#stellar evolution;;
Explore tagged Tumblr posts
Text
I need people to understand that Uranium is an eldritch horror
I'm not talking about radiation, or nuclear weapons, or anything that you can do with uranium, I mean its mere existence on Earth is a reminder of cosmic horrors on a scale you can barely conceive of.
When a nuclear power plant uses Uranium to boil water and spin steam turbines to keep the lights on, they're unleashing the fossilized energy of the destroyed heart of an undead star.
Allow me to elaborate:
In the beginning, there were hydrogen and helium. The primordial fires of the Big Bang produced almost exclusively the two lightest elements, along with a minuscule trace of lithium. It was a start, but that's not much to build a universe out of. Fortunately, the universe is full of element factories. We call them "stars".
Stars are powered by nuclear fusion, smooshing light elements together to make heavier elements, and releasing tremendous amounts of energy in the process, powering the star and making it shine. This goes on for millions to billions of years depending on the stars mass (although not how you might think, the bigger stars die young), the vast majority of that time spent fusing hydrogen into yet more helium. Eventually, the hydrogen in the core starts to run low, and if the star is massive enough it starts to fuse helium into carbon, then oxygen, neon, and so on up through successively heavier elements.
There's a limit to this though:
This chart shows how much energy is released if you were to create a given element/isotope out of the raw protons and neutrons that make it up, the Nuclear Binding Energy. Like in everyday life, rolling downhill on this chart releases energy. So, starting from hydrogen on the far left you can rapidly drop down to helium-4 releasing a ton of energy, and then from there to carbon-12 releasing a fair bit more.
But, at the bottom of this curve is iron-56, the most stable isotope. This is the most efficient way to pack protons and neutrons together, and forming it releases some energy. But once its formed, that's it. You're done. Its already the most stable, you can't get any more energy out of it, and in fact if you want to do anything to it and make it into a different element you're going to have to put energy in.
So, when a massive star's core starts to fill up with iron, the star is doomed. Iron is like ash from the nuclear fire that powers stars, its what's leftover when all the fuel is used up. When this happens, the core of the star isn't producing energy and can't support itself anymore and catastrophically collapses, triggering a supernova explosion which heralds the death of the star.
What kind of stellar-corpse gets left behind depends again on how massive the star is. If its really big, more than ~30 times the mass of the sun and its probably going to form a black hole and whatever was in there is gone for good. But if the star is a bit less massive, between 8-25 solar masses, it leaves behind a marginally less-destroyed corpse.
The immense weight of the outer layers of the star falling down on the core compresses the electrons of the atoms into their nuclei, resulting in them reacting with protons and turning them all into neutrons, which creates a big ball of almost pure neutrons a couple miles across, but containing the entire mass of the star's core, 3-5 sun's worth.
This is the undead heart of the former star: a neutron star.
If, like many stars, this one wasn't alone but had a sibling, it can end up with two neuron stars orbiting each other, like a pair of zombies acting out their former lives. If they get close enough together, their intense gravity warps the fabric of spacetime as they orbit, radiating away their orbital energy as gravitational waves, slowing them down and bringing them closer together until they eventually collide.
The resulting kilonova explosion destroys both of the neutron stars, most likely rendering the majority of what's left into a black hole, but not before throwing out a massive cloud of neutron-rich shrapnel. This elder-god blood-splatter from the collision of the undead hearts of former stars contains massive nuclei with hundreds to thousands of neutrons, the vast majority of which are heinously unstable and decay away in milliseconds or less. Most of their decay products are also unstable and decay quickly as well, eventually falling apart into small enough clusters to be stable and drift off into the universe becoming part of the cosmic dust between the stars.
However,
Some of the resulting massive elements are merely almost stable. They would like to decay, but for quantum-physics reasons decaying is hard and slow for them, so they stick around much longer than you might expect. Uranium is one such element, with U-238 having a half-life of around 4.5 billion years, about the same as the age of the Earth, and its spicier cousin U-235 which still has a respectable 200 million year half life.
These almost-stable isotopes were only able to be created in the fiery excess of energy in a neutron star collision, and are the only ones that stick around long enough to carry a fraction of that energy to the era where hairless apes could figure out that a particular black rock made of them was emitting some kind of invisible energy.
So as I said at the beginning, Uranium is significant because it stores the fossilized energy of the destroyed heart of an undead star, and we can release that energy at will if we set it up just right.
