#antigen recognition
Explore tagged Tumblr posts
Text
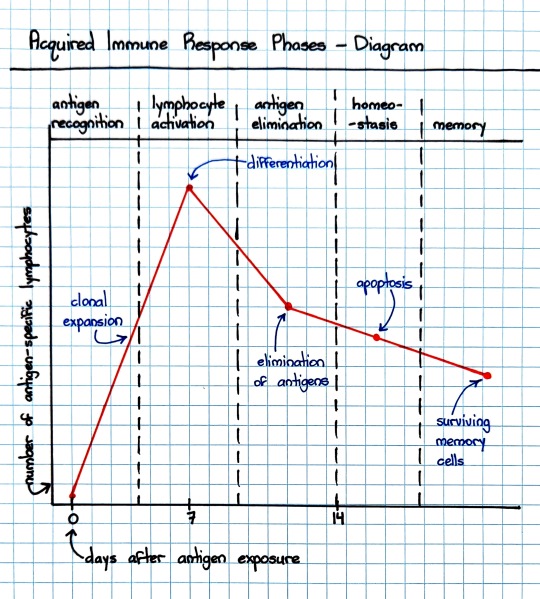
Patreon
#studyblr#notes#medblr#medical notes#med notes#immunology#immunology notes#immune system#immune system notes#antigen recognition#antigen elimination#homeostasis#biology#life science#health science#medical science#science#scienceblr#sciblr#memory cells#apoptosis#lymphocytes#t cells#b cells
16 notes
·
View notes
Text
Fourth dose bivalent COVID-19 vaccines outperform monovalent boosters in eliciting cross-reactive memory B cells to Omicron subvariants - Published Aug 8, 2024
Remember when that kid called me an uneducated idiot when I suggested that monovalent vaccines provide less long-lasting broad protection from all covid strains? I present Scientific Catharsis
Abstract Bivalent COVID-19 vaccines comprising ancestral Wuhan-Hu-1 (WH1) and the Omicron BA.1 or BA.5 subvariant elicit enhanced serum antibody responses to emerging Omicron subvariants. Here, we characterized the RBD-specific memory B cell (Bmem) response following a fourth dose with a BA.1 or BA.5 bivalent vaccine, in direct comparison with a WH1 monovalent fourth dose. Healthcare workers previously immunized with mRNA or adenoviral vector monovalent vaccines were sampled before and one-month after a fourth dose with a monovalent or a BA.1 or BA.5 bivalent vaccine. Serum neutralizing antibodies (NAb) were quantified, as well as RBD-specific Bmem with an in-depth spectral flow cytometry panel including recombinant RBD proteins of the WH1, BA.1, BA.5, BQ.1.1, and XBB.1.5 variants. Both bivalent vaccines elicited higher NAb titers against Omicron subvariants compared to the monovalent vaccine. Following either vaccine type, recipients had slightly increased WH1 RBD-specific Bmem numbers. Both bivalent vaccines significantly increased WH1 RBD-specific Bmem binding of all Omicron subvariants tested by flow cytometry, while recognition of Omicron subvariants was not enhanced following monovalent vaccination. IgG1+ Bmem dominated the response, with substantial IgG4+ Bmem only detected in recipients of an mRNA vaccine for their primary dose. Thus, Omicron-based bivalent vaccines can significantly boost NAb and Bmem specific for ancestral WH1 and Omicron variants, and improve recognition of descendent subvariants by pre-existing, WH1-specific Bmem, beyond that of a conventional, monovalent vaccine. This provides new insights into the capacity of variant-based mRNA booster vaccines to improve immune memory against emerging SARS-CoV-2 variants and potentially protect against severe disease.
One-sentence summary Omicron BA.1 and BA.5 bivalent COVID-19 boosters, used as a fourth dose, increase RBD-specific Bmem cross-recognition of Omicron subvariants, both those encoded by the vaccines and antigenically distinct subvariants, further than a monovalent booster.
41 notes
·
View notes
Text
Will these vaccines provide immediate protection?
Vaccines contain an active ingredient used to trigger generation of immune memory. That active ingredient, the antigen, is what initiates the immune response, starting with innate immune recognition and ending with adaptive immunity generation (read here for a more detailed summary on viral immunology).
Adaptive immune response leads to antibody by plasma cells, activation of helper T cells and cytotoxic T cells, and generation of memory B and T cells.
This process takes time, roughly 2 weeks after we encounter the foreign invader (either the vaccine antigen or an actual pathogen). Once memory B and T cells are created, the response to a second encounter is much quicker because all the preparation is already done. The adaptive immune system is ready to go: B cells can start spitting out antibodies, helper T cells can augment the B cell responses, and cytotoxic T cells can directly kill infected cells to limit the spread of infection.
Vaccines train the immune system in advance of an infection.
The ability to immediately respond means that even if virus infects you after vaccination, your body can fight it more quickly, reducing viral load, lessening disease severity, and decreasing the likelihood of more serious illness or death.
#novavax#covid#covid 19#sars cov 2#vaccines#vaccine#vaccination#get vaccinated#long covid#tw death#illness#chronic illness#covid vaccine#mrna#jn.1#kp.2#sf9#Novavax vaccine#mrna vaccine
16 notes
·
View notes
Link
The launch of AlphaFold signaled a revolution in computational modeling: armed with new knowledge of protein structures and unencumbered by limitations on resources and time, scientists were able to use the tool to further their understanding of protein structure, functionality, and interactions. However, AlphaFold’s success in the realm of antibody-antigen modeling is limited. A new study from the University of Maryland evaluates its accuracy and provides new insights into the factors influencing protein modeling.
Antibodies are an important part of the immune system and defend the body from a host of pathogenic organisms due to their high specificity and affinity to antigens. Usually, these targets are engaged through the use of hypervariable CDR loops present in the variable domain. The high specificity of these interactions makes the development of antibodies an important consideration in fields like therapeutics and vaccine development.
The high-resolution structure development of antibody-antigen complexes has served to significantly enhance our understanding of immunity and its underlying mechanisms, allowing for the design of immunogens and antibodies. However, experimental methods for structure determination pose many challenges and constraints on resources and time, often making it infeasible to obtain experimentally derived characterizations for most complexes. In order to fill this need, an array of computational tools have been created – general methods for protein-protein docking have been utilized but haven’t been very successful due to their failure to consider the mobile nature of important CDR loops. Hence, tools have been developed specifically to model antibody-antigen complexes, but obtaining accurate predictions remains challenging.
Continue Reading
26 notes
·
View notes
Text
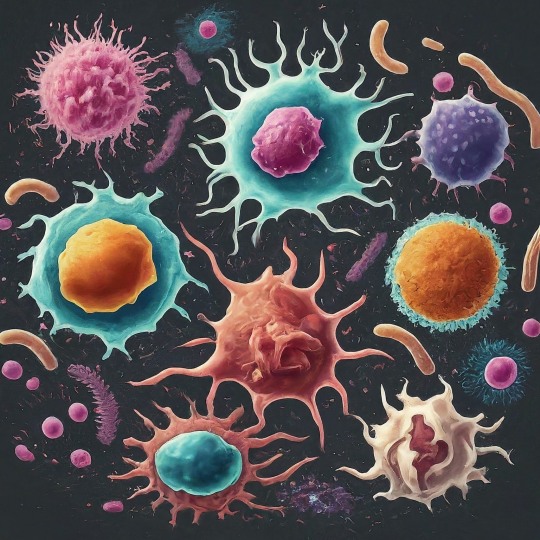
The Immune System's All-Star Team: The Mighty Cells That Protect You
Your body is like a bustling city, constantly facing threats from outside invaders like viruses and bacteria. Thankfully, you have a team of dedicated defenders keeping you safe: your immune cells! Our immune system is a marvel of biological defense, tirelessly safeguarding our bodies from harmful invaders like bacteria, viruses, and parasites. At the forefront of this defense are numerous types of immune cells, each with its unique functions and capabilities. Did you know the average adult has about 2 trillion white blood cells, which contain most immune cells? That's more people than live in China! These tiny warriors come in different shapes and sizes, each with unique superpowers to protect you. Let's meet some of the key players:
The Innate Force: First up, we have the innate immune system. This frontline defense acts fast and nonspecifically, providing immediate protection against any threat. The key players: 1. Neutrophils: Think of these guys as the city's SWAT team. They're the first responders, rushing to attack invaders with toxic chemicals and swallowing them whole with their arsenal of enzymes! These are the most abundant immune cells, are short-lived but highly effective. Unfortunately, they die in the fight, leaving behind a green gooey mess (pus) that signals infection.
