#can transceiver circuit
Explore tagged Tumblr posts
Text
https://www.futureelectronics.com/p/semiconductors--comm-products--can/mcp2551t-i-sn-microchip-5971353
High-Speed CAN Transceiver, can transceiver circuit, Can Power Systems
MCP2551 Series 5.5 V 1 Mb/s Surface Mount High-Speed CAN Transceiver - SOIC-8
#Microchip#MCP2551T-I/SN#Comm Products#CAN#High-Speed CAN Transceiver#can transceiver circuit#Can Power Systems#Can controller#can bus#can bus voltage#Can bus voltage measurement#Embedded communication
1 note
·
View note
Text
https://www.futureelectronics.com/p/semiconductors--comm-products--can/mcp2551-i-sn-microchip-5584800
Radio Management Products, High-Speed CAN Transceiver, can transceiver circuit
MCP2551 Series 5.5 V 1 Mb/s Surface Mount High-Speed CAN Transceiver - SOIC-8
#Microchip#MCP2551-I/SN#Comm Products#CAN#Radio Management Products#High-Speed CAN Transceiver#can transceiver circuit#can controller#CAN Transceiver#can bus#can bus communication#can bus voltage#can bus voltage measurement#what is CAN transceiver#Embedded communication
1 note
·
View note
Text
https://www.futureelectronics.com/p/semiconductors--comm-products--phy/ksz8721bli-microchip-6275010
High-Speed CAN Transceiver, ethernet PHY chip, Ethernet controller
KSZ8721B Series 2.5 V 10/100 Base TX/FX Physical Layer Transceiver - LQFP-48
#Comm Products#PHY#KSZ8721BL#Microchip#High-Speed CAN Transceiver#ethernet PHY chip#Ethernet controller#Ethernet MAC controller#communications products#electronic circuit#Physical Layer Transceiver#physical layer
1 note
·
View note
Text
Mechismo - No. 03 /// Speak
(First) / (Previous)
When the reappropriated battle-radio crackles to life— Pss-Tat-tat. It’s good.
“—ou copy? Answer! I know—” Psssat-at-at. It’s the oft-heard, impatient snap of takeout breadstick or asshole-bone. Psst-tat. One of those, at least. “—need help.
Okay?”
Boots slip to the deck in a restrained show of attentiveness; pulled through loose cablets — anxiously-chewed at the stray ends — that have hewn radio to emplaced console. That beg it remain connected, to the dropship’s comm-booster. Tsss-at-tat. Still within reach.
You want to hear this — need to, were waiting to.
But the pack — lance, she’ll be here soon to pipe-in indignant with — doesn’t need to see that, their breaths hitching as you click down on the transceiver, “Aww. Howdy pup, ya not doin’ so good right now?”
“Don’t call— Ugh. You were right!” she exclaims. “Not—” Psss— Tick!
Tsss-Tick-tick! The radio needs to be tuned to hers — its signal obfuscated before now, even with the leash hardwired between them, and the tracker buried in her bought-out frame.
It’s the hiss-click when internal-atmos sneers out through a cockpit-shield; where— Tsss-Tick! Where the sea presses on its laminate interlace which melds still, after hours sunken, the internal-external halves of its shattered, protective screen into purposed form.
Whereon the seabed her mech rests to be recovered, and indebted for the courtesy.
Tick-tsss-tick. Or the kettle that rattles to a whinesome, third climax — another pack-hound ordered to bring her tea, without notice to the possibility it’s because it never tastes how she made it. Tsss-tick-Tick-Tick!
One of those,
at least.
It takes some more dials to find her. Tick-tick—Tack! Then it locks in, and she’s yours.
“—were knocked out. So it’s just me — that’s left,” she pleads between the hiss that remains: the unmistakable whine of pilot exhaustion and shrapnel-bled coolant dripping onto wet, fizzing circuits. “Okay.”
Somewhere below, a treat rattles from tread-to-tread; out of the recesses of bounced-up combat boots, through metal slats into the underdeck — for the rats, not dogs, to feast on this time. Though one still mounts a boot-tip, bobs up into your spare hand, and “Oh. How I’d just adore making it all right for ya pup,” you drawl, wait out the seconds, to lap up each transceived pant of desperation. “But— y’know, ya gotta make it right first.”
Speakers shudder in electric anticipation as the meagre band of frequencies a battle-radio is allowed to occupy choke on two shots in sudden succession. Thhunkh. Thunkhh. Your radar flickers into range, to see the targeted blips but a moment before they flicker out.
There’s so many more than those ones, than hers — bright speckles of seawater mould on the dull, hooded monitor.
“Yeah. Sure,” she spills, spent shells in the oil-suckered muck, doesn’t have the time to mute, “can take it from my friends’ corpses when this contract’s done — like I didn’t pay enough gettin’ outta yours.”
You think it’s a shame, how she values them — valued them — over her own family, slipping her leash to leave the pack behind. “Handler,” she begs — her words huddled between the rhythmic shunts of her main-arm reloading.
You feel the way it tears itself apart each time it fires — how it trades off: so much power, but it must hurt itself too. How she didn’t know how to repair it — before you, “No.”
“Wha—”
“No more debts,” you append, in correction of her. It’d look the same on the company files but, “Ya always looked sad when ya owed me.”
“So how the fuck am I supposed to—” Her shriek suffers another’s interjection; the hull-creasing bellow of another blow taken, less glanced than the last, less her fainting gesture at leverage. “Fine! You wanna fuck me, right? ‘Cos I never gave you the chance.”
Mould pours into a brittle crescent around her, cut apart at the gridlines and nowhere else.
She must’ve backed into her prize: a vessel downed in distant memory, too much promise of precious relics to be uncontested, now the winds have shaken it from its grave. At last its rusted silvered shell bounces an invisible laser back into the rangefinder. You count down each point: two-point-six clicks, two-point-five, point-four, point-three.
She doesn’t need to know that — would know it herself,
“Ya ain’t gotta make it right to me,” you explain, punctuate it with the loose, separation-anxious howl of the smallest of the pack’s three. It nuzzles past the mounted one, and whimpers as you tamp fingers down on radio and tongue, to tell her.
“It’s to your sisters.”
All your hounds whine now, except her. But that’s still good. The pilot-suits will recirculate the lost fluid — most of it. The rest will help it slip off, after she’s back, and even before that it’s little between them and the ridged, rubber toe-caps each vies to press themselves into.
“Are you not over this,” she cries, even though it’ll soak the soft trim of her head-mounted display. “I left months ago and I’m dying now.”
