#stem cell research center
Explore tagged Tumblr posts
Text
Why GMP Compliance Is Paramount For High-Quality Mesenchymal Stem Cell?
The past twenty years have witnessed a fascinating unboxing of the mesenchymal stem cells. These microscopic marvels hold the potential to revolutionize how we approach disease. These mesenchymal stem cells are extracted from adult cells, and hence, they rarely receive any ethical backlash. Unlike most stem cells, mesenchymal ones boast remarkable versatility and can morph into diverse cell types, from bone to blood vessels. These cells carry several regenerative and anti-inflammatory prowess, which has propelled them to the forefront of stem cell therapy. The use in therapeutic applications has ignited a surge in demand that outpaces our current production capabilities.
Given the surge in demand for MSCs for research, providing researchers with high-quality stem cells for reproducible research is necessary. Enter the realm of Good Manufacturing Practices (GMP), our roadmap towards building factories for these cellular powerhouses, ensuring not just quantity but unparalleled quality and safety. Buckle up, science researchers, for we’re about to delve into the intricate dance of scaling up MSC production while upholding the highest standards, paving the way for a future where these microscopic maestros weave their magic on a grand scale.
#Mesenchymal Stem Cells#MSCs#GMP Compliance Is Paramount For High-Quality Mesenchymal Stem Cell#Challenges With Mesenchymal Stem Cells#Cell culture#customized primary cells#primary cells#biotech company#stem cells#exosomes#stem cell research center#regenerative medicine#bioengineering#Kosheeka
0 notes
Text
youtube
Stem Cell Treatment For Fibromyalgia | Orthopedic Disease Treatment | Pain | Regenerative | Cells |
https://www.globalstemcellcare.com/orthopedic/stem-cell-treatment-for-fibromyalgia/
#Fibromyalgia#Chronic Pain#Fibromyalgia Awareness#Pain Management#Chronic Fatigue#Stem Cell Therapy#Stem Cells for Fibromyalgia#Fibromyalgia Treatment#Fibro Healing#Stem Cell Research#Stem Cells#Fibromyalgia Care#Alternative Fibromyalgia Treatment#Innovative Fibro Treatment#Exosome#Exosome Therapy#Best Stem Cell Hospital in India#Best Stem Cell Center in Delhi#Orthopedic Disease Treatment#Pain Relief#Pain Management.#advanced stem cell treatment#Youtube
0 notes
Text
Old news, but something people should remember
Never let them tell you "We didn't know covid was so bad" or "It's mild for kids." We knew.
Also preserved on our archive
By John Anderer
PHILADELPHIA, Pa. — The news about coronavirus and children just got a lot worse. A troubling study by researchers at the Children’s Hospital of Philadelphia reports a “high proportion” of children infected with SARS-CoV-2 show elevated levels of a biomarker tied to blood vessel damage. Making matters worse, this sign of cardiovascular damage is being seen in asymptomatic children as well as kids experiencing COVID-19 symptoms.
Additionally, many examined children testing positive for SARS-CoV-2 are being diagnosed with thrombotic microangiopathy (TMA). TMA leads to clots in small blood vessels and has been linked to severe COVID symptoms among adult patients.
“We do not yet know the clinical implications of this elevated biomarker in children with COVID-19 and no symptoms or minimal symptoms,” says co-senior author David T. Teachey, MD, Director of Clinical Research at the Center for Childhood Cancer Research at CHOP, in a media release. “We should continue testing for and monitoring children with SARS-CoV-2 so that we can better understand how the virus affects them in both the short and long term.”
The complex connection between kids and COVID It’s fairly well established at this point that most children who contract coronavirus experience little to no symptoms. However, a small portion of young patients develop major symptoms or a post-viral inflammatory response to COVID-19 called Multisystem Inflammatory Syndrome in Children (MIS-C).
TMA in adults has a connection to more severe cases of COVID-19. Scientists believe the component of the immune system called “complement cascade” helps to mediate TMA in adults. The complement cascade is supposed to enhance and strengthen immune responses when a threat is present, but it can also backfire and lead to more inflammation. Up until now, the role of complement cascade during childhood TMA hadn’t been investigated.
To research the topic of “complement activation” in kids with SARS-CoV-2, researchers analyzed a group of 50 pediatric COVID-19 patients between April and July 2020. Among the group, 21 showed minimal to no symptoms, 11 experienced severe symptoms, and 18 developed MIS-C.
To search for complement activation and TMA among each patient, researchers used soluble C5b9 (sC5b9) as a biomarker. Scientists have used this substance for quite some time to assess the severity of TMA after stem cell procedures. In brief terms, the higher the level of sC5b9 in a transplant patient, the greater their mortality risk.
No symptoms doesn’t mean there’s no problem Study authors discovered elevated levels of C5b9 in both patients with severe COVID-19 and MIS-C. While this didn’t surprise researchers, they did get a shock from seeing high levels of C5b9 among even asymptomatic youngsters.