2. Macrophages: These are the veterans, the wise generals of the immune system. They go beyond mere engulfing, processing antigens (foreign molecules) and presenting them to other immune cells for recognition and attack. They also act as scavengers, cleaning up debris and orchestrating healing. These are the cleaners and recyclers. They gobble up dead neutrophils, debris, and even worn-out cells, keeping your city sparkling clean.
3. Natural Killer (NK) Cells: These are the ninjas of the immune system. They silently patrol, sniffing out suspicious cells infected with viruses or even cancer and eliminating them with a swift punch. The Adaptive Arsenal:
The Adaptive Arsenal: If the innate system fails, the adaptive immune system steps in. This highly specific defense remembers past encounters and tailors its response to each unique threat. The cells of adaptive immune system are:
B Cells: These are the antibody factories, producing highly specific proteins called antibodies that neutralize pathogens and toxins. Each B cell produces a unique antibody, like a lock and key, targeting specific invaders. They whip up special proteins called antibodies that lock onto specific invaders, like sticky notes, marking them for destruction.
T Cells: These are the generals, coordinating the entire defense. There are different types of T cells:
Helper T Cells: These are the commanders, directing and coordinating the immune response through chemical signals. They activate B cells, macrophages, and other immune cells, orchestrating a multi-pronged attack.
Cytotoxic T Cells: These are the elite soldiers, directly targeting and eliminating infected cells or cancer cells. They recognize and bind to specific enemy markers, unleashing a lethal attack.
Memory T Cells: These are the veterans, remembering past encounters with invaders and helping the immune system respond faster next time.
The Unsung Heroes: Beyond these main players, numerous other immune cells contribute to our defense. These include:
Dendritic cells: These antigen-presenting cells capture and process pathogens, presenting their fragments to T cells for activation. They're like the scouts, gathering enemy intel and relaying it to the command center.
Mast cells: These cells reside in tissues and release inflammatory chemicals in response to allergens or parasites. They're like the alarm system, alerting the immune system to local threats.
Eosinophils: These specialize in fighting parasitic infections, releasing toxic chemicals to neutralize them.
Basophils: These are involved in allergic reactions and contribute to wound healing.
The beauty of the immune system lies in its intricate collaboration. These diverse cell types work together in a complex and beautiful dance, each playing a specific role to achieve a common goal: protecting our health. They communicate extensively through chemical signals, creating a complex network of interactions. Imagine B cells producing antibodies that bind to a pathogen, flagging it for destruction. Macrophages engulf and eliminate the tagged pathogen, while T cells coordinate the attack and eliminate any infected cells. Dendritic cells present captured fragments to T cells, priming them for future encounters. This seamless cooperation ensures a swift and effective response to any threat.
Your immune system is constantly learning. Each time you get a vaccine or fight off an infection, your immune cells create memory T cells, making you more resistant to future attacks. Understanding these cellular heroes can help us appreciate the incredible machinery that keeps us healthy and appreciate the importance of maintaining a strong immune system. It also allows us to make informed decisions about supporting our immune system. Maintaining a healthy lifestyle, getting adequate sleep, managing stress, and consuming a balanced diet can all contribute to a robust immune response.
#science sculpt#life science#science#biology#biotechnology#artists on tumblr#immunology#immunotherapy#immune cells#b cells#t cells#neutrophil#macrophages#immunity#health and wellness#medicine#healthcare#illustration#illustrative art
9 notes
·
View notes
Text
Gather Round
The immune system's B cells recognise their foe (antigens) with receptors (also known as immunoglobulin or antibody) on their cell surface. Here, super resolution microscopy combined with 4D image analysis reveals how these receptors on the cell surface localise into clusters as ridges and finger-like projections called microvilli to aid recognition of their targets
Read the published research paper here
Video adapted from work by Deniz Saltukoglu and colleagues
Department of Molecular Immunology, Biology III, Faculty of Biology, University of Freiburg, Freiburg, Germany
Video originally published with a Creative Commons Attribution 4.0 International (CC BY 4.0)
Published in The EMBO Journal, January 2023
You can also follow BPoD on Instagram, Twitter and Facebook
#science#biomedicine#biology#immunology#immunoglobulin#B cells#immune system#antibody#antigen#lattice light-sheet microscopy#super resolution microscopy#4D imaging
11 notes
·
View notes
Text
Virus latency (or viral latency) is the ability of a pathogenic virus to lie dormant (latent) within a cell, denoted as the lysogenic part of the viral life cycle.[1] A latent viral infection is a type of persistent viral infection which is distinguished from a chronic viral infection. Latency is the phase in certain viruses' life cycles in which, after initial infection, proliferation of virus particles ceases. However, the viral genome is not eradicated. The virus can reactivate and begin producing large amounts of viral progeny (the lytic part of the viral life cycle) without the host becoming reinfected by new outside virus, and stays within the host indefinitely.[2]
Episomal latency refers to the use of genetic episomes during latency. In this latency type, viral genes are stabilized, floating in the cytoplasm or nucleus as distinct objects, either as linear or lariat structures. Episomal latency is more vulnerable to ribozymes or host foreign gene degradation than proviral latency (see below).
Advantages of episomal latency include the fact that the virus may not need to enter the cell nucleus, and hence may avoid nuclear domain 10 (ND10) from activating interferon via that pathway. Disadvantages include more exposure to cellular defenses, leading to possible degradation of viral gene via cellular enzymes.[12]
Proviral latency: A provirus is a virus genome that is integrated into the DNA of a host cell
All interferons share several common effects: they are antiviral agents and they modulate functions of the immune system. Administration of Type I IFN has been shown experimentally to inhibit tumor growth in animals, but the beneficial action in human tumors has not been widely documented. A virus-infected cell releases viral particles that can infect nearby cells. However, the infected cell can protect neighboring cells against a potential infection of the virus by releasing interferons. In response to interferon, cells produce large amounts of an enzyme known as protein kinase R (PKR). This enzyme phosphorylates a protein known as eIF-2 in response to new viral infections; the phosphorylated eIF-2 forms an inactive complex with another protein, called eIF2B, to reduce protein synthesis within the cell. Another cellular enzyme, RNAse L—also induced by interferon action—destroys RNA within the cells to further reduce protein synthesis of both viral and host genes. Inhibited protein synthesis impairs both virus replication and infected host cells. In addition, interferons induce production of hundreds of other proteins—known collectively as interferon-stimulated genes (ISGs)—that have roles in combating viruses and other actions produced by interferon.[13][14] They also limit viral spread by increasing p53 activity, which kills virus-infected cells by promoting apoptosis.[15][16] The effect of IFN on p53 is also linked to its protective role against certain cancers.[15]
Another function of interferons is to up-regulate major histocompatibility complex molecules, MHC I and MHC II, and increase immunoproteasome activity. All interferons significantly enhance the presentation of MHC I dependent antigens. Interferon gamma (IFN-gamma) also significantly stimulates the MHC II-dependent presentation of antigens. Higher MHC I expression increases presentation of viral and abnormal peptides from cancer cells to cytotoxic T cells, while the immunoproteasome processes these peptides for loading onto the MHC I molecule, thereby increasing the recognition and killing of infected or malignant cells. Higher MHC II expression increases presentation of these peptides to helper T cells; these cells release cytokines (such as more interferons and interleukins, among others) that signal to and co-ordinate the activity of other immune cells.[17][18][19]
Epstein–Barr virus lytic reactivation (which can be due to chemotherapy or radiation) can result in genome instability and cancer.[5]
HSV reactivates upon even minor chromatin loosening with stress,[7] although the chromatin compacts (becomes latent) upon oxygen and nutrient deprivation.[8]
Cytomegalovirus (CMV) establishes latency in myeloid progenitor cells, and is reactivated by inflammation.[9] Immunosuppression and critical illness (sepsis in particular) often results in CMV reactivation.[10] CMV reactivation is commonly seen in patients with severe colitis.[11]
viral latency is so fucked up
11 notes
·
View notes
Text
Mitochondrial Dysfunction in Autoimmune Disorders

Mitochondria, the energy-producing organelles of eukaryotic cells, are involved in a myriad of cellular functions beyond ATP production, including calcium homeostasis, reactive oxygen species (ROS) generation, apoptosis regulation, and the modulation of immune responses. In autoimmune diseases, mitochondrial dysfunction is increasingly recognized as a key factor in disease pathogenesis. These disorders, characterized by the immune system's aberrant recognition and attack on self-antigens, often manifest in conditions such as systemic lupus erythematosus (SLE), rheumatoid arthritis (RA), and multiple sclerosis (MS). This article delves into the mechanisms linking mitochondrial dysfunction to autoimmune disorders, highlighting cellular changes, signaling pathways, and potential therapeutic approaches.
