#pyruvate oxidation
Explore tagged Tumblr posts
Text
All right @respectthepetty, what's the love story happening here?

#(no pressure to answer of course. I just immediately thought of you when I read it)#color coded [metabolic processes] in love#conversations with respectthepetty#gillianthecat goes back to school#hw lb#from my textbook#cellular respiration#glycolysis#pyruvate oxidation#citric acid cycle#oxidative phosphorylation#color symbolism#color coded boys in love
24 notes
·
View notes
Text
Cellular Respiration ₊˚⊹♡
Cellular Respiration - a series of chemical reactions that convert the chemical energy in fuel molecules into the chemical energy of adenosine triphosphate (aka ATP)
converts chemical potential energy in organic molecules to chemical potential energy in ATP
Chemical Reaction: C6H12O6 + 6O2 --> 6CO2 + 6H2O + energy!!
Why does cellular respiration produce a large amount of energy?
The sum of the potential energy in the chemical bonds of the reactants (glucose and oxygen) is higher than that of the products (carbon dioxide and water) Because of this, a lot of energy is released
Note: energy is not released all at once, but in a series of chemical reactions!!
How is ATP produced?
ATP energy is produced in 2 ways!
substrate-level phosphorylation - an organic molecule transfers a phosphate group directly to ADP (adenosine diphosphate) to make ATP
oxidative phosphorylation - the chemical energy of organic molecules is transferred first to electron carriers which transport electrons released during the catabolism of organic molecules to the electron transport chain. through this process, they harness the energy used to make ATP.
(there are more notes, but i'm currently a lil too lazy to write them!!)
brief summary of things I still need to learn:
NAD and FAD
4 stages of cellular respiration
1 - glycolysis
2 - pyruvate is oxidized to another molecule called acetyl coenzyme, or acetyl-CoA
3 - citric acid cycle
4- oxidative phosphorylation
12 notes
·
View notes
Text
hi guys ive realized i never talk here Did you know that in glycolysis, a glucose molecule is split into 2 3-carbon intermediates that then get oxidized to 2 pyruvates . awesome right
#.txt#is that right god i hope it's right . i love bio ok but cellular respiration is fucking hard like tf#i am not having a good time i have so much work to do and ive fallen behind in my english class . say lah vee or whatever
7 notes
·
View notes
Note
Yeets myself into your askbox
HUUUUUUUUUUUUUUUUUGSSSSSSSSS!!
- @emotionally-small-dragon
Did you know that mitochondria is the powerhouse of the cell?
Mitochondria are one of the defining characteristics of eukaryotic cells and it is absolutely the powerhouse in the sense it’s where organic macromolecules such as pyruvate, fatty acids, and amino acids are oxidized to generate chemical energy the cell can utilize to perform work.
What he may be alluding to is the fact prokaryotes use simplified biochemical oxidation reduction reactions to generare cellular energy and they do not require mitochondria.
The powerhouse analogy is very apt as power plants generate power by oxidizing carbon containing molecules and using the heat generated to perform work such as creating pressure gradients that can be used to generate electrical gradients. Mitochondria oxidize carbón containing molecules to create an electrochemical gradient known as the proton motor force which atp synthase used to generate atp, the cellular energy molecule.
3 notes
·
View notes
Note
sup
hi anon
thank you for asking
i am procrastinating on here hshsahhahahahahahah
i am haunted.
HAUNTED I TELL YOU. there's so much to do. what the fuck. why is there. so much to do.
WHAT IS PYRUVATE OXIDATION? WHAT IS THE DIFFERENCE BETWEEN SUBSTRATE-LEVEL PHOSPHORYLATION AND OXIDATIVE PHOSPHORYLATION.
WHY IS THINGS FALL APART CONSIDERED GOOD LITERATURE.
WHY IS MY ENGINEERING TEACHER REAL
I'M ABOUT TO END UP ON THE FUCKING NEWS
nah but otherwise i'm chill
sup with you?
2 notes
·
View notes
Text

roxy's adventures! today she got to learn about pyruvate oxidation and the citric acid cycle 🥰
#roxys adventures#puddle talks#im going to make this a series. she goes lots of places#one time she went to someone elses class amd got to learn about trebuchets#her name is roxanne but we call her roxy#beanie baby#ty beanie babies#biology
6 notes
·
View notes
Text
SMART researchers develop a method to enhance effectiveness of cartilage repair therapy
New Post has been published on https://sunalei.org/news/smart-researchers-develop-a-method-to-enhance-effectiveness-of-cartilage-repair-therapy/
SMART researchers develop a method to enhance effectiveness of cartilage repair therapy
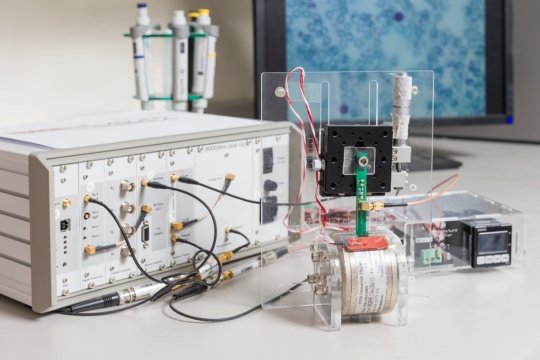
Researchers from the Critical Analytics for Manufacturing Personalized-Medicine (CAMP) interdisciplinary research group at the Singapore-MIT Alliance for Research and Technology (SMART), MIT’s research enterprise in Singapore, alongside collaborators from the National University of Singapore Tissue Engineering Programme, have developed a novel method to enhance the ability of mesenchymal stromal cells (MSCs) to generate cartilage tissue by adding ascorbic acid during MSC expansion. The research also discovered that micro-magnetic resonance relaxometry (µMRR), a novel process analytical tool developed by SMART CAMP, can be used as a rapid, label-free process-monitoring tool for the quality expansion of MSCs.