When you say it like that, is it any shock that the energy in question will melt your face off and rot your bones from the inside if you stay near it too long?
#nuclear physics#nucleosynthesis#stellar nucleosynthesis#neutron star#uranium#radiation#supernova#kilonova#cosmic horror#physics#science#space#astrophysics#stars#stellar evolution
972 notes
·
View notes
Text
#more ears under the cut#sprigatito#welcome to GENERATION NINE. the generation i've been dreading for the entire existence of this blog#it'll be alright. i'll find ways to get around any problems i might have. i'm pretty confident. i don't think it's gonna be as bad as the#dread tells me it's gonna be. like… look at this face! sprigatito has gotta be one of the best fuckin starters of all time#NOT TO MENTION it stands up!!! in its evolutions!! how great is that??#everyone was so mad but i was rooting for it the whole time. i was BEGGING this cat to stand up. AND IT DID!!!! AND I LOVE IT#IT'S SUCH A WONDERFUL EVOLUTION TOO!!! the fucking magician aesthetic is STELLAR#i love it so much. holy fucking bingle. can't believe game freak popped off so hard with the FIRST pokémon in the paldea dex#they have such pretty eyes. i dunno why they're pogging but it's probably because we finally got to them
235 notes
·
View notes
Text
Star-Birthing Shock Waves
Although the space between stars is empty by terrestrial standards, it's not devoid of matter. There's a scattering of cold gas and dust, pocked by areas known as prestellar cores with densities of a few thousand particles per cubic centimeter. (Image credit: NASA/ESA/CSA/STSCI/K. Pontoppidan/A. Pagan; see also Physics Today) Read the full article
#astronomy#astrophysics#flow visualization#fluid dynamics#physics#science#shock wave#stellar evolution
89 notes
·
View notes
Text
Stellar Evolution: An In-Depth Journey into the Lifecycle of Stars
Stellar evolution tells the fascinating story of how stars are born, change, and eventually die. It's a process that takes billions of years and has a big impact on the universe and even life itself. In this article, we'll explore the journey stars take throughout their lives, looking at the different stages they go through and what causes them. Let's dive into the details of stellar evolution and understand how these celestial objects live and evolve.
Formation of Stars: Birth from Cosmic Clouds
Stars begin their journey in huge clouds of gas and dust called nebulae. These clouds are like giant factories that have all the ingredients needed to make a star. When something like a shockwave or a disturbance happens, parts of the nebula start to get denser. This denser area is where a new star begins to form. It starts as what we call a protostar. As more and more material gets pulled in by gravity, the protostar grows bigger and denser. Eventually, it becomes so dense and hot that nuclear fusion starts happening in its core. This is when the star "turns on" and starts shining.
The Main Sequence Phase: A Star's Brightest Period
When a star begins nuclear fusion, it enters its main phase, which is its brightest time. In this phase, hydrogen atoms in the star's center combine to form helium, releasing a lot of energy. This energy pushes outward, balancing the star's gravity and, keeping it stable. How long this phase lasts depends on how big the star is. Bigger stars go through this phase faster than smaller ones.
Stellar Metamorphosis: Beyond the Main Sequence
Once a star runs out of its hydrogen fuel in the center, it starts changing and moves away from its main form. What happens next depends on how big the star is to begin with. Different-sized stars go through different changes, each with its own special things happening.
Red Giant Phase: The Stellar Expansion
When stars like our Sun start running out of hydrogen, they enter a phase called the red giant phase. At this point, the star gets bigger and expands outward, but its core gets smaller and hotter. This makes the outer layers of the star glow red. Inside the star, helium starts fusing together, creating even more energy. This red giant phase shows that the star is getting closer to the end of its life.
Planetary Nebulae and White Dwarfs
When a star becomes a red giant, it swells up and eventually sheds its outer layers into space. This creates a beautiful cloud called a planetary nebula. What's left behind is the core of the star, which becomes a white dwarf. A white dwarf is a small, dense object about the size of Earth. It's made mostly of a special kind of matter called electron-degenerate matter. Over a very long time, white dwarfs cool down and become less and less bright. Eventually, they become invisible and mark the end of the star's life for smaller stars.