Mitochondrial Function in Immune Cells
Mitochondria are critical for cellular energy metabolism, primarily through oxidative phosphorylation, which occurs in the inner mitochondrial membrane and generates ATP. However, their roles extend far beyond energy production. Mitochondria are involved in immune cell activation, differentiation, and the regulation of apoptosis. Immune cells, including T lymphocytes, B lymphocytes, dendritic cells, and macrophages, rely on mitochondrial function for their metabolic demands during immune responses. These cells undergo rapid changes in mitochondrial dynamics and energy production during immune activation.
Mitochondria also produce ROS as byproducts of oxidative phosphorylation, and these ROS, under normal conditions, function as signaling molecules that regulate immune responses. However, excessive ROS generation, stemming from mitochondrial dysfunction, can lead to oxidative stress, damage to cellular components, and dysregulated immune responses that are characteristic of autoimmune diseases.
Mitochondrial Dysfunction and Autoimmune Pathogenesis
In autoimmune diseases, mitochondrial dysfunction contributes to disease progression through multiple interconnected mechanisms, including dysregulated immune responses, enhanced oxidative stress, impaired mitochondrial dynamics, and defective apoptosis. The following section outlines key mechanisms linking mitochondrial dysfunction to autoimmune pathogenesis.
1. Oxidative Stress and Immune Dysregulation
Mitochondria are a major source of ROS, which are produced as intermediates during oxidative phosphorylation in the electron transport chain (ETC). Under normal conditions, ROS are tightly regulated by antioxidant systems, including superoxide dismutase (SOD), catalase, and glutathione peroxidase. However, in autoimmune diseases, mitochondrial dysfunction often results in increased ROS production, overwhelming the antioxidant capacity of the cell and resulting in oxidative stress.
Oxidative stress can damage cellular macromolecules such as lipids, proteins, and nucleic acids. In immune cells, excessive ROS lead to the activation of several signaling pathways, including the nuclear factor-kappa B (NF-κB) pathway, which promotes the expression of pro-inflammatory cytokines such as TNF-α, IL-6, and IL-1β. In systemic lupus erythematosus (SLE), rheumatoid arthritis (RA), and multiple sclerosis (MS), ROS-induced activation of these inflammatory pathways amplifies the autoimmune response, driving tissue damage and inflammation.
Moreover, oxidative stress exacerbates the production of autoantibodies, particularly in diseases like SLE, where ROS contribute to the formation of immune complexes that target self-antigens, including nuclear components like double-stranded DNA (dsDNA) and mitochondrial antigens.
2. Mitochondrial Dynamics in Autoimmune Diseases
Mitochondrial dynamics, encompassing processes such as mitochondrial fission, fusion, and mitophagy, are critical for maintaining mitochondrial function and cellular homeostasis. Mitochondrial fission, mediated by proteins such as dynamin-related protein 1 (Drp1), leads to the division of mitochondria, while fusion, regulated by mitofusins (Mfn1/2) and optic atrophy 1 (OPA1), facilitates the merging of mitochondria to restore mitochondrial function and DNA integrity. Mitochondrial fusion is crucial for maintaining the respiratory capacity and metabolic flexibility of immune cells.
In autoimmune disorders, an imbalance in mitochondrial dynamics is often observed. For example, in rheumatoid arthritis (RA), T cells exhibit excessive mitochondrial fission and impaired fusion, leading to mitochondrial fragmentation, reduced mitochondrial membrane potential, and diminished oxidative phosphorylation capacity. This dysfunction contributes to the failure of immune cells to produce sufficient ATP during activation, resulting in altered cytokine production and a hyperactive immune response.
Similarly, in multiple sclerosis (MS), aberrant mitochondrial dynamics in oligodendrocytes and T cells contribute to neuroinflammation and demyelination. The dysregulated fission and fusion processes impair mitochondrial function, leading to increased oxidative stress, mitochondrial damage, and exacerbation of inflammatory cascades that damage myelin.
3. Mitochondrial-Derived Damage-Associated Molecular Patterns (DAMPs)
Mitochondria are also sources of damage-associated molecular patterns (DAMPs), which are intracellular molecules released upon cellular injury or stress. DAMPs, such as mitochondrial DNA (mtDNA), cardiolipin, and mitochondrial N-formyl peptides, serve as danger signals recognized by pattern recognition receptors (PRRs) on immune cells. These include toll-like receptors (TLRs), particularly TLR9, which recognizes mtDNA, and TLR2/4, which are involved in the recognition of mitochondrial lipids.
In autoimmune diseases, mitochondrial dysfunction leads to the release of mtDNA and other mitochondrial components into the extracellular space, where they act as endogenous ligands for PRRs. The activation of these receptors triggers inflammatory responses, including the activation of NF-κB, interferon pathways, and the inflammasome, all of which amplify immune responses and contribute to chronic inflammation and tissue damage.
In SLE, circulating mtDNA fragments have been detected in the serum, correlating with disease activity. The presence of mtDNA in the bloodstream is thought to exacerbate the activation of autoreactive immune cells and the production of autoantibodies, particularly those targeting nuclear antigens.
4. Impaired Apoptosis and Autoimmunity
Mitochondria are key regulators of apoptosis, particularly the intrinsic or mitochondrial pathway of cell death. The mitochondrial pathway is initiated by the release of pro-apoptotic factors such as cytochrome c and apoptosis-inducing factor (AIF) from the mitochondrial intermembrane space into the cytosol. This release activates caspases, leading to cell death. In autoimmune diseases, mitochondrial dysfunction often leads to defective apoptosis, which prevents the elimination of autoreactive immune cells.
In diseases like systemic lupus erythematosus (SLE), defective apoptosis of T cells and B cells contributes to the survival of autoreactive lymphocytes that would normally be eliminated through programmed cell death. The persistence of these cells results in the production of autoantibodies, including anti-dsDNA and anti-mitochondrial antibodies, which target self-tissues and exacerbate inflammation.
Moreover, impaired mitophagy, the process by which damaged mitochondria are selectively degraded by autophagy, further contributes to the accumulation of dysfunctional mitochondria, promoting sustained oxidative stress and inflammation.
Mitochondrial Dysfunction in Specific Autoimmune Disorders
1. Systemic Lupus Erythematosus (SLE)
In SLE, mitochondrial dysfunction plays a pivotal role in disease pathogenesis. Increased ROS production, defective mitophagy, and impaired apoptosis contribute to the activation of autoreactive T cells and B cells, leading to the production of autoantibodies. The release of mitochondrial DAMPs, such as mtDNA, amplifies the inflammatory response, and mitochondrial damage is directly linked to the severity of disease activity in SLE.
2. Rheumatoid Arthritis (RA)
In RA, mitochondrial dysfunction in immune cells, particularly in synovial T cells and macrophages, is associated with increased mitochondrial fragmentation, oxidative stress, and dysregulated immune activation. ROS and mitochondrial-derived DAMPs contribute to the chronic inflammatory environment in the synovium, driving the production of pro-inflammatory cytokines and promoting joint destruction.
3. Multiple Sclerosis (MS)
Mitochondrial dysfunction in MS primarily affects oligodendrocytes, the myelinating cells in the central nervous system. Impaired mitochondrial function in these cells contributes to neuroinflammation and demyelination. Additionally, T cells with dysfunctional mitochondria play a role in the perpetuation of inflammation, leading to the destruction of myelin and axonal injury in the CNS.
Therapeutic Implications
Targeting mitochondrial dysfunction presents a promising therapeutic strategy for autoimmune diseases. Potential approaches include:
Mitochondrial Antioxidants: Compounds like MitoQ, MitoTEMPO, and other mitochondrial-targeted antioxidants can help reduce oxidative stress, protect mitochondrial function, and alleviate inflammation.
Modulating Mitochondrial Dynamics: Agents that promote mitochondrial fusion or inhibit excessive fission, such as Mfn2 activators, could restore mitochondrial integrity in immune cells, reducing inflammation and improving immune regulation.
Enhancing Mitophagy: Stimulating mitophagy through compounds such as spermidine or activation of the PINK1/PARK2 pathway may help remove damaged mitochondria and reduce the accumulation of mitochondrial DAMPs.
Targeting Mitochondrial DAMPs: Inhibiting the release of mtDNA and other mitochondrial DAMPs using TLR inhibitors or blocking downstream signaling pathways may reduce the inflammatory response in autoimmune diseases.
Conclusion
Mitochondrial dysfunction is a key factor in the pathogenesis of autoimmune diseases. Through mechanisms such as increased oxidative stress, impaired mitochondrial dynamics, and dysregulated apoptosis, mitochondrial dysfunction exacerbates immune activation and inflammation. The accumulation of mitochondrial-derived DAMPs further amplifies the autoimmune response. Targeting mitochondrial health offers a promising therapeutic strategy to mitigate the effects of mitochondrial dysfunction and improve disease outcomes in autoimmune disorders.
