#recombinant dna
Explore tagged Tumblr posts
Text
The Boston Globe's view of the response at MIT to the decision by the lay members of the Cambridge Experimentation Review Board to allow recombinant DNA experiments to continue in the city.
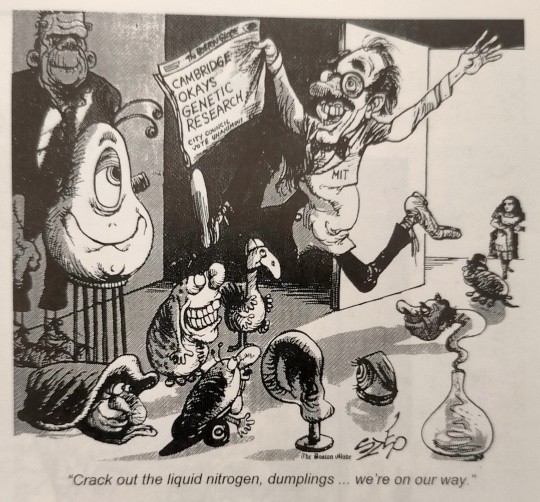
"Frankenstein's Footsteps: Science, Genetics and Popular Culture" - Jon Turney
#book quotes#frankenstein's footsteps#jon turney#nonfiction#boston globe#mit#massachusetts institute of technology#cambridge experimentation review board#recombinant dna#science experiments#liquid nitrogen#genetic research#political cartoon
1 note
·
View note
Text
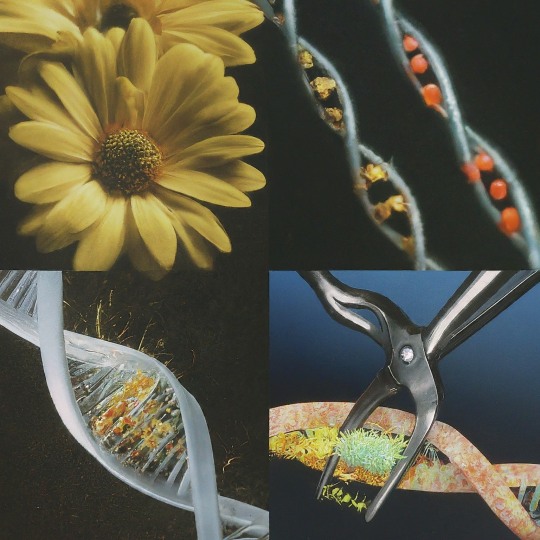
A snip, a splice : Power of rDNA Technology
Deoxyribonucleic acid (DNA), the blueprint of life, holds the secrets to the intricate workings of every living organism. But what if we could manipulate this blueprint, adding, removing, or tweaking its code? This revolutionary concept forms the core of recombinant DNA (rDNA) technology, a powerful tool that has transformed biology and medicine.
The story starts in the early 1970s with two brilliant scientists; Stanley Cohen at Stanford University and Herbert Boyer at the University of California, San Francisco. Cohen, a microbiologist, had been studying plasmids – small circular DNA molecules found in bacteria. Boyer, a biochemist, was an expert on restriction enzymes – molecular scissors that could cut DNA at specific sequences. Their collaboration proved groundbreaking. They envisioned combining these tools to create the first ever recombinant DNA molecule. Cohen provided the plasmids, which would act as vectors to carry foreign DNA into host cells. Boyer, on the other hand, used restriction enzymes to cut both the plasmid and the desired foreign DNA, allowing them to be pieced together. Through meticulous experimentation, they successfully created the first recombinant DNA molecule, forever altering the course of biology.
Cohen and Boyer's work wouldn't have been possible without the earlier discoveries of restriction enzymes. These "molecular scissors" were independently identified by three separate research groups in the 1960s. Werner Arber in Switzerland, along with Hamilton Smith and Daniel Nathans in the US, unraveled the role of restriction enzymes in bacterial defense mechanisms. These enzymes helped bacteria defend against invading viruses by cutting up their foreign DNA. Recognizing the potential of these "genetic scalpels," the groundwork was laid for their application in rDNA technology.