Articular cartilage, a connective tissue that protects the bone ends in joints, can degenerate due to injury, age, or arthritis, leading to significant joint pain and disability. Especially in countries — such as Singapore — that have an active, aging population, articular cartilage degeneration is a growing ailment that affects an increasing number of people. Autologous chondrocyte implantation is currently the only Food and Drug Administration-approved cell-based therapy for articular cartilage injuries, but it is costly, time-intensive, and requires multiple treatments. MSCs are an attractive and promising alternative as they have shown good safety profiles for transplantation. However, clinical use of MSCs is limited due to inconsistent treatment outcomes arising from factors such as donor-to-donor variability, variation among cells during cell expansion, and non-standardized MSC manufacturing protocols.
The heterogeneity of MSCs can lead to variations in their biological behavior and treatment outcomes. While large-scale MSC expansions are required to obtain a therapeutically relevant number of cells for implantation, this process can introduce cell heterogeneity. Therefore, improved processes are essential to reduce cell heterogeneity while increasing donor cell numbers with improved chondrogenic potential — the ability of MSCs to differentiate into cartilage cells to repair cartilage tissue — to pave the way for more effective and consistent MSC-based therapies.
In a paper titled “Metabolic modulation to improve MSC expansion and therapeutic potential for articular cartilage repair,” published in the scientific journal Stem Cell Research and Therapy, CAMP researchers detailed their development of a priming strategy to enhance the expansion of quality MSCs by modifying the way cells utilize energy. The research findings have shown a positive correlation between chondrogenic potential and oxidative phosphorylation (OXPHOS), a process that harnesses the reduction of oxygen to create adenosine triphosphate — a source of energy that drives and supports many processes in living cells. This suggests that manipulating MSC metabolism is a promising strategy for enhancing chondrogenic potential.
Using novel PATs developed by CAMP, the researchers explored the potential of metabolic modulation in both short- and long-term harvesting and reseeding of cells. To enhance their chondrogenic potential, they varied the nutrient composition, including glucose, pyruvate, glutamine, and ascorbic acid (AA). As AA is reported to support OXPHOS and its positive impact on chondrogenic potential during differentiation — a process in which immature cells become mature cells with specific functions — the researchers further investigated its effects during MSC expansion.
The addition of AA to cell cultures for one passage during MSC expansion and prior to initiation of differentiation was found to improve chondrogenic differentiation, which is a critical quality attribute (CQA) for better articular cartilage repair. Longer-term AA treatment led to a more than 300-fold increase in the yield of MSCs with enhanced chondrogenic potential, and reduced cell heterogeneity and cell senescence — a process by which a cell ages and permanently stops dividing but does not die — when compared to untreated cells. AA-treated MSCs with improved chondrogenic potential showed a robust shift in metabolic profile to OXPHOS. This metabolic change correlated with μMRR measurements, which helps identify novel CQAs that could be implemented in MSC manufacturing for articular cartilage repair.
The research also demonstrates the potential of the process analytical tool developed by CAMP, micromagnetic resonance relaxometry (μMRR) — a miniature benchtop device that employs magnetic resonance imaging (MRI) imaging on a microscopic scale — as a process-monitoring tool for the expansion of MSCs with AA supplementation. Originally used as a label-free malaria diagnosis method due to the presence of paramagnetic hemozoin particles, μMRR was used in the research to detect senescence in MSCs. This rapid, label-free method requires only a small number of cells for evaluation, which allows for MSC therapy manufacturing in closed systems — a system for protecting pharmaceutical products by reducing contamination risks from the external environment — while enabling intermittent monitoring of a limited lot size per production.
“Donor-to-donor variation, intrapopulation heterogeneity, and cellular senescence have impeded the success of MSCs as a standard of care therapy for articular cartilage repair. Our research showed that AA supplementation during MSC expansion can overcome these bottlenecks and enhance MSC chondrogenic potential,” says Ching Ann Tee, senior postdoc at SMART CAMP and first author of the paper. “By controlling metabolic conditions such as AA supplementation, coupled with CAMP’s process analytical tools such as µMRR, the yield and quality of cell therapy products could be significantly increased. This breakthrough could help make MSC therapy a more effective and viable treatment option and provide standards for improving the manufacturing pipeline.”
“This approach of utilizing metabolic modulation to improve MSC chondrogenic potential could be adapted into similar concepts for other therapeutic indications, such as osteogenic potential for bone repair or other types of stem cells. Implementing our findings in MSC manufacturing settings could be a significant step forward for patients with osteoarthritis and other joint diseases, as we can efficiently produce large quantities of high-quality MSCs with consistent functionality and enable the treatment of more patients,” adds Professor Laurie A. Boyer, principal investigator at SMART CAMP, professor of biology and biological engineering at MIT, and corresponding author of the paper.
