#CO2 feedstock
Explore tagged Tumblr posts
Text
Avantium strengthened its partnership with SCG Chemicals to pilot the production of PLGA
CEO Tom Van Aken and Her Majesty Queen Maxima. Source: Avantium Avantium N.V., a leading company in renewable and circular polymer materials, strengthened its partnership with SCG Chemicals Public Company Limited, a leading chemical player in Asia and an innovator of chemical innovations and solutions. The companies signed a multi-year collaboration to pilot the production of PLGA…

View On WordPress
0 notes
Text
The synthetic biology and precision fermentation space is a hotbed of entrepreneurial activity these days. But it’s not every day you come across a startup that’s using genetic engineering to produce natural rubber — a substance that’s challenging to reproduce in a lab because of how long its polymer is. Paris-based baCta has a proof of concept up and running that uses engineered bacteria (Escherichia coli) to yield natural rubber in vitro. The startup says its method, which relies upon a renewable feedstock — currently it’s using glucose but is aiming to diversify into acetate and carbon — is carbon neutral.
Continue Reading.
126 notes
·
View notes
Text
Excerpt from this story from RMI:
1. Batteries Become Everybody’s Best Friend
Battery prices continue to drop and their capacity continues to rise. The cost of electric vehicle (EV) batteries are now about 60 percent what they were just five years ago. And around the world, batteries have become key components in solar-plus-storage microgrids, giving people access to reliable power and saving the day for communities this past hurricane season.
2. Americans Get Cheaper (and Cleaner) Energy
State public utility commissions and rural electric co-operatives around the country are taking steps to deliver better service for their customers that also lowers their rates. At the same time, real momentum is building to prevent vertically integrated utilities from preferencing their coal assets when there are cleaner and cheaper alternatives available.
3. A Sustainable Shipping Future Gets Closer
More than 50 leaders across the marine shipping value chain — from e-fuel producers to vessel and cargo owners, to ports and equipment manufacturers — signed a Call to Action at the UN climate change conference (COP29) to accelerate the adoption of zero-emission fuels. The joint statement calls for faster and bolder action to increase the use of zero and near-zero emissions fuel, investment in zero-emissions vessels, and global development of green hydrogen infrastructure, leaving no country behind.
4. Corporations Fly Cleaner
In April, 20 corporations, including Netflix, JPMorgan Chase, Autodesk, and more, committed to purchase about 50 million gallons of sustainable aviation fuel (SAF), avoiding 500,000 tons of CO2 emissions — equivalent to the emissions of 3,000 fully loaded passenger flights from New York City to London. SAF is made with renewable or waste feedstocks and can be used in today’s aircraft without investments to upgrade existing fleets and infrastructure.
5. More and More Places Go From Coal to Clean
Around the world, coal-fired power plants are closing down as communities switch to clean energy. From Chile to the Philippines to Minnesota coal-to-clean projects are creating new jobs, improving local economic development, and generating clean electricity. In September, Britain became the first G7 nation to stop generating electricity from coal — it’s turning its last coal-fired power plant into a low-carbon energy hub. And in Indonesia, the president vowed to retire all coal plants within 15 years and install 75 gigawatts of renewable energy.
6. Methane Becomes More Visible, and Easier to Mitigate
Methane — a super-potent greenhouse gas — got much easier to track thanks to the launch of new methane tracking satellites over the past year. In March, the Environmental Defense Fund launched MethaneSAT, the first for a non-governmental organization, and the Carbon Mapper Coalition soon followed with the launch of Tanager-1. By scanning the planet many times each day and identifying major methane leaks from orbit, these new satellites will put pressure on big emitters to clean up.
7. EVs Speed By Historic Milestones
This past year was the first time any country had more fully electric cars than gas-powered cars on the roads. It’s no surprise that this happened in Norway where electric cars now make up more than 90 percent of new vehicle sales. And in October, the United States hit a milestone, with over 200,000 electric vehicle charging ports installed nationwide.
8. Consumers Continue to Shift to Energy-Efficient Heat Pumps for Heating and Cooling
Heat pumps have outsold gas furnaces consistently since 2021. And while shipments of heating and cooling equipment fell worldwide in 2023, likely due to broad economic headwinds, heat pumps held on to their market share through. And over the past 12 months, heat pumps outsold conventional furnaces by 27 percent. Shipments are expected to continue increasing as states roll out home efficiency and appliance rebate programs already funded by the Inflation Reduction Act – worth up to $10,000 per household in new incentives for heat pump installations. Link: Tracking the Heat Pump & Water Heater Market in the United States – RMI
9. China Reaches Its Renewable Energy Goal, Six Years Early
China added so much renewable energy capacity this year, that by July it had surpassed its goal of having 1,200 gigawatts (GW) of clean energy installed by 2030. Through September 2024, China installed some 161 GW of new solar capacity and 39 GW of new wind power, according to China’s National Energy Administration (NEA). China is deploying more solar, wind, and EVs than any other country, including the United States, which is — by comparison — projected to deploy a record 50 GW of solar modules by the end of 2024.
10. De-carbonizing Heavy Industry
For steel, cement, chemicals and other heavy industries, low-carbon technologies and climate-friendly solutions are not only increasingly available but growing more affordable. To speed this process, Third Derivative, RMI’s climate tech accelerator, launched the Industrial Innovation Cohorts to accelerate the decarbonization of steel, cement, and chemicals. Also on the rise: clean hydrogen hubs — powered by renewable energy — designed to supply green hydrogen to chemical, steel, and other heavy industries to help them shift to low-carbon production processes.