Here's a simplified breakdown of the rDNA process:
Isolation of DNA: The journey starts with isolating DNA from a donor organism.
Cleavage with Restriction Enzymes: Specific enzymes cut the DNA at defined sequences.
Selection of Vector: A carrier molecule (often a plasmid) is chosen to transport the recombinant DNA.
Ligation: The DNA fragments and vector are stitched together using DNA ligase, an enzyme.
Transformation: The recombinant DNA enters a host cell (usually bacteria or yeast).
Selection and Expression: The transformed cells are selected, and the gene of interest is expressed, leading to the desired protein production.
Since its inception, rDNA technology has played a pivotal role in several groundbreaking advancements. Let's take a whirlwind tour through some of the most significant moments in R-DNA history:
1978: Birth of Insulin on the Factory Floor: Scientists achieved a feat of genetic engineering by using R-DNA to produce human insulin in bacteria. This marked a turning point for diabetics, offering a readily available and more consistent source of this life-saving hormone.
1980s: Gene Wars and the Rise of GMOs: The 1980s saw the development of genetically modified organisms (GMOs). Plants were engineered with genes for insect resistance or herbicide tolerance, sparking debates about the safety and ethics of this technology. R-DNA research continues to be at the forefront of discussions regarding genetically modified foods.
1990s: The Human Genome Project Sets Sail: This ambitious international project aimed to sequence the entire human genome. R-DNA techniques played a crucial role in deciphering the 3 billion letters of our genetic code, opening doors for personalized medicine and a deeper understanding of human health and disease.
2000s: Gene Therapy Takes Center Stage: The first successful gene therapy trials for inherited diseases like severe combined immunodeficiency (SCID) took place. R-DNA technology offered a glimmer of hope for treating genetic disorders by introducing healthy genes to replace defective ones.
2010s and Beyond: CRISPR Takes Over: The emergence of CRISPR-Cas9, a revolutionary gene editing tool based on R-DNA principles, has ushered in a new era of genetic manipulation. With unprecedented precision, scientists can now edit genes in various organisms, holding immense potential for gene therapy, crop improvement, and even the eradication of diseases.
But with great power comes great responsibility, and R-DNA raises a host of ethical concerns.Tinkering with the building blocks of life carries the risk of unintended consequences. Engineered genes could escape and disrupt ecosystems, or modified organisms could have unforeseen health effects. The ability to edit human genes opens the door to designer babies, raising questions about social equity and the potential misuse of the technology for eugenics.
Who Controls the Tools? Access to R-DNA technology could be restricted to wealthy nations or corporations, exacerbating existing inequalities. Biosecurity is also a concern, as the technology could be misused for bioterrorism. Creating entirely new organisms forces us to confront what it means to be "natural." Should we modify plants and animals for human benefit, or preserve their original forms? R-DNA technology is a powerful tool, and we must have open discussions about its ethical implications. Scientists, policymakers, and the public all need to be involved in shaping the future of this technology. As we move forward, open dialogue and collaboration between scientists, policymakers, and the public are crucial to ensure the safe and ethical application of this powerful technology.
The journey of rDNA technology is a testament to human ingenuity and its potential to reshape our world. From decoding the secrets of life to creating solutions for healthcare, agriculture, and beyond, rDNA technology continues to evolve, promising a future filled with exciting possibilities.
#science sculpt#life science#science#molecular biology#biology#biotechnology#artists on tumblr#dna#double helix#genetics#recombinant#genetic engineering#insulin#research#education#learning#academics#scientific research#scientific illustration#medical science#scifi#daily dose of science#scientific advancements#scientific tools#medical school
16 notes
·
View notes
Text
Three-quarters of my kids’ grandparents have brown hair and brown eyes.
Naturally, I have a blonde kid and a blue-eyed kid.
Because genetics.