The research is conducted by SMART and supported by the National Research Foundation Singapore under its Campus for Research Excellence and Technological Enterprise program.
0 notes
Text
SMART researchers develop a method to enhance effectiveness of cartilage repair therapy
New Post has been published on https://thedigitalinsider.com/smart-researchers-develop-a-method-to-enhance-effectiveness-of-cartilage-repair-therapy/
SMART researchers develop a method to enhance effectiveness of cartilage repair therapy


Researchers from the Critical Analytics for Manufacturing Personalized-Medicine (CAMP) interdisciplinary research group at the Singapore-MIT Alliance for Research and Technology (SMART), MIT’s research enterprise in Singapore, alongside collaborators from the National University of Singapore Tissue Engineering Programme, have developed a novel method to enhance the ability of mesenchymal stromal cells (MSCs) to generate cartilage tissue by adding ascorbic acid during MSC expansion. The research also discovered that micro-magnetic resonance relaxometry (µMRR), a novel process analytical tool developed by SMART CAMP, can be used as a rapid, label-free process-monitoring tool for the quality expansion of MSCs.
Articular cartilage, a connective tissue that protects the bone ends in joints, can degenerate due to injury, age, or arthritis, leading to significant joint pain and disability. Especially in countries — such as Singapore — that have an active, aging population, articular cartilage degeneration is a growing ailment that affects an increasing number of people. Autologous chondrocyte implantation is currently the only Food and Drug Administration-approved cell-based therapy for articular cartilage injuries, but it is costly, time-intensive, and requires multiple treatments. MSCs are an attractive and promising alternative as they have shown good safety profiles for transplantation. However, clinical use of MSCs is limited due to inconsistent treatment outcomes arising from factors such as donor-to-donor variability, variation among cells during cell expansion, and non-standardized MSC manufacturing protocols.
The heterogeneity of MSCs can lead to variations in their biological behavior and treatment outcomes. While large-scale MSC expansions are required to obtain a therapeutically relevant number of cells for implantation, this process can introduce cell heterogeneity. Therefore, improved processes are essential to reduce cell heterogeneity while increasing donor cell numbers with improved chondrogenic potential — the ability of MSCs to differentiate into cartilage cells to repair cartilage tissue — to pave the way for more effective and consistent MSC-based therapies.
In a paper titled “Metabolic modulation to improve MSC expansion and therapeutic potential for articular cartilage repair,” published in the scientific journal Stem Cell Research and Therapy, CAMP researchers detailed their development of a priming strategy to enhance the expansion of quality MSCs by modifying the way cells utilize energy. The research findings have shown a positive correlation between chondrogenic potential and oxidative phosphorylation (OXPHOS), a process that harnesses the reduction of oxygen to create adenosine triphosphate — a source of energy that drives and supports many processes in living cells. This suggests that manipulating MSC metabolism is a promising strategy for enhancing chondrogenic potential.
Using novel PATs developed by CAMP, the researchers explored the potential of metabolic modulation in both short- and long-term harvesting and reseeding of cells. To enhance their chondrogenic potential, they varied the nutrient composition, including glucose, pyruvate, glutamine, and ascorbic acid (AA). As AA is reported to support OXPHOS and its positive impact on chondrogenic potential during differentiation — a process in which immature cells become mature cells with specific functions — the researchers further investigated its effects during MSC expansion.
The addition of AA to cell cultures for one passage during MSC expansion and prior to initiation of differentiation was found to improve chondrogenic differentiation, which is a critical quality attribute (CQA) for better articular cartilage repair. Longer-term AA treatment led to a more than 300-fold increase in the yield of MSCs with enhanced chondrogenic potential, and reduced cell heterogeneity and cell senescence — a process by which a cell ages and permanently stops dividing but does not die — when compared to untreated cells. AA-treated MSCs with improved chondrogenic potential showed a robust shift in metabolic profile to OXPHOS. This metabolic change correlated with μMRR measurements, which helps identify novel CQAs that could be implemented in MSC manufacturing for articular cartilage repair.
The research also demonstrates the potential of the process analytical tool developed by CAMP, micromagnetic resonance relaxometry (μMRR) — a miniature benchtop device that employs magnetic resonance imaging (MRI) imaging on a microscopic scale — as a process-monitoring tool for the expansion of MSCs with AA supplementation. Originally used as a label-free malaria diagnosis method due to the presence of paramagnetic hemozoin particles, μMRR was used in the research to detect senescence in MSCs. This rapid, label-free method requires only a small number of cells for evaluation, which allows for MSC therapy manufacturing in closed systems — a system for protecting pharmaceutical products by reducing contamination risks from the external environment — while enabling intermittent monitoring of a limited lot size per production.
