#Azimuth 86
Explore tagged Tumblr posts
Video
A Waterfall in Maligne Canyon (Jasper National Park) by Mark Stevens Via Flickr: A setting looking to the east while taking in views of a waterfall in Maligne Canyon around the First Bridge area. This is in Jasper National Park. I chose to work with Aurora HDR Pro to better bring that complete setting in the image. I later exported a TIFF image to DxO PhotoLab 6 where I did some final adjustments with contrast, saturation and brightness for the final image.
#Alberta and Glacier National Park#Aurora HDR#Aurora HDR Pro#Azimuth 86#Canadian Rockies#Central Front Ranges#Day 3#DxO PhotoLab 6 Edited#Evergreen Trees#Evergreens#First Bridge#Forest#Forest Landscape#HDR#Hillside of Trees#Jasper National Park#Landscape#Landscape - Scenery#Looking East#Maligne Canyon#Maligne Canyon Loop#Maligne Canyon Trail#Maligne Range#Nature#Nikon D850#No People#Outside#Project365#Rocky Mountains#Rolling Hillsides
3 notes
·
View notes
Note
PS Neither the egg fic nor the vegan freak have anything to do with M/gastar before you try it. That's all pure Starscream stanning, baby. And one of them is St/rop, the supposedly ""good""" ship LOL.
List of female Transformers Main Complete list Following is a thorough list of the various female Transformers in canon thus far. Many of these characters were Japan-exclusive, featured only in fiction, or exist as limited-run exclusive toys. Female characters who had multiple toys are listed only once. Generation 1 (Numbers indicate order of appearance.) Chromia (1) Moonracer (2) Firestar (3) Elita One (4) Greenlight (5) Lancer (6) Arcee (7) Beta (8) An Autobot rebel (9) Paradron Medic (11) Nancy (12) Minerva (13) Clipper (14) Karmen (18) Glyph (20) Road Rage (21) Discharge[1] (22) Windy[1] (23) Vibes (24) Roulette (25) Flareup (32) Flip Sides (34) Rosanna (35) Windrazor (38) Thunderblast (46) Cassiopeia (47) Nautica (51) Windblade (52) Victorion (61) Velocity (63) Javelin (62) Proxima (64) Roadmaster (65) Acceleron (66) Override (69) Rust Dust (70) Pyra Magna (71) Skyburst (72) Stormclash (73) Jumpstream (74) Dust Up (75) Scorpia[1] (76) Eos (80) Lifeline (83) Quickslinger (84) Hotwire[2] (98) Strongarm (99) Slide[2] (104) Crush Bull[2] (107) Oiler[2] (108) Broadside[2] (109) Sky High[2] (110) Circuit[2] (116) Pyra Ignatia Spark[2] (118) Scorchfire (122) Orthia (126) Smashdown[2] (128) Esmeral (15) Lyzack (16) Clio (17) Nightracer (19) Shadow Striker (26) Howlback (31) Flamewar (33) Flip Sides (34) Crasher (39) Freezon[1] (44) Nightracer (49) Slipstream (50) Twirl (54) Nickel (60) Swift (77) Killjoy (79) Blackout[2] (81) Spaceshot[2] (82) Crash Test (85) Trickdiamond (92) Moonheart (93) Megaempress (94) Flowspade (95) Lunaclub (96) Megatronia (100) Buckethead[1] (103) Diveplane[1] (112) Seawave[1] (113) Mindgame (114) Tracer[2] (115) Devastator[2] (117) Cindersaur[2] (125) Shadow Striker (127) Nova Storm[2] (129) Termagax (133) Kaskade (135) Heavywait (138) Tyrannocon Rex (139) Cheesecake robot (10) Roulette and Shadow Striker's sister (27) Path Finder (28) Small Foot (29) Devcon's galpal (30) One of Optimus Prime's rescuees (36) Angela (37) Four members of the Kaon upperclass (40-43) Ma-Grrr (45) Red waitress Transformer (48) Windshear (53) Solus Prime (55) Female protester (56) Lightbright (57) Strafe (58) Mistress of Flame (59) Exocet (67) Vertex (68) Aileron (78) Gnash (86) Slice (87) Thrashclaw (88) Shred (89) A pair of Devisen twins (90-91) Maxima (97) Sieg[3] (101) Kari (102) Anode (105) Lug (106) X-Throttle (111) Rum-Maj (119) Praesidia Magna (120) Fastbreak (121) Crash Test (122) Stardrive (123) Magrada (124) Leviathan (130) Codexa (131) Gauge (132) Lodestar (134) Shutter (136) Sharpclaw (137) Cargohold (140) Half-qualifiers: Alana, turned into a Transformer for a short time. Aunty, female Cybertronian intelligent computer. Combination granny and attack-dog-bots, human-sized drones supposedly based on Transformer technology. One of Maccadam's bartenders Nightbird Overlord, has a female side to him. Some of the "Teletraan" computers like 15 and 10 are female. There appears to be a female design among a group of old generics. Bayonet, the fake female Decepticon disguise of Britt. In the French dub of The Transformers: The Movie, Shrapnel and Starscream are considered female. Shrapnel is also female in the Russian dub. Beast Era (Numbers indicate order of appearance.) Airazor (2) Kitte Shūshū (5) Rage (6) Botanica (7) Sonar[1] (13) Crystal Widow (14) Crossblades (15) Stiletto (16) Transmutate[1] (18) Binary (19) Wedge Shape[1] (24) Aura (25) Legend Convoy[1] (26) Stockade[2] (28) Rav (29) Hammerstrike[2] (31) Triceradon[2] (35) Skimmer (36) Nyx (44) Blackarachnia (1) Scylla (3) Antagony (4) Strika (8) Manta Ray[1] (17) Ser-Ket (20) Dead-End[2] (27) Jai-Alai (30) Max-B[2] (32) Gaidora (33) Soundbyte/Soundbite (34) Liftoff (37) Freefall (38) Snarl-blast[2] (39) Vertebreak (43) Skold (45) Libras (9) Virgol (10) Cancix[1] (11) Possibly Sagittarii (12) Dipole (21) Vamp (22) Plasma[2] (23) Deep Blue (40) At least two bridge officers of the Terrastar (41-42) Half-qualifiers: NAVI-ko, female Cybertronian intelligent computer NAVI (Yukikaze), female Cybertronian intelligent computer NAVI (Gung Ho), female Cybertronian
intelligent computer DNAVI, female Cybertronian intelligent computer Medusa, an Intruder-built robot modified with Cybertronian technology Robots in Disguise (2001) (Numbers indicate order of appearance.) Optimus Prime[2] (1) Nightcruz[1] (3) Scourge[2] (2) Half-qualifiers: T-AI, female Cybertronian intelligent computer. Unicron Trilogy (Numbers indicate order of appearance.) Airazor (5) Arcee (9) Autobot nurses (10) Two Velocitronian band members (11-12) Override[4] (13) Joyride[4] (15) Quickslinger (16) Crystal Widow (24) Treadbolt (33) Chromia (34) Thunderblast (14) Spacewarp (30) Sureshock (1) Combusta (2) Falcia (3) Twirl (4) Sunburn (6) Cliffjumper[1] (7) Ironhide[1] (8) Spiral[1] (9) Offshoot[1] (17) Breakage[1] (18) Kickflip[1] (19) Mudbath[1] (20) Heavy Metal[1] (21) "Disco ball" (22) Road Rebel[1] (23) Guardian Speed[1] (25) Mugen[1] (26) Bingo/Triac[1] (27) Wedge Shape[1] (28) Sprite (29) Boom Tube (31) Windrazor (32) Rán (33) Half-qualifiers: A possible scooterformer Dark Nitro Convoy, evil clone of a character whose gender was switched in translation Red Alert, minimally-altered release of a toy that was female in Japan Midnight Express, unaltered release of a toy that was female in Japan Hourglass, a female character who might be a Cybertronian Bombshell, a female character who might be a Cybertronian Carillon, a female character who might be a Cybertronian Vector Prime, the former multiversal entity who was female in some universes Movie continuity family (Numbers indicate order of appearance.) Arcee (1) Elita-One (2) Chromia (4) Perihelion (8) HMS Alliance (9) Windblade (13) Fracture (3) Alice (5) Shadow Striker (6) Override[3] (7) Diabla (10) Howlback (11) Shatter (12) Nightbird Airazor Half-qualifiersJetfire claims to have a mother who may or may not have been a Transformer. Animated (Numbers