24 notes
·
View notes
Text

Unlocking nickel's potential: New study reveals how to use single atoms to turn CO₂ into valuable chemical resources
Nickel and nitrogen co-doped carbon (Ni-N-C) catalysts have shown exceptional performance in converting CO2 into CO, a valuable chemical feedstock. However, the exact working mechanism of these catalysts has remained elusive—until now. The study "Unveiling the Adsorbate Configurations in Ni Single Atom Catalysts during CO2 Electrocatalytic Reduction using Operando XAS, XES and Machine Learning" provides direct experimental insights into the nature of adsorbates (molecules that stick to the catalyst's surface) forming at the nickel sites and the evolving structure of the active sites during the CO2 reduction reaction (CO2RR). The work is published in the journal Physical Review Letters.
Read more.
13 notes
·
View notes
Text
From Extraction to Usage: The Lifecycle of Natural Gas
The journey of natural gas from the depths of the earth to the blue flames on a stove is a complex and multifaceted process. As a fossil fuel, natural gas plays a pivotal role in the global energy supply, accounting for approximately 24% of global energy consumption. This narrative will traverse through the lifecycle of natural gas, highlighting the intricacies involved in its extraction, processing, transportation, and usage, as well as its environmental and economic impacts.
Extraction and Production:
Natural gas extraction begins with geological surveys to identify promising reserves, followed by drilling either on land or offshore. In 2022, the United States, one of the largest producers, extracted approximately 99.6 billion cubic feet per day. The extraction often employs techniques like hydraulic fracturing, which alone contributed to around 67% of the total U.S. natural gas output in 2018. The extracted gas, containing various hydrocarbons and impurities, requires substantial processing to meet commercial quality standards.
Processing and Purification:
Once extracted, natural gas undergoes several processing steps to remove water vapor, hydrogen sulfide, carbon dioxide, and other contaminants. This purification is essential not only for safety and environmental reasons but also to increase the energy efficiency of the gas. Processing plants across the globe refine thousands of cubic feet of raw gas each day, ensuring that the final product delivered is almost pure methane, which is efficient for burning and less polluting than unprocessed gas.
Transportation:
Transporting natural gas involves an expansive network of pipelines covering over a million miles in the United States alone. In regions where pipeline infrastructure is not feasible, liquified natural gas (LNG) provides an alternative. LNG exports from the U.S. reached record highs in 2022, with approximately 10.6 billion cubic feet per day being shipped to international markets. LNG carriers and storage facilities are integral to this global trade, making natural gas accessible worldwide.
Storage:
Strategic storage ensures that natural gas can meet fluctuating demands, particularly during peak usage periods. Underground storage facilities can hold vast quantities; for instance, the U.S. can store approximately 4 trillion cubic feet of gas, helping to manage supply and stabilize prices. These reserves play a critical role in energy security and in buffering any unexpected disruptions in supply.
Distribution:
Natural gas distribution is the final leg before reaching end-users. Companies manage complex distribution networks to deliver gas to industrial facilities, power plants, commercial establishments, and residences. The U.S. alone has over 2 million miles of distribution pipelines, ensuring that natural gas accounts for nearly 30% of the country’s electricity generation and heating for about half of American homes.
Usage and Consumption:
The versatility of natural gas makes it a preferred source for heating, cooking, electricity generation, and even as a feedstock for producing plastics and chemicals. In the residential sector, an average American home might consume about 200 cubic feet per day for heating and cooking. For electricity, combined-cycle gas turbine plants convert natural gas into electricity with more than 60% efficiency, significantly higher than other fossil-fueled power plants.
Environmental Considerations and Future Prospects:
While natural gas burns cleaner than coal, releasing up to 60% less CO2 for the same energy output, it is not without environmental challenges. Methane, a potent greenhouse gas, can escape during various stages of the natural gas lifecycle. However, advances in technology and regulatory measures aim to mitigate these emissions. As the world leans towards a lower-carbon future, the role of natural gas is pivotal, with investments in carbon capture and storage (CCS) technologies and the potential integration with renewable energy sources.
4 notes
·
View notes
Text
it's been 2 years since the last of these were posted, so I did some digging (on the plastics specifically, since I am currently working on a research project about industrial bio-based plastics).
firstly: the soft/plant elements are indeed now made of bio-based plastic (bio-PE to be specific) from sugar cane.* bio-PE is not biodegradable, though it's a common misconception that all bio-based plastics are biodegradable. (the reason I am saying bio-based is because depending on who you ask, bioplastic sometimes includes biodegradable plastics that are petro-based. this, of course, just makes the misconception more common). in fact, the reason that replacing their previous plastic with bio-PE worked so smoothly is because bio-PE is a drop-in polymer, meaning that it's the same chemical composition as an existing petro-based plastic (in this case, PE aka polyethylene), just from a renewable source. this makes manufacturing them much easier because they can use existing processes. about 2% of LEGO's total production is bio-PE.
secondly: it looks like the current prototypes for hard bricks are recycled PET from plastic water bottles. PET is not as strong as ABS, the current plastic that they're using, so they are apparently working on a process to strengthen it.
thirdly: it really isn't that easy. while drop-in polymers allow for bio-based materials to substitute in for many existing materials, a lack of robust recycling means that they'll still wind up in landfills. on the other hand, biodegradable plastics are typically only rated for industrial composting, which is hard to find, and many places won't actually take biodegradable plastics, leaving them to also go to landfills, where they'll anaerobically break down into methane, a more potent greenhouse gas than CO2. bio-based plastics unfortunately aren't a magic bullet. the best approach is one that combines a lot of approaches: increasing access to recycling, manufacturing more bio-based where possible, using renewable energy for manufacturing, and, notably, cutting down on how much plastic is made, used, and discarded in the first place. this is obviously a huge project, which requires sustained collective action and education rather than nice moves from corporations. though I do like the 100% renewable energy very much!