Some of the lab data regarding TMA had to be obtained after the fact. This meant researchers didn’t have a complete dataset to work with for all 50 studied patients. Among 22 patients researchers did have complete data for, 86 percent (19 children) were diagnosed with TMA. Every child had elevated levels of sC5b9, even those without TMA.
“Although most children with COVID-19 do not have severe disease, our study shows that there may be other effects of SARS-CoV-2 that are worthy of investigation,” Dr. Teachey concludes. “Future studies are needed to determine if hospitalized children with SARS-CoV-2 should be screened for TMA, if TMA-directed management is helpful, and if there are any short- or long-term clinical consequences of complement activation and endothelial damage in children with COVID-19 or MIS-C. The most important takeaway from this study is we have more to learn about SARS-CoV-2. We should not make guesses about the short and long-term impact of infection.”
The study is published in Blood Advances.
Study Link: ashpublications.org/bloodadvances/article/4/23/6051/474421/Evidence-of-thrombotic-microangiopathy-in-children
#mask up#covid#pandemic#covid 19#wear a mask#public health#coronavirus#sars cov 2#still coviding#wear a respirator
45 notes
·
View notes
Text

AI model improves 4D STEM imaging for delicate materials
Researchers at Monash University have developed an artificial intelligence (AI) model that significantly improves the accuracy of four-dimensional scanning transmission electron microscopy (4D STEM) images. Called unsupervised deep denoising, this model could be a game-changer for studying materials that are easily damaged during imaging, like those used in batteries and solar cells. The research from Monash University's School of Physics and Astronomy, and the Monash Center of Electron Microscopy, presents a novel machine learning method for denoising large electron microscopy datasets. The study was published in npj Computational Materials. 4D STEM is a powerful tool that allows scientists to observe the atomic structure of materials in unprecedented detail.
Read more.
#Materials Science#Science#Materials Characterization#Computational materials science#Electron microscopy#Monash University
19 notes
·
View notes
Text
Developing Zone
A method for creating human brain organoids – lab-grown mini brain models – with a compartment of cells called the outer subventricular zone closely matching the processes involved in development of the brain's neocortex in life
Read the published research article here
Video from work Ryan M. Walsh, Raffaele Luongo and Elisa Giacomelli, and colleagues
Center for Stem Cell Biology and Developmental Biology Program, Memorial Sloan Kettering Cancer Center, New York, NY, USA; Institute of Oncology Research (IOR), Bellinzona Institutes of Science (BIOS+), Bellinzona, Switzerland
Video originally published with a Creative Commons Attribution – NonCommercial – NoDerivs (CC BY-NC-ND 4.0)
Published in Cell Reports, April 2024
You can also follow BPoD on Instagram, Twitter and Facebook
18 notes
·
View notes
Text
Lots of great news on HIV prevention coming out in just the last few days (this article in addition to the person cured after stem cell treatments):
A twice-yearly injection could help prevent HIV infections, according to the results of a new study described by medical experts as a breakthrough. In a randomized trial involving more than 5,000 young women and girls in South Africa and Uganda, none of those who received the prevention shots contracted HIV. The results were published in the New England Journal of Medicine on Wednesday. “This appears to be a new breakthrough for HIV prevention. If these injections can be widely distributed at low cost, it would dramatically reduce the risk of new HIV infections worldwide,” said Sarah Palmer, co-director of the Center for Virus Research at the Westmead Institute for Medical Research in Sydney, who was not involved in the peer-reviewed study. “It is especially encouraging this research focused on young women in Africa who are so highly at-risk for HIV infection.�� Worldwide there are about 1.3 million new HIV infections every year, with women and girls accounting for 44 percent of them. In sub-Saharan Africa, that proportion is 62 percent.
14 notes
·
View notes
Text

Low gravity in space travel found to weaken and disrupt normal rhythm in heart muscle cells
Johns Hopkins Medicine scientists who arranged for 48 human bioengineered heart tissue samples to spend 30 days at the International Space Station report evidence that the low gravity conditions in space weakened the tissues and disrupted their normal rhythmic beats when compared to Earth-bound samples from the same source.
The scientists said the heart tissues "really don't fare well in space," and over time, the tissues aboard the space station beat about half as strongly as tissues from the same source kept on Earth.
The findings, they say, expand scientists' knowledge of low gravity's potential effects on astronauts' survival and health during long space missions, and they may serve as models for studying heart muscle aging and therapeutics on Earth.
A report of the scientists' analysis of the tissues is published in the Proceedings of the National Academy of Sciences.
Previous studies showed that some astronauts return to Earth from outer space with age-related conditions, including reduced heart muscle function and arrythmias (irregular heartbeats), and that some—but not all—effects dissipate over time after their return.
But scientists have sought ways to study such effects at a cellular and molecular level in a bid to find ways to keep astronauts safe during long spaceflights, says Deok-Ho Kim, Ph.D., a professor of biomedical engineering and medicine at the Johns Hopkins University School of Medicine. Kim led the project to send heart tissue to the space station.