5 notes
·
View notes
Text
youtube
#HRD testing#ovarian cancer#homologous recombination deficiency#personalized medicine#PARP inhibitors#DNA repair#targeted therapy#genomic testing#oncology research#molecular oncology#standardized testing#cancer diagnostics#tumor biomarkers#cancer genomics#harmonization#precision oncology#clinical outcomes#cancer therapy#patient care#genomic assays.#Youtube
0 notes
Text
What is Biotech? Unlocking the Power of Biology
“Unlocking the Power of Biology: Biotech Innovations Transforming Our World” Biotechnology: Revolutionizing Industries and Improving Lives Biotech, short for biotechnology, is a rapidly evolving field that combines biology, genetics, and engineering to develop innovative solutions for various industries and aspects of our lives. From healthcare and agriculture to environment and energy, biotech…

View On WordPress
#Innovation#agriculture#biofuels#bioinformatics#biology#biomanufacturing#biomaterials#bioprocessing#biosensors#Biotech#biotechnology#gene editing#gene therapy#genetic engineering#genetics#genomics#healthcare#personalized medicine#recombinant DNA technology#regenerative medicine.#science#sustainability#synthetic biology#technology
0 notes
Text
R-loops work with TERRA project at the G4 telomere meeting: and ILF-3 is not the correct option to erase SASPects
Telomeres are specialized structures at the ends of linear chromosomes that protect genome stability. At their level, particular forms of chromatin may be found. G-quadruplexes (G4s) are noncanonical nucleic acid structures pivotal to cellular processes and disease pathways. Deciphering G4-interacting proteins is imperative for unraveling G4’s biological significance. Very recently, scientists…

View On WordPress
#ALT pathway#DNA damage#DNA methylation#DNA:RNA hybrid structures#G-quadruplexes#heterochromatin#homologous recombination#senescence#telomerase#telomere stability#telomeric proteins
0 notes
Text
https://app.socie.com.br/read-blog/144372_recombinant-dna-technology-market-analysis-size-share-and-forecast-2031.html
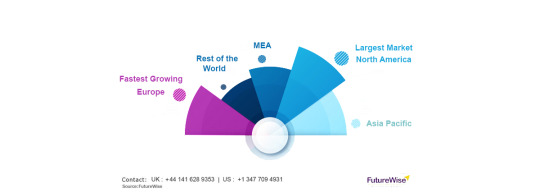
Recombinant DNA Technology Market Analysis, Size, Share, and Forecast 2031
#Recombinant DNA Technology Market#Recombinant DNA Technology Market Scope#Recombinant DNA Technology Market Size
0 notes
Text
Recombinant Protein Expression Market Outlines, Future Trends, Insight And Quality Analysis

Recombinant protein expression is a powerful technique used to produce proteins in large quantities by introducing the gene encoding the protein of interest into a host organism, typically bacteria, yeast, insect cells, or mammalian cells
The Recombinant Protein Expression was valued at $2,393.0 million in 2023 and is expected to reach $6,963.6 million by 2033, growing at a CAGR of 11.27% between 2023 and 2033
Gene Expression Analysis Overview
Selection of Expression System: The choice of expression system depends on factors such as the size and complexity of the protein, desired post-translational modifications, and downstream applications.
Common expression systems include bacterial (e.g., Escherichia coli), yeast (e.g., Saccharomyces cerevisiae), insect cells (e.g., Sf9 cells), and mammalian cells (e.g., Chinese hamster ovary cells).
Cloning of the Gene: The gene encoding the protein of interest is isolated and cloned into an expression vector. The vector contains regulatory elements such as promoters and enhancers that drive gene expression in the host organism.
Transformation or Transfection: The recombinant expression vector is introduced into the host organism by transformation (in bacteria and yeast) or transfection (in insect cells and mammalian cells).
Protein Production: The host cells are cultured under optimized conditions to produce the recombinant protein.
Protein Purification: After protein expression, the recombinant protein is purified from the host cell lysate or culture supernatant.