*the complexities of comparing and contrasting feedstock options is more than I feel like typing up lol

#sorry to be a bit of a bummer but I'm literally doing a project on industrial uses of bio-based plastics RIGHT NOW#and the amount of misinformation that goes around about biodegradable plastics especially is HUGE#also I'm going to assume best intentions but I really have no idea where lynnafred could have gotten that info#just kidding: found an old newsweek article which claims biodegradability and links to a lego post that has no mention of biodegradability#and instead stresses that bioPE is technically identical to petroPE#also additional lego W: only refers to it as bio-based plastic instead of the much less clear bioplastic#slimemoldscholarship#slimemoldscholar's science communication
413K notes
·
View notes
Text
What are Some Alternative Fuel Sources for Construction Machines?
In 2020, the construction industry accounted for approximately 11% of global carbon dioxide emissions, largely due to the energy-intensive processes of producing building materials like steel, cement, and glass.
This share is part of the broader impact of the buildings and construction sector, which is responsible for nearly 39% of global energy-related CO2 emissions.
The emissions include both operational energy (used during the lifecycle of buildings) and embodied energy (from material production and construction activities).
As sustainability goals tighten and regulations become stricter, the demand for low-carbon solutions in heavy machinery has skyrocketed.
Alternative fuels such as hydrogen, biodiesel, and electric power are not only addressing these concerns but are also proving economically viable in the long run.
Some alternative fuel sources for construction vehicles are transforming the industry by reducing dependency on conventional diesel and minimizing environmental impact.
Hydrogen: The future workhorse?
Hydrogen is emerging as a frontrunner for powering construction machinery. Its versatility — used in combustion engines or fuel cells — makes it an attractive option.
Hydrogen fuel cells, for instance, combine hydrogen and oxygen to produce electricity, emitting only water as a byproduct. This makes them perfect for large-scale operations like earthmoving and mining, where emissions reduction and efficiency are paramount.
However, challenges persist. Hydrogen infrastructure, from production to distribution, remains underdeveloped. Additionally, the production of green hydrogen — derived from renewable energy — is not yet cost-competitive with traditional fuels.
Despite these hurdles, ongoing advancements in technology and supportive government incentives are paving the way for hydrogen adoption in construction.
Biodiesel: One of the many renewable fuels
Biodiesel offers a practical, near-term solution for the industry. It’s produced from renewable sources like vegetable oils or animal fats and can be blended with traditional diesel.
Biodiesel is compatible with existing diesel engines, requiring minimal modification, making it a low-barrier entry point for contractors looking to reduce emissions without investing heavily in new machinery.
Its scalability, however, depends on the regional availability of feedstocks and policies that encourage its use over fossil fuels.
In regions with robust agricultural industries, biodiesel has gained traction as a sustainable alternative.
Electrification
Electric-powered machinery is also gaining ground, particularly for smaller construction equipment. Electric motors offer zero emissions, reduced noise levels, and lower maintenance costs due to fewer moving parts.
These benefits make electric equipment ideal for urban construction projects where noise and air quality regulations are stringent.
These a few notable options for alternative fuel for construction machinery: Adopting these alternative fuel sources can significantly contribute to the construction industry's sustainability goals while ensuring efficient and reliable performance of machinery.
0 notes
Text
Methanol Market Analysis: Trends, Growth, and Opportunities Through 2031
The methanol market is witnessing significant growth due to its versatility and increasing adoption across energy, automotive, and chemical industries. This vital chemical compound plays a key role in fostering sustainability, especially with its applications in clean energy and environmental solutions. Below, we explore the market dynamics, segmental insights, regional trends, and emerging opportunities shaping the methanol industry's future.
Market Insights: Methanol as a Catalyst for Sustainability
Methanol’s extensive use in the production of formaldehyde, acetic acid, and fuel additives positions it as an essential feedstock in the chemical and energy industries. With rising concerns about carbon emissions, methanol is also being used as an alternative fuel and in renewable energy storage systems. The market is projected to grow steadily, supported by advancements in production processes, such as the synthesis of methanol from biomass and CO2 recycling.
Request Sample of the Report - https://www.skyquestt.com/sample-request/methanol-market
Segmental Analysis: Diverse Applications Driving Growth
The methanol market is segmented into key applications, feedstocks, and end-user industries:
Applications:
Chemical Manufacturing: Methanol is a key ingredient in producing a variety of chemicals, including formaldehyde and dimethyl ether (DME).
Energy: Its adoption as a clean fuel and in methanol fuel cells is expanding rapidly.
Other Uses: Methanol is used in pharmaceuticals, adhesives, and as an anti-freeze agent.
Feedstocks:
Natural gas dominates methanol production, while coal and renewable sources like biomass are gaining attention for their sustainability benefits.
End-User Industries:
Automotive, energy, and construction industries are among the largest consumers of methanol, reflecting its diverse applications.
Get Customized Reports with your Requirements, Free -https://www.skyquestt.com/speak-with-analyst/methanol-market
Regional Insights: A Global Perspective
Asia-Pacific:
The region leads in methanol production and consumption, driven by rapid industrial growth in China and India.
Investments in coal-to-methanol projects further bolster the region's dominance.
North America:
Shale gas exploitation provides an abundant and cost-effective feedstock, making the U.S. a significant player in methanol production.
Europe:
Stringent environmental regulations drive the adoption of green methanol, particularly in energy and transportation sectors.
Middle East & Africa:
With rich natural gas reserves, this region is emerging as a key hub for methanol production, catering to both local and global markets.