#Mitochondrial dysfunction#Autoimmune disorders#Oxidative stress#Reactive oxygen species (ROS)#Mitochondrial dynamics#Mitochondrial fission#Mitochondrial fusion#Mitophagy#Mitochondrial DNA (mtDNA)
0 notes
Text
Biologic Response–Modifying and Antirheumatic Drugs
Anatomy, Physiology, and Pathophysiology Overview
Overview of Immunomodulators
Cancer treatment has traditionally involved surgery, radiation, and chemotherapy.
Surgery and radiation are usually local or regional therapies. Chemotherapy is generally systemic, but it often does not completely eliminate all of the cancer cells in the body. Adjuvant therapy is frequently used to destroy undetected distant micrometastases.
The two major components of the body’s immune system are humoral immunity, mediated by B-cell functions (primarily antibody production), and cell-mediated immunity, which is mediated by T-cell functions. The humoral and cellular immune systems act together to recognize and destroy foreign particles and cells in the blood or other body tissues. The humoral immune system is composed of lymphocytes that are known as B cells until they are transformed into plasma cells when they come in contact with an antigen (foreign substance). The plasma cells then manufacture antibodies to that antigen.
Over the last two decades, medical technology has developed a group of drugs impacting the immune system, and a new addition to these drugs includes the biologic response-modifying drugs or biologic response modifiers (BRMs).
These drugs alter the body’s response to diseases such as cancer and autoimmune, inflammatory, and infectious diseases and can enhance or restrict the patient’s immune response to disease, can stimulate a patient’s hematopoietic function, and prevent disease.
Biologic response–modifying drugs provide another treatment option for patients who have malignancies and/or are receiving chemotherapy and need to boost blood cell counts.
Two broad classes of biologic response–modifying drugs are hematopoietic drugs and immunomodulating drugs. Subclasses of immunomodulating drugs include interferons, monoclonal antibodies, interleukin receptor agonists and antagonists, and miscellaneous drugs. Disease-modifying antirheumatic drugs are drugs used to treat rheumatoid arthritis.
Immunomodulating drugs therapeutically alter a patient’s immune response.
An intact immune system can identify cells as malignant and destroy them; a healthy immune system can distinguish between tumor cells and normal body tissues.
People develop cancerous cells in their bodies on a regular basis; normally the immune system is able to eliminate these cells before they multiply to uncontrollable levels. Only when the natural immune responses fail to keep pace with these initially microscopic cancer cell growths does a person develop a true “cancer” requiring clinical intervention.
Biologic response–modifying drugs work by one of three mechanisms: (1) enhancement or restoration of the host’s immune system defenses against the tumor; (2) direct toxic effect on the tumor cells, which causes them to lyse, or rupture; or (3) adverse modification of the tumor’s biology, which makes it harder for the tumor cells to survive and reproduce.
Some immunomodulating drugs are used to treat autoimmune, inflammatory, and infectious diseases. They either reduce the patient’s inappropriate immune response (as in rheumatoid arthritis) or strengthen the immune response against microorganisms and cancer cells.
https://bb-csuohio.blackboard.com/bbcswebdav/pid-7116323-dt-content-rid-80385717_1/xid-80385717_1
Immune System
Tumor cells express chemical compounds on their surfaces that signal the immune system that these cells are a threat. These chemical markers are called tumor antigens or markers.
An antigen is any substance that the body’s immune system recognizes as foreign. Recognition of antigens varies among individuals, which is why some people are more prone than others to immune-related diseases such as allergies, inflammatory diseases, and cancer.
Humoral immunity is mediated by B-cell functions (primarily antibody production), and cell-mediated immunity is mediated by T-cell functions.
Attack against tumor cells by antibodies produced by the B lymphocytes of the humoral immune system prepares those tumor cells for destruction by the T lymphocytes.
The immune system in a healthy individual is genetically preprogrammed to be able to mount an antibody response against literally millions of different antigens resulting from the individual’s lifetime antigen exposure and through exposure to new antigens passed down.
Antibodies that a single plasma cell makes are all identical and are called monoclonal.
Monoclonal antibodies have also been prepared synthetically using recombinant DNA technology, which has resulted in newer drug therapies.
When B lymphocytes differentiate into plasma cells, some of these B cells become memory cells that “remember” the exact characteristics of a particular foreign antigen, allowing a stronger and faster immune response in re-exposure to the same antigen.
The functional cells of the cell-mediated immune system are the T lymphocytes, which mature in the thymus. T cells include cytotoxic T cells, T helper cells, and T suppressor cells.
Cytotoxic T cells directly kill their targets by causing cell lysis or rupture.
T helper cells are considered the master controllers of the immune system. They direct the actions of many other immune components, such as lymphokines and cytotoxic T cells.
Cytokines are non-antibody proteins serving as chemical mediators of physiologic functions.
Lymphokines are a subset of cytokines released by T lymphocytes upon contact with antigens; they serve as chemical mediators of the immune response.
T suppressor cells have an effect on the immune system that is opposite to that of T helper cells and serve to limit or control the immune response. A healthy immune system has about twice as many T helper cells as T suppressor cells at any given time.
The cancer-killing cells of the cellular immune system are the macrophages (derived from monocytes), natural killer (NK) cells (another type of lymphocyte), and polymorphonuclear leukocytes (not lymphocytes), which are also called neutrophils.
In contrast, T suppressor cells have an important negative influence on antitumor actions of the immune system. Overactive T suppressor cells may be responsible for clinically significant cancer cases by permitting tumor growth beyond the immune system’s control.
https://bb-csuohio.blackboard.com/bbcswebdav/pid-7116323-dt-content-rid-80385710_1/xid-80385710_1
Pharmacology Overview
Therapeutic effects of the biologic response-modifying drugs include enhancement of hematopoietic function, regulation or enhancement of the immune response, inhibition of metastases, prevention of cell division, or inhibition of cell maturation.
Fingolimod (Gilenya) is a newer oral drug for the treatment of relapsing forms of MS to reduce the frequency of clinical exacerbations and delay disability progression. It is a sphingosine 1-phosphate receptor modulator.
Hematopoietic Drugs
Erythropoietic drugs (epoetin alfa and darbepoetin alfa), three colony-stimulating factors (filgrastim, pegfilgrastim, and sargramostim) promote the synthesis of various types of major blood components by promoting the growth, differentiation, and function of their corresponding precursor cells in the bone marrow.
All hematopoietic drugs decrease the duration of chemotherapy-induced anemia, neutropenia, and thrombocytopenia and allow higher dosages of chemotherapy to be given; decrease bone marrow recovery time after bone marrow transplantation or irradiation; and stimulate other cells in the immune system to destroy or inhibit the growth of cancer cells, as well as virus- or fungus-infected cells.
All of these drugs are produced by recombinant DNA technology, which allows them to be essentially identical to their endogenously produced counterparts.
Neutrophils are the most important granulocytes for fighting infection. Colony-stimulating factors stimulate neutrophils to grow and mature and thus directly oppose the detrimental bone marrow actions of chemotherapy.
Colony-stimulating factors also enhance the functioning of mature cells of the immune system, such as macrophages and granulocytes. This increases the ability of the body’s immune system to kill cancer cells, as well as virus- and fungus-infected cells.
The effect of hematopoietic drugs on the bone marrow cells also reduces the recovery time after bone marrow transplantation and radiation therapy.
Filgrastim and sargramostim have significant drug interactions when these two drugs are given with myelosuppressive antineoplastic drugs. When myelosuppressive antineoplastics are given with them, the drugs directly antagonize each other. Filgrastim and sargramostim are not given within 24 hours of administration of myelosuppressive antineoplastics.
https://bb-csuohio.blackboard.com/bbcswebdav/pid-7116323-dt-content-rid-80385711_1/xid-80385711_1
Interferons
Interferons are proteins with three basic properties: antiviral, antitumor, and immunomodulating. There are three different groups of interferon drugs—the alpha, beta, and gamma interferons—each with its own antigenic and biological activity.
Interferons are most commonly used in the treatment of certain viral infections and types of cancer.
Interferons are recombinantly manufactured substances that are identical to the interferon cytokines that are naturally present in the human body.
Overall, interferons have three different effects on the immune system. They can (1) restore its function if it is impaired, (2) augment (amplify) the immune system’s ability to function as the body’s defense, and (3) inhibit the immune system, useful when the immune system has become dysfunctional, causing autoimmune disease such as multiple sclerosis.