Market Segmentation
Segmentation 1: By Application
Segmentation 2: By End User
Segmentation 3: By Product
Segmentation 4: By Expression System
Segmentation 5: By Region
Protein expression in North America is a dynamic and crucial field with a significant impact across industries.
The region, especially North America, is a global leader in biopharmaceuticals, relying extensively on protein expression for producing biologics, including monoclonal antibodies and vaccines.
North America holds the largest share of the protein expression market
Download our sample page now click here !
Application for Recombinant Protein Expression Market
Drug Discovery
Structural Biology
Disease Modelling
Enzyme Production
Vaccines Development
Therapeutic Proteins
Immunoassays
Key Market Players
Agilent Technologies, Inc.
Bio-Rad Laboratories, Inc.
Charles River Laboratories International, Inc.
Danaher Corporation (Abcam plc.)
GenCefe Co., Ltd.
Genscript Biotech Corporation
And many others
Market Dynamics
Market Drivers
Increasing Demand for Protein Biologics Creating the Need for Protein Expression
Market Restraints
Long and Complicated Regulatory Timelines and Approvals of Recombinant Proteins and Biologics
Market Opportunities
Rising Awareness of Proteomics in Emerging Countries
Visit our Life Sciences & Biopharma page for better understanding
Key factors contributing to the growth of the recombinant protein expression market
Expanding applications of recombinant proteins in drug discovery, biomanufacturing, and diagnostic assays
Rising prevalence of chronic diseases and the need for innovative therapies.
Recent Developments in the Recombinant Protein Expression Market
In January 2024, Evosep, a leader in sample preparation for mass spectrometry-based proteomics, partnered with Thermo Fisher Scientific Inc., a global scientific leader, to advance clinical proteomics research. This collaboration would combine Evosep's sample separation technology with Thermo Fisher Scientific Inc.'s mass spectrometry instruments, enhancing proteomics research capabilities.release would support pharmaceutical and biotechnology companies engaged in the manufacturing of therapeutic proteins, with the goal of improving product quality and expediting time-to-market.
Key Questions Answered
Q What is the estimated global market size for the protein expression market?
Q What are the future trends expected in the protein expression market?
Q What does the supply chain and value chain of the protein expression market look like?
Q What is the regulatory framework of the protein expression market?
Q How has the COVID-19 outbreak affected the future trajectory of the protein expression market?
Q What are the market entry barriers and opportunities in the protein expression market?
Q What are the major market drivers, challenges, and opportunities of the protein expression market?
Q How is each segment of the protein expression market expected to grow during the forecast period, and what is the anticipated revenue generated by each of the segments by the end of 2033?
Q What is the growth potential of the global protein expression market in North America, Europe, Asia-Pacific, Latin America, and Rest-of-the-World, and what are the driving and challenging factors of the market in each of these regions?
Q Who are the leading players with significant offerings in the protein expression market, and what is the current market dominance for each of these leading players? Who are the next frontiers in the protein expression market?
Conclusion
In conclusion, the recombinant protein expression market continues to thrive and evolve as a vital component of numerous industries, including biotechnology, pharmaceuticals, agriculture, and research
0 notes
Text
As per the report from nova one advisor, the global recombinant DNA technology market size was valued at USD 767.84 billion in 2023 and is projected to reach USD 1,202.46 billion by 2032, growing at a CAGR of 5.11% from 2023 to 2032 according to a new report by Nova One Advisor.
0 notes
Text
As per the report from nova one advisor, the global recombinant DNA technology market size was valued at USD 767.84 billion in 2023 and is projected to reach USD 1,202.46 billion by 2032, growing at a CAGR of 5.11% from 2023 to 2032 according to a new report by Nova One Advisor.
0 notes
Text
i start college on wednesday can somebody please hit me over the head with a brick
#aka sitting in my room being miserable on zoom calls :’)#why didn’t i apply to a 4 year and get housing?#easy. scared#i’m also not taking a single class relevant to my degree please just let me in the lab alreadyyyyyyy#i don’t WANT to take cultural diversity i want to work with recombinant dna#i’m being so annoying about this but like.