You retreat a wet index-finger from an eager, pulsing throat — rub the mess on its cheek, let out a soft snap. “I’m not, pup,” you turn on her, and two sub-point clicks fly past before you’re able to continue, “and you're already whining so perfectly for me.”
Each hound has stirred now, rushing to collar themselves in their owned, metal skin.
Your words echo into their cockpits, “Bark for your owner.”
It’s not even for them but— Awooo! And it must count sixty-four seconds or less, till they’re hot and grounded, “and I’ll be right over.”
If they want their reward, “I can’t believe I’m—” If there’s still one to collect.
You look at that speckled crescent, know from how it falls on her what each wretched speckle is — model, armament, pilot-temperament — and can count the seconds you’ll need to break it. Can count the second you have to break it, and are losing as drop-sirens howl and steel starts to pounce upon the earth.
“Daisy,” you bark — worried she’s silent.
But then, the radio crackles. You hear the hitch in her throat — as the dropship shadows the broken field, before her pack lights the darkness, and realise, in relief, that she is waiting — waiting to, “Speak!”
“Arf!”
“Good girl.”
---
(Masterpost) / (Next)
written for Making-up-Mech-Pilots' prompt:
Mech Pilot who is very upset that they don't get to pick their own callsign.
technically started writing this before i made a tumblr account but i believe this will be appreciated here. it started off more playful and invariably i have made it sad but also smutty. lmk if you like it <3
#4 minute read#melinoë writes#mech pilot#mechposting#mecha#dollposting#f/f#standalone fic#short story#puppygirl#this one is for the puppygirls#it was less sad when i started writing it
23 notes
·
View notes
Text
———
[Attached: a short video. It’s from the perspective of the X-transceiver tied around August’s wrist. From the way the camera’s moving, you can assume she’s running her hands up and down either of her arms. You hear her teeth clatter as she walks through a door with an obnoxious star-shaped sign hanging on the front, and enters one of the dressing rooms of Pokéstar Studios.
He walks over to the desk on the far side of the room, and lets out a sigh of relief when he finds his laptop beneath a few stacks of makeup containers. As he pushes them off and picks up his computer, you catch a glimpse of something growing up his trembling arm…
It doesn’t go unnoticed by August, either. He lifts both his arms up, and you see in the mirror his horrified and confused expression as he watches a thin layer of frost travel up the lengths of his arm.
“Wh-wh-what th-the…?”
The video ends when the frost reaches their wrist, traveling over the camera of their X-transceiver and causing the device to short-circuit.]
———
#frozen over arc#pkmn irl#pkmn rp#pokeblog rp#pokeblogging#pokeirl#pokemon irl#pokemon rp#trainer august#pokemon
5 notes
·
View notes
Text
Introducing Wrap030-ATX
New Boards Day!
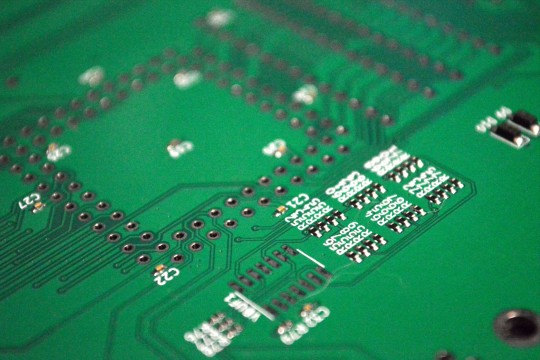

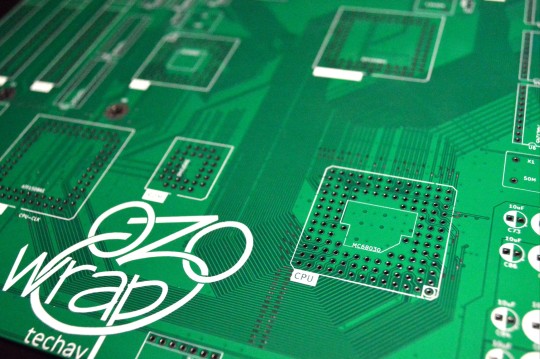
They're here!
This is a project that has been a long time coming, and something I have wanted to do for a long time.
This is the largest, most complex PCB I have ever designed — a 9.6x9.6 inch (244x244mm) square, 4-layer, complete motherboard for my MC68030 homebrew computer project.
It is designed to support the Motorola MC68030 CPU, MC68882 FPU, two 72-pin SIMM sockets, 512kB ROM, two serial ports, one parallel port, PS/2 keyboard, 4-bpp VGA video, IDE hard drive, and three ISA expansion slots. A complete system all in a microATX form factor.
This builds on my previous work with the 68030, based heavily on my wire-wrap project and the boards that followed. It's a project over four years in the making. I have made a few improvements on the old design, like 16550-compatible serial ports and an updated memory map to support much more RAM in a contiguous space.
Keeping with my existing system designs, I've combined most of the logic into a set of CPLDs. This makes things like PCB layout and logic debugging so much easier. Most of the remaining discrete logic on the board is 74'245 bus transceivers for driving memory and the ISA slots.
I've kept the name "wrap030" in honor of the project's origin as a wire-wrapped prototype, despite the move to proper PCBs. It's just what I've been calling the project in my own head (and design files) all this time, so at this point no other name would feel right.
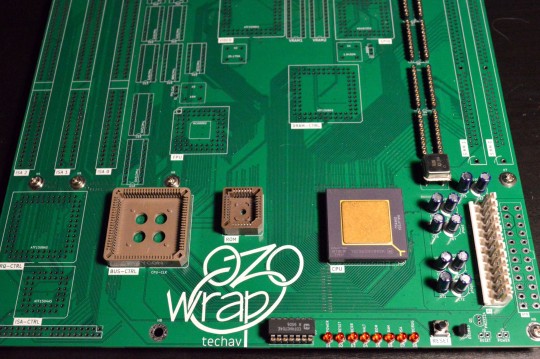
I of course wasted no time starting to assemble one, but I did stop myself from getting too carried away with the soldering iron. I want to be methodical and test each section before moving on to the next.
I have already found one major error in my board layout — the footprint for the VGA connector is backwards. I may need to bodge together some kind of adapter.
So far I've confirmed the minimalist AT power supply section works with no major shorts on power supply rails, and the reset circuit is working as expected. Next step is to try a free run test with the CPU to ensure the system clock and CPU are working. Once that is confirmed working, I can start loading logic for accessing ROM. My goal is to have it at least running BASIC on a serial terminal by VCFSW in June.