Is this report aligned with your requirements? Interested in making a Purchase -https://www.skyquestt.com/buy-now/methanol-market
Key Market Trends: Innovations and Sustainability
· Green Methanol Production: The push toward sustainability is fostering innovations in producing methanol from renewable feedstocks such as biomass and captured carbon dioxide.
· Expanding Fuel Applications: Methanol is gaining recognition as a marine fuel and as a potential hydrogen carrier in fuel cell technologies.
· Advances in Chemical Synthesis: Methanol-to-olefins (MTO) and methanol-to-gasoline (MTG) technologies are enabling efficient and sustainable chemical production.
Market Dynamics: Drivers, Challenges, and Opportunities
The methanol market is shaped by several factors influencing its growth trajectory:
Market Drivers:
o The rising demand for eco-friendly fuels and chemicals aligns methanol with global sustainability goals.
o Expanding industrialization in emerging economies fuels the demand for methanol in construction and automotive industries.
Challenges:
o Fluctuations in feedstock prices and the development of competing renewable energy sources could impede market growth.
o Regulatory pressures on environmental impacts remain a critical challenge.
Opportunities:
o Technological advancements in producing methanol from renewable sources present significant opportunities for growth.
o Growing interest in methanol as a marine fuel and in hydrogen fuel cells highlights its potential in future energy applications.
Methanol's Role in the Future Economy
The methanol market is on a growth trajectory, supported by its versatile applications and alignment with global sustainability goals. Despite challenges such as feedstock price volatility, the market is poised for expansion due to increasing demand in energy and chemical sectors and innovations in green methanol production. As industries adapt to a greener future, methanol is set to play a critical role in reducing emissions and driving industrial transformation worldwide
1 note
·
View note
Text
Methanol Market: Driving Sustainability Across Energy and Manufacturing Sectors

The global Methanol Market, often referred to as wood alcohol, is a vital component of the global industrial ecosystem. This versatile chemical feedstock finds its application across numerous industries, including petrochemicals, energy, automotive, pharmaceuticals, and construction. As the world moves toward sustainable energy solutions, the methanol market is gaining significant momentum, supported by its dual role in conventional and renewable applications. This comprehensive exploration examines the trends, challenges, and potential growth of the methanol market, with projections spanning 2024 to 2030.
Methanol Market Overview: Future Prospects Unveiled
The global methanol market has exhibited remarkable growth in recent years, reflecting its broad utility across industries. In 2023, the market size was valued at $31.20 billion and is expected to grow to $32.60 billion by 2024. Projections indicate a steady rise, with the market anticipated to reach $42.74 billion by 2030, growing at a compound annual growth rate (CAGR) of 4.60%.
Methanol’s extensive applications in the chemical industry, renewable energy sector, and agriculture, among others, underscore its growing significance. Its adaptability to meet both traditional and innovative needs solidifies its role as a crucial resource in industrial and environmental advancements.
Key Drivers of Methanol Market Growth
Methanol’s growing importance is propelled by several factors, each contributing to its expanding demand and prominence in diverse sectors.
Chemical Industry Demand
Methanol serves as a critical feedstock in the production of essential chemicals such as formaldehyde, acetic acid, and methyl methacrylate (MMA). These derivatives are indispensable in manufacturing automotive components, pharmaceuticals, and construction materials. Emerging economies, with their expanding industrial bases, are significantly boosting the demand for these methanol-based chemicals.
Renewable Energy and Green Fuel Innovations
The global shift toward sustainable energy has positioned methanol as a promising alternative to conventional fuels. Green methanol, derived from renewable sources like biomass, CO2, and municipal waste, is gaining traction for its low carbon footprint. Its adoption in transportation and marine shipping sectors exemplifies its potential to replace fossil fuels with cleaner energy solutions.
Automotive Industry Applications
The automotive sector is exploring methanol as a low-emission alternative to traditional fuels like gasoline and diesel. Methanol is finding applications in fuel cells and hybrid systems, aligning with global initiatives to embrace eco-friendly technologies. Both governments and manufacturers are driving efforts to incorporate methanol into vehicle designs, further accelerating its adoption.
Agriculture Sector Utilization
Methanol plays a pivotal role in the agriculture industry as a key ingredient in the synthesis of fertilizers, herbicides, and pesticides. With global food demand rising due to population growth and shifting consumption patterns, the agricultural sector’s reliance on methanol is set to increase significantly.
Petrochemical Industry Integration
Methanol remains a cornerstone of the petrochemical sector, serving as a raw material for plastics, resins, and solvents. These products are essential for manufacturing industrial goods and consumer items, ensuring methanol’s continued relevance in this rapidly industrializing world.
Segmentation of the Methanol Market
Methanol’s diverse applications have led to its classification into various segments based on production methods and end-use industries.
Production Methods
One of the most efficient techniques for methanol production is the Lurgi Low Pressure method, which converts natural gas into methanol. This process is characterized by reduced production costs and high efficiency, ensuring its sustained importance given the global abundance of natural gas reserves.
Energy Applications
Methanol’s role in energy generation is expanding as it emerges as a cleaner alternative to coal and oil. Its use in renewable energy solutions, including fuel cells, highlights its potential to contribute to the global energy transition.
Green Fuels
The development of renewable methanol from sustainable sources is gaining momentum. This eco-friendly variant addresses carbon emission challenges and aligns with international goals for greener energy solutions.
Construction Industry
Methanol-based derivatives are critical in producing adhesives, paints, and coatings used in construction. The rapid pace of urbanization and infrastructure development, particularly in emerging economies, is driving the demand for these methanol-derived materials.
Automotive and Marine Sectors
In the automotive industry, methanol is gaining attention as both a fuel additive and a direct fuel for internal combustion engines. Similarly, in the marine sector, methanol is being adopted as a low-emission fuel, complying with stricter environmental regulations for maritime transport.