“Donor-to-donor variation, intrapopulation heterogeneity, and cellular senescence have impeded the success of MSCs as a standard of care therapy for articular cartilage repair. Our research showed that AA supplementation during MSC expansion can overcome these bottlenecks and enhance MSC chondrogenic potential,” says Ching Ann Tee, senior postdoc at SMART CAMP and first author of the paper. “By controlling metabolic conditions such as AA supplementation, coupled with CAMP’s process analytical tools such as µMRR, the yield and quality of cell therapy products could be significantly increased. This breakthrough could help make MSC therapy a more effective and viable treatment option and provide standards for improving the manufacturing pipeline.”
“This approach of utilizing metabolic modulation to improve MSC chondrogenic potential could be adapted into similar concepts for other therapeutic indications, such as osteogenic potential for bone repair or other types of stem cells. Implementing our findings in MSC manufacturing settings could be a significant step forward for patients with osteoarthritis and other joint diseases, as we can efficiently produce large quantities of high-quality MSCs with consistent functionality and enable the treatment of more patients,” adds Professor Laurie A. Boyer, principal investigator at SMART CAMP, professor of biology and biological engineering at MIT, and corresponding author of the paper.
The research is conducted by SMART and supported by the National Research Foundation Singapore under its Campus for Research Excellence and Technological Enterprise program.
#Administration#aging#Analytics#approach#arthritis#author#Behavior#Biological engineering#Biology#cartilage#cell#cell cultures#cell therapy#Cells#change#Composition#contamination#development#Diseases#drug#effects#Electrical engineering and computer science (EECS)#energy#engineering#enterprise#Environment#evaluation#Food#Foundation#functions
0 notes
Text
The Importance of Bioenergetics in Health
Introduction
Bioenergetics is a critical field of study that focuses on the mechanisms of energy transfer and transformation within living organisms. It is fundamental to understanding cellular function, metabolism, and the overall health of organisms. This article examines why bioenergetics serves as the basis for healthy living, describes the bioenergetics cycle in detail, defines bioenergetics deficit, and discusses strategies to reverse the downward spiral of the bioenergetics cycle.
Why is Bioenergetics the Basis of Healthy Living?
Bioenergetics is vital for several reasons:
Energy Production and Cellular Function: All cellular processes, including biosynthesis, signal transduction, and muscle contraction, require energy, primarily in the form of adenosine triphosphate (ATP). Effective energy production is essential for maintaining cellular homeostasis and function.
Metabolic Pathways: Bioenergetics encompasses key metabolic pathways, including glycolysis, the Krebs cycle, and oxidative phosphorylation. These pathways are crucial for converting substrates from food into ATP, ensuring that cells have adequate energy for physiological functions.
Homeostasis and Adaptation: Energy metabolism plays a significant role in maintaining homeostasis. Organisms must efficiently manage energy intake, storage, and expenditure to adapt to changes in environmental conditions, physical activity, and stressors.
Health and Disease Prevention: Disruptions in bioenergetic pathways are linked to various chronic diseases, including obesity, diabetes, and neurodegenerative disorders. Understanding bioenergetics can help identify potential therapeutic targets and preventive measures.
The Bioenergetics Cycle
The bioenergetics cycle consists of a series of interconnected processes that convert food into usable energy. The key components include:
1. Nutrient Intake
Nutrients from dietary sources—carbohydrates, proteins, and fats—serve as the primary substrates for energy production. Each macronutrient undergoes distinct metabolic pathways to generate ATP.
2. Digestion and Absorption
In the gastrointestinal tract, complex macromolecules are broken down into simpler units:
Carbohydrates → Monosaccharides (e.g., glucose).
Proteins → Amino acids.
Fats → Fatty acids and glycerol.
These components are absorbed into the bloodstream and transported to cells.
3. Cellular Uptake
Cellular uptake of nutrients is facilitated by transport proteins, primarily insulin-mediated glucose transport in muscle and adipose tissues. Other nutrients utilize specific transport mechanisms to enter cells.
4. Metabolic Pathways
Glycolysis
Occurs in the cytoplasm, converting glucose into pyruvate.
Produces a net yield of 2 ATP and 2 NADH per glucose molecule.
Krebs Cycle (Citric Acid Cycle)
Takes place in the mitochondria, where acetyl-CoA (derived from pyruvate and fatty acids) undergoes oxidation.
Produces 3 NADH, 1 FADH2, and 1 GTP (or ATP) per cycle, along with carbon dioxide as a waste product.
Oxidative Phosphorylation
Located in the inner mitochondrial membrane, this process uses NADH and FADH2 to drive ATP synthesis.
The electron transport chain (ETC) complexes transfer electrons, creating a proton gradient that powers ATP synthase.
Oxygen serves as the final electron acceptor, forming water.
5. Energy Utilization
The ATP produced is utilized for various cellular functions, including muscle contraction, biosynthetic reactions, and maintaining ion gradients.
6. Waste Removal
Byproducts of metabolism, particularly carbon dioxide and urea, must be efficiently removed from the body to maintain metabolic balance and prevent toxicity.
What is a Bioenergetics Deficit?
A bioenergetics deficit arises when the energy expenditure of an organism exceeds its energy intake or when energy production is impaired. This condition can manifest as:
Causes of Bioenergetics Deficit
Inadequate Nutritional Intake: Insufficient caloric intake or macronutrient imbalances can lead to decreased energy availability.