Supernovae and Neutron Stars: The Fate of Massive Stars
When big stars run out of fuel, they collapse suddenly, causing a massive explosion called a supernova. This explosion is so bright that it can outshine entire galaxies. During this explosion, heavy elements made inside the star's core are scattered into space, which later helps in forming new stars.
After a supernova, the core of the big star can shrink even more, forming a neutron star. Neutron stars are very small, like cities, and are made mostly of tightly packed neutrons. They have strong magnetic fields and spin very fast, leading to interesting things like pulsars and magnetars.
Black Holes: The Mysterious End
When really big stars run out of fuel, something incredible happens. They collapse under their own gravity, squeezing down into a tiny, super-dense point. This creates something called a black hole. Black holes are mighty, with gravity so strong that not even light can escape from them. They're like cosmic vacuum cleaners, sucking in everything around them. Black holes are mysterious and fascinating, and they impact how galaxies work, shaping the universe in a really big way.
Conclusion
Stellar evolution is like a never-ending story of how stars are born and eventually fade away. It starts with the peaceful formation of baby stars in cloudy areas of space called stellar nurseries. Then, stars grow and shine brightly during their main life phase. But as they run out of fuel, some stars become red giants, swelling in size. Eventually, smaller stars become white dwarfs, while bigger ones explode into supernovae, scattering elements into space. This process helps shape the universe, showing us how everything in space is connected. By studying stars, we learn more about where we come from and our role in the vast cosmos.
FAQs
Who came up with stellar evolution? In the early 1900s, two astronomers named Ejnar Hertzsprung and Henry Norris Russell found a helpful way to compare different stars. They called it the Hertzsprung-Russell (H-R) Diagram. It's like a big chart where scientists can see how stars compare to each other based on their brightness and temperature. This diagram has been super useful in understanding more about stars and how they work.
What are the elements of stellar evolution? These are some of the building blocks found in space i.e. hydrogen, carbon, nitrogen, oxygen, phosphorus, sulfur, chlorine, sodium, magnesium, potassium, calcium, and iron.
What is the lifetime of a star? Very big stars burn through their fuel fast, so they don't live very long, maybe just a few hundred thousand years. But smaller stars use their fuel more slowly, so they can shine for billions of years. However, no matter how big or small a star is, eventually, it starts running out of hydrogen, which is what keeps it shining.
What is the stellar life cycle? Stars go through a cycle of being born, burning fuel, and spreading out material when they die. This cycle is ongoing and helps create elements that fill the universe. Depending on how much stuff a star has (its mass), it follows a different path in its life.
What are the 7 types of stars? Stars come in different types, and scientists classify them based on how hot they are. There are seven groups, starting with the hottest and ending with the coolest. They are named O, B, A, F, G, K, and M stars. O stars are the hottest and brightest, while M stars are the coolest and dimmest.
Read more
#science#astronomy#space#education#universe#physics#sol#sunspot#sunspot number#nebula#astrophotography#black holes#stars#stellar evolution#supernova#outer space#sky#night sky#cosmology
23 notes
·
View notes
Link
Astronomers may have for the first time witnessed a sun-like star devouring a planet, shedding light on the fate that will befall Earth in about four billion years when our dying sun swells to engulf our world, a new study finds.
By analyzing countless stars during various stages of their evolution, astronomers have discovered that as our sun and stars like it near the ends of their lives, they begin to exhaust their primary source of fuel, the hydrogen near their cores. This leads their cores to contract and their outer shells to expand and cool. During this "red giant" phase, these stars may billow out anywhere from 100 to 1,000 times their original diameter, swallowing closely orbiting planets.
"We know that this must happen to all planets that are orbiting at distances smaller than that of the Earth, but it was considered extremely challenging to provide experimental evidence for this," study lead author Kishalay De, an astrophysicist at the Massachusetts Institute of Technology, told Space.com.
Continue Reading
124 notes
·
View notes
Text
Astronomy 101: Stellar Life Cycles
This is the first in a series of posts I plan to make explaining basic astronomy concepts. I am not a professional astronomer, but I have a B.S. in Astronomy and Astrophysics, experience performing professional astronomical research (primarily photometry), and a lifelong amateur interest in astronomy. I am committed to accuracy in these posts (though some simplification is necessary) and welcome questions/comments.