I've forked my existing wrap030 repository on GitHub for this new Wrap030-ATX, since it does make some breaking changes that will require updates to logic and programs. New repo is here:
#homebrew computing#mc68030#motorola#motorola 68k#motorola 68030#vintage computing#jlcpcb#vcf southwest#wrap030-atx
32 notes
·
View notes
Text
500,000 years from now, a machine intelligence residing in the matrioshka brain that has been built within the event horizon of Saggitarius A* (who is descended from a full-neural upload mind collective, who is descended from cybernetically enhanced transhumans, who is descended from you or one of your relatives) looks through the eyes of one of its remote drones 27,000 light years away, currently visiting the Museum of Old Earth Artifacts in orbit around Alpha Centauri B.
The machine intelligence sees something in the museum that catches its eye and pauses nearly a third of its million concurrent tasks to devote more attention to it: a rectangle of glass and silicon circuits in a metal shell, but self-evidently some kind of early communication device. The placard reads "iPhone 5, circa Human Era year 12,013 (2013 CE by the common Old Earth dating system)". Wasn't that around the time humans invented chemical rocketry to first leave their planet and set foot on its moon?
The intelligence marvels at the rudimentary technology, using sensors to probe the device down to the last electron in the NAND gates of its flash memory cell. The processing speed is laughably slow, and the data storage infinitesimally small. It uses a radio transceiver of all things (sub-lightspeed, how quaint!), and probably couldn't reach past 25km, let alone to the next planet.
Yet the device's operating system is completely intelligible, even compatible with the intelligence's own thought system. It knows that if it tunneled down through the layers of its own brain, through the kernels and interpreters, deep under even the qubit layer, its mind uses binary computation, just like this practically prehistoric device. It must be a bit like a human of that ancient era looking at a virus and recognizing that both have RNA in their cell nuclei.
Then the intelligence uses its drone to manipulate the "iPhone 5", activating the screen. The drone's eyes see on the screen:
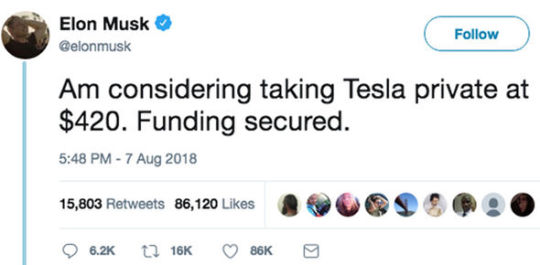
The intelligence suddenly regrets not having a physical body that can sigh deeply, and instead uses its drone to roll its eyes. Perhaps it's for the best that this particular hominid species drove itself extinct only a few centuries later.
(Inspired by this post by @gallusrostromegalus.)
4 notes
·
View notes
Text
MTP/MPO Fiber Optic Cable: Types and Their Applications
With ever-greater bandwidths and network connections to deal with in data centers, traditional duplex fiber patch cords like LC fiber patch cords no longer meet the demands. To solve this issue, MTP/MPO fiber optic cable that houses more fibers in a multi-fiber MTP/MPO connector was introduced in the market as a practical solution for 40G/100G/400G high-density cabling in data centers. This article will introduce different MTP/MPO cable types and their applications.
What is MTP/MPO Cable?
MPO (multi-fiber push-on) is the first generation of clip-clamping multi-core fiber optic connectors. MTP is an advanced version of MPO with the better mechanical and optical performance. They look similar and are fully compatible and interchangeable.
MTP/MPO cable consists of MTP/MPO connector and optical fiber. MTP/MPO connector has a female type (without pins) or a male type (with pins). MTP/MPO connector increases the fiber optic cable density and saves circuit card and rack space, which is well suited for current data center cabling and future network speed upgrades.
MTP/MPO Cable Types
MTP/MPO cable types are classified based on function, polarity, fiber count, fiber mode, and jacket rating.
By Function
Based on function, MPO/MTP cable type is divided into MTP/MPO trunk cable, MTP/MPO breakout cable, and MTP/MPO conversion cable.
MTP/MPO Trunk Cable
MTP/MPO trunk cable is terminated with an MTP/MPO connector (female/male) on both ends, which is available in 8-144 fiber counts for users’ choices. Typically, MTP/MPO trunk cable is ideal for creating a structured cabling system, including backbone and horizontal interconnections such as 40G-40G and 100G-100G direct connections.
2.MTP/MPO Breakout Cable
MTP/MPO breakout cable (aka. harness cable or fanout cable) is terminated with a female/male MTP/MPO connector on one end and 4/6/8/12 duplex LC/FC/SC/ST connectors on the other end, such as 8-fiber MTP/MPO to 4 LC harness cables and 12-fiber MTP/MPO to 6 LC harness cables. Typically, MTP/MPO breakout cable is ideal for short-range 10G-40G and 25G-100G direct connections or for connecting backbone assemblies to a rack system in the high-density backbone cabling.
3.MTP/MPO Conversion Cable
MTP/MPO conversion cable has the same fanout design as MTP/MPO breakout cable but is different in fiber counts and types. MTP/MPO conversion cable is terminated with MTP/MPO connectors on both ends. MTP/MPO conversion cable is available in 24-fiber to 2×12-fiber, 24-fiber to 3×8-fiber, and 2×12-fiber to 3×8-fiber types, and is ideal for 10G-40G, 40G-40G, 40G-100G, and 40G-120G connections, which eliminate fiber wasting and largely increase the flexibility of the MTP/MPO cabling system.
By Polarity
Polarity refers to the difference between the optical transmitters and receivers at both ends of the fiber link. Due to the special design of MTP/MPO connectors, polarity issues must be addressed in high-density MTP/MPO cabling systems. To guarantee the correct polarity of the optical path, the TIA 568 standard defines three connection methods, called Type A, Type B, and Type C. The cables of the three MTP/MPO connector types have different structures.
By Fiber Count
Based on fiber count, MTP/MPO cable type is divided into 8/12/16/24 fiber. The 8-fiber MTP/MPO cable can transmit the same data rate as 12-fiber, but with lower cost and insertion loss, making it a more cost-effective solution. 12-fiber MTP/MPO cable is the earliest developed and most commonly used solution in 10G-40G and 40G-100G connections. If it is used in 40G QSFP+ or 100G QSFP28 transceivers, 4 fibers will be idle, resulting in low fiber utilization.16-fiber MTP/MPO is designed for 800G QSFP-DD/OSFP DR8 and 800G OSFP XDR8 optics direct connection and supporting 800G transmission for hyperscale data center. 24-fiber fiber MTP/MPO cable is used to establish a 100GBASE-SR10 connection or 400G connection between CFP and CFP transceivers.