The beneficial actions of interferons make them excellent drugs for the treatment of viral infections, various cancers, and some autoimmune disorders.
The most commonly used interferon products are in the alfa class and are referred to as leukocyte interferons because they are produced from human leukocytes. Two newer types of interferon alfa include peginterferon alfa-2a and peginterferon alfa-2b.
Contraindications to the use of interferons include known drug allergy and may include autoimmune disorders, hepatitis or liver failure, concurrent use of immunosuppressant drugs, Kaposi’s sarcoma related to AIDS, and severe liver disease.
The most common adverse effects can be broadly described as flulike symptoms: fever, chills, headache, malaise, myalgia, and fatigue.
The major dose-limiting adverse effect of interferons is fatigue.
https://bb-csuohio.blackboard.com/bbcswebdav/pid-7116323-dt-content-rid-80385712_1/xid-80385712_1
Monoclonal Antibodies
Monoclonal antibodies are becoming the standard of therapy in many areas, including the treatment of cancer, rheumatoid arthritis and other inflammatory diseases, multiple sclerosis, and organ transplantation. They can specifically target cancer cells with minimal effect on healthy cells.
The mab suffix in a drug name is usually an abbreviation for “monoclonal antibody.”
The FDA recently approved biosimilar products to infliximab (Inflectra), etanercept (Ereizi), and adalimumab (Amjevita).
Vedolizumab (Entyvio) was approved in 2014 for the treatment of Crohn’s disease and ulcerative colitis. The most common side effects are nausea, nasopharyngitis, upper respiratory tract infection, arthralgia, pyrexia, fatigue, headache, cough, and infusion-related reactions.
Patients receiving these very potent drugs can manifest acute symptoms that are comparable to classic allergy or flulike symptoms, such as fever, dyspnea, and chills.
https://bb-csuohio.blackboard.com/bbcswebdav/pid-7116323-dt-content-rid-80385713_1/xid-80385713_1
Interleukins and Related Drugs
Interleukins are a natural part of the immune system and are classified as lymphokines.
Lymphokines are soluble proteins that are released from activated lymphocytes such as natural killer cells.
Interleukins cause multiple effects on the immune system, one of which is antitumor action.
Aldesleukin was previously indicated only for the treatment of metastatic renal cell carcinoma. It is now also approved for the treatment of metastatic melanoma.
Denileukin diftitox is currently indicated only as therapy for a skin-based lymphoma known as cutaneous T-cell lymphoma, which often metastasizes to other areas of the body.
Anakinra and tocilizumab are indicated for symptom control in patients with rheumatoid arthritis for whom other therapy has failed.
Therapy with aldesleukin is commonly complicated by severe toxicity. A syndrome known as capillary leak syndrome is responsible for the severe toxicities of aldesleukin.
Common adverse effects associated with denileukin diftitox administration are GI upset, hypoalbuminemia, elevated liver enzyme levels, edema, dyspnea, cough, fever, chills, asthenia, generalized pain, chest pain, infection, and headache.
Anakinra has a much milder adverse effect profile that includes local reactions at the injection site, various respiratory tract infections, and headaches.
Tocilizumab has a high risk of causing anaphylaxis.
The most common side effects of ixekizumab are neutropenia, immunogenicity, infection, and injection site reactions.
Some additional medications can be broadly classified as miscellaneous immunomodulating drugs. A special term for immunostimulant drugs that are nonspecific is adjuvant.
https://bb-csuohio.blackboard.com/bbcswebdav/pid-7116323-dt-content-rid-80385714_1/xid-80385714_1
Pathophysiology Overview
Rheumatoid Arthritis
Rheumatism is a general term for any of several disorders characterized by inflammation, degeneration, or metabolic derangement of connective tissue structures, especially joints and related structures such as muscles, tendons, bursae, fibrous tissue, and ligaments.
Rheumatoid arthritis is a chronic autoimmune disorder that commonly causes inflammation and tissue damage in joints. It can also cause anemia and diffuse inflammation in the lungs, eyes, and pericardium of the heart, and subcutaneous nodules under the skin.
Disease-modifying antirheumatic drugs (DMARDs) not only provide antiinflammatory and analgesic effects, but they can arrest or slow the disease processes associated with arthritis.
They exhibit anti-inflammatory, antiarthritic, and immunomodulating effects and work by inhibiting the movement of various cells into an inflamed, damaged area, such as a joint.
DMARDs often have a slow onset of action of several weeks versus minutes to hours for NSAIDs; sometimes these drugs are also referred to as slow-acting antirheumatic drugs (SAARDs).
They were previously thought of as second-line drugs for the treatment of arthritis because they can have much more toxic adverse effects than do the NSAIDs. The American College of Rheumatology updated its treatment guidelines in 2012 and again in 2015, and now recommends the use of DMARDs as first-line therapy in many patients.
Nonbiologic DMARDs include methotrexate, leflunomide, hydroxychloroquine, and sulfasalazine.
The biologic DMARDs include adalimumab, anakinra, certolizumab, etanercept, golimumab, infliximab, adalimumab, abatacept, rituximab, tocilizumab and, most recently, tofacitinib.
Tofacitinib (Xeljanz) is a drug of the Janus kinase inhibitor (JAK) class for adult patients with moderately to severely active RA with an inadequate response or intolerance to methotrexate. However, serious infections including TB and other infections are concerns with the use of the drug and the patient needs to know the signs and symptoms to report to the prescriber. Similar drugs include baricitinib (Olumiant), fedratinib (Inrebic), ruxolitinib (Jakafi) and upadacitinib (Rinvoq). This class of drugs is given orally.
DMARDs are not used in patients with active bacterial infection, active herpes zoster, active or latent tuberculosis, or acute or chronic hepatitis B or C.
Etanercept, infliximab, and adalimumab are not to be used in patients with heart failure, lymphoma, or multiple sclerosis.
Methotrexate and leflunomide are to be avoided during pregnancy and lactation.
The recommended therapy with nonbiologic DMARDs usually begins with methotrexate or leflunomide for most patients.
Biologic DMARDs are generally reserved for those patients whose disease does not respond to methotrexate or leflunomide. The biologic DMARDs include etanercept, infliximab, adalimumab, abatacept, and rituximab.
https://bb-csuohio.blackboard.com/bbcswebdav/pid-7116323-dt-content-rid-80385715_1/xid-80385715_1
Pathophysiology Overview
Rheumatoid Arthritis
Rheumatism is a general term for any of several disorders characterized by inflammation, degeneration, or metabolic derangement of connective tissue structures, especially joints and related structures such as muscles, tendons, bursae, fibrous tissue, and ligaments.
Rheumatoid arthritis is a chronic autoimmune disorder that commonly causes inflammation and tissue damage in joints. It can also cause anemia and diffuse inflammation in the lungs, eyes, pericardium of the heart, and subcutaneous nodules under the skin.
Disease-modifying antirheumatic drugs (DMARDs) not only provide anti-inflammatory and analgesic effects, but they can arrest or slow the disease processes associated with arthritis.
They exhibit anti-inflammatory, antiarthritic, and immunomodulating effects and work by inhibiting the movement of various cells into an inflamed, damaged area, such as a joint.
DMARDs often have a slow onset of action of several weeks versus minutes to hours for NSAIDs; sometimes these drugs are also referred to as slow-acting antirheumatic drugs (SAARDs).
They were previously thought of as second-line drugs for the treatment of arthritis because they can have much more toxic adverse effects than NSAIDs. The American College of Rheumatology updated its treatment guidelines in 2012 and again in 2015, and now recommends the use of DMARDs as first-line therapy in many patients.
Nonbiologic DMARDs include methotrexate, leflunomide, hydroxychloroquine, and sulfasalazine.
The biologic DMARDs include adalimumab, anakinra, certolizumab, etanercept, golimumab, infliximab, adalimumab, abatacept, rituximab, tocilizumab and, most recently, tofacitinib.
Tofacitinib (Xeljanz) is a drug of the Janus kinase inhibitor (JAK) class for adult patients with moderately to severely active RA with an inadequate response or intolerance to methotrexate. However, serious infections including TB and other infections are concerns with the use of the drug and the patient needs to know the signs and symptoms to report to the prescriber. Similar drugs include baricitinib (Olumiant), fedratinib (Inrebic), ruxolitinib (Jakafi), and upadacitinib (Rinvoq). This class of drugs is given orally.
DMARDs are not used in patients with active bacterial infection, active herpes zoster, active or latent tuberculosis, or acute or chronic hepatitis B or C.
Etanercept, infliximab, and adalimumab are not to be used in patients with heart failure, lymphoma, or multiple sclerosis.