Pharmaceutical Applications
Methanol is integral to synthesizing important pharmaceutical compounds, including antibiotics and pain relievers. Its role in drug manufacturing highlights its versatility and critical contribution beyond industrial uses.
Major Players Driving the Methanol Market
The methanol market is highly competitive, with prominent companies shaping its trajectory through innovation and strategic investments. Leading players such as Methanax, SABIC, CNPC, Petronas, and LyondellBasell are actively investing in research and development to improve production efficiency and explore new applications for methanol. Their focus on renewable methanol and green technologies underscores their commitment to sustainability and market leadership.
Regional Dynamics of the Methanol Market
The methanol market’s growth varies across regions, influenced by factors such as industrial activity, regulatory policies, and resource availability.
North America: The United States leads methanol production and consumption in North America, driven by advancements in renewable methanol applications.
Europe: Stringent environmental regulations in Europe are fostering the adoption of methanol as a green energy source. Germany and the Netherlands are prominent contributors to this trend.
Asia-Pacific: With China at the forefront, the Asia-Pacific region dominates methanol consumption, propelled by rapid industrialization and a focus on renewable energy.
Latin America: Brazil and Mexico are experiencing increased methanol usage in petrochemical and energy applications, alongside growing investments in renewable technologies.
Middle East and Africa: Rich natural gas reserves make the Middle East a key player in methanol production, while its use in energy and petrochemical industries continues to expand.
Challenges Facing the Methanol Industry
Despite its growth, the methanol market faces challenges that could impact its trajectory:
Raw Material Price Volatility: Methanol production depends heavily on natural gas, and fluctuations in its prices can affect market stability.
Environmental Concerns: Conventional methanol production relies on fossil fuels, raising sustainability concerns. While greener methods are emerging, they require substantial investment and time for widespread adoption.
Regulatory Pressure: Stricter global environmental regulations are reshaping market dynamics, increasing operational costs but driving innovation in sustainable practices.
Conclusion: Methanol’s Bright Future
Methanol’s versatility and adaptability make it a cornerstone of industrial and environmental progress. With a projected market size of $42.74 billion by 2030, its critical role in diverse applications, from green energy to agriculture, underscores its growing importance.
As industry leaders continue to innovate and invest in sustainable solutions, methanol is poised to play a transformative role in addressing global energy and environmental challenges. From powering vehicles and ships to supporting construction and pharmaceuticals, methanol stands at the forefront of a rapidly changing world, driving both industrial growth and sustainability.
Browse More:
Injection Molded Plastics Market Analysis
Electric Bus Charging Infrastructure Market Trends
Chiller Unit Market 2024
Rubber Antioxidant Market Overview
They want to buy everyone… : BJP leader Dilip Ghosh alleges Trinamool trying to silence family of RGK victim
0 notes
Text
Fossil fuels are destroying our planet. Big oil is evil. Coal is an addiction. These are hyperbolic statements uttered without basis in the public square as we continue with lifestyles dependent on hydrocarbons and their derivatives.
This dissonance exists cognitively in individuals whose choices are inconsistent with their thinking. Another dissonance manifests itself as elites who are wedded to an apocalyptic climate vision fearmongering to people who are just fine with their “high-carbon lives.”
Whatever one thinks about hydrocarbons, nobody can deny the sheer ubiquity of the things produced through their use as an energy source or feedstock.
From the plastic in smartphones to the synthetic fibers in clothing, from life-saving medical equipment to the asphalt on roads, petroleum-based products are woven into the fabric of modern life. Even the components of many “green” technologies, such as solar panels and wind turbines, are made with materials derived from fossil fuels.
In a document stating that petrochemicals are the basis for more than 6,000 everyday products, the U.S. Department of Energy lists a fraction of them to make the point of their prevalence.
The success of the climate industrial complex in demonizing coal, oil and natural gas despite their enormous benefits speaks to the broadness of the alarmists’ network: career politicians, unelected international political organizations, media houses, government-funded scientists in academia and corporate CEOs addicted to subsidies. All this is fed by an education system that puts ideological indoctrination ahead of inculcation of independent thought to beget complaisant minds.
Yet, this schizophrenic relationship to hydrocarbons may not run as deeply as it sometimes seems. Despite the crescendo calling for a rapid transition to solar and wind, global consumption of fossil fuels continues to climb. That is because the alternatives are neither affordable nor dependable.
In 2023, the world set new records for hydrocarbon consumption. This trend is not limited to developing nations struggling to industrialize like China and India; it’s equally evident in some of the world’s most advanced economies.
The U.S. was the world’s largest oil producer in 2023, with an average of 12.9 million barrels per day (b/d), breaking the previous U.S. global record of 12.3 million b/d set in 2019. Norway, often hailed as a leader in environmental policy, issued hundreds of new drilling permits for oil and gas exploration in 2024.
Dependence on fossil fuels is not waning; it’s intensifying at a rate never seen before.
Aramco CEO Amin Nasser says, “Global South is likely to see significant growth in oil demand for a long time…. If so, more than 100 million barrels per day would realistically still be required by 2050… This is a stark contrast with those predicting that oil will, or must, fall to just 25 million barrels per day by then…. Being short 75 million barrels every day would be devastating for energy security and affordability.”
Meanwhile, the preachers of the anti-fossil fuel gospel live hypocritically, enjoying fossil fuel-guzzling private jets and lavish sea-front mansions. That their affluence rests on an economic bedrock of fossil fuels is not lost on impoverished countries seeking betterment through the use of hydrocarbons as they are pressured to adopt “green” policies.