Impaired Metabolism: Conditions like mitochondrial dysfunction, insulin resistance, or hormonal imbalances can disrupt normal energy production.
Increased Energy Demand: Situations such as intense physical activity, chronic stress, or illness can elevate energy requirements beyond available supply.
Symptoms of Bioenergetics Deficit
Chronic fatigue
Decreased physical performance
Impaired cognitive function
Mood disturbances
Weight loss or gain due to altered metabolic rates
How to Reverse the Downward Spiral of the Bioenergetics Cycle
Reversing a bioenergetics deficit requires a multifaceted approach that targets nutritional, metabolic, and lifestyle factors. Key strategies include:
1. Optimize Nutritional Intake
Balanced Diet: Ensure adequate caloric intake with a focus on whole foods that provide complex carbohydrates, healthy fats, and high-quality proteins. This includes fruits, vegetables, whole grains, lean meats, and legumes.
Nutrient Timing: Consider meal timing strategies to optimize energy availability, especially around physical activity.
2. Enhance Metabolic Function
Regular Exercise: Engage in both aerobic and resistance training. Exercise enhances mitochondrial biogenesis, improves insulin sensitivity, and promotes efficient energy use.
Strength Training: Increases muscle mass, which raises resting metabolic rate and enhances energy utilization.
3. Manage Stress
Stress Reduction Techniques: Implement mindfulness practices such as meditation, yoga, and deep breathing exercises to help mitigate stress-induced energy expenditure.
4. Support Mitochondrial Health
Nutraceuticals: Supplements such as Coenzyme Q10, L-carnitine, and alpha-lipoic acid may support mitochondrial function and enhance energy production.
Dietary Antioxidants: Foods rich in antioxidants (e.g., berries, dark chocolate, and leafy greens) can help mitigate oxidative stress and support mitochondrial health.
5. Monitor and Adjust
Track Energy Intake and Expenditure: Utilize food diaries or apps to monitor caloric intake, macronutrient balance, and physical activity levels.
Seek Professional Guidance: Consult healthcare providers or nutritionists for personalized assessments and interventions.
Conclusion
Bioenergetics is fundamental to understanding the processes that underpin healthy living. The intricate bioenergetics cycle illustrates how energy is produced, utilized, and managed within the body. Recognizing the signs of bioenergetics deficit and implementing targeted strategies can help individuals restore energy balance and enhance overall health. By prioritizing nutrition, metabolic function, and lifestyle choices, we can effectively reverse the downward spiral of the bioenergetics cycle and foster a state of optimal well-being.
0 notes
Quote
Brain metastasis of advanced breast cancer often results in deleterious consequences. Metastases to the brain lead to significant challenges in treatment options, as the blood–brain barrier (BBB) prevents conventional therapy. Thus, we hypothesized that creation of a nanoparticle (NP) that distributes to both primary tumor site and across the BBB for secondary brain tumor can be extremely beneficial. Here, we report a simple targeting strategy to attack both the primary breast and secondary brain tumors utilizing a single NP platform. The nature of these mitochondrion-targeted, BBB-penetrating NPs allow for simultaneous targeting and drug delivery to the hyperpolarized mitochondrial membrane of the extracranial primary tumor site in addition to tumors at the brain. By utilizing a combination of such dual anatomical distributing NPs loaded with therapeutics, we demonstrate a proof-of-concept idea to combat the increased metabolic plasticity of brain metastases by lowering two major energy sources, oxidative phosphorylation (OXPHOS) and glycolysis. By utilizing complementary studies and genomic analyses, we demonstrate the utility of a chemotherapeutic prodrug to decrease OXPHOS and glycolysis by pairing with a NP loaded with pyruvate dehydrogenase kinase 1 inhibitor. Decreasing glycolysis aims to combat the metabolic flexibility of both primary and secondary tumors for therapeutic outcome. We also address the in vivo safety parameters by addressing peripheral neuropathy and neurobehavior outcomes. Our results also demonstrate that this combination therapeutic approach utilizes mitochondrial genome targeting strategy to overcome DNA repair–based chemoresistance mechanisms.