Methotrexate and leflunomide are to be avoided during pregnancy and lactation.
The recommended therapy with nonbiologic DMARDs usually begins with methotrexate or leflunomide for most patients.
Biologic DMARDs are generally reserved for those patients whose disease does not respond to methotrexate or leflunomide. The biologic DMARDs include etanercept, infliximab, adalimumab, abatacept, and rituximab.
https://bb-csuohio.blackboard.com/bbcswebdav/pid-7116323-dt-content-rid-80385716_1/xid-80385716_1
Nursing Process
Nursing management associated with the administration of biological response–modifying drugs focuses on the use of careful aseptic technique and other measures to prevent infection; proper nutrition; oral hygiene; monitoring of blood counts; and management of adverse effects, including joint and bone pain and flulike symptoms.
Before administering these medications, document a baseline assessment of the following: vital signs, skin turgor and intactness, bowel sounds and patterns, and breath sounds.
Assess potential intravenous and subcutaneous sites, and, if appropriate, note chemotherapy-induced absolute neutrophil nadir (low point).
With the use of filgrastim, assess for any existing joint or bone pain because of the possible adverse effects of mild to severe bone pain with filgrastim.
Do not administer filgrastim and sargramostim within 24 hours of a myelosuppressive antineoplastic, and follow the time frame for their use (as prescribed), whether in an inpatient or home setting.
With biological response-modifying drugs, assess the patient for the presence of any conditions that represent contraindications or cautions to their administration.
Assess the following systems: (1) respiratory system with attention to rate, rhythm, and depth as well as breath sounds, listening for any adventitious (abnormal) sounds; (2) cardiac system with attention to vital signs, heart sounds, heart rate and rhythm, and oxygen saturation levels, as well as assessment for edema and/or shortness of breath, presence of cyanotic discoloration around the mouth or nail beds, and any chest pain; (3) central nervous system with a focus on baseline mental status, as well as assessment for any seizure-like activity or central nervous system abnormalities; and (4) immune system, noting any history of chronic illnesses, ability to fight off infections, and history of suppressed immunity.
Before interferons are given, assess the patient’s history of drug allergies as well as any history of autoimmune disorders, hepatitis, liver failure, or AIDS.
Monitor the patient for capillary leak syndrome (CLS) if they are taking interleukins. Identification of early symptoms will allow critical, supportive treatment with discontinuation of the interleukin drug. Assess for drug allergy as well as contraindications of organ transplantation and abnormal thallium cardiac stress testing results or abnormal pulmonary function tests.
The recommended therapy with nonbiologic DMARDs usually begins with methotrexate or leflunomide for most patients. Biologic DMARDs are generally reserved for those patients whose disease does not respond to methotrexate or leflunomide. The biologic DMARDs include etanercept, infliximab, adalimumab, abatacept, and rituximab. Assess for contraindications to the use of DMARDs, such as active bacterial infections, active herpes, active/latent tuberculosis, and acute or chronic hepatitis B or C.
Before initiation of therapy with leflunomide, perform a complete assessment of hepatic functioning as well as baseline blood cell counts. Because of the possible adverse effects of diarrhea and respiratory infections, assess GI functioning and bowel patterns.
Etanercept is to be avoided in those with sepsis and active infections, so conduct a thorough assessment of white blood cell (WBC) counts and for any signs and symptoms of infection, or a history of infection. Some dosage forms may contain latex; it is critical to also assess for latex allergy.
Premedication with acetaminophen and diphenhydramine may be deemed necessary to help minimize any allergic-type reaction when any of the biological response-modifying drugs are administered.
With some of the biological response-modifying drugs, treatment with opioids, other antihistamines, and/or anti-inflammatory drugs may be required for the management of bone pain and chills if treatment with acetaminophen or diphenhydramine is not successful.
Antiemetics may also be needed for any drug-related nausea or vomiting and may be given before the administration of the specific biological response-modifying drug.
With concerns of infection, as with many of the biologic response-modifying drugs, monitor the patient’s vital signs with attention to temperature and also for the occurrence of chills and headaches.
With monoclonals, serious infections are a major concern.
Therapeutic responses to biologic response–modifying drugs include a variety of responses, such as a decrease in the growth of the lesion or mass, decreased tumor size, and an easing of symptoms related to the tumor or disease process. Other therapeutic effects are an improvement in WBC, red blood cell (RBC), platelet counts, and/or a return of blood counts to normal levels, and absence of infection, anemias, and hemorrhage.
DMARDs are expected to have therapeutic results within a documented time frame (often weeks) with the patient experiencing increased ability to move joints, less discomfort, and an overall increased sense of improvement and well-being.
The toxicity of these drugs may be manifested by liver, renal, and respiratory dysfunction and, for methotrexate, bone marrow suppression.
0 notes
Text
The role of glycotransferases in research

Drug discovery and biotechnological innovations depend on a variety of tools and technologies to understand biological processes, disease progression, and drug development. One of the most prominent roles played in these fields are of Glycosyltransferases (GTs). These are enzymes that catalyze the transfer of sugar moieties from activated donor molecules to specific acceptor molecules, forming glycosidic bonds. This process is known as glycosylation, and it is one of the most crucial post-translational modifications in biological systems, impacting protein function, cellular communication, and immune responses.
This functional significance of GTs places them at the forefront of biochemical and biomedical research. In this field, they are used to elucidate mechanisms of disease, drug discovery, and biotechnological innovation.
The enzymatic activity of glycotransferases typically involves two substrates: a sugar donor (usually a nucleotide-sugar such as UDP-glucose) and an acceptor molecule (which can be a protein, lipid, or other sugar). During catalysis, GTs facilitate the transfer of the sugar moiety to the acceptor, forming a glycosidic bond that is essential for the structural integrity and function of the glycosylated product.
Biological functions of glycosyltransferases:
Cell-cell recognition and communication
Protein folding and stability
Metabolic pathways and energy storage
Pathogen interactions
Glycosyltransferases in disease research
Genetic disorders
Any disorders in glycosyltransferase genes can lead to congenital disorders of glycosylation (CDGs), a group of inherited metabolic disorders that affect multiple organ systems. Studies in this field enables the development of enzyme replacement therapies to restore normal glycosylation in affected individuals.
Cancer
Defects in glycosylation patterns can trigger many cancers, where changes in GT expression can affect cell adhesion, migration, and immune evasion.Tumor-associated carbohydrate antigens (TACAs) result from abnormal glycosyltransferase activity and alsoserve as potential biomarkers for early cancer detection, and as targets for immunotherapy.
Infectious diseases
Glycosyltransferases are also involved in pathogen-host interactions, such as those seen in viral infections.Targeting these enzymes has emerged as a strategy to reduce viral replication and spread.
Glycosyltransferases in drug discovery and biotechnology:
GT inhibitors have shown promise in cancer and infectious disease treatments.
Glycosyltransferases are valuable tools in synthetic biology, enabling the production of designer glycoproteins, glycopeptides, and oligosaccharides. These synthetic products are used for developing glycan-based vaccines, drug delivery systems, and therapeutic antibodies.
Glycosyltransferases are essential for developing glycan-based vaccines, particularly those targeting bacterial infections.
It is evident that Glycosyltransferases are central to many biological processes and have profound implications for health, disease, and biotechnology. Their roles in cellular communication, immune responses, and disease pathogenesis make them invaluable in research aimed at developing targeted therapies and biotechnological innovations.
0 notes
Text
MEN'S HEALTH AWARENESS MONTH
The 'Movember' campaign takes place every November, to raise awareness and funds for men's mental health, suicide prevention, prostate cancer and testicular cancer.
To mark the awareness month, experts from Fakeeh University Hospital share advice on what men in the UAE need to start doing to boost their health and live their best life
IT’S TIME TO ‘MAN UP’ AND TAKE CHARGE OF YOUR HEALTH
If you’re a man in your 30s or older, seeing a doctor or getting a checkup is most likely the last thing on your mind when you have a million other things to worry about. But, if recent data is anything to go by, it is the most critical thing you can do.
According to the latest figures from SEHA, men in the UAE are not doing enough to take charge of their health. 70 percent of Emirati men under the age of 30 years are known to suffer from obesity and diabetes[1]. Not enough men between 20 to 30 years of age are undergoing regular full physical work-ups, and screening for prevalent health issues.
In recognition of Men’s Health Month, the message is clear: men (young and old) need to take charge of their own health by proactively learning where their health is at and preemptively seeking treatment and guidance. To mark the occasion, experts across multiple specialties at Fakeeh University Hospital share their advice on the best place to start.
youtube
WHEN / WHY A UROLOGIST SHOULD BE PART OF YOUR LIFE
As men enter their 30s and later, quality-of-life issues become more pertinent, requiring early action to monitor and detect any urology issues. They may resist at first, especially if they need to be dragged to their primary care physician for annual checkups but seeing a urologist can make everyday life better.