Even if the findings from the Intergovernmental Panel on Climate Change (IPCC) assessment reports are accepted, the predictions of harm from global warming are overblown. According to the IPCC, the cost of a warmer planet would be in the range of 2-4% of the gross domestic product (GDP) by 2100 – a tiny amount considering that the average person is expected to be much richer by then and the cost of “green” policies are likely to impoverish entire nations.
Every major economy thriving today was built on fossil fuels. Their contributions in the areas of energy, transportation, construction, agriculture, manufacturing, healthcare and more have produced unprecedented levels of prosperity, health and life expectancy.
Moreover, the increase in atmospheric CO2 from the combustion of hydrocarbons has been a boon, greening vast areas of Earth and producing record crop harvests to feed billions of people.
It’s past time to abandon the climate myth and embrace fossil fuels for the gifts that they are.
This commentary was first published at BizPac Review on October 28, 2024.
Vijay Jayaraj is a Science and Research Associate at the CO2 Coalition, Arlington, Virginia. He holds an M.S. in environmental sciences from the University of East Anglia and a postgraduate degree in energy management from Robert Gordon University, both in the U.K., and a bachelor’s in engineering from Anna University, India.
0 notes
Text
Researchers report that replacing 50% of animal products with alternative proteins by 2050 could free up enough agricultural land to generate renewable energy equivalent in volume to today's coal-generated power while simultaneously removing substantial CO2 from the atmosphere. The study, published in the journal One Earth, is based on a CO2-removal method known as "bioenergy with carbon capture and storage" (BECCS), which involves cultivating quickly growing crops whose biomass can then be stored permanently in geological formations or used as a feedstock to produce renewable energy.
Continue Reading.
#Science#Enviroment#Earth Science#Animal Husbandry#Carbon Emissions#Carbon Capture#Climate Change#Diet
105 notes
·
View notes
Text
Excerpt from this story from Canary Media:
Airlines are banking on sustainable aviation fuel to reduce the industry’s planet-warming pollution. But the amount of lower-carbon alternatives available to them right now represents just a few drops in an ocean of petroleum.
On Wednesday, the U.S. Department of Energy announced a nearly $3 billion effort that it said could significantly boost America’s output of sustainable aviation fuel, or SAF, over the next few years, Canary Media has exclusively learned.
The agency’s Loan Programs Office has made conditional commitments to two companies in the Great Plains region that are working to turn crops and waste feedstocks into jet fuel.
Montana Renewables, a subsidiary of the industrial manufacturer Calumet, could receive a loan guarantee of up to $1.44 billion to expand its existing renewable fuels facility in Great Falls, Montana. The company makes biofuels for planes and trucks using vegetable oils and leftover animal fats and greases. The expansion would allow Montana Renewables to produce about 315 million gallons per year of biofuels, most of which will be SAF — equal to nearly eight times the country’s total SAF production capacity in 2023.
Colorado-based Gevo is vying for a loan guarantee of $1.46 billion to build a new jet-fuel refinery in Lake Preston, South Dakota. The facility, named Net-Zero 1, would turn corn into ethanol to produce up to 60 million gallons of SAF per year. Because the ethanol-making process creates carbon dioxide emissions, Gevo is planning to capture CO2 at the refinery and send it via the proposed — and highly contentious — Summit Carbon Solutions pipeline to a storage site in North Dakota.
Patrick Gruber, CEO of Gevo, said the announcement “marks a watershed moment for the Net-Zero 1 project and a critical step forward” in the company’s mission to produce low-carbon jet fuel.
The projects are the first SAF-related ventures to win the backing of the Loan Programs Office, which has issued $42.4 billion in loans and loan guarantees and made $21.6 billion in conditional commitments as of June 2024. The office is supporting other clean energy initiatives such as battery manufacturing, virtual power plants, fuel cell production, and the repowering of old nuclear plants.
The two fuel producers will have to meet certain milestones before they can close on the federal loan guarantees and start putting the financing to work.
6 notes
·
View notes
Text
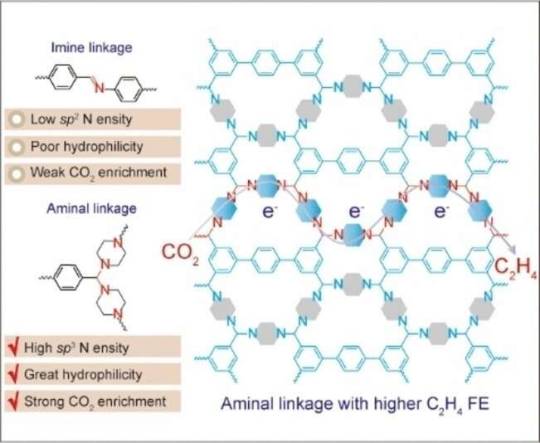
A metal-free organic framework for electrocatalytic production of ethylene from carbon dioxide
Use of the greenhouse gas CO2 as a chemical raw material would not only reduce emissions, but also the consumption of fossil feedstocks. A novel metal-free organic framework could make it possible to electrocatalytically produce ethylene, a primary chemical raw material, from CO2. As a team has reported in the journal Angewandte Chemie International Edition, nitrogen atoms with a particular electron configuration play a critical role for the catalyst. Ethylene (ethene, C2H4) is an essential starting material for many products, including polyethylene and other plastics. Ethylene is produced industrially by the high-energy cracking and rectification of fossil feedstocks.
Read more.
#Materials Science#Science#Organic materials#Carbon dioxide#Electrocatalysts#Covalent organic frameworks#Nitrogen
22 notes
·
View notes
Text
MIT engineers make converting CO2 into useful products more practical
New Post has been published on https://sunalei.org/news/mit-engineers-make-converting-co2-into-useful-products-more-practical/
MIT engineers make converting CO2 into useful products more practical

As the world struggles to reduce greenhouse gas emissions, researchers are seeking practical, economical ways to capture carbon dioxide and convert it into useful products, such as transportation fuels, chemical feedstocks, or even building materials. But so far, such attempts have struggled to reach economic viability.