To create the cardiac payload, scientist Jonathan Tsui, Ph.D. coaxed human induced pluripotent stem cells (iPSCs) to develop into heart muscle cells (cardiomyocytes). Tsui, who was a Ph.D. student in Kim's lab at the University of Washington, accompanied Kim as a postdoctoral fellow when Kim moved to Johns Hopkins University in 2019. They continued the space biology research at Johns Hopkins.
Tsui then placed the tissues in a bioengineered, miniaturized tissue chip that strings the tissues between two posts to collect data about how the tissues beat (contract). The cells' 3D housing was designed to mimic the environment of an adult human heart in a chamber half the size of a cell phone.
To get the tissues aboard the SpaceX CRS-20 mission, which launched in March 2020 bound for the space station, Tsui says he had to hand-carry the tissue chambers on a plane to Florida, and continue caring for the tissues for a month at the Kennedy Space Center. Tsui is now a scientist at Tenaya Therapeutics, a company focused on heart disease prevention and treatment.
Once the tissues were on the space station, the scientists received real-time data for 10 seconds every 30 minutes about the cells' strength of contraction, known as twitch forces, and on any irregular beating patterns. Astronaut Jessica Meir, Ph.D., M.S. changed the liquid nutrients surrounding the tissues once each week and preserved tissues at specific intervals for later gene readout and imaging analyses.
The research team kept a set of cardiac tissues developed the same way on Earth, housed in the same type of chamber, for comparison with the tissues in space.
When the tissue chambers returned to Earth, Tsui continued to maintain and collect data from the tissues.
"An incredible amount of cutting-edge technology in the areas of stem cell and tissue engineering, biosensors and bioelectronics, and microfabrication went into ensuring the viability of these tissues in space," says Kim, whose team developed the tissue chip for this project and subsequent ones.
Devin Mair, Ph.D., a former Ph.D. student in Kim's lab and now a postdoctoral fellow at Johns Hopkins, then analyzed the tissues' ability to contract.
In addition to losing strength, the heart muscle tissues in space developed irregular beating (arrhythmias)—disruptions that can cause a human heart to fail. Normally, the time between one beat of cardiac tissue and the next is about a second. This measure, in the tissues aboard the space station, grew to be nearly five times longer than those on Earth, although the time between beats returned nearly to normal when the tissues returned to Earth.
The scientists also found, in the tissues that went to space, that sarcomeres—the protein bundles in muscle cells that help them contract—became shorter and more disordered, a hallmark of human heart disease.
In addition, energy-producing mitochondria in the space-bound cells grew larger, rounder and lost the characteristic folds that help the cells use and produce energy.
Finally, Mair, Eun Hyun Ahn, Ph.D.—an assistant research professor of biomedical engineering—and Zhipeng Dong, a Johns Hopkins Ph.D. student, studied the gene readout in the tissues housed in space and on Earth. The tissues at the space station showed increased gene production involved in inflammation and oxidative damage, also hallmarks of heart disease.
"Many of these markers of oxidative damage and inflammation are consistently demonstrated in post-flight checks of astronauts," says Mair.
Kim's lab sent a second batch of 3D engineered heart tissues to the space station in 2023 to screen for drugs that may protect the cells from the effects of low gravity. This study is ongoing, and according to the scientists, these same drugs may help people maintain heart function as they get older.
The scientists are continuing to improve their "tissue on a chip" system and are studying the effects of radiation on heart tissues at the NASA Space Radiation Laboratory. The space station is in low Earth orbit, where the planet's magnetic field shields occupants from most of the effects of space radiation.
IMAGE: Heart tissues within one of the launch-ready chambers. Credit: Jonathan Tsui
5 notes
·
View notes
Text
Voting over abortion rights shows how naive and clueless you are. Natural abortifacients grow all around you. Cultures that aren’t centered around scamming each other have been using them for thousands of years. Your body will tell you when you are pregnant. Relying on doctors for any of this is a childish cry for attention. Every girl I’ve known that made a big deal over pregnancy was an attention seeking narcissist that conjured up scenarios to exploit for sympathy, attention, and resources they had no need for. They acted like they rely on doctors for all of this while telling people they’re pregnant without any medical confirmation that they’re actually pregnant. Mature intelligent women tell the least amount of people necessary and already know how to handle it themselves if they are not in the place to raise a child. Aborted babies are sold to “research companies” to sell the blood and stem cells to superstitious rich people obsessed with immortality and eternal youth.
5 notes
·
View notes
Text
Washington Post:
Scientists found a major clue why 4 of 5 autoimmune patients are women By Mark Johnson and Sabrina Malhi February 1, 2024 at 11:02 a.m. EST
An international team led by scientists at Stanford University has discovered a probable explanation for a decades-old biological mystery: why vastly more women than men suffer from autoimmune diseases such as lupus and rheumatoid arthritis.
Women account for about 80 percent of the people afflicted with autoimmune diseases, a collection of more than 100 ailments that burden a combined 50 million Americans, according to the nonprofit Autoimmune Association. In simple terms, these illnesses manipulate the body’s immune system to attack healthy tissue.