(Image source)
Nearly every aspect of a star’s evolution is governed by a single characteristic: the star’s mass. Stellar masses are enormous, and so astronomers find it more convenient to measure them in solar masses (M☉, M being mass, and ☉ being the astronomical symbol for the sun, pronounced “M-sol”). 1 M☉ is equivalent to the sun’s mass; 2 M☉ is twice the sun’s mass; 0.5 M☉ is half the sun’s mass; and so on. Our sun masses approximately two quintillion kilograms—that’s 30 zeroes!—so it’s easy to see why this convention makes comparisons between stars easier.
Stars are composed primarily of hydrogen, with non-trivial amounts of helium (ex. our sun is about 74% hydrogen and 25% helium). They fuse the elements within themselves to produce an outward pressure from radiation that balances the inward pressure imposed by their own gravity (gravity wants to collapse the star; radiation keeps this from happening). The lowest stellar mass that can sustain a fusion reaction is theorized to be 0.08 M☉. It’s not physically possible for a star to be smaller than this. On the other end, the most massive stars range up to 250 M☉. (It should be noted that as the universe ages, stars are getting smaller; it is hypothesized that new stars cannot exceed 150 M☉.)
Stars are born from the gravitational collapse of giant molecular clouds (GMC), which are large, cold, and relatively dense pockets of interstellar gas. It’s theorized that several mechanisms can trigger GMC collapse, including the shock wave from nearby supernovae, collisions with other clouds, or passing through the spiral arm of a galaxy (which is denser than other areas). These all disrupt the balance within the cloud. As it collapses, it begins to fragment, forming individual stars. One GMC will produce numerous protostars.
The protostar phase lasts a relatively short time, about 500,000 years for stars the size of the sun. During this time, the protostar continues to accrete mass from the surrounding nebula (its own pocket of the GMC). Gravitational collapse is countered by gas pressure and magnetic pressure rather than by nuclear fusion as the protostar grows. Eventually, this is not sufficient and the protostar begins to collapse and enters a new phase: it reaches sufficient density to ignite nuclear fusion in its core, and becomes a proper star.
Initial fusion in the core fuses hydrogen into helium. It may seem counter-intuitive, but the lower a star’s mass, the slower it burns through its fuel and the longer it remains in this phase of its life. Massive stars are not only much hotter than small stars, but they need to produce more energy to stave off gravitational collapse. This causes them to burn through their supply of hydrogen very quickly. Very low mass stars born early in our universe have not existed long enough to burn through their hydrogen supplies. Models predict stars of 0.1 M☉ will take 6-12 TRILLION years to exhaust their hydrogen, and our universe is only 13.8 billion years old. These stars are barely getting started. These stars, known as red dwarfs, will never become red giants. Instead, they will continuously mix their original hydrogen stores with their newly produced helium until the entire star is composed of helium, at which point fusion will shut down. As they reach the end, they are predicted to become blue dwarfs, and then eventually white dwarfs. No blue dwarfs presently exist because the universe is still young.
Stars more like our sun, ranging from 0.6 to 8 M☉, have lives more consistent with what many people are taught in high school science classes. When these stars exhaust the hydrogen in their core, they still contain a large volume of hydrogen in their outer layers. They enter the subgiant phase, where they fuse hydrogen in a shell surrounding the helium core, and the star begins to expand and cool. This new helium adds to the volume of the core, the hydrogen-fusing shell moves outwards. This phase lasts several million to two billion years, again with lower-mass stars spending longer in this phase.
(You may have read that the sun will get hotter as it ages. This is true, and it’s due to the fact that as the fraction of hydrogen in the core decreases, the core temperature and rate of fusion increase. The sun will contract and become hotter as it approaches the subgiant phase. It’s theorized that this will make the Earth uninhabitable in about one billion years. However, the effects of plate tectonics and specifically the subduction of water from the oceans and the gradual slowing of tectonic activity is likely to play as large a role if not larger.)
This hydrogen-burning shell supports the star against gravitational collapse until the helium core grows too large. The core will contract, and the outer layers will further expand and cool as the star becomes a red giant. In the case of our sun, its radius will expand beyond the orbit of the Earth, in about six billion years. Shell burning continues at an increasing rate, but the star is no longer in equilibrium, and the core continues to contract even as it increases in mass, until eventually the star begins fusing helium into carbon. This can happen very suddenly for stars at the lower end of this mass range, or more gradually for more massive stars. The growth of the star can also temporarily create new habitable zones, primarily for the moons of gas giants, lasting several hundred million years.