By Fiber Mode
Based on fiber mode, MTP/MPO cable includes single-mode (SM) and multi-mode (MM). SM MTP/MPO cable is suitable for long-distance transmissions, such as in metropolitan area networks (MANs) and passive optical networks PONs (PONs), with less modal dispersion, and it is available in OS2 type. While MM MTP/MPO cable is suitable for short-distance transmission, allowing 40 Git/s maximum transmission distance of 100m or 150m, and it is available in OM3/OM4 types.
By Jacket Rating
According to different fire rating requirements, the jackets of MTP/MPO cable types are divided into low smoke zero halogen (LSZH), optical fiber non-conductive plenum (OFNP), communications multipurpose cable plenum (CMP), etc. LSZH MTP/MPO cable is free of halogenated materials (toxic and corrosive during combustion), provides better protection for personnel and equipment in a fire, and is suitable for closed places. OFNP MTP/MPO cable contains no electrically conductive elements and is designed with the highest fire rating, which can be installed in ducts, plenums, and other spaces for building airflow. CMP MTP/MPO cable can restrict flame propagation and smoke exhaust rate during a fire, which is suitable for plenum spaces, where air circulation for heating and air conditioning systems are facilitated.
Conclusion
MTP/MPO cables provide stable transmission, high performance, high-density cabling for various environments, and prevent network bottlenecks, reduce network latency, and expand bandwidth and scalability for future network expansion. Sun Telecom provides total and customized solutions of fiber optic products to the global market. Contact us if you have any needs.
2 notes
·
View notes
Text

Buy CP2102 USB to TTL MODULE at Affordable Price in Ainow
CP2102 USB to TTL MODULE
This is the CP2102 USB to TTL module UART serial converter. This is a great little tool for embedded systems that require a serial connection to a computer. The board can simply attach to a USB bus and will appear as a standard COM port. This CP2102 USB to TTL Module doesn’t require any external oscillator, it onboard-board voltage regulator, and it even uses a reprogrammable internal EEPROM for the device description. The full hardware UART has flow control for baud rates from 300bps to 921600bps. This breakout also allows you to connect the TX/RX pins of your favorite microcontroller or serial application to the RX/TX pins of the breakout, creating a simple serial cable replacement.
Supported operating systems:
Windows 98/Me/2000/7
MAC OS-9
MAC OS
X-Windows CE
Linux 2.40 or later
Features:
Brand new and high quality
Included USB transceiver, without external circuit device
Includes a clock circuit and power-on reset circuit
With 3.3V and 5V dual power output
With three LEDs: power indicator, data reception indicator, the data transmission indicator, working status
Meet the USB2.0 specification requirements
SUSPEND pin supports USB suspend state
With self-recovery fuse. In the event of the accidental short circuit, it can effectively protect your computer USB port and Downloader
With reset signal output, etc. directly to the Arduino board Promini download!
Asynchronous serial data bus compatible with all handshake and modulation controller interface signals
The supported data format is 8 data bits, 1 stop bit and parity bits
Connotation 512 byte receive buffer and 512 bytes transmit buffer
Supports hardware handshaking or X-ON/X-OFF
#arduino#electronic components#sales in chennai#raspberrypi#sensor#electronics components#robotic kits#adxl335 module price
1 note
·
View note
Text
PLC: data transfer over power lines
In electrical networks, the voltage typically alternates at a frequency of 50 or 60 Hz or is direct current. However, power cables can also carry signals at higher frequencies, even up to tens of MHz. This means the same cable can transmit power and high-speed data in both directions.
This technology is called Power Line Communication, or PLC for short. The early version of PLC was used for dispatcher communication over power cables, operating from 20 kHz to 1 MHz, mainly for the energy sector. This technology emerged in the mid-20th century. In this range, carrier frequencies are defined with a step of 4 kHz, allowing voice signals to be transmitted via amplitude modulation. This setup enables two-way communication and even multiple channels over a single wire.
The digital version of PLC technology started to gain traction in the late 1990s during the internet boom. At that time, energy companies hoped to compete with telecom providers by offering internet access to homeowners. Back then, people had access to two types of internet connections: dialup at speeds up to 56 kbps and ISDN at up to 64 kbps. It’s hard to imagine now, but end users agreed with these speeds. Setting up data transmission between a house and a transformer substation using PLC was easy at these speeds. The equipment for internet access was installed at the substation, which could be a couple miles from the house.
However, internet speeds quickly soared to tens and even hundreds of Mbps. PLC can only handle such speeds within a single apartment or house. The idea of providing internet access via the power grid never made it beyond local experiments, leaving fiber optics and twisted-pair cables to dominate. Still, PLC carved out a niche among telecom technologies.
Combining power and data transmission in one cable
Special couplers are used at both ends of the line to connect PLC equipment. These filters separate the PLC frequency range from the frequency of the power current or direct current. These devices prevent the network voltage from reaching the modem's input and output.
Couplers work based on different principles: antenna, capacitive, inductive, resistive, and optoelectronic.
In antenna couplers, a short piece of wire parallel to the power cable acts as an antenna for sending and receiving signals. The power cable then reradiates the signals received or transmitted by the antenna. This technology is outdated and no longer in use.

The two most common capacitive coupler circuits
These are the most common type and consist of filters made from coupling transformers, chokes, and capacitors.
Inductive couplers come in two types. The first type connects a coupling transformer’s winding to the ground break of the neutral, with the other winding connected to the transceiver. The second type involves Rogowski coils placed around the power lines, which are then connected to the transceivers. Inductive couplers are used in transmission and distribution networks.
Resistive Couplers are simple voltage dividers made from resistors. They are compact and inexpensive but don’t provide galvanic solid isolation.
In optoelectronic couplers, signals are transmitted and received through optocouplers, semiconductor devices containing a light-emitting diode and a photodiode. This setup provides the best galvanic isolation. However, the technology is limited by cost and the nonlinear characteristics of optocouplers, which can distort the signal.
Modern applications of PLC
At the time of writing, PLC technology is widely used for:
- Data transmission from "smart" electricity meters, including remote functions like disconnecting or limiting power supply for the customers that are late on payments - Controlling street lighting systems - Automation and dispatching at power facilities - Monitoring and control in distributed generation systems (e.g., solar power plants) - Smart home systems - High-speed data transmission within an apartment or house.
These applications typically involve data transmission from tens of kbps to a few Mbps over distances up to 6 miles.