1 note
·
View note
Text
wrizard's super basic guide to y-chromosome-based identification!!
for those interested, on this fitzcovery day:
a dear friend asked me to explain why i felt completely insane about the phrase "genetic distance of one" and, as usual, i got overexcited and wrote an entire thing about it complete with goofy images! it's on twt HERE, but i figured it would also be nice to pop it up here also. SO. with the caveat that it has been many years since my last bio class and this is VERY OVERSIMPLIFIED. here's
Human DNA is grouped into chromosomes. We generally have TWO of each chromosome: 22 pairs (numbered 1-22), plus one pair of sex chromosome (typically either two X-chromosomes (XX), or one X-chromosome and one Y-chromosome (XY)). That's 23 pairs, or 46 chromosomes, in total.
When producing sex cells, matching chromosome pairs will RECOMBINE (swap bits of information) - eg. one Chromosome 4 will remix itself with the other Chromosome 4, making TWO UNIQUE C4s. When the cell splits into two sex cells, each sex cell will carry ONE unique C4.
That's sexual reproduction! Every new offspring is genetically unique - new combinations of traits pop up quickly, and if they improve reproductive fitness, can be passed on to future offspring. This allows for rapid adaptation and changes in a species over time.
But what about Y-chromosomes, which don’t have pairs? They can't recombine in the way paired chromosomes can - which means Y-chromosomes pretty much only change via mutation (errors in copying DNA). Mutation is VERY slow, especially compared to recombination.
This means that when an XY parent passes down their Y-chromosome to a child, chances are high that chromosome will have few, if any, changes – as opposed to X-chromosomes, which recombine in both XX parents and children, shuffling genetic information all over the place.
Due to this slow rate of change, Y-chromosomes can be more easily tracked through the generations than other human chromosomes. A Y-chromosome might be passed down nearly unchanged for hundreds of years from genetic father to genetic son.
GENETIC DISTANCE refers to the measurement of difference between two sets of DNA. The lower the genetic distance, the more closely related the two samples are likely to be. A genetic distance of 1 means the samples are close to identical.
Because we know how slowly Y-chromosomes change over time, we know that if the Y-chromosomes of two people have a low genetic distance, this implies that those people are paternally related – even if the two people live/lived hundreds of years apart.
In the case of Captain James Fitzjames, genetic data was extracted from a set of unidentified remains (a molar from a disarticulated mandible). 17 genetic markers from the molar’s Y-chromosome were compared to the Y-chromosome of a confirmed paternal relative of the Captain.
Those 17 markers were the same in both samples, giving the two Y-chromosomes a genetic distance of one – meaning, with the genetic information available, the living relative and the unidentified decedent are more than 2000 TIMES more likely to be paternally related than not.
EDIT: DOIP I MISREAD THE CHART 16 of 17 match, not all 17!!
Along with all the information we have from the historical record, the context of the remains, and this new comparative genetic analysis, we can safely conclude that this particular set of remains belong to Captain Fitzjames.
160 years isn't long in the grand scheme. Every identified set of remains is another reminder that these were people, not just a distant curiosity. It's humbling to remember not just that we have identified Cpt. Fitzjames, but that still, today, we have a genetic distance of one.
Photos and Y-chromosome comparison chart taken from Stephen, Fratpietro, and Park's paper "Identification of a senior officer from Sir John Franklin’s Northwest Passage expedition" from the Journal of Archaeological Science: https://www.sciencedirect.com/science/article/pii/S2352409X24003766?via%3Dihub
hope my nonsense is helpful and/or informative and/or at least made you smile!! if you like this sort of thing :) cheers doves
#james fitzjames#the terror#wriz writes#wriz draws#I GUESS LMAO.#cw bones#finally a use for my 4/5 of an anthro minor 🙏
345 notes
·
View notes
Text
Filters in the way of technologically advanced life in the universe and how likely I think they are
1. Abiogenesis (4.4-3-8 billion years ago): Total mystery. The fact that it happened so quickly on Earth (possibly as soon as there was abundant liquid water) is a tiny bit of evidence for it being easy. Amino acids and polycyclic hydrocarbons are very common in space, but nucleotides aren't, and all hypothetic models I've seen require very specific conditions and a precise sequence of steps. (It would be funny if the dozen different mechanisms proposed for abiogenesis were all happening independently somewhere.)