New research by engineers at MIT could lead to rapid improvements in a variety of electrochemical systems that are under development to convert carbon dioxide into a valuable commodity. The team developed a new design for the electrodes used in these systems, which increases the efficiency of the conversion process.
The findings are reported today in the journal Nature Communications, in a paper by MIT doctoral student Simon Rufer, professor of mechanical engineering Kripa Varanasi, and three others.
“The CO2 problem is a big challenge for our times, and we are using all kinds of levers to solve and address this problem,” Varanasi says. It will be essential to find practical ways of removing the gas, he says, either from sources such as power plant emissions, or straight out of the air or the oceans. But then, once the CO2 has been removed, it has to go somewhere.
A wide variety of systems have been developed for converting that captured gas into a useful chemical product, Varanasi says. “It’s not that we can’t do it — we can do it. But the question is how can we make this efficient? How can we make this cost-effective?”
In the new study, the team focused on the electrochemical conversion of CO2 to ethylene, a widely used chemical that can be made into a variety of plastics as well as fuels, and which today is made from petroleum. But the approach they developed could also be applied to producing other high-value chemical products as well, including methane, methanol, carbon monoxide, and others, the researchers say.
Currently, ethylene sells for about $1,000 per ton, so the goal is to be able to meet or beat that price. The electrochemical process that converts CO2 into ethylene involves a water-based solution and a catalyst material, which come into contact along with an electric current in a device called a gas diffusion electrode.
There are two competing characteristics of the gas diffusion electrode materials that affect their performance: They must be good electrical conductors so that the current that drives the process doesn’t get wasted through resistance heating, but they must also be “hydrophobic,” or water repelling, so the water-based electrolyte solution doesn’t leak through and interfere with the reactions taking place at the electrode surface.
Unfortunately, it’s a tradeoff. Improving the conductivity reduces the hydrophobicity, and vice versa. Varanasi and his team set out to see if they could find a way around that conflict, and after many months of trying, they did just that.
The solution, devised by Rufer and Varanasi, is elegant in its simplicity. They used a plastic material, PTFE (essentially Teflon), that has been known to have good hydrophobic properties. However, PTFE’s lack of conductivity means that electrons must travel through a very thin catalyst layer, leading to significant voltage drop with distance. To overcome this limitation, the researchers wove a series of conductive copper wires through the very thin sheet of the PTFE.
“This work really addressed this challenge, as we can now get both conductivity and hydrophobicity,” Varanasi says.
Research on potential carbon conversion systems tends to be done on very small, lab-scale samples, typically less than 1-inch (2.5-centimeter) squares. To demonstrate the potential for scaling up, Varanasi’s team produced a sheet 10 times larger in area and demonstrated its effective performance.
To get to that point, they had to do some basic tests that had apparently never been done before, running tests under identical conditions but using electrodes of different sizes to analyze the relationship between conductivity and electrode size. They found that conductivity dropped off dramatically with size, which would mean much more energy, and thus cost, would be needed to drive the reaction.
“That’s exactly what we would expect, but it was something that nobody had really dedicatedly investigated before,” Rufer says. In addition, the larger sizes produced more unwanted chemical byproducts besides the intended ethylene.
Real-world industrial applications would require electrodes that are perhaps 100 times larger than the lab versions, so adding the conductive wires will be necessary for making such systems practical, the researchers say. They also developed a model which captures the spatial variability in voltage and product distribution on electrodes due to ohmic losses. The model along with the experimental data they collected enabled them to calculate the optimal spacing for conductive wires to counteract the drop off in conductivity.
In effect, by weaving the wire through the material, the material is divided into smaller subsections determined by the spacing of the wires. “We split it into a bunch of little subsegments, each of which is effectively a smaller electrode,” Rufer says. “And as we’ve seen, small electrodes can work really well.”
Because the copper wire is so much more conductive than the PTFE material, it acts as a kind of superhighway for electrons passing through, bridging the areas where they are confined to the substrate and face greater resistance.
To demonstrate that their system is robust, the researchers ran a test electrode for 75 hours continuously, with little change in performance. Overall, Rufer says, their system “is the first PTFE-based electrode which has gone beyond the lab scale on the order of 5 centimeters or smaller. It’s the first work that has progressed into a much larger scale and has done so without sacrificing efficiency.”
The weaving process for incorporating the wire can be easily integrated into existing manufacturing processes, even in a large-scale roll-to-roll process, he adds.
“Our approach is very powerful because it doesn’t have anything to do with the actual catalyst being used,” Rufer says. “You can sew this micrometric copper wire into any gas diffusion electrode you want, independent of catalyst morphology or chemistry. So, this approach can be used to scale anybody’s electrode.”
“Given that we will need to process gigatons of CO2 annually to combat the CO2 challenge, we really need to think about solutions that can scale,” Varanasi says. “Starting with this mindset enables us to identify critical bottlenecks and develop innovative approaches that can make a meaningful impact in solving the problem. Our hierarchically conductive electrode is a result of such thinking.”
The research team included MIT graduate students Michael Nitzsche and Sanjay Garimella, as well as Jack Lake PhD ’23. The work was supported by Shell, through the MIT Energy Initiative.
0 notes
Text
MIT engineers make converting CO2 into useful products more practical
New Post has been published on https://thedigitalinsider.com/mit-engineers-make-converting-co2-into-useful-products-more-practical/
MIT engineers make converting CO2 into useful products more practical


As the world struggles to reduce greenhouse gas emissions, researchers are seeking practical, economical ways to capture carbon dioxide and convert it into useful products, such as transportation fuels, chemical feedstocks, or even building materials. But so far, such attempts have struggled to reach economic viability.