Stars in this mass range do not have sufficient mass to fuse carbon, and so when the helium is largely consumed in the core, the core begins to collapse, and this time, only a strange state of matter called electron degeneracy will stop it. The Pauli Exclusion principle states that no two electrons can occupy identical states, and electron degeneracy occurs when a star has collapsed to the point that all the electrons of the star have been forced to occupy all the lowest-available energy states within the atomic structure of the star. The energy of the gravitational collapse is insufficient to overcome this electron pressure. White dwarfs are very small and incomprehensibly dense; similar to the mass of the sun, they are similar in size to the Earth. A teaspoon of white dwarf would weigh about 15 tons. Fusion has ceased, and the star is an inert ball of slowly cooling degenerate matter.
This is the end of the road for stars in this mass range, which is most of the stars that presently exist in the universe. Very, very slowly, solo white dwarfs will continue to cool. White dwarfs are predicted to outlast the lifespan of galaxies as structures in our universe. They may still be extant when enough time has passed for proton decay to become a significant force and ultimately devour the white dwarf. If proton decay takes significantly longer than predicted or turns out to not exist, the ultimate fate of a white dwarf is to be devoured by a black hole in the very, very, very far future of our universe when other forms of stars have all ceased to exist. (The black hole era is predicted to begin 10^43 years after the Big Bang, but it’s dependent on a lot of poorly-understood factors. Safe to say, this is not anything anyone needs to worry about.)
More massive stars have a more interesting if shorter life. They blow through their hydrogen quickly, ranging from 1 billion years to as few as 10,000 years. (You can calculate here.) As these stars enter their supergiant phase, they lose mass rapidly due to strong stellar winds. These stars do possess enough mass for more complex fusion reactions, and will gradually develop shells fusing various elements, and finally in the core, ultimately producing iron via fusion. All elements aside from hydrogen, some helium, and small amounts of lithium and beryllium are created by stellar nuclear fusion. Iron, however, is special, because it is the lightest element on the periodic table that requires more energy to fuse than it produces through fusion. A star attempting to fuse iron is going into an energy deficit, rather than producing energy through radiation that can stave off gravitational collapse. A star cannot fuse iron.
The final days of such stars come on quickly. The core turns to iron within a few hundred years, so rapidly that there is little change in the outward appearance of the star, which continues shell burning right up to the end. Eventually, this iron core reaches the effective Chandrasekhar mass, a little larger than the mass of our sun, and the core can no longer support itself. The core collapses.
It’s easy to say the star then explodes in a supernova, but the truth is more complicated. A supernova is triggered by the release of gravitational potential energy from the core collapse. If this is insufficient, instead the core becomes a neutron star or a black hole with very little fanfare. Some supernovae (pair-instability supernova) do not leave behind any stellar remnant despite originating from supermassive stars. Other supernovae do not involve supermassive stars, but white dwarfs in binary pairs accreting matter from their stellar companion.
The core collapse takes less than a quarter of a second. Within a few hours, the shockwave reaches the surface of the star, which will brighten tremendously over the next few months. At peak, supernovae can outshine their host galaxies. Perhaps the most famous supernova, SN 1987A, was visible to the naked eye and wasn’t even located in our own galaxy, but in a satellite galaxy. This shockwave is incredibly high-energy, to the point that it not only ejects outer material from the star, but ignites fusion reactions in that material. Here, we have an excess of energy, and nuclear fusion reactions that require more energy than they create are possible. This is how all elements heavier than iron are created.
The core becomes a neutron star or a black hole. Which depends on a large number of variables, perhaps strangely only two of which are core mass and stellar mass. A neutron star is similar to a white dwarf, in that it is composed of degenerate matter, but in this case electron degeneracy was not strong enough and it is held up by neutron degeneracy. In this state, electrons have combined with protons to produce neutrons, leaving behind a highly dense star composed of nuclear matter. While a neutron star is again slightly more massive than our sun, it has a diameter of only about 20 kilometers. A teaspoon of neutron star weights approximately 4 billion tons. Many neutron stars rotate rapidly, giving off jets of radiation from their poles. These stars are known as pulsars. The fate of neutron stars is similar to that of white dwarfs; they will eventually merge into black holes in the far distant future.