Dr. Hosam Al Qudah, Consultant and Lead Urologist Fakeeh University Hospital believes that starting an open dialogue with a urologist is key to addressing the problems men often do not feel comfortable discussing: “I recommend every man over the age of 40 to start seeing a urologist regularly. This isn’t just something you do to protect yourself from prostate cancer. This is about taking charge of your prostate, urinary, and sexual health – to talk about the issues that you may find uncomfortable to address. This is also where I see our role as urologists – to normalize these as regular health conversations”.
In their late 40s, men may begin to face difficulty urinating due to an enlarged prostate, which is part of getting older. But too many trips to the bathroom — day and night — can make daily life more of a pain than it needs to be. To start, a urologist may recommend some lifestyle changes. This can include avoiding caffeine and alcohol. An enlarged prostate can also be treated with medications to relieve symptoms or even partially shrink the prostate.
The greatest concern here is the lifetime risk of prostate cancer, which can be predicted by a single prostate-specific antigen (PSA) determination that men are recommended to take during their 40s. “All men should take a baseline PSA test when they hit their mid-40s,” says Dr. Hosam. “It’s a simple blood test can help determine your risk of developing prostate cancer and show us specifically what we need to do to screen you in the future.”
If your PSA is .7 or below, you may only need to be screened every five years or so and your lifetime risk of prostate cancer is around 10% or less. If you’re at higher risk with a score of 1 or above, you may benefit from more frequent screening. If you reach 60 and your score is below a 1 or 2, it is safe to spread out the screening interval again. “Cancer screenings can be lifesavers,” continued Dr. Hosam, “but even a semi-regular visit to your urologist can keep you feeling good and make the aging problems all of us men face a little easier to cope with.”
Erectile dysfunction and declining libido are other issues that are not uncommon for men starting in their late 40s and early 50s, with about 1 in 10 adult males suffering from it[1]. The cause isn’t always physical, but a urologist can help if it is. “Your urologist can check your hormones with a simple blood test and prescribe testosterone replacements if you have low testosterone,” said Dr. Hosam.
#best hospital#healthcare#dubai#hospital#health and wellness#uae#urology doctor#urology specialist#Youtube
0 notes
Text
Covid-19 associated autoimmune encephalitis in Iraq. A Case Report by Faiq B. Basa in Journal of Clinical Case Reports Medical Images and Health Sciences
Abstract
Covid-19 infection frequently causes neurological symptoms. One of the mechanisms of indirect nervous system involvement is through inflammatory response and immune dysregulation. There are few recorded cases of indirect involvement of CNS by auto-antibodies. We present a case of a 6-year-old Iraqi boy with anti–N-methyl-d-aspartate receptor (anti-NMDAR) autoimmune encephalitis, associated with Covid-19. He presented with recent repeated attacks of refractory focal seizures preceded by behavioral changes. Serum and CSF anti-NMDAR antibodies were positive. Early recognition and treatment of autoimmune encephalitis are crucial as the prognosis is promising with early immunotherapy. Autoimmune encephalitis should be included in the main differential diagnosis whenever refractory epilepsy or new onset status epilepticus is faced. In the era of COVID-19, high vigilance is required as a possible association may increase autoimmune encephalitis incidence.
Key Words: Autoimmune encephalitis, Covid-19, refractory seizures, Anti-NMDA receptor encephalitis.
Introduction
The new coronavirus disease (Covid-19) was initially detected in Wuhan, China, in December 2019 [1]. There are now established Covid-19 cases with neurological symptoms, which developed either through direct harm of the central nervous system (CNS) caused by the severe acute respiratory syndrome coronavirus 2 (SARS‑CoV‑2), or via autoimmune processes [2, 3]. Several neurological complications have been described: encephalitis, meningitis, cerebrovascular diseases, acute disseminated encephalomyelitis (ADEM), and encephalopathies [4, 5].
Encephalitis is an inflammatory condition affecting the brain. Its etiologies are diverse. There are two main types of immune mediated encephalitis: (1) the paraneoplastic encephalitis syndromes, often associated with antibodies against intracellular neuronal proteins (onconeuronal proteins) [6]; (2) encephalitis syndromes associated with antibodies against neuronal cell surface/synaptic proteins, known as autoimmune encephalitis (AE) [7]. While paraneoplastic encephalitis syndromes are invariably cancer-related, autoimmune encephalitis syndromes may occur in the presence or absence of cancer.
AE includes a spectrum of disorders depending on the type of autoantibodies that are directed against various receptors and synaptic receptors and channels. The most common type of AE is the anti–N-methyl-d-aspartate receptor (anti-NMDAR) encephalitis, which was first described by Dalmau and colleagues in 2008 [8].
More recently, many new antibodies targeting neuronal surface antigens and synaptic proteins causing different forms of AE have been discovered, including those caused by autoantibodies targeting GABAB-R, GABAA-R, α-amino-3-hydroxy-5-methyl-4-isoxazolepropionic acid receptor (AMPAR), and contactin associated protein-like 2 (CASPR2), leucine-rich glioma inactivated 1 (LGI1), dipeptidyl-peptidase-like protein 6 (DPPX), Ig LON5, and others [9]. Additionally, AE might be the diagnosis behind new onset refractory seizure or status (NORSE), atypical movement disorders, rapidly progressive dementia of unknown etiology, or psychiatric and behavioral disturbances, sometimes mistaken for primary psychiatric illnesses [10].
In addition to the established association of AE with tumors like, e.g., ovarian teratoma in anti-NMDAR encephalitis, also the possibility of an association with SARS-CoV-2 infection arose. At present, there are few reported cases with positive anti-NMDAR encephalitis in association with COVID-19 [11, 12]. Nevertheless, this possible association will need more attention when neurologists, psychiatrists, and physicians face cases of unexplained refractory seizure and/or psychiatric manifestation.
Here, we report a unique case of a 6-year-old boy who presented with repeated attacks of refractory focal seizures, associated with autoimmune encephalitis.
Case Report Presentation
The parents of a 6-year-old boy from Erbil, Iraq, with normal perinatal and developmental history and no other known medical illnesses noticed an unusual decreased physical and cognitive activity, as new-emerging eating disorders. After a few days, while sleeping, the child developed abnormal body movements restricted to the left face and left body side with impaired consciousness. Therefore, he was taken to a pediatric emergency department.
After admission, the focal seizures, altered consciousness, and fever continued for two weeks. Consequently, he had to be intubated for 5 days in a Respiratory Care Unit (RCU), from which he was discharged after the seizures became less frequent. As per clinical findings, the patient was drowsy and mute, he had orofacial dyskinesia, and spastic quadriparesis, with infrequent focal seizures.
A brain MRI showed bilateral deep white matter cerebral hemisphere increased T2/FLAIR signal . EEG detected diffuse slowing and abnormal epileptic discharge .
Cerebrospinal Fluid (CSF) was clear and colorless. CSF glucose level was 55.7 mg/dL, and CSF total protein 14.6 mg/dL. No abnormal cells were detected. CSF oligoclonal bands (OCB) were positive. Real-time polymerase chain reaction (RT-PCR) assay was positive for severe acute respiratory syndrome coronavirus 2 (SARS‑CoV‑2). Central Nervous System (CNS) tuberculosis and Viral Encephalitis were excluded by Cerebrospinal fluid polymerase chain reaction (CSF-PCR) assays. Serum and CSF anti-NMDA receptor IgG Ab were positive.
The therapeutic intervention started with intravenous (IV) methylprednisolone therapy (15 mg/kg/day) for 5 days, followed by intravenous immunoglobulin (IVIG), according to the high-dose immunomodulatory therapy strategy (1,000 mg/kg), for 5 days [13]. Anti-seizure medication (ASM) was commenced as well.
In the following weeks a mild improvement in terms of enhanced consciousness, limbs movements, and weight gain was observed. Therefore, the nasogastric tube was removed, and oral feeding restarted. We monitored the child through regular evaluations and biochemical analyses until complete clinical recovery. ASM was not discontinued. The overall treatment was well tolerated.
Discussion
Autoimmune encephalitis is a condition that can be easily missed as it is not commonly considered in the differential diagnosis of various medical presentations. However, such diagnosis should be always taken into consideration when a person, particularly a child, presents with a new onset of refractory status epilepticus (NORSE) and/or new behavioral or psychiatric conditions. An early diagnosis of AE is essential, as the treatment is different from other conditions. With correct timely interventions the outcome is frequently favorable.