indicate order of appearance.) Sari Sumdac (2) Arcee (3) Elita-1 (4) Red Alert (6) Botanica (8) Flareup (10) Rosanna (11) Glyph (12) Lickety-Split (13) Lightbright (14) Chromia (16) Clipper (17) Quickslinger (18) Kappa Supreme (19) Override Prime (20) Windy (21) Road Rage (25) Flashpoint (26) Minerva (27) Sureshock (28) Nightbeat (29) Sunstreaker (30) Blackarachnia (1) Slipstream (5) Strika (7) Flip Sides (9) Antagony (15) Wingthing (22) Beta (23) Drag Strip (24) Half-qualifiers: Teletran-1, female Cybertronian intelligent computer TransTech (Numbers indicate order of appearance.) Blackarachnia (5) Strika (3) Unnamed medic (1) Andromeda (2) Cyclis (4) Sonar (6) Hammerstrike (7) Scorpia (8) Proxima (9) Half-qualifiers: Axiom Nexus News Editor, a 'bot with one male and one female personality Shattered Glass (Numbers indicate order of appearance.) Crasher (1) Esmeral (6) Howlback (7) Arcee (2) Andromeda (3) Elita-One (4) Strongarm (8) Windblade (9) Nautica (10) Beta (5) Half-qualifiers: Teletraan-X, female Cybertronian intelligent computer. Aligned continuity family (Numbers indicate order of appearance.) Akiba Prime Arc Arcee Arcee Blade Assault Star Brushfire Cameo Catapult Chevalier Chromia Deep Blue Ether Walker Firestar Galaxy Flare Galaxy 'Questrian Glow Matronly Docent Quickshadow Rocket Plume Solus Prime Strongarm Tempest Spin Thunderclap Upkeep Windblade Airachnid Astraea Aurora Speeder Balewing Coldstar Crimson Phantom Cyberwarp Cyclone Dancer Diabla Duststorm Fallen Angel Filch Flamewar Flash Runner Glowstrike Hoverbolt Helter-Skelter Hurricane Hunter Ida Lensflare Metal Thunder Nebula Ripper Night Dancer Overhead Retrofit Rollcage Scatterspike Skyjack Slink Slipstream Spiral Zealot Supernova Flame Variable Star Void Pulse Zizza Ser-Ket Ripclaw Azimuth Cogwheel Elita One Mercury Moonracer Nightra Override Bot Shots (Numbers indicate order of appearance.) Buzzclaw (1) Kre-O (Numbers indicate order of appearance.) Chromia (1) Arcee (3) Strika (4) Minerva (5) Windblade (6) Paradron Medic (10) Strongarm (12) Skimmer[1] (13) Airachnid (2) Thunderblast (7) Blackarachnia (8) Slipstrike (9) Ida (11) Liftoff[1] (14) Freefall[1] (15) Angry Birds Transformers (Numbers indicate order of appearance.) Stella as:Arcee
(1) Airachnid (2) Chromia (4) Novastar (10) Moonracer (11) Greenlight (12) Silver as:Windblade (3) Energon Windblade (5) Elita-One (8) Matilda as:Energon Nautica (6) Nautica (7) Strongarm (9) Zeta as:Nightbird (13) Rosanna (15) Zeta as:Slipstream (14) Cyberverse (Numbers indicate order of appearance.) Arcee Chromia Clobber Jazz[3] Windblade Alpha Strike Nova Storm Shadow Striker Skywarp Slipstream Blackarachnia Cosmos Operatus Solus Prime Half-qualifiers: In the Japanese dub of Cyberverse, Thrust was female, and went by the name Red Wing. Acid Storm fluctuates between the male and female Seeker body types in show. Mae Catt would explain this on Twitter as this being "just something Acid Storm likes to do" and that pronouns are "up to Acid Storm". This would imply Acid Storm is non-binary gender fluid, thus they semi-qualify for the list. BotBots (Numbers indicate order of appearance.) Aday Angry Cheese Arctic Guzzlerush Bankshot Big Cantuna Bok Bok Bok-O Bonz-Eye Bot-T-Builder Bottocorrect Bratworst Brock Head Chef Nada Clawsome Crabby Grabby Cuddletooth Dingledeedoo Disaster Master Disgusto Desserto DJ Fudgey Fresh Doctor Flicker Drama Sauce Drillit Yaself Face Ace Fail Polish Fit Ness Monster Flare Devil Flood Jug Fomo Frohawk Frostfetti Frostyface Glam Glare Fancy Flare Glitch Face