In a paper published Thursday in the journal Cell, researchers present new evidence that a molecule called Xist — pronounced like the word “exist” and found only in women — is a major culprit in these diseases.
Better understanding of this molecule could lead to new tests that catch autoimmune diseases sooner and, in the longer term, to new and more effective treatments, researchers said.
Women typically have two X chromosomes, while men usually have an X and a Y. Chromosomes are tight bundles of genetic material that carry instructions for making proteins. Xist plays a crucial role by inactivating one of the X chromosomes in women, averting what would otherwise be a disastrous overproduction of proteins.
However, the research team found that in the process Xist also generates strange molecular complexes linked to many autoimmune diseases.
Although scientists conducted much of their work in mice, they made an intriguing discovery involving human patients: The Xist complexes ― long strands of RNA entangled with DNA and proteins ― trigger a chemical response in people that is a hallmark of autoimmune diseases.
Discovery of the role played by the Xist molecule does not explain how men get these diseases, or why a few autoimmune diseases, such as Type 1 diabetes, have a higher incidence among men.
“Clearly there’s got to be more, because one-tenth of lupus patients are men,” said David Karp, chief of the division of rheumatic diseases at the UT Southwestern Medical Center in Dallas. “So it’s not the only answer, but it’s a very interesting piece of the puzzle.”
A tale of two X’s
An illustration shows one of the two X chromosomes typically found in women being disabled to ensure the right levels of protein production. (Emily Moskal/Stanford Medicine) Autoimmune diseases have long proved difficult to address. Treatments are limited, and many of the diseases are chronic, requiring lifelong management. Most have no cure, leaving millions of Americans hoping that science will eventually offer better explanations for these ailments.
Stephanie Buxhoeveden was 25 when she began experiencing vision problems in her left eye and found herself unable to hold a syringe in her left hand — a critical tool for her nursing job. The reason: multiple sclerosis, an autoimmune condition in which the immune system attacks the protective covering of the brain, spinal cord and optic nerves.
“I was overwhelmed and scared because I knew there was no cure,” the Virginia resident said. “All of these things that I had laid out, planned and worked really hard for all of a sudden were completely up in the air and no longer guaranteed.”
Previous theories had suggested that the gender imbalance in these diseases might be caused by the main female hormones, estrogen and progesterone, or by the mere presence of a second X chromosome.
A tantalizing clue stemmed from men who have two X chromosomes and one Y chromosome, a rare condition called Klinefelter syndrome. These men run a much higher risk of suffering from autoimmune diseases, suggesting that the number of X chromosomes plays an important role.
Howard Y. Chang, senior author on the Cell paper and professor of dermatology and genetics at Stanford, said he began thinking about the ideas that led to the new discovery when he identified more than 100 proteins that either bind directly to Xist or to other proteins that bind to Xist. Looking at those collaborator proteins, he noticed that many had been linked to autoimmune diseases.
Chang and his team engineered male mice that produced Xist to test whether males that made the molecule would also have higher rates of autoimmune diseases.
Since Xist by itself is not sufficient to cause an autoimmune disease, the scientists used an environmental trigger to induce a lupus-like disease in these mice. They observed that male mice then produced Xist at levels close to those of regular female mice, and well above those of regular male mice.
In humans, genetics and environmental factors, such as a viral or bacterial infection, can also help trigger autoimmune diseases.
The scientists obtained serums from human patients with dermatomyositis, a rare autoimmune disease that causes muscle weakness and skin rash. Serum is the part of the blood that contains antibodies that fight disease. They found that in these patients, Xist complexes produce what are called autoantibodies. Instead of defending the body from invaders, as an antibody would, the autoantibody targets features of the body.
Inactivation of the second X chromosome remains an important process “that you don’t necessarily want to get rid of or tinker with too much,” said Karp of UT Southwestern.
“But this work takes it in a totally different direction, and says that the mechanism that is needed to turn off the second X chromosome, that mechanism in itself might be responsible for generating autoimmunity,” Karp said.
A better understanding of the mechanisms in these diseases would be significant if researchers can use it to develop new diagnostic tools, he added: “We are still using laboratory tests that were developed in the 1940s and ’50s and ’60s because they were easy to do, and they detected the most robust autoimmune responses.”
A long road to new treatments
Jeffrey A. Sparks, an associate physician and director of immuno-oncology and autoimmunity at Brigham and Women’s Hospital who was not involved in the study, said that it will be interesting to see how the treatment options available now might fit into this newfound mechanism.
“The sky’s the limit here,” Sparks said, adding, “I think once you understand the fundamental mechanisms, you could think about developing therapies, early detection and preventions.”
Major advances in treatment, though, may be years away, according to Keith B. Elkon, an adjunct professor of immunology and associate director at the Center for Innate Immunity and Immune Diseases at the University of Washington.
Still, he said, scientific breakthroughs in the past 20 years have prolonged the lives of many people with autoimmune conditions.