2. Oxygenic photosynthesis (3.5 billion years ago) (to fuel abundant biomass, and provide oxygen or some other oxidizer for fast metabolism): Not so sure. Photosynthesis is just good business sense -- sunlight is right there -- and appeared several times among bacteria. But the specific type of ultra-energetic photosynthesis that cracks water and releases oxygen appeared only once, in Cyanobacteria. That required merging two different photosynthetic apparati in a rather complex way; and all later adoptions of oxygenic photosynthesis involved incorporating Cyanobacteria by endosymbiosis. For all that it's so useful, I don't know if I'd expect to see it on every living planet.
3. Eukaryotic cell (2.4 billion years ago?): Probably the narrowest bottleneck on the list. Segregated mitochondria with their own genes and a nucleus protecting the main genome are extremely useful both for energy production (decentralized control to maximize production without overloading) and for genetic storage (less DNA damage due to reactive metabolic waste). But there's a chicken-and-egg problem in which incorporating mitochondria to make energy requires an adjustable cytoskeleton, but that consumes so much energy it would require mitochondria already in place. Current models have found solutions that involve a very specific series of events. Or maybe not? Metabolic symbiosis, per se, is common, and there may have been other ways to gene-energy segregation. Besides, after the origin of eukaryotes, endosymbiosis occurred at least nine more times, and even some bacteria can incorporate smaller cells.
4. Sexual reproduction (by 1.2 billion years ago): Without meiotic sex (combining mutations from different lineages, decoupling useful traits from harmful ones, translating a gene in multiple way), the evolution of complex beings is going to be painfully slow. Bacteria already swap genes to an extent, and sexual recombination is bundled in with the origin of eukaryotes so I probably shouldn't count it separately (meiosis is just as energy-intensive as any other use of the cytoskeleton). Once you have recombination, life cycles with spores or gametes and sex differentiation probably follow almost inevitably.
5. Multicellularity (800 million years ago?): Quite common, actually. Happens all the time among eukaryotes, and once in a very limited form even among bacteria. Now we'd want complex organized bodies with geometry-defining genes, but even that happened thrice: in plants, fungi, and animals. As far as I know, various groups of yeasts are the only regressions to unicellularity.
6. Brains and sense organs (600 million years ago): Nerve cells arose either once or twice, depending on whether Ctenophora (comb-jellies) and Eumetazoa (all other animals except sponges) form a single clade or not. Some form of cellular sensing and communication is universal in life, though, so a tissue specialized for signal transmission is probably near inevitable once you have multicellular organisms whose lifestyle depends on moving and interacting with the environment. Sense organs that work at a distance are also needed, but image-forming eyes evolved in six phyla, so no danger there (and there's so many other potential forms of communication!). Just to be safe, you'll also want muscles and maybe mineralized skeletons on the list, but I don't think either is particularly problematic. An articulated skeleton is probably better than a rigid shell, but we still have multiple examples of that (polyplacophorans, brittle stars, arthropods, vertebrates).
7. Life on land (400 million years ago): (Adding this because air has a lot more oxygen to fuel brains than water (the most intelligent aquatic beings are air-breathers), and technology in water has the issue of fire.) You're going to need a waterproof integument, some kind of rigid support system, and kidneys to regulate water balance. Plenty of animal lineages moved on land: vertebrates, insects, millipedes, spiders, scorpions, multiple types of crabs, snails, earthworms, etc. Note that most of those are arthropods: this step seems to favor exoskeletons, which help a great deal in retaining water. Of course this depends on plants getting on land first, which on Earth happened only once, and required the invention of spores and cuticles. (Actually there are polar environments where all photosynthesis occurs in water, but they are recently settled and hardly the most productive.)