Simultaneous targeting of peripheral and brain tumors with a therapeutic nanoparticle to disrupt metabolic adaptability at both sites | PNAS
0 notes
Text
Navigating Regulatory Frameworks in the Pyridoxine Hydrochloride Vitamin B6 Market

The Many Benefits of Pyridoxine Hydrochloride in Nutritional Supplements Introduction to Pyridoxine Hydrochloride Pyridoxine hydrochloride, also known as pyridoxine HCl, is the hydralcohol HCl salt form of vitamin B6. It is commonly used in nutritional supplements and processed foods due to its high bioavailability. Pyridoxine hydrochloride plays a crucial role in over 100 enzymatic reactions involved in protein metabolism and synthesis of neurotransmitters. Role in Protein Metabolism As a cofactor for enzymes involved in amino acid metabolism, pyridoxine hydrochloride is important for building proteins and breaking down amino acids from dietary proteins. It helps convert the amino acids glycine and serine into other nonessential amino acids. Pyridoxine is also necessary for the synthesis of hemoglobin and other proteins in red blood cells. A deficiency can result in anemia due to impaired red blood cell formation. Pyridoxine supports skin, hair, and nail health by aiding in the metabolism of sulfur-containing amino acids like cysteine and methionine. Stress Reduction and Neurological Benefits Pyridoxine hydrochloride plays an important role as a cofactor for the synthesis of neurotransmitters like serotonin, norepinephrine, epinephrine, and gamma-aminobutyric acid (GABA). These neurotransmitters help regulate mood, reduce stress and anxiety, balance hormones, and promote restful sleep. Adequate vitamin B6 also supports nerve signaling and myelin sheath formation. Studies have found pyridoxine can help alleviate symptoms of carpal tunnel syndrome, reduce stress-induced cortisol levels, and potentially protect against age-related neurological decline. Energy Production and Cardiovascular Health Pyridoxine acts as a coenzyme in the metabolism of carbohydrates, fats, and proteins to extract energy. It supports the functioning of the citric acid cycle, where pyruvate is oxidized to produce energy carriers like ATP and NADH in the mitochondria. Maintaining healthy homocysteine levels is important for cardiovascular health, and B6 is involved in folate-dependent homocysteine metabolism. Some research links pyridoxine supplementation to reduced inflammation and lower risk of heart disease. Cell Growth and DNA Synthesis As a coenzyme for enzymes involved in chemical methylation processes, pyridoxine is crucial for cell growth and DNA synthesis. It plays a role in histamine synthesis, which regulates inflammatory responses, and the production of antioxidants like glutathione. Pyridoxine is essential during pregnancy for rapidly growing fetal tissues as well as in childhood for growth and development. A deficiency can lead to disorders like vascular purpura, seizures in newborns, and peripheral neuropathy in children. Regulation of Hormone Levels Pyridoxine is involved in hormone synthesis and metabolism as a cofactor for various enzymes. This includes the production of sex hormones like estrogen, testosterone, and progesterone. It aids thyroid hormone regulation and helps convert cortisol into its inactive form. Pyridoxine also indirectly promotes melatonin synthesis for quality sleep. Supplementing with pyridoxine may help relieve premenstrual syndrome (PMS) symptoms and improve fertility in women. In men, it may support testosterone and healthy sperm production. In summary, pyridoxine hydrochloride is an important water-soluble B vitamin with wide-ranging benefits. As a cofactor for many enzymatic reactions central to metabolism, it supports protein synthesis, energy production, cell growth, nerve signaling, and hormone balance. More research continues to uncover additional roles for this versatile micronutrient in reducing stress, boosting immunity, and promoting heart health. Pyridoxine supplementation remains widely used due to its effectiveness and low cost.
#Pyridoxine Hydrochloride Vitamin B6 Market Growth#Pyridoxine Hydrochloride Vitamin B6 Market Trends#Pyridoxine Hydrochloride Vitamin B6 Market
0 notes
Text
Coenzyme Q10 (Ubiquinone) Market: Forthcoming Trends and Share Analysis by 2030
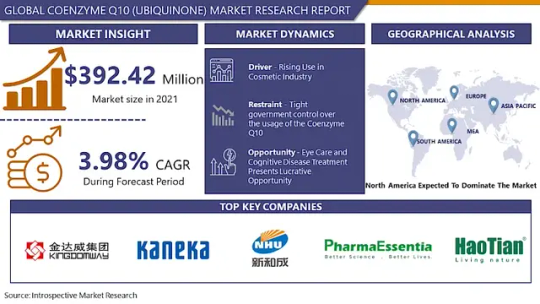
Coenzyme Q10 (Ubiquinone) Market was valued at USD 392.42 million in 2021 and is expected to reach USD 515.70 million by the year 2028, at a CAGR of 3.98%.
A coenzyme is referring to an organic molecule that holds together the active sites of certain enzymes to help in the catalysis of a reaction. Additionally, coenzymes can role as intermediate carriers of electrons during these reactions or are transferred between enzymes as functional groups. For instance, while the conversion of pyruvate to acetyl coenzyme A (CoA), various coenzymes including free CoA, lipoic acid (LA), thiamine pyrophosphate (TPP), flavin adenine dinucleotide (FAD), two cellular redox enzymes including oxidized nicotinamide adenine dinucleotide (NAD) and minimized nicotinamide adenine dinucleotide (NADH) are required. Moreover, coenzymes, which are frequently vitamins or derivatives of vitamins, hence act as a crucial role in the regulation of most enzyme activities.
Get Full PDF Sample Copy of Report: (Including Full TOC, List of Tables & Figures, Chart) @
https://introspectivemarketresearch.com/request/887
The latest research on the Coenzyme Q10 (Ubiquinone) market provides a comprehensive overview of the market for the years 2023 to 2030. It gives a comprehensive picture of the global Coenzyme Q10 (Ubiquinone) industry, considering all significant industry trends, market dynamics, competitive landscape, and market analysis tools such as Porter's five forces analysis, Industry Value chain analysis, and PESTEL analysis of the Coenzyme Q10 (Ubiquinone) market. Moreover, the report includes significant chapters such as Patent Analysis, Regulatory Framework, Technology Roadmap, BCG Matrix, Heat Map Analysis, Price Trend Analysis, and Investment Analysis which help to understand the market direction and movement in the current and upcoming years. The report is designed to help readers find information and make decisions that will help them grow their businesses. The study is written with a specific goal in mind: to give business insights and consultancy to help customers make smart business decisions and achieve long-term success in their particular market areas.