“In 1950, if you’ve got a diagnosis of lupus, it would have been as bad as getting a diagnosis of cancer,” Elkon said. “But over the last 15, 20 years there’ve been really striking breakthroughs in understanding disease. It’s at the cusp of now being manageable.”
Buxhoeveden, who is now 36 and a PhD candidate in nursing, is using immunosuppressants to manage her MS. She said she was encouraged by the fact that “we’ve made progress like this study to better understand what it is that triggers it.”
#washington post#autoimmune disorder#80 percent of sufferers are women#no wonder the research too so long
9 notes
·
View notes
Text

20 July 2017 | Catherine, Duchess of Cambridge and Prince William, Duke of Cambridge arrive for a visit of the German Cancer Research Center on the second day of their visit to Germany in Heidelberg, Germany. The Duke and Duchess of Cambridge will meet researchers including Nobel Prize winner prof. Dr. Harald zur Hausen, and visit the stem cell research lab. The royal couple are on a three-day trip to Germany that includes visits to Berlin, Hamburg and Heidelberg. (c) Thomas Niedermueller/Getty Images
#Catherine#Duchess of Cambridge#Princess of Wales#Prince William#Duke of Cambridge#Prince of Wales#Britain#2017#Thomas Niedermueller#Getty Images
6 notes
·
View notes
Text
Noninvasive Technique Reveals How Cells’ Gene Expression Changes Over Time - Technology Org
New Post has been published on https://thedigitalinsider.com/noninvasive-technique-reveals-how-cells-gene-expression-changes-over-time-technology-org/
Noninvasive Technique Reveals How Cells’ Gene Expression Changes Over Time - Technology Org
MIT researchers can now track a cell’s RNA expression to investigate long-term processes like cancer progression or embryonic development.
DNA – artistic impression. Image credit: Image by kjpargeter on Freepik
Sequencing all of the RNA in a cell can reveal a great deal of information about its function and what it is doing at a given time. However, the sequencing process destroys the cell, making it difficult to study ongoing changes in gene expression.
An alternative approach developed at MIT could enable researchers to track such changes over extended periods of time. The new method, which is based on a noninvasive imaging technique known as Raman spectroscopy, doesn’t harm cells and can be performed repeatedly.
Using this technique, the researchers showed that they could monitor embryonic stem cells as they differentiated into several other cell types over several days. This technique could enable studies of long-term cellular processes such as cancer progression or embryonic development, and one day might be used for diagnostics for cancer and other diseases.
“With Raman imaging you can measure many more time points, which may be important for studying cancer biology, developmental biology, and a number of degenerative diseases,” says Peter So, a professor of biological and mechanical engineering at MIT, director of MIT’s Laser Biomedical Research Center, and one of the authors of the paper.
Koseki Kobayashi-Kirschvink, a postdoc at MIT and the Broad Institute of Harvard and MIT, is the lead author of the study, which appears today in Nature Biotechnology. The paper’s senior authors are Tommaso Biancalani, a former Broad Institute scientist; Jian Shu, an assistant professor at Harvard Medical School and an associate member of the Broad Institute; and Aviv Regev, executive vice president at Genentech Research and Early Development, who is on leave from faculty positions at the Broad Institute and MIT’s Department of Biology.
Imaging gene expression
Raman spectroscopy is a noninvasive technique that reveals the chemical composition of tissues or cells by shining near-infrared or visible light on them. MIT’s Laser Biomedical Research Center has been working on biomedical Raman spectroscopy since 1985, and recently, So and others in the center have developed Raman spectroscopy-based techniques that could be used to diagnose breast cancer or measure blood glucose.
However, Raman spectroscopy on its own is not sensitive enough to detect signals as small as changes in the levels of individual RNA molecules. To measure RNA levels, scientists typically use a technique called single-cell RNA sequencing, which can reveal the genes that are active within different types of cells in a tissue sample.
In this project, the MIT team sought to combine the advantages of single-cell RNA sequencing and Raman spectroscopy by training a computational model to translate Raman signals into RNA expression states.
“RNA sequencing gives you extremely detailed information, but it’s destructive. Raman is noninvasive, but it doesn’t tell you anything about RNA. So, the idea of this project was to use machine learning to combine the strength of both modalities, thereby allowing you to understand the dynamics of gene expression profiles at the single cell level over time,” Kobayashi-Kirschvink says.
To generate data to train their model, the researchers treated mouse fibroblast cells, a type of skin cell, with factors that reprogram the cells to become pluripotent stem cells. During this process, cells can also transition into several other cell types, including neural and epithelial cells.
Using Raman spectroscopy, the researchers imaged the cells at 36 time points over 18 days as they differentiated. After each image was taken, the researchers analyzed each cell using single molecule fluorescence in situ hybridization (smFISH), which can be used to visualize specific RNA molecules within a cell. In this case, they looked for RNA molecules encoding nine different genes whose expression patterns vary between cell types.
This smFISH data can then act as a link between Raman imaging data and single-cell RNA sequencing data. To make that link, the researchers first trained a deep-learning model to predict the expression of those nine genes based on the Raman images obtained from those cells.