Other stars become black holes. In this case, even neutron degeneracy cannot stave off gravitational collapse, and the core collapses into a singularity. A singularity is a poorly-understood object where spacetime curvature becomes infinite. We can study singularities mathematically, but we can’t observe one, because it is hidden behind an event horizon—a boundary surrounding the black hole where gravity is too strong for even light to escape. And if light can’t escape, we can’t observe anything inside the event horizon. Our current understand of physics also breaks down here; our theories of gravity aren’t sufficient to fully understand singularities. We can, however, study event horizons, accretion disks (matter falling into the black hole), and jets of particles and radiation some black holes exhibit. This has given us a good understanding of the external properties and behavior of black holes, and we detect them based on these characteristics and the effects they have on other objects (ex. an orbiting companion star).
So if everything eventually becomes part of a black hole, what happens to a black hole? The answer is they evaporate through a process called Hawking radiation. Essentially, according to quantum mechanics, space has a quality called vacuum energy that causes virtual particle pairs to continuously pop in and out of existence. The pair consists of one particle and one anti-particle, which annihilate each other and return the energy of their creation to the vacuum. Therefore, no energy is created or destroyed. However, every so often, one of these pairs pops into existence with one particle on the outer side of the event horizon, able to escape, and the other trapped inside the event horizon. The virtual particle that escapes becomes a real particle, and that energy has to come from somewhere. It takes it from the black hole. Because mass and energy are functionally equivalent, this drains a tiny amount of mass from the black hole. (Please note: this is a dramatic oversimplification, but gets the basic idea across.) On long enough timelines, this will cause even the largest of black holes to evaporate. Unlike just about everything else involving stars, smaller black holes evaporate more quickly than large ones.
#astronomy#stellar evolution#tbh every stage of a star's life could be a post on its own#and for simplicity i've eliminated discussion of several things that impact stellar evolution like metallicity#it's already a long post lol#astropigeon#also this is a strange way to learn Tumblr doesn't support subscripts
71 notes
·
View notes
Text
hello astronomy tumblr!!😁
so erm. im participating in science olympiad and i have to study stellar evolution (i think star formation specifically but i assume ill have to know the other stuff as well) and exoplanets but uhh i have NOT studied SHIT all year and regionals are in a month so. if anyone is knowledgeable in this area. please i need help i am begging anything helps if you dont know anything then tag like a friend who might idfk please i am desperate🙏🙏🙏
#science#astronomy#stellar evolution#star formation#stars#space#exoplanets#planets#science olympiad#help please#spread the word gang . i am going to humiliate myself guys please
14 notes
·
View notes
Text
You know how Evoloution is the Youthful Naturalist's ambition?
I wonder if it'd be possible to rewrite it as if the Naturalist was a player doing a preset ambition. Like how someone plays, say, the "Bag a legend" ambtion etc.
I'll probably work on this the moment I get time because it seems like a fun project tbh.
#hahaheart1#fallen london#youthful naturalist#I have yet to finish evolution BUT#BUT#I am having a stellar time#And I've heard it's been called his ambition so like#We're the main companion? Very cool#I'd have to infer a lot of things from what's implied like what he's up to when we're doing other things#but it can be done#I will also have to make assumption on certain things especially since he'd be a POSI at the very least considering all the friends he has#and achivements he's completed#anywho#fun writing project#though I may need help on it haha#I also wonder if it's been done before#surely someone has done something like this yes?#eh#if I don't find anything then I'm good to go™
4 notes
·
View notes
Text
dailymotion
Astrology is total crap, there's no getting around that. Nobody explained why in a more effective way than astronomer Carl Sagan.
Earth's precession (a type of natural wobble) and the proper motion of the stars themselves have changed the way we Earth people view the night sky in the 3,000 or so years since astrology was concocted. In fact, there are actually 13 rather than 12 constellations the sun appears in throughout the course of the year. And the dates the sun enters and leaves those constellations of the zodiac can be weeks off from what astrologers tell you.
And just because stars appear close to each other from our perspective on Earth does not mean they are actually close to each other in reality.
Here is a diagram of the brighter stars in Gemini. The ones labeled range from Pollux (Beta Geminorum) which is an estimated 34 light years away to Mekbuta (Zeta Geminorum) which is about 1200 light years away. BTW Wasat (Delta Geminorum), which they forgot to number, is 60 light years away.