Leading players involved in the Coenzyme Q10 (Ubiquinone) Market include:
Kingdomway, Kaneka, Space Biology, NHU, Pharma Essentia, Yuxi Jiankun, Haotian, ZMC and Other Major Players.
If You Have Any Query Coenzyme Q10 (Ubiquinone) Market Report, Visit:
https://introspectivemarketresearch.com/inquiry/887
Segmentation of Coenzyme Q10 (Ubiquinone) Market:
By Type
Chemical Synthesis
Microbial Fermentation
By Application
Food
Medicine
Cosmetics
By Regions: -
North America (US, Canada, Mexico)
Eastern Europe (Bulgaria, The Czech Republic, Hungary, Poland, Romania, Rest of Eastern Europe)
Western Europe (Germany, UK, France, Netherlands, Italy, Russia, Spain, Rest of Western Europe)
Asia Pacific (China, India, Japan, South Korea, Malaysia, Thailand, Vietnam, The Philippines, Australia, New Zealand, Rest of APAC)
Middle East & Africa (Turkey, Bahrain, Kuwait, Saudi Arabia, Qatar, UAE, Israel, South Africa)
South America (Brazil, Argentina, Rest of SA)
Highlights from the report:
Market Study: It includes key market segments, key manufacturers covered, product range offered in the years considered, Global Coenzyme Q10 (Ubiquinone) Market, and research objectives. It also covers segmentation study provided in the report based on product type and application.
Market Executive Summary: This section highlights key studies, market growth rates, competitive landscape, market drivers, trends, and issues in addition to macro indicators.
Market Production by Region: The report provides data related to imports and exports, revenue, production and key players of all the studied regional markets are covered in this section.
Coenzyme Q10 (Ubiquinone) Profiles of Top Key Competitors: Analysis of each profiled Roll Hardness Tester market player is detailed in this section. This segment also provides SWOT analysis of individual players, products, production, value, capacity, and other important factors.
If you require any specific information that is not covered currently within the scope of the report, we will provide the same as a part of the customization.
Acquire This Reports: -
https://introspectivemarketresearch.com/checkout/?user=1&_sid=887
About us:
Introspective Market Research (introspectivemarketresearch.com) is a visionary research consulting firm dedicated to assisting our clients to grow and have a successful impact on the market. Our team at IMR is ready to assist our clients to flourish their business by offering strategies to gain success and monopoly in their respective fields. We are a global market research company, that specializes in using big data and advanced analytics to show the bigger picture of the market trends. We help our clients to think differently and build better tomorrow for all of us. We are a technology-driven research company, we analyse extremely large sets of data to discover deeper insights and provide conclusive consulting. We not only provide intelligence solutions, but we help our clients in how they can achieve their goals.
Contact us:
Introspective Market Research
3001 S King Drive,
Chicago, Illinois
60616 USA
Ph no: +1-773-382-1049
Email: [email protected]
#Coenzyme Q10 (Ubiquinone)#Coenzyme Q10 (Ubiquinone) Market#Coenzyme Q10 (Ubiquinone) Market Size#Coenzyme Q10 (Ubiquinone) Market Share#Coenzyme Q10 (Ubiquinone) Market Growth#Coenzyme Q10 (Ubiquinone) Market Trend#Coenzyme Q10 (Ubiquinone) Market segment#Coenzyme Q10 (Ubiquinone) Market Opportunity#Coenzyme Q10 (Ubiquinone) Market Analysis 2023
0 notes
Text
What is the Breathing Mechanism?
Breathing is a fundamental and automatic process that allows us to take in and release oxygen, an essential element for our survival. It is a mechanism that enables the exchange of gases in our body, ensuring the delivery of oxygen to our cells and the removal of carbon dioxide, a waste product generated by cellular respiration.
Breathing Mechanism
The breathing mechanism involves two distinct processes: inspiration and expiration. These two steps work together harmoniously to ensure the continuous exchange of gases in our respiratory system.
Inspiration and Mechanism of Inspiration
During inspiration, the diaphragm and the intercostal muscles contract, causing the ribcage to expand and the diaphragm to flatten. This action increases the volume of the thoracic cavity, leading to a decrease in pressure within the lungs. As a result, air rushes in from the surrounding atmosphere into the lungs, filling up the expanded alveoli, the tiny sacs responsible for gas exchange. This process is largely driven by the contraction of the diaphragm, which plays a crucial role in initiating the inhalation of air.
Expiration and Mechanism of Expiration
Expiration, on the other hand, is a passive process that occurs when the diaphragm and the intercostal muscles relax. As they relax, the thoracic cavity decreases in volume, causing an increase in pressure within the lungs. This increased pressure forces air out of the lungs into the atmosphere, completing the process of exhalation.
Contrary to popular belief, respiration is not synonymous with breathing. While breathing is the physical act of inhaling and exhaling air, respiration refers to the complex series of chemical reactions that take place within our cells to produce energy.