Then, they used a computational program called Tangram, previously developed at the Broad Institute, to link the smFISH gene expression patterns with entire genome profiles that they had obtained by performing single-cell RNA sequencing on the sample cells.
The researchers then combined those two computational models into one that they call Raman2RNA, which can predict individual cells’ entire genomic profiles based on Raman images of the cells.
Tracking cell differentiation
The researchers tested their Raman2RNA algorithm by tracking mouse embryonic stem cells as they differentiated into different cell types. They took Raman images of the cells four times a day for three days, and used their computational model to predict the corresponding RNA expression profiles of each cell, which they confirmed by comparing it to RNA sequencing measurements.
Using this approach, the researchers were able to observe the transitions that occurred in individual cells as they differentiated from embryonic stem cells into more mature cell types. They also showed that they could track the genomic changes that occur as mouse fibroblasts are reprogrammed into induced pluripotent stem cells, over a two-week period.
“It’s a demonstration that optical imaging gives additional information that allows you to directly track the lineage of the cells and the evolution of their transcription,” So says.
The researchers now plan to use this technique to study other types of cell populations that change over time, such as aging cells and cancerous cells. They are now working with cells grown in a lab dish, but in the future, they hope this approach could be developed as a potential diagnostic for use in patients.
“One of the biggest advantages of Raman is that it’s a label-free method. It’s a long way off, but there is potential for the human translation, which could not be done using the existing invasive techniques for measuring genomic profiles,” says Jeon Woong Kang, an MIT research scientist who is also an author of the study.
Written by Anne Trafton
Source: Massachusetts Institute of Technology
You can offer your link to a page which is relevant to the topic of this post.
#aging#Aging news#algorithm#approach#Biology#biotechnology#Biotechnology news#blood#blood glucose#breast cancer#Broad Institute#Cancer#cell#cell types#Cells#change#chemical#Composition#data#deal#development#diagnostics#Diseases#DNA#dynamics#embryonic development#embryonic stem cells (ESC)#engineering#Evolution#Faculty
2 notes
·
View notes
Text
What are Fibroblast Cells?
he pig genome is three times closer to our genome that that of mice. Take the example of cystic fibrosis, the introduction of the mutation responsible for the disease in mice has not shown the exact course of the disease however, recent reports show the successful recapitulation of the disease in pig models. As there is a similarity between the cardiovascular system, the gastrointestinal tract, and the pancreas between pigs and humans, research on diseases and toxicology can be carried out on these models with Swine Fibroblast Cells (Walters et al, 2013).
#pig cell#fibroblast cells#pig stem cells#porcine cells#pig cells#what are fibroblast cells#what type of cells are fibroblasts#pig dna vs human dna#human and pig dna similarity#Cell culture#customized primary cells#primary cells#biotech company#stem cells#exosomes#stem cell research center#regenerative medicine#bioengineering#Kosheeka
0 notes
Text
Really? Does Pershing not have any guards? Not even just one? He is a scientist and he is technically a defector. Wouldn’t somebody, from either side want to, you know... *stab stab* I really thought that box was going to be a pipe bomb or Poloniom-210 or at least a dead cat or something.
Also, why are they both so taken with Coruscant? It was the Imperial Center for twenty years, it’s not really something the New Republic built. (Yes, I know whatsherface said she’d been there before, but it really shouldn’t be that different from when it was Imperial. Not for mass appeal, family friendly things like a fair. Bread and circuses type of thing. You have to keep the public happy, even if you are crushing them beneath your boot heel. A certain section of the population would not notice a difference from what it was before. They would just whine about all the foreigners.)
I’m also not entirely convinced of this amnesty program, having grown up in a country that had a rather significant change in government. Especially for lower ranking officers and technical personnel, I see no reason why they wouldn’t just be sent back to work doing what they did before. My country’s army didn’t all get fired and replaced when the new government took over. They just all integrated with the new government’s forces. And those who couldn’t handle that could just fuck off elsewhere, most likely to somewhere with a desert. I mean, America should know this! They didn’t arrest all the Confederates at the end of the Civil War. And Imperials aren’t cultists. They don’t really need rehabilitation and reintegration.
And the ethics of cloning aren’t complicated! Not for organs and things! It’s not like they’re talking about, ooh, the dreadful war crimes we committed when we cloned a bunch of disposable people to die horribly in a war for us, in hushed tones like we talk about Nazi gas chambers or, like, the actions of Unit 731. It’s just cloning somebody a new heart! Presumably from their own tissue! We’re not even getting into the ethics of stem cell research or surrogate donors to carry the organs in until they’re fully grown. No one should care. I have no idea why Pershing doesn’t just go into the private sector.
