells (MSCs) – Derived from bone marrow or adipose tissue, MSCs play a crucial role in stimulating tooth regeneration.
Induced Pluripotent Stem Cells (iPSCs) – These reprogrammed adult cells mimic embryonic stem cells and hold promise for customized regenerative treatments.
Biotechnology companies are also exploring genetic engineering, bioactive molecules, and scaffolding technologies to enhance tooth regrowth. The integration of bioprinting and tissue engineering is expected to revolutionize the market in the coming years.
Emerging Technologies and Innovations in the Market
The field of tooth regeneration is witnessing rapid technological advancements, including:
Biodegradable Scaffolds – These structures act as a framework for tooth regrowth, supporting cell attachment and tissue formation before dissolving naturally.
3D Bioprinting – Researchers are using 3D printing to create complex, patient-specific tooth structures from living cells and biomaterials.
Growth Factor-Based Therapies – Bioactive proteins and molecules stimulate stem cells to regenerate tooth tissues effectively.
Gene Therapy – Scientists are exploring genetic modification techniques to activate tooth regrowth mechanisms in patients.
Nanotechnology in Regenerative Dentistry – Nanomaterials are being developed to enhance the efficiency of stem cell therapy and improve dental tissue regeneration.
Competitive Landscape and Key Players
The tooth regeneration market is highly competitive, with biotech firms, pharmaceutical companies, and research institutions leading the race to develop commercialized treatments. Some of the key players involved in this sector include:
Straumann Group – A global leader in dental solutions, investing heavily in regenerative dentistry technologies.
BioEden – A pioneer in dental stem cell storage and regenerative applications.
Riken Center for Developmental Biology – Conducting cutting-edge research on tooth regeneration using stem cells.
Unilever and Other Consumer Goods Companies – Exploring innovative oral health products with regenerative properties.
These companies are actively collaborating with academic institutions and government agencies to accelerate research and clinical trials.
Regulatory and Ethical Challenges in the Market
Despite its promising potential, the tooth regeneration market faces several regulatory and ethical hurdles, including:
Stringent Approval Processes – Regulatory bodies such as the FDA and EMA impose strict guidelines on regenerative therapies, delaying market entry.
High Costs of Research and Treatment – The complexity of developing safe and effective regenerative treatments results in high costs, limiting accessibility.
Ethical Concerns Over Stem Cell Use – The sourcing and manipulation of stem cells, especially embryonic stem cells, raise bioethical issues.
Long Clinical Trial Durations – Tooth regeneration therapies require extensive testing and validation, prolonging commercialization timelines.
Limited Public Awareness – Many patients remain unaware of regenerative dental treatments, affecting early market adoption.
Regional Market Growth and Expansion
The tooth regeneration market is expanding globally, with significant growth observed in:
North America – The U.S. leads in research funding, clinical trials, and technological innovation.
Europe – Countries like Germany, the UK, and Switzerland are investing in regenerative dental research.
Asia-Pacific – Japan and China are emerging as key players due to advancements in biotechnology and government support for regenerative medicine.
Latin America & Middle East – These regions are gradually adopting advanced dental solutions, but market penetration remains low.
Future Outlook and Industry Forecast
The future of the tooth regeneration market looks promising, with several key trends expected to shape the industry:
Increased Commercialization of Regenerative Dental Products – More biotech companies are expected to launch clinically approved products in the coming years.
Integration of AI and Digital Health in Regenerative Dentistry – AI-driven diagnostics and treatment planning will enhance precision in regenerative dental care.
More Clinical Trials and FDA Approvals – As research progresses, more regenerative therapies will receive regulatory approval, increasing market accessibility.
Affordability and Accessibility Improvements – With further advancements, costs are expected to decrease, making tooth regeneration treatments more affordable.
Consumer Acceptance and Market Expansion – Growing awareness and education about regenerative dentistry will drive adoption among patients and dental professionals.
Conclusion
The tooth regeneration market represents a transformative shift in dentistry, offering natural, long-term solutions for tooth loss. While technological advancements, stem cell research, and biotech innovations continue to drive the market, challenges such as regulatory approvals, high costs, and ethical considerations need to be addressed. As clinical trials progress and commercialization accelerates, tooth regeneration has the potential to become a mainstream dental treatment, significantly improving oral health worldwide.
#Tooth Regeneration Market#Tooth Regeneration Market trends#Tooth Regeneration#Tooth Regeneration treatement#Tooth Regeneration medications
0 notes