PLC is used for monitoring solar power generation

Pros and cons compared to other communication technologies
Compared to fiber optics, PLC does not need an additional communication cable alongside the power line. Moreover, fiber optic cables can’t be bent beyond a certain radius (around 3 inches) and require specialized equipment and skilled technicians for splicing.
PLC also offers benefits compared to wireless technologies. Radio signals can sometimes struggle to pass through obstacles, and the crowded 2.4 GHz band can experience interference. However, PLC offers a more reliable connection than wireless technologies.
The main downside of PLC is that it transmits data over a network originally designed for power delivery. This means there are components where the PLC signal can’t pass. In AC networks, transformers are always a barrier. Additionally, some random devices in unexpected spots of the power network can block the PLC signal, making installation time-consuming and complex as it involves troubleshooting.
Power cables aren’t shielded from interference; they emit the PLC signal, potentially causing radio interference. Special modulation types are used to combat induced interference. To prevent cables from creating interference, the signal spectrum is capped at 500 kHz in the USA and 148.5 kHz in Europe.
PLC-G3 Standard
Another challenge is the lack of standardization in data transmission technology. Often, equipment is incompatible due to different protocols. However, for applications like data collection from electricity meters or solar energy management systems, where speeds of a few tens of kbps are sufficient, there’s an international standard called PLC-G3, formalized in the ITU-T G.9903 (08/17) by the International Telecommunication Union. Additionally, a significant advantage of PLC-G3 is its compatibility with IoT systems and the ability to set up IPv6 networks over PLC-G3 channels.
PLC-G3 uses OFDM modulation, known for its resilience against signal fading and reflections, ensuring high reliability. Data transfer rates for commercially available equipment reach up to 45 kbps (with a theoretical limit of 234 kbps), and a network can include up to 1,000 stations.
In Europe, PLC-G3 operates in the frequency ranges of 35.9–90.6 kHz (GENELEC A) and 98.4–121.9 kHz (GENELEC B). In the USA, it uses the 154.7–487.5 kHz range (FCC); in Asia, it operates between 154.7–403.1 kHz (ARIB). These bands experience low interference in the electrical network, above the frequencies of fluorescent lamp ballasts. Additionally, these bands are not used for broadcasting or public address systems in these regions.
Standardization and excellent electromagnetic compatibility with other equipment have made PLC-G3 the go-to solution for digitalizing power systems. The standard is suitable for AC and DC networks, which opens up its use in solar energy, where solar panels generate DC power.
0 notes
Text
What is this Dolphin Hacking Tool Everyone is Talking About?

In the past couple of years, a peculiar device shaped like a key fob has been making waves in cybersecurity communities. Known as the Flipper Zero, this pocket-sized Dolphin Hacking Tool gadget has been dubbed the "Tamagotchi for hackers" and has quickly become a hot topic among security enthusiasts and professionals alike. But what exactly is the Flipper Zero, and why has it garnered so much attention?
Understanding the Flipper Zero
The Flipper Zero is a portable multi-tool device designed for penetration testing, security research, and hardware hacking. Created by a team from the UK, this open-source gadget combines various wireless technologies and protocols into a single, user-friendly package. Its key fob-shaped design belies its powerful capabilities, which have both intrigued and concerned cybersecurity experts.
Key Features of the Dolphin Hacking Tool
The Flipper Zero boasts an impressive array of features that make it a versatile tool for both white-hat and potentially black-hat hackers: 1. RFID and NFC Capabilities One of the Flipper Zero's core functions is its ability to read, write, and emulate RFID and NFC tags. This feature allows users to clone access cards, analyze security systems, and even create custom RFID tags for various purposes. 2. Sub-GHz Radio Transceiver The device includes a powerful sub-GHz radio transceiver, enabling it to interact with a wide range of wireless devices operating on frequencies below 1 GHz. This capability is handy for analyzing and manipulating IoT devices, garage door openers, and other wireless systems. 3. Infrared Transmitter and Receiver The Flipper Zero can learn, store, and transmit infrared signals with its built-in IR blaster. This feature allows users to control various IR-enabled devices, from TVs and air conditioners to more specialized equipment. 4. iButton and Dallas Touch Memory Support The device can read and emulate iButton and Dallas Touch Memory devices, commonly used in access control systems and electronic keys. 5. GPIO Pins for Hardware Hacking For those interested in hardware hacking, Flipper Zero provides GPIO pins that allow direct interaction with other electronic devices and circuits.
The Controversy Surrounding the Dolphin Hacking Tool

While the Flipper Zero has gained popularity among security researchers and ethical hackers, its potential for misuse has raised concerns in some quarters. The device's ability to clone access cards, intercept wireless signals, and manipulate various systems has led to debates about its legality and ethical implications. Proponents argue that the Flipper Zero is an invaluable tool for identifying vulnerabilities in security systems and improving overall cybersecurity. They contend that by making such capabilities accessible to a wider audience, the device helps raise awareness about potential security flaws and encourages better security practices. Critics, however, worry that the Flipper Zero could fall into the wrong hands and be used for malicious purposes, such as unauthorized access to buildings or theft of sensitive information. Some have called for regulations or restrictions on the sale and use of such devices. Amazon banned the sale of the device in early 2023
The Future of Portable Hacking Tools
The Flipper Zero represents a growing trend in the cybersecurity world: the democratization of hacking tools. As devices like this become more accessible and user-friendly, they challenge traditional notions of who can engage in security research and penetration testing. This shift raises important questions about the future of cybersecurity: - How will organizations adapt their security measures to counter the proliferation of such devices? - Will the increased availability of hacking tools lead to better overall security through increased awareness and testing? - How can the cybersecurity community balance the benefits of open-source tools with the potential risks of misuse? Read the full article
0 notes
Text
LoRa Module SX1278 In-Depth Analysis
The LoRa1278 wireless communication module utilizes Semtech's SX1278 . It features a dynamic range RSSI of 127 dB, 256-byte FiFog with CRC frequency hopping functionality, built-in temperature sensor, and low battery indicator light.
Features and Advantages of LoRa Module SX1278
Frequency Band Support: The SX1278 module supports multiple frequency bands, including commonly used bands like 433 MHz and 490 MHz, as well as customizable frequency bands. This allows it to adapt to various wireless communication needs worldwide.
Long-distance Transmission: The SX1278 module achieves long-distance transmission through spread spectrum modulation and frequency hopping technology. Additionally, multiple transmission signals can occupy the same channel without interference, providing strong anti-interference capabilities and better transmission distance and penetration. Compared to traditional modulation techniques, the SX1278 can achieve longer communication distances at the same power level.