#star wars#the mandalorian#slightly more bitching#wouldn't genetic engineering cover things like GMOs? how do all the farmers feel about that?#I just don't believe that they would outlaw all cloning and genetic engineering research#it's too broad a field#one they *must* have dipped into before to be able to spread across so many different planets#it feels too much like a convenient law put in place solely to needle Pershing for the sake of the plot#I can see ways that they could make it work in a logical and natural sense but they just have not put in the work#I don't buy it
8 notes
·
View notes
Text
Also preserved on our archive
Some interesting science analyzed
BY BROOKS LEITNER
Imagine lying back in an enclosed chamber where you bask for 90 minutes in a sea of pure oxygen at pressures two to three times that felt at sea level. This is the world of hyperbaric oxygen therapy (HBOT), a technology that’s been around for decades and is now being explored as a possible treatment for Long COVID.
"The silence on the inside is deafening at first,” says John M.,* who has undergone dozens of HBOT treatments for his persistent Long COVID symptoms. Fortunately, there is a television outside the chamber in view, and it is easy to communicate with the provider if needed. While the potential protocol is still being refined, patients may undergo up to 40 HBOT sessions to address some of the most problematic, lingering symptoms of this complex condition.
HBOT is a therapeutic process that has been widely used to treat such conditions as decompression sickness in scuba divers, carbon monoxide poisoning, and diabetic foot ulcers. In HBOT, the body is exposed to 100% oxygen, a significant increase from the 21% oxygen concentration we typically breathe. The therapy takes place in an enclosed chamber where the air pressure is elevated above normal levels. The combination of high-pressure and high-oxygen conditions enhances the amount of oxygen that can reach the body's tissues. The hope is that this therapy can provide the same relief and healing to people with Long COVID that it does for those with other conditions.
According to John M., HBOT was the first treatment that helped with his sleep and reduced his heart palpitations. “At one point after hospitalization, my Long COVID symptoms were so bad that I could barely walk or talk. HBOT was a great tool that really assisted with my recovery,” he said. John added that he hopes the medical community will achieve a better understanding of how HBOT can help relieve suffering for patients with Long COVID and that more research will increase access to this innovative therapy.
Does HBOT improve Long COVID symptoms? One key observation from the work of Inderjit Singh, MBChB, an assistant professor at Yale School of Medicine (YSM) specializing in pulmonary, critical care, and sleep medicine, is that Long COVID patients often experience debilitating fatigue. Based on Dr. Singh’s previous Long COVID research, the exhaustion is thought to be linked to the muscles’ inability to efficiently extract and utilize oxygen.
To picture how HBOT might work, you can think of your muscles as engines sputtering, struggling to get the fuel they need. If oxygen is the gas that fuels the muscles, it’s as if you are trying to complete your daily routine while the gas tank is running on “empty.” By aiming to directly address this oxygen utilization impairment, HBOT may be a potential solution.
A systematic review by researchers at the China Medical University Hospital noted that HBOT could tackle another major factor in the Long COVID puzzle: oxidative stress. This relates to the body's struggle to maintain balance when harmful molecules, known as free radicals, run amok, causing chronic inflammation.
Research co-authored by Sandra K. Wainwright, MD, medical director of the Center for Hyperbaric Medicine and Wound Healing at Greenwich Hospital in Connecticut, suggests that HBOT, with its high-oxygen environment, might dampen this chronic inflammation by improving mitochondrial activity and decreasing production of harmful molecules. Other potential benefits of HBOT in the treatment of Long COVID may include restoration of oxygen to oxygen-starved tissues, reduced production of inflammatory cytokines, and increased mobilization of hematopoietic stem cells—primary cells that transform into red blood cells, white blood cells, and platelets.
HBOT for Long COVID: Current and ongoing research Several small-scale reports have indicated that HBOT is safe for patients with Long COVID.
To address this question, a trial that followed the gold standard of modern medical research—a randomized, placebo-controlled, double-blind design—assigned 73 Long COVID patients to either receive 40 sessions of HBOT or a placebo of only 21% oxygen. The study observed positive changes in attention, sleep quality, pain symptoms, and energy levels among participants receiving HBOT. In a longitudinal follow-up study published in Scientific Reports, the authors at the Tel Aviv University found that clinical improvements persisted even one year after the last HBOT session was concluded. In a second study, the same authors focused on heart function, measured by an echocardiogram, and found a significant reduction in heart strain, known as global longitudinal strain, in patients who received HBOT.
In another study, 10 patients with Long COVID underwent 10 HBOT treatments over 12 consecutive days. Testing showed statistically significant improvement in fatigue and cognitive function. Meanwhile, an ongoing trial at the Karolinska Institute in Sweden has reported interim safety results wherein almost half of the Long COVID patients in the trial reported cough or chest discomfort during treatment. However, it was unclear whether HBOT exacerbated this symptom or if this adverse effect was due to the effort of participation by patients suffering from more severe Long COVID symptoms.
Is HBOT currently available as a treatment for Long COVID? For HBOT to become a mainstream treatment option for Long COVID, several critical priorities must be addressed. First, there is currently no established method for tailoring HBOT dosages to individual patients, so researchers must learn more about the specific features or symptoms that indicate potential benefits from HBOT. At the same time, we need to identify factors that may be associated with any adverse outcomes of HBOT. And finally, it’s important to determine how long these potentially beneficial effects last in a larger cohort. Will just a few HBOT trials be enough to restore patients to their baseline health, or will HBOT become a recurring component of their annual treatment regimen?