New research by engineers at MIT could lead to rapid improvements in a variety of electrochemical systems that are under development to convert carbon dioxide into a valuable commodity. The team developed a new design for the electrodes used in these systems, which increases the efficiency of the conversion process.
The findings are reported today in the journal Nature Communications, in a paper by MIT doctoral student Simon Rufer, professor of mechanical engineering Kripa Varanasi, and three others.
“The CO2 problem is a big challenge for our times, and we are using all kinds of levers to solve and address this problem,” Varanasi says. It will be essential to find practical ways of removing the gas, he says, either from sources such as power plant emissions, or straight out of the air or the oceans. But then, once the CO2 has been removed, it has to go somewhere.
A wide variety of systems have been developed for converting that captured gas into a useful chemical product, Varanasi says. “It’s not that we can’t do it — we can do it. But the question is how can we make this efficient? How can we make this cost-effective?”
In the new study, the team focused on the electrochemical conversion of CO2 to ethylene, a widely used chemical that can be made into a variety of plastics as well as fuels, and which today is made from petroleum. But the approach they developed could also be applied to producing other high-value chemical products as well, including methane, methanol, carbon monoxide, and others, the researchers say.
Currently, ethylene sells for about $1,000 per ton, so the goal is to be able to meet or beat that price. The electrochemical process that converts CO2 into ethylene involves a water-based solution and a catalyst material, which come into contact along with an electric current in a device called a gas diffusion electrode.
There are two competing characteristics of the gas diffusion electrode materials that affect their performance: They must be good electrical conductors so that the current that drives the process doesn’t get wasted through resistance heating, but they must also be “hydrophobic,” or water repelling, so the water-based electrolyte solution doesn’t leak through and interfere with the reactions taking place at the electrode surface.
Unfortunately, it’s a tradeoff. Improving the conductivity reduces the hydrophobicity, and vice versa. Varanasi and his team set out to see if they could find a way around that conflict, and after many months of trying, they did just that.
The solution, devised by Rufer and Varanasi, is elegant in its simplicity. They used a plastic material, PTFE (essentially Teflon), that has been known to have good hydrophobic properties. However, PTFE’s lack of conductivity means that electrons must travel through a very thin catalyst layer, leading to significant voltage drop with distance. To overcome this limitation, the researchers wove a series of conductive copper wires through the very thin sheet of the PTFE.
“This work really addressed this challenge, as we can now get both conductivity and hydrophobicity,” Varanasi says.
Research on potential carbon conversion systems tends to be done on very small, lab-scale samples, typically less than 1-inch (2.5-centimeter) squares. To demonstrate the potential for scaling up, Varanasi’s team produced a sheet 10 times larger in area and demonstrated its effective performance.
To get to that point, they had to do some basic tests that had apparently never been done before, running tests under identical conditions but using electrodes of different sizes to analyze the relationship between conductivity and electrode size. They found that conductivity dropped off dramatically with size, which would mean much more energy, and thus cost, would be needed to drive the reaction.
“That’s exactly what we would expect, but it was something that nobody had really dedicatedly investigated before,” Rufer says. In addition, the larger sizes produced more unwanted chemical byproducts besides the intended ethylene.
Real-world industrial applications would require electrodes that are perhaps 100 times larger than the lab versions, so adding the conductive wires will be necessary for making such systems practical, the researchers say. They also developed a model which captures the spatial variability in voltage and product distribution on electrodes due to ohmic losses. The model along with the experimental data they collected enabled them to calculate the optimal spacing for conductive wires to counteract the drop off in conductivity.
In effect, by weaving the wire through the material, the material is divided into smaller subsections determined by the spacing of the wires. “We split it into a bunch of little subsegments, each of which is effectively a smaller electrode,” Rufer says. “And as we’ve seen, small electrodes can work really well.”
Because the copper wire is so much more conductive than the PTFE material, it acts as a kind of superhighway for electrons passing through, bridging the areas where they are confined to the substrate and face greater resistance.
To demonstrate that their system is robust, the researchers ran a test electrode for 75 hours continuously, with little change in performance. Overall, Rufer says, their system “is the first PTFE-based electrode which has gone beyond the lab scale on the order of 5 centimeters or smaller. It’s the first work that has progressed into a much larger scale and has done so without sacrificing efficiency.”
The weaving process for incorporating the wire can be easily integrated into existing manufacturing processes, even in a large-scale roll-to-roll process, he adds.
“Our approach is very powerful because it doesn’t have anything to do with the actual catalyst being used,” Rufer says. “You can sew this micrometric copper wire into any gas diffusion electrode you want, independent of catalyst morphology or chemistry. So, this approach can be used to scale anybody’s electrode.”
“Given that we will need to process gigatons of CO2 annually to combat the CO2 challenge, we really need to think about solutions that can scale,” Varanasi says. “Starting with this mindset enables us to identify critical bottlenecks and develop innovative approaches that can make a meaningful impact in solving the problem. Our hierarchically conductive electrode is a result of such thinking.”
The research team included MIT graduate students Michael Nitzsche and Sanjay Garimella, as well as Jack Lake PhD ’23. The work was supported by Shell, through the MIT Energy Initiative.
#000#air#applications#approach#Building#Capture#carbon#Carbon dioxide#catalyst#challenge#change#chemical#chemistry#climate#CO2#communications#conductors#Conflict#data#Design#development#diffusion#economic#efficiency#electrochemical#electrode#electrodes#electrolyte#electrons#Emissions
0 notes