8. Human-like intelligence (a few million years ago?): There seems to a be a general trend in which the max intelligence attainable by animals on Earth has increased over time. There's quite a lot of animals today that approach or rival apes in intelligence: elephants, toothed cetaceans, various carnivorans, corvids, parrots, octopodes, and there's even intriguing data about jumping spiders. Birds seem to have developed neocortex-like brain structures independently. Of course humans got much farther, but the fact that even other human species are gone suggests that a planet is not big enough for more than one sophont, so the uniqueness of humans might not necessarily imply low probability. (We seem to exist about halfway through the habitability span of Earth land, FWIW.) The evolution of sociality should probably be lumped here: we'll want a species that can teach skills to its offspring and cooperate on tasks. But sociality is also a common and useful adaptation: many species on our list (octopodes are a glaring exception) are intensely social and care for their offspring. I mentioned above that the land-step favors exoskeletal beings, which in turns favors small size; but the size ranges of large land arthropods and very intelligent birds overlap, so that's not disqualifying.
9. Agriculture and urban civilization (11,000 years ago): Agriculture arrived quite late in the history of our species, but when it arrived -- i.e. at the end of the Wurm glaciation -- it arrived independently in four to eight different places around the world, in different biogeographic realms and climates, so I must assume that at least some climate regimes are great for it (glacial cycles are a minority of Earth's history; but did agriculture need to come after glaciations? Maybe a shock of seasonality did the trick). And once you have agriculture, complex urbanized societies follow most of the time, just a few millennia later. Even writing arose at least three times (Near East, China, and Mexico), and then spread quickly.
10. Scientific method and industrialization (300 years ago): We're getting too far from my expertise here, but whatever. The Eurasian Axial Age suggests that all civilizations with a certain degree of wealth, literacy, and interconnection will spawn a variety of philosophies. Philosophical schools that focus on material causes and effects like the Ionians or Charvaka have appeared sometimes, but often didn't win over more supernaturalist schools. Perhaps in pre-industrial times pure materialism isn't as useful! You may need to thread a needle between interconnected enough to exchange and combine ideas, and also decentralized enough that the intellectual elite can't quash heterodoxy. As for industrialization, that too happened only once, though that's another case in which the first achiever would snuff out any other. I hear Song China is a popular contender for alternative Industrial Revolutions (with coal-powered steelworks!); Imperial Rome and the Abbasid Caliphate are less convincing ones. For whatever reason, it didn't take until 18th century Britain.
11. Not dying randomly along the way: Mass extinctions killing off a majority of species happened over and over -- the Permian Great Dying, the Chicxulub impact, the early Oxygen Crisis -- but life has always rebounded fairly quickly and effectively. It's hard enough to sterilize an agar plate, let alone a planet. Disasters on this scale are also unlikely to happen in the lifespan of planet-bound civilizations, unless of course the civilizations are causing them. A civilization might still face catastrophic climate change, mega-pandemics, and nuclear war, not to mention lesser setbacks like culture-wide stagnation or collapse, and I couldn't begin to estimate how common, or ruinous, they would actually be.
****
I have no idea how common the origin of life is, but the vast majority of planets with life will only have bacterial mats and stromatolites. Of the tiny sliver that evolved complex cells, a good chunk will have their equivalents of plants and animals, most of which may have intelligent life at least on primate- or cetacean-level at some later point. At any given time, a tiny fraction of those will have agricultural civilizations, at an even tinier fraction of that will have post-industrial science and technology. Let's say maybe 1 planet with industrial technology out of 100 with agriculture, 100,000 with hominid-level intelligence, 10 million with animal-like organisms, 100 millions with complex cells, and 10 billions with life at all?