For now, HBOT remains an experimental therapy—and as such is not covered by insurance. This is a huge issue for patients because the therapy is expensive. According to Dr. Wainwright, a six-week course of therapy can run around $60,000. That’s a lot to pay for a therapy that’s still being studied. In the current completed studies, different treatment frequencies and intensities have been used, but it’s unclear how the treatment conditions affect the patient’s outcome.
“I have had some patients notice improvements after only 10 or 15 treatments, whereas some others need up to 45 treatments before they notice a difference,” notes Dr. Wainwright. “I think that HBOT is offering some promising results in many patients, but it is probably a strong adjunctive treatment to the other spectrum of things Long COVID patients should be doing, like participating in an exercise, rehab, and nutritional program.”
Dr. Singh notes that “a major challenge for research is the heterogeneity of Long COVID. It is hard to determine which symptoms to treat and enroll patients into trials based on them.”
Perhaps treatments that target multiple issues caused by Long COVID, like HBOT, may help overcome this challenge.
*Not his real name.
Brooks Leitner is an MD/PhD candidate at Yale School of Medicine.
The last word from Lisa Sanders, MD: Hyperbaric oxygen therapy (HBOT) is just one of the many existing treatments that are being looked at to treat Long COVID. We see this with many new diseases—trying to use a treatment that is effective in one set of diseases to treat another. And there is reason for optimism: We know that HBOT can deliver high levels of oxygen to tissues in need of oxygen. That’s why it’s used to treat soft tissue wounds. If reduced oxygen uptake is the cause of the devastating fatigue caused by Long COVID, as is suggested by many studies, then perhaps a better delivery system will help at least some patients.
Studies referenced:
bmcinfectdis.biomedcentral.com/articles/10.1186/s12879-023-08002-8
www.ncbi.nlm.nih.gov/pmc/articles/PMC8806311/
www.nature.com/articles/s41598-024-53091-3
www.nature.com/articles/s41598-022-15565-0
www.frontiersin.org/journals/medicine/articles/10.3389/fmed.2024.1354088/full
www.ncbi.nlm.nih.gov/pmc/articles/PMC11051078/#:~:text=Proposed%20Mechanism%20of%20HBOT%20o
#long covid#hbottherapy#HBOT#hyperbaric oxygen therapy#mask up#covid#pandemic#wear a mask#public health#covid 19#still coviding#wear a respirator#coronavirus#sars cov 2
20 notes
·
View notes
Photo
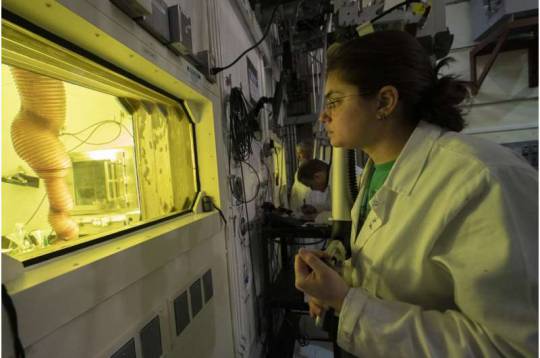
Researchers streamline production of purified actinium-225 isotope, for potential cancer treatments
Thanks to a recent upgrade to the medical isotope facilities at the U.S. Department of Energy's (DOE) Brookhaven National Laboratory, actinium-225 (Ac-225), an isotope that shows great promise for treating cancer, can now be produced, purified, and shipped ready for use directly from the Lab. The first shipment left Brookhaven in mid-March.
"This upgrade will streamline the overall production and distribution of Ac-225 to research centers by eliminating the need to ship material off site for final processing," said Cathy Cutler, Director of Medical Isotope Research & Production (MIRP) at Brookhaven Lab.
The promise of Ac-225 stems from the way this radioactive isotope decays. It emits alpha particles, which can deliver a lethal punch to cancerous cells over a short distance. Attaching Ac-225 to molecules that target tumors could potentially kill cancer cells without harming surrounding tissue. Ac-225 can also be used to generate bismuth-213, another alpha-emitter.
Read more.
16 notes
·
View notes
Text

Abnormal Vessel
Faulty vessels (vascular malformations) are a feature of many human diseases. Here, anomalous vessel cells grown in the lab from manipulated human pluripotent stem cells provides a model to study their characteristics and potential treatments
Read the published research article here
Image from work by Zihang Pan and Qiyang Yao, and colleagues
Department of Physiology and Pathophysiology, School of Basic Medical Sciences, State Key Laboratory of Vascular Homeostasis and Remodeling, Clinical Stem Cell Research Center, Peking University Third Hospital, Peking University, Beijing, China
Image originally published with a Creative Commons Attribution 4.0 International (CC BY 4.0)
Published in Cell Stem Cell, November 2024
You can also follow BPoD on Instagram, Twitter and Facebook
8 notes
·
View notes