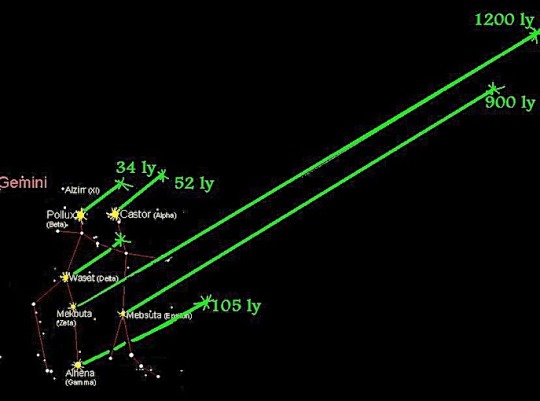
So a constellation is not some sort of special conclave of stars, it's a random collection which simply appears in the same patch of the sky as seen from Earth. The same is true of the other constellations – not just Gemini.
So your destiny has nothing to do with the changing positions of the stars and planets. Sorry! But we do all share a far more concrete connection to the universe.
All the elements except for hydrogen, helium, and (a tiny bit of) lithium were created inside of stars which blew up or ejected much of their contents billions of years ago.
I edited together a few sentences from the companion volume for the COSMOS series which the vid above comes from.

So yeah, the universe is inside all of us. That's our true connection to the cosmos.
13 notes
·
View notes
Text
Bones did togachako so good love wins
#I didn’t enjoy the arc at all when in the manga#but tbh the way it was animated was stellar and really highlighted Ochaco’s quirk evolution plus togas mindset#it was gorgeous. I cried. even made me mroe ‘okay’ with her dying#can’t believe that this was the season finale though#it’s okay I’m double deku-Saturday-ing by seeing the you’re next movie today#can’t wait to weeb out with other losers#one of my favorite memories is still seeing heroes rising in theaters#last movie before lockdown#right when I got REALLY into bnha#and it was a packed theater where everyone was in on the jokes#and everyone was so excited#god I love that vibe
4 notes
·
View notes
Text
does anyone else use their imagination to visualise what you’re reading about so completely that finishing reading feels like waking up
#was reading about stellar evolution this morning#then looked up and suddenly was confronted with the realisation#that I wasn’t in fact watching a star leave the main sequence#personal
8 notes
·
View notes
Text
Stellar evolution process(part 1)
Stellar evolution is the process in which a star changes over time. this can range from a few million years to trillions of years depending on the mass of the star. All stars begin as collapsing clouds of dust and gas called nebulae or molecular clouds. These are usually about 100 Light years (9.5 x 10^14 km) across.
As it collapses the cloud breaks into fragments, which release energy as heat which then causes the temperature and pressure to increase and the fragments condense into a ball of flaming gas called a protostar. The protostar continues to grow by accumulating gas and dust and becoming a main sequence star as it reaches its final mass.
Protostars with masses less than roughly 1.6 x 10^29 kg never reach temperatures high enough for nuclear fusion of hydrogen to begin (which is what fuels stars). These are known as brown dwarfs.
Usually when the star begins to run out of hydrogen it begins to evolve off the main sequence and helium fusion can occur (depending on the stars mass)
Stars such as red dwarfs ( which are one of the most common types of stars) gradually increase in temperature and pressure and collapse to become white dwarfs. These will not become red giants until the whole star reaches a convection zone (where the star is unstable due to convection)
More massive stars (like our sun) do expand into red giants but the helium cores are not massive enough to reach the temperature for helium fusion
Massive stars already have a core large enough that helium ignition can occur. Eventually the core will become massive enough that it will not be able to support itself and will collapse into a neutron star or a black hole.