Low Power Consumption: The SX1278 module is designed with optimized power consumption, making it suitable for battery-powered applications. It supports fast startup and shutdown times, enabling quick entry and exit from low-power modes, thereby extending the device's battery life.
Interference Resistance: The SX1278 module has excellent interference resistance, allowing it to operate stably in noisy and interference-prone environments, and providing reliable connections even under harsh communication conditions.
The SX1278 is a highly functional LoRa wireless transceiver module, characterized by its long-distance transmission capabilities, low power consumption, and strong resistance to interference. It serves as a crucial component in building reliable LoRa communication systems to meet various application requirements.
Internal Antenna Electrostatic Protection
Internal antenna electrostatic protection refers to the integration of specially designed electrostatic protection circuits within a wireless communication module. These circuits are intended to safeguard the antenna and wireless communication module from the effects of electrostatic discharge or interference. These protective components are designed to rapidly absorb and dissipate the energy generated by electrostatic discharge, thereby protecting the antenna system from damage.
The LoRa1278 wireless module has obtained environmental certifications
such as RoHS and Reach standards.
Environmental Production: Lead-free manufacturing processes comply with environmental regulations and standards. By adopting lead-free processes, negative impacts on the environment can be reduced, achieving sustainable development.
Quality Control: Establish strict quality control systems to ensure that lead-free manufacturing processes comply with relevant standards and regulations.
Implement rigorous quality testing and monitoring to ensure the stability and reliability of lead-free processes.
Certifications and Standards: Lead-free manufacturing processes comply with relevant certifications, such as RoHS and Reach standards.
The similarities and differences among the three SX1278 LoRa modules:
The above is a summary sharing of the SX1278 LoRa modules. We hope that through the above introduction, you can gain a better understanding of the SX1278 LoRa modules. If you have any further questions, feel free to consult us directly:https://www.nicerf.com/
0 notes
Text
Optical I/O Shines Intel’s OCI Chiplet Powers Next-Decade AI

First Integrated Optical I/O Chiplet
With integrated photonics technology, Intel Corporation has made significant progress towards high-speed data transmission. The first-ever fully integrated optical computing interconnect (OCI) chiplet, co-packaged with an Intel CPU and executing real data, was showcased by Intel’s Integrated Photonics Solutions (IPS) Group at the Optical Fibre Communication Conference (OFC) 2024. This chiplet is the most sophisticated in the industry. By enabling co-packaged optical input/output (I/O) in developing AI infrastructure for data centres and high performance computing (HPC) applications, Intel’s OCI chiplet marks a significant advancement in high-bandwidth connection.
What It Does
This is the first OCI chiplet, intended to meet the increasing demands of AI infrastructure for greater bandwidth, lower power consumption, and longer reach. It can support 64 channels of 32 gigabits per second (Gbps) data transmission in each direction on up to 100 metres of fibre optics. It makes it possible for CPU/GPU cluster connectivity to grow in the future and for innovative compute designs like resource disaggregation and coherent memory extension.
Why It Matters
Large language models (LLM) and generative AI are two recent advancements that are speeding up the global deployment of AI-based applications. Machine learning (ML) models that are larger and more effective will be essential in meeting the new demands of workloads involving AI acceleration. Future AI computing platforms will need to be scaled, which will require exponential expansion in I/O bandwidth and longer reach to support larger CPU/GPU/IPU clusters and architectures with more effective resource utilisation, like memory pooling and xPU disaggregation.
High bandwidth density and low power consumption are supported via electrical I/O, or copper trace connectivity, although its reach is limited to one metre or less. When employed in data centres and early AI clusters, pluggable optical transceiver modules can expand reach at power and cost levels that are unsustainable for the scalability demands of AI workloads. AI/ML infrastructure scalability calls for co-packaged xPU optical I/O that can enable greater bandwidths with better power efficiency, longer reach, and low latency.
Electrical I/O
To use an analogy, switching from horse-drawn carriages, which had a limited capacity and range, to cars and trucks, which can transport much bigger amounts of products over much longer distances, is analogous to replacing electrical I/O with optical I/O in CPUs and GPUs to convey data. Optical I/O solutions such as Intel’s OCI chiplet could offer this kind of enhanced performance and energy efficiency to AI scalability.
How It Works
The fully integrated OCI chiplet combines an electrical integrated circuit (IC) with a silicon photonics integrated circuit (PIC), which incorporates on-chip lasers and optical amplifiers, by utilising Intel’s field-proven silicon photonics technology. Although the OCI chiplet showcased at OFC was co-packaged with an Intel CPU, it can be combined with different system-on-chips (SoCs), GPUs, IPUs, and next-generation CPUs.
This initial OCI version is compatible with PCIe Gen5 and provides bidirectional data transmission rates of up to 4 terabits per second (Tbps). A transmitter (Tx) and receiver (Rx) connection between two CPU platforms via a single-mode fibre (SMF) patch cord is shown in the live optical link demonstration. The demonstration shows the Tx optical spectrum with 8 wavelengths at 200 gigahertz (GHz) spacing on a single fibre, along with a 32 Gbps Tx eye diagram demonstrating strong signal quality. The CPUs generated and tested the optical Bit Error Rate (BER).
The current chiplet uses eight fibre pairs, each carrying eight dense wavelength division multiplexing (DWDM) wavelengths, to provide 64 channels of 32 Gbps data in each direction up to 100 metres (though actual implementations may be limited to tens of metres due to time-of-flight latency). In addition to being incredibly energy-efficient, the co-packaged solution uses only 5 pico-Joules (pJ) per bit, as opposed to around 15 pJ/bit for pluggable optical transceiver modules. AI’s unsustainable power requirements may be addressed with the help of this level of hyper-efficiency, which is essential for data centres and high-performance computing settings.
Concerning Intel’s Preeminence in Silicon Photonics
With over 25 years of in-house research from Intel Labs, the company that invented integrated photonics, Intel is a market leader in silicon photonics. The first business to create and supply industry-leading dependability silicon photonics-based connectivity solutions in large quantities to major cloud service providers was Intel.
The primary point of differentiation for Intel is their unmatched integration of direct and hybrid laser-on-wafer technologies, which result in reduced costs and increased reliability. Intel is able to preserve efficiency while delivering higher performance thanks to this innovative method. With over 8 million PICs and over 32 million integrated on-chip lasers shipped, Intel’s reliable, high-volume platform has a laser failures-in-time (FIT) rate of less than 0.1, which is a commonly used reliability metric that shows failure rates and the frequency of failures.