Respiration Mechanism
The process of respiration can be divided into three main steps: glycolysis, the Krebs cycle, and oxidative phosphorylation. Glycolysis occurs in the cytoplasm of the cell and involves the breakdown of glucose into smaller molecules called pyruvate. The Krebs cycle takes place in the mitochondria and further breaks down the pyruvate molecules, releasing carbon dioxide and generating small amounts of ATP, the energy currency of cells. Finally, oxidative phosphorylation occurs within the inner mitochondrial membrane and is the most energy-producing step of respiration. Here, electrons are passed along a series of proteins, creating a proton gradient that is then utilized to produce a large amount of ATP.
Understanding the breathing mechanism and the process of respiration is crucial for comprehending the intricate workings of our respiratory system. By maintaining a healthy breathing pattern and optimizing the exchange of gases, we can ensure the sufficient delivery of oxygen to our cells and the efficient removal of carbon dioxide. Our breath is not merely an automatic process; it is a vital element that sustains our life.
Keen on easily understanding complex concepts, as demonstrated above? Dive into our Tutoroot Blog section for simplified learning. Enhance your grasp of subjects and have your questions answered through Tutoroot online tuition.Try Tutoroot’s online home tuitions today by booking a FREE DEMO session.
0 notes
Text
TCA cycle: This pathway oxidizes pyruvate to produce carbon dioxide, energy in the form of ATP, and reduced coenzymes (NADH and FADH2)
TCA Cycle :- The citric acid cycle, also known as the Krebs cycle or the tricarboxylic acid (TCA) cycle, is a series of chemical reactions that occurs in the mitochondria of eukaryotic cells and in the cytosol of prokaryotic cells. The TCA cycle is a major pathway of aerobic respiration, which is the process by which organisms use oxygen to break down molecules like glucose to produce…
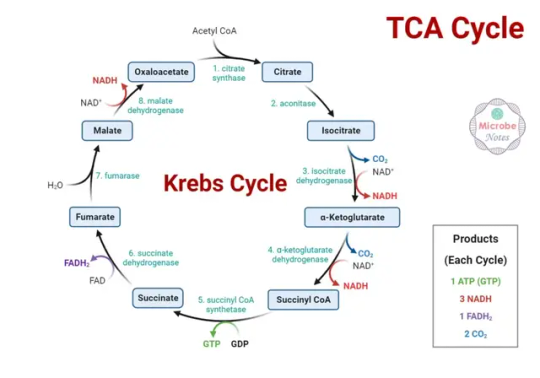
View On WordPress
0 notes
Text
Biochemistry 10e Jeremy Berg Test Bank

Biochemistry 10e Jeremy Berg Test Bank
Table of Contents
1 Biochemistry in Space and Time 2 Protein Composition and Structure 3 Binding and Molecular Recognition 4 Protein Methods 5 Enzymes: Core Concepts and Kinetics 6 Enzyme Catalytic Strategies 7 Enzyme Regulatory Strategies 8 DNA, RNA, and the Flow of Genetic Information 9 Nucleic Acid Methods 10 Exploring Evolution and Bioinformatics 11 Carbohydrates and Glycoproteins 12 Lipids and Biological Membranes 13 Membrane Channels and Pumps 14 Signal-Transduction Pathways 15 Metabolism: Basic Concepts and Themes 16 Glycolysis and Gluconeogenesis 17 Pyruvate Dehydrogenase and the Citric Acid Cycle 18 Oxidative Phosphorylation 19 Phototrophy and the Light Reactions of Photosynthesis 20 The Calvin–Benson Cycle and the Pentose Phosphate Pathway 21 Glycogen Metabolism 22 Fatty Acid and Triacylglycerol Metabolism 23 Protein Turnover and Amino Acid Catabolism 24 Integration of Energy Metabolism 25 Biosynthesis of Amino Acids 26 Nucleotide Biosynthesis 27 Biosynthesis of Membrane Lipids and Steroids 28 DNA Replication, Repair, and Recombination 29 RNA Functions, Biosynthesis, and Processing 30 Protein Biosynthesis 31 Control of Gene Expression 32 Principles of Drug Discovery and Development Biochemistry 10e Jeremy Berg Test Bank sample Download Read the full article
0 notes
Text
Archaea can induce the host AKT PI3K, AMPK, HIF alpha and NFKB producing the Warburg metabolic phenotype.10 The increased glycolytic hexokinase activity indicates the generation of the Warburg phenotype. A high fibre and high MCT modified vegetarian ketogenic diet can inhibit hexokinase activity and glycolysis and reverse the Warburg phenotype. The generation of the Warburg phenotype is due to activation of HIF alpha. This stimulates anaerobic glycolysis, inhibits pyruvate dehydrogenase, inhibits mitochondrial oxidative phosphorylation, stimulates heme oxygenase, stimulates VEGF and activates nitric oxide synthase. The low carbohydrate diet generates less of glucose and inhibits the glycolytic pathway. This reverses the Warburg phenotype. The high fibre intake generates short chain fatty acids butyrate and propionate. Short chain fatty acids bind to lymphocyte GPCR receptors and are immunosuppressive. The reduction in cytokine generation inhibits the Warburg phenotype. The anti-archaeal and anti-viroidal action of MCT and dietary fiber also inhibits the generation of the Warburg phenotype.11-15
0 notes