171 notes
·
View notes
Text
ENDOO SHUT THE FUCK UPP
SO U MEAN TO TELL ME
THAT OUR FAN THEORIES
ARE CORRECT.

(endo-san, if you've planned this from the beginning, then a thousand applauds for you for sticking to this plot & foreshadowings for so long. If u were just throwing shit up & rely on fans' theories to see where you're going next, then great job for making it seem so seamless. wtf.)

I still don't buy the "Donovan is an alien" narrative completely; I still think he changed after he secretly volunteered to be a lobotomy experiment subject, to get the superpower he wants to reach a greater understanding in humanity.
Although, if Endo really wish to introduce a terrestial being into this series, then, does that mean Anya's telepathic ability is really born from the lab's experiment? Is she born from the sperm of an alien?? Alien DNA recombination?? Lolol. *edit: this is just me hoping that Anya's telepathic ability was developed in vitro, bc the idea of those mad scientists opening up Anya's skull & cutting up her brain whe she was a baby is just sick beyond belief & i cant accept that.*
I am so so glad to see Fiona in this chapter, trying to extract extra info from Desmond's staff! It really hammers down how incredible this valuable opportunity is for Westalis. Great job Endo-san, for not forgetting to include this 👍
Wait.

Wait a damn minute.
If what Melinda say is true, Donovan can read minds,
Then Donovan must've read Twillight's mind on their first meeting; his fake persona & and Operation Strix have already been busted.

Maybe this is not just a mere illustration inside Twillight's head, maybe this really IS Donovan's reaction when he find out about Operation Strix?? LMAO
Or maybe Donovan can only read minds to a certain extent –not fully like Anya, so maybe he can only get the gist of what people are thinking; so he doesn't know Operation Strix yet, but he knows that Twilight wants to extract some information from him.
(though I wanna add, even if Twillight is from the opposing country, I hope Twillight's sincerity to meet in the middle could reach Donovan too.)
I've known these theories for awhile, but could these be true afterall?? (We're already at this stage, but I still don't wanna assume fanons as canons.)
Then.
IF Damian —at the family dinner— was remembering Anya's confession that she can read minds, and he wished he could read what's on his father's mind too,
Does it mean Anya's telepathic ability is already busted by Donovan?

Is my theory from ch 106 correct afterall???
Oh wAIT.
FUCK I forgot if Donovan read Melinda's mind too, then he must've learned that Melinda has befriended Yor Forger. The mother of Anya Forger. The daughter of Loid Forger.
Combine that information from Melinda and information from Damian that Anya can read minds....
... does it mean Donovan has assumed that Twillight from Westalis is using his telepathic "daughter" as a spy in Eden Academy?

Funny thing is, Twilight himself has no idea about all this implication. He's a million steps away from Donovan.
HAHAHAHHAA
(lol how could I not read this from miles away)
Argh fuck, I'm really worried for Anya now.
This could very well escalate into the "war" of obtaining Anya as an asset. Maybe it'll still be light-hearted, like manipulating Damian's romantic feelings for Anya by inviting her to have dinner together, but still. At first, I thought Donovan might get Anya expelled from school because she's dangerous, but I figure she's just too valuable to be tossed away; it's better to keep her close while extracting info from her as much as he can. Or use her ability to the fullest.
At least Anya is now aware that Donovan has the same ability as her, so at least she can proceed with more caution now.
I hate seeing Anya being the only one aware of the whole situation & she struggles alone to keep it all together — like what she has done all this time. That's why I really want Anya to come clean with Twilight so he can at least get a picture of the whole situation he's facing right now, and protect her properly, but I know it'll massively shift the Forger dynamic we're used to. I have no idea how will Endo handle this development from now on.
Well, that's enough anxiety for tonight.
Stay safe Anya! 🙏🥺🥺🥺
#spy x family#spy x family manga#sxf#spy x family spoilers#sxf spoilers#sxf manga#spy x family chapter 110#sxf ch 110#sxf manga spoilers#sxf theory
139 notes
·
View notes