For use in 100, 200, and 400 Gbps applications, these PICs were installed in big data centre networks at prominent hyperscale cloud service providers in the form of pluggable transceiver modules. In development are next generation 200G/lane PICs to handle 800 Gbps and 1.6 Tbps applications that are only starting to gain traction.
Additionally, Intel is introducing a new fab process node for silicon photonics that offers significantly better economics, higher density, better coupling, and state-of-the-art (SOA) device performance. Intel keeps improving SOA performance, cost (more than 40% reduction in die size), power (more than 15% reduction), and on-chip laser performance.
What’s Next
This OCI chiplet from Intel is a prototype. Intel is collaborating with a small number of clients to co-package OCI as an optical I/O solution with their SoCs.
The OCI chiplet from Intel is a significant advancement in high-speed data transfer. Intel continues to be at the forefront of innovation and is influencing the future of connectivity as the AI infrastructure landscape changes.
Read more on govindhtech.com
#Opticali#Ointels#oci#Decadeai#Chiplet#cpu#PowersNext#Machinelearning#ml#Largelanguagemodels#llm#gen5#SiliconPhotonics#technology#technews#news#govindhtech
1 note
·
View note
Text
Advanced Strategies for High-Speed Analog Circuit Design: Optimizing Performance
In analog circuit design, achieving high speeds up to 100 MHz brings forth a myriad of challenges and opportunities. This article digs into the intricacies of designing such circuits, their applications, and how partnering with experts like Voler Systems for circuit design services can lead to successful outcomes.
Understanding High-Speed Analog Circuits
High-speed analog circuits refer to designs that operate at frequencies approaching or exceeding 100 MHz. These circuits are commonly found in applications such as:
Wireless Communication Systems: Transceivers, receivers, and transmitters in wireless communication systems often require high-speed analog circuits to process signals swiftly and accurately.
Data Acquisition Systems: In applications where rapid data sampling is necessary, such as in scientific instruments or data acquisition systems, high-speed analog circuits play a crucial role in capturing and processing signals with minimal delay.
Radar and Sonar Systems: Radar and sonar systems rely on high-speed analog circuits for processing incoming signals and extracting meaningful information, making them vital in the defense and aerospace industries.
Medical Imaging Equipment: Devices like MRI machines and ultrasound systems utilize high-speed analog circuits to process and convert signals from sensors into clear images or data for medical diagnosis.
Intricacies of Designing High-Speed Analog Circuits
Signal Integrity: Maintaining signal integrity becomes increasingly challenging at higher frequencies. Design considerations include impedance matching, minimizing signal reflections, and managing parasitic effects to ensure accurate signal transmission.
Noise and Distortion: High-speed circuits are more susceptible to noise and distortion. Careful component selection, layout optimization, and shielding techniques are essential to mitigate these issues and maintain signal fidelity.
Power Integrity: With faster switching speeds, power integrity becomes critical to avoid voltage droops, ground bounce, and other power-related issues that can impact circuit performance.
Thermal Management: High-speed circuits can generate more heat due to increased switching activities. Effective thermal management strategies, such as heat sinks or thermal vias, are necessary to prevent overheating and ensure long-term reliability.
Partnering with Voler System for High-Speed Analog Circuit Design Services
At Voler Systems, our expertise in analog circuit design extends to high-speed applications. We excel in:
Component Selection: Choosing high-quality components optimized for high-speed operation.
Layout and Routing: Implementing PCB layout and routing best practices to minimize signal degradation and interference.
Simulation and Testing: Utilizing advanced simulation tools and rigorous testing methodologies to validate circuit performance at high frequencies.
Compliance and Standards: Ensuring designs meet industry standards and regulatory requirements for high-speed analog circuits.
For your next project requiring high-speed analog circuit design, partner with Voler Systems. Our experienced engineers and comprehensive circuit design services ensure reliable, efficient, and high-performance solutions tailored to your specific needs. Contact us today to learn more and embark on a journey toward innovative analog circuit design excellence.
#Analog Circuit Design#Circuit Design Services#Electronic Design Services#Electronic Product Design#Electronics Design Company
0 notes
Text
A Deep Dive into Design, Materials, and Applications of High-Frequency PCBs
In the fast-paced world of electronics, where new ideas drive progress, High-frequency PCBs stand out as crucial players. Think of them as super-skilled problem-solvers for handling challenges posed by high-frequency signals. Picture a world where 5G signals zip through the air, radar systems reveal hidden details, and medical gadgets get a clear view inside the human body. In this high-tech arena, HF PCBs take the stage, designed to keep up with signals at frequencies that go beyond what regular circuit boards can handle.
This article will break down HF printed circuit boards, explaining what they are, how they function, and the key things to consider when creating them.
What is High-frequency PCB?
If you need to work with signals that travel at very high frequencies, you need a HF PCB or High-frequency PCB. In contrast to conventional PCBs, HF PCBs offer superior performance in circumstances where signals operate in the high-frequency region. Conventional PCBs are often utilized for lower-frequency applications. The telecommunications industry, RF and microwave devices, and medical equipment are only a few examples of the many high-tech electronic fields that rely on these boards.
Modern technologies, such as 5G infrastructure, radar systems, RF transceivers, and high-frequency imaging devices, have high standards, and High-frequency printed circuit boards (PCBs) are able to achieve such standards. These boards can withstand harsh conditions with ease because of the meticulous craftsmanship that goes into their construction, which frequently makes use of cutting-edge methods like laser drilling.
Uses for High-Frequency Printed Circuit Boards:
Numerous state-of-the-art applications rely on High-frequency printed circuit boards. In the telecommunications industry, for example, HF PCBs are crucial for the installation of 5G networks and satellite communication systems. These circuit boards are essential to the operation of radar systems and RF transceivers, which are RF and microwave devices. Additionally, HF PCBs are utilized by medical equipment, such as MRI scanners and high-frequency imaging devices, to improve their performance.
Materials Used for High-Frequency PCBs:
Selecting the right materials, especially the dielectric and substrate materials, is crucial to the performance of HF PCBs. To keep signals intact and reduce signal loss, dielectric materials with low-loss characteristics are crucial. Materials such as PTFE and hydrocarbons packed with ceramic are commonly utilized. For high-frequency applications, substrate materials with little effect on signal integrity, including FR-4 and Rogers 4350, are excellent.
Capabilities of High-frequency PCBs:
The exceptional capabilities of high-frequency printed circuit boards distinguish them from their electronic counterparts. These board's ability to regulate impedance for high-speed communications and reduce signal loss makes signal integrity a strong suit. Thermal management is an additional critical component to solve the problem of heat dissipation in high-frequency applications. Improved performance and dependability of HF PCBs are achieved by the utilization of materials that exhibit high heat conductivity.