Though SARS-Cov-2 virus rarely invades the nervous system, Covid-19 infection frequently causes neurological symptoms like headache, delirium, anosmia, and dysgeusia [14]. One of the mechanisms of indirect nervous system involvement is through inflammatory response and immune dysregulation. There are few recorded cases of indirect involvement of CNS by auto-antibodies that are directed against the surface and synaptic protein. This case is one of the rare cases of Anti NMDA antibody autoimmune encephalitis that is associated with Covid-19 infection [15]. It indicates that in the era of COVID-19, high vigilance is required as a possible association may increase AE incidence.
A recent systemic review that analyzed 16 studies, including a total of 161 patients with NORSE [16], showed that the most frequent cause was AE. In addition to the well-known association with teratoma and cancer, AE, and specifically Anti-NMDA receptor Ab encephalitis, could be associated with a SARS‑CoV‑2 infection, either concomitantly or as post-infection manifestation. In this reported case, immunotherapy, in addition to anti-seizure medication, showed to be effective.
The main limitation of this report is the relatively short follow-up period. Observation of the child is ongoing to detect possible medium- or long-term consequences.
Conclusion
This case demonstrated that autoimmune encephalitis should be always considered when facing a new onset of refractory epilepsy and/or abnormal behaviors, and that it could be associated with SARS-Cov2 infection. Early diagnosis of an autoimmune encephalitis and ensuing correct treatment can be lifesaving.
Statement of Ethics: The parents of the child involved in this study have given their written informed consent to publish his case (including the publication of images). Ethical approval is not required for this study in accordance with national guidelines.
Conflict of Interest Statement: The authors have no conflicts of interest to declare.
Funding Sources: This case report required no funding.
#Autoimmune encephalitis#Covid-19#refractory seizures#Anti-NMDA receptor encephalitis#Journal of Clinical Case Reports Medical Images and Health Sciences publication fee.
1 note
·
View note
Text
What’s the difference between Pfizer/BioNTech, Moderna, and Novavax COVID-19 vaccines?
The Pfizer-BioNTech and Moderna COVID-19 vaccines use mRNA as the active ingredient. The mRNA is converted by our cells into the antigen, in this case, the spike protein of SARS-CoV-2.
The vaccine contains the mRNA, which is synthesized in the lab using a DNA template, the building blocks of RNA, and the enzyme that puts those building blocks together into the right order. mRNA is the molecule template for every protein in every organism. The mRNA sequence is a code for our cells to link amino acids together into functional proteins
mRNA is very fragile, so it is encased in a lipid nanoparticle (LNP) that protects it until it gets into our cells. When the vaccine is administered, the mRNA is released and is used to synthesize the spike protein which is displayed by cells that produced it. That spike protein is recognized by our innate immune cells like dendritic cells and macrophages as well as B cells, which initiates immune response and generation of memory immunity.

In contrast, Novavax is a protein-based vaccine, which contains the prefabricated antigen - the spike protein - instead of the template for it. To make the antigen, we turn cells into protein-producing factories in the lab.
Novavax uses Sf9 cells (moth cells) infected with an insect-specific virus that has been genetically engineered to contain the gene for the spike protein of SARS-CoV-2. These viruses will hijack the cellular machinery of the Sf9 cells to produce lots of spike proteins and baby viruses. Those will continue to reproduce and produce proteins, which will be harvested, purified, and formulated with the other ingredients in the final vaccine.
When the vaccine is injected, the antigen will be recognized by the same innate immune cells listed above, which will trigger the same immune response pathway.

While the vaccines use different technologies, ingredients, and manufacturing processes, the immune responses center around recognition of the spike protein and generating adaptive immune responses targeting that antigen.
#novavax#covid#covid 19#sars cov 2#vaccines#vaccine#vaccination#get vaccinated#long covid#illness#chronic illness#covid vaccine#mrna#jn.1#kp.2#sf9#Novavax vaccine#mrna vaccine#Pfizer-BioNTech#Moderna#moderna vaccine#pfizer vaccine#pfizer
6 notes
·
View notes
Text
“Kappa Light Chain Deficiency”, Victor McKusick, Mendelian Inheritance in Man, 1966. 卡帕轻链缺陷。(IGKC).
Here I present: “Kappa Light Chain Deficiency”, Victor McKusick, Mendelian Inheritance in Man’, 1966. 卡帕轻链缺陷。(IGKC). INTRODUCTION. Immunoglobulins are the antigen recognition molecules of B cells. The immunoglobulin light chain is the small polypeptide subunit of the antibody (immunoglobulin). A typical antibody is composed of two (2) immunoglobulin heavy chains and two (2) immunoglobulin…
0 notes
Text
On activation of the downstream signaling, the body will recognize and responds to viral RNAs. This recognition of single and double-stranded RNA is by endosomal receptors such as Toll-like receptors (TLR3), TLR7, and TLR8. Double-stranded and 5′-triphosphate modified RNA is recognized by the cytosolic receptor’s retinoic acid-inducible gene-I (RIG-1) and melanoma differentiation-associated protein 5 (MDA-5). Allergic reactions and anaphylactic shock were reported when the body’s immune system is activated in an uncontrolled manner. This overstimulation of the immune system on a molecular level will limit the protein translation, expression of antigens, and vaccine efficacy. This limitation can be overcome by nucleobase modifications. N1-methylpseudouridin, a modified nucleobase, will increase the protein output and decrease the activation of TLR3. Modified nucleotides through RIG-1 not only affect protein-RNA interaction but also decrease the ability of mRNAs to disseminate immunological signals. Translation efficiency of mRNA into protein is improved because of N1-methylpseudouridin.
1 note
·
View note
Text
Fwd: Graduate position: UHamburg_Germany.EvolutionaryGeneticsImmunity
Begin forwarded message: > From: [email protected] > Subject: Graduate position: UHamburg_Germany.EvolutionaryGeneticsImmunity > Date: 4 August 2024 at 05:11:01 BST > To: [email protected] > > > > We invite applications for a PhD position to study the evolution, > genetics and genomics of the adaptive immune system (especially > MHC/HLA), using humans and/or fish as a model system. The specific topic > is open and will be matched with the candidate's interests and skills. > We use both computational and molecular approaches in our lab. > > The position is a full-time PhD position funded for initially 3 years. > In Germany, PhD students are expected to complete their PhD work within > three years, as they don't have to collect course credits. However, > extensions are possible depending on funding availability. A Masters > degree in a relevant field is required to apply. > > *The application deadline is August 25* > > Please follow this link to apply online through the university system: > > https://ift.tt/Cqp2X9B > > In our group we are studying the genetic basis for variation in > immunocompetence and disease susceptibility in vertebrates, with a > particular focus on the adaptive immune system and specifically the > process of antigen presentation (MHC/HLA) and recognition (TCR/T cell > repertoires). Our main model systems are humans and three-spined > sticklebacks (a small, but cool fish). We usually take an evolutionary > perspective and aim to understand the factors and mechanisms that > maintain genetic diversity in the context of host-pathogen coevolution > (in both humans and fish), but we are also interested in the > consequences of this diversity for the individual's health and have > several ongoing collaborations with clinical groups on specific complex > diseases in humans (e.g. HIV, Tuberculosis, autoimmunity, cancer). > Several project ideas are available in this context and can be tailored > to the candidate’s interest and experience. > > We expect the successful candidate to have a decent background in > molecular and evolutionary biology. Some knowledge of immunology and > bioinformatics would be a plus. For more specific requirements and > duties, including a minor level of teaching, please see the advert link > above. We offer an inspiring research environment with expertise in > molecular, evolutionary, and computational biology, immunology and > population genetics/genomics. Our group has state-of-the art molecular > labs, including NGS sequencing capacity, and has priority access to the > university's HPC cluster. > > Our newly renovated labs and offices in the Institute for Animal Cell > and Systems Biology at the University of Hamburg are situated in the > middle of Hamburg, the second largest city in Germany. The institute is > neighboring the main university campus with its bustling student life > and cafes, and is easy to reach by bike or any public transport (and > car, if you must). > > Please see also our lab website for more info: > https://ift.tt/WEJAeBN > > Please do not hesitate to contact me for informal inquiries, > Tobias Lenz > > > > Prof. Dr. Tobias Lenz, Heisenberg-Professor > Research Unit for Evolutionary Immunogenomics > University of Hamburg > Department of Biology > Institute of Animal Cell and Systems Biology > Martin-Luther-King-Platz 3 > 20146 Hamburg, Germany > > Email: [email protected] > > https://ift.tt/WEJAeBN > > > > [email protected] > > (to subscribe/unsubscribe the EvolDir send mail to > [email protected]
0 notes