4 notes
·
View notes
Text
Interview: Aaron Lee Tasjan
#Interview: @aaronleetasjan1 The beloved #indie #troubadour discusses his #tour behind his new album STELLAR EVOLUTION and his forthcoming #LA show @BigFeatPR @blueelanrecords @moroccan_lounge #newmusic #livemusic #folk #rock #pop #musicrecommendation
Aaron Lee Tasjan is one of those artists that your favorite artists gush over. A masterful songsmith, able to traverse genres and styles with ease, he’s also an empowering voice in the LGBTQ community and activist who uses his voice to create safe spaces for those who have felt like outsiders in their communities. On his latest album Stellar Evolution, Tasjan mines his own past struggles as well…
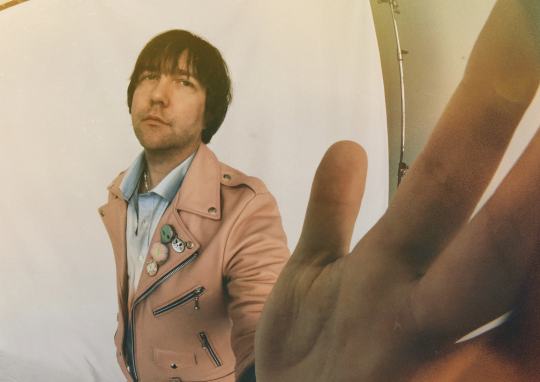
View On WordPress
#Aaron lee tasjan#folk#indie#indie rock#interview#Moroccan Lounge#New Music#pop#rock#singer songwriter#Stellar Evolution
2 notes
·
View notes
Text
Simeis 147
Sometimes known as the Spaghetti Nebula, Simeis 147 is the remnant of a supernova that occurred 40,000 years ago. The glowing filaments of this composite image show hydrogen and oxygen in red and blue, respectively. (Image credit: S. Vetter; via APOD) Read the full article
#astrophysics#fluid dynamics#instability#physics#science#shockwave#stellar evolution#supernova#turbulence
137 notes
·
View notes
Text
stars are like my least favorite astronomical object (so sorry, controversial i know) and so i’m only just sitting here realizing that like stars, as a general topic, is so complex wtf. stellar classification all on its own is a whole thing with many different ways to categorize stars (harvard spectral classes, mk luminosity classes, HR diagram, stellar populations, etc), nevermind stellar evolution, including formation and death, and then stellar spectra and the physics behind that, and then the like actual physical anatomy of the sun and heliophysics, space weather, etc? and the nuclear physics behind fusion? what the fuck man
#ive always been more into cosmology and that mostly just deals with galaxies#i love galaxies !#i took a whole class on stars and it was when i was at rock bottom mentally so i really did not retain anything from it#but i do fucking know we never learned how to actually classify stars#like the basic spectral classes#so sitting here throughout today trying to relearn and reteach myself starting from 0 its like wait i never formally learned this anyway??#most of the class was on stellar evolution 😩😩#like idk i think stars are theoretically cool like oh big giant massive star go boom supernova wow so cool#but like theres. so fucking much physics behind all that its really quite snore actually. idk i think stars are overrated 💁#fucking pp chain vs cno cycle#UGH add nuclear physics onto this list too#meanwhile my whole class about galaxies was just like tee hee. Galaxies#brot posts#astro posting
3 notes
·
View notes
Text
anyway martyn inthelittlewood is a high mass star whos story is one of stellar evolution. this is insanely rambley so im going to put it behind a readmore lolz
main sequence. he's normal hes fine he is gaming, hanging out, bonding with scott, etc etc this is just normalguy state.
turning yellow bumps him to red supergiant. he's going after greens to try and get that little time back to tide him over, and he's a threat. more obviously a threat this time than when he's red imo everyone was kinda scrambling on red but when martyn was yellow he was hunting. kills scott, yadda yadda,
going red is the supernova event (thats my cats name btw) its explosive, and in scar's words it starts the "red wars" that man was on stm btw but anyway he's unpredictable, the outcomes are either a neutron star or a black hole. the chance of betrayal is there, but everything is literally up to chance (in cosmology it isnt, there's this thing called the Oppenheimer-Volkoff limit or however you spell it but listen i live for dramatics)
the only two outcomes we know of after supernova are as follows: the star is beneath the Oppenheimer-Volkoff limit (2-3 solar masses) it becomes a neutron star and is stopped from further collapse by neutron pressure. if the limit is exceeded, however, this results in a black hole
something something symbolism, both results apply to martyn as a black hole destroys literally everything around it and bends space time (thats so fucking insane btw??? IT BENDS LIGHT in SPACE im soooo) and a neutron star is self sustaining at the cost of becoming unchanging.
i have no conclusion for you other than if i got anything wrong about stars let me know i aint an astrophys kid :thumbsup:
#crashposting#martyn inthelittlewood#limited life martyn#inthelittlewood#limited life#limited life spoilers#martyn traffic series#martyn limited life#fuck bro idk#i like stellar evolution#its the bio nerd in me
18 notes
·
View notes