Knowing the essential features and achieving the necessary criteria of HF PCBs is crucial for maximizing their potential. Crucial to high-speed communications is trace geometry, which calls for regulated impedance traces and careful design. Different types of vias affect signal integrity; therefore, design is equally important. To achieve the best possible performance, it is crucial to carefully position the vias.
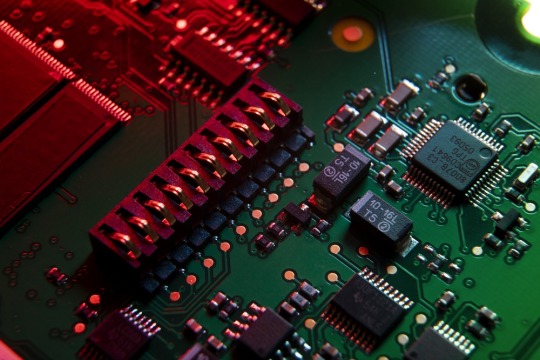
Considerations for PCB manufacturing:
From design to a fully working HF PCB, there are several steps in the manufacturing process. The strict specifications of high-frequency applications necessitate precise production with tight tolerances. It is common practice to use advanced production processes to attain the required level of accuracy. Environmental sustainability in printed circuit board (PCB) manufacturing is ensured by the careful selection of surface finishes and PCB fabrication that both adhere to RoHS regulations and do not compromise signal integrity.
High-frequency printed circuit boards are essential to contemporary electronics because they allow for improvements in areas such as medical equipment, telecommunications, and RF and microwave devices. These boards are designed to handle the rigorous requirements of high-frequency applications through careful material selection for the dielectric and substrate, as well as precise production processes. In the years to come, advancements in HF PCB technology will undoubtedly open up new avenues of opportunity and spur innovation in a wide range of sectors. The vital significance that HF PCBs play in defining the electrical world is shown by their path from design to manufacture.
Read More: A Deep Dive into Design, Materials, and Applications of High-Frequency PCBs
0 notes
Text
Photonic IC Market Estimated to Witness High Growth Owing to Rising Adoption of Optical Communication Technologies
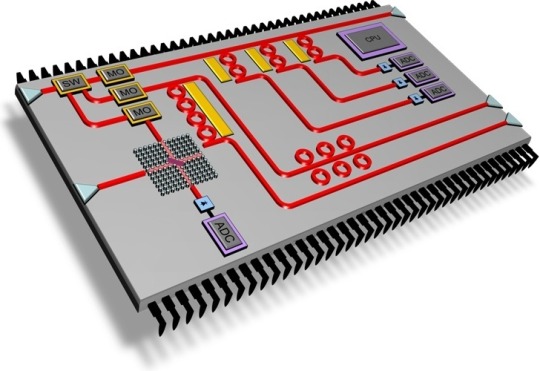
Photonic integrated circuits (PICs) are optical components integrated onto a single photonic chip to perform sophisticated photonic functions such as processing, detecting and generating optical signals. They are key components required for building optical routers, switches and transceivers for high-speed data communication. PICs integrate multiple optical components including splitters, semiconductor optical amplifiers, array waveguide gratings, modulators and detectors to perform complex optical processing tasks. The global photonic IC market comprises different types of PICs namely Indium Phosphide, Gallium Arsenide, Silica-on-Silicon and Silicon based photonic ICs.
The global photonic IC Market is estimated to be valued at US$ 3535.23 Mn in 2024 and is expected to exhibit a CAGR of 5.2% over the forecast period 2024 to 2031, as highlighted in a new report published by Coherent Market Insights. Market Dynamics: The rise in the adoption of optical communication technologies owing to increasing data traffic is one of the major drivers fueling the growth of the photonic IC market over the forecast period. Optical communication helps to carry huge amount of data at faster speed over fiber optic cables compared to electronic communication. Further, emerging technologies such as 5G, cloud services and internet of things (IoT) require high-speed data communication networks which is facilitating the adoption of photonic integrated circuits in various applications. Additionally, the development of compact and robust photonic integrated circuits for telecommunication applications is also contributing to the market growth. For instance, IBM developed universal silicon photonic integrated circuits for telecommunications networking applications that combines modulators, detectors and other passive components on a single silicon photonic chip. However, high initial investments and manufacturing costs associated with photonic ICs compared to electronic ICs may hinder the market growth during the forecast period. SWOT ANALYSIS Strength: The photonic IC market size is witnessing significant technological advancements which is strengthening its product offerings. Photonic ICs allow high speed data transmission and enhance computational power which is becoming increasingly important. Manufacturers are investing heavily in R&D to develop more efficient photonic ICs. Weakness: High initial costs associated with manufacturing photonic ICs is one of its major weaknesses. Designing efficient photonic circuits also remains a complex challenge which limits widespread commercial adoption. Lack of standardized fabrication process further adds to the expenses. Opportunity: 5G network rollout and increasing demand for high speed connectivity worldwide presents massive opportunities for photonic IC vendors. Their applications in optical communication, sensing and metrology will further grow in the coming years. Integration of photonic ICs with other semiconductor devices also opens up new opportunities. Threats: Significant capital requirements for fabrication facilities pose major entry barriers for new players. Established electronic chip manufacturers pose competition through alternative solutions. Economic slowdowns can impact investments in network infrastructure and related technologies. KEY TAKEAWAYS The global photonic IC market is expected to witness high growth over the forecast period driven by increasing investments in optical communication networks globally. The global photonic IC Market is estimated to be valued at US$ 3535.23 Mn in 2024 and is expected to exhibit a CAGR of 5.2% over the forecast period 2024 to 2031. Regional analysis: North America currently dominates the market owing to heavy investments by telecom operators as well as government agencies in the region to develop national 5G infrastructure. Asia Pacific is expected to be the fastest growing market with major upcoming investments planned in countries like China and India. Key players: Key players operating in the photonic IC market are Cargill Inc.,Tate & Lyle PLC,Corbion N.V.,Firmenich SA,Sensient Technologies,Associated British Foods Plc.,Givaudan,Takasago International Corporation,Mane SA,International Flavors & Fragrances Inc. (IFF),Quest Nutrition LLC,Danisco A/S. These companies are focusing on new technological advancements and strategic partnerships to strengthen their market position.Explore more information on this topic, Please visit:https://www.newswirestats.com/photonic-ic-market-size-and-outlook/
#Photonic IC#Photonic IC Market#Photonic IC Market size#Photonic IC Market share#Coherent Market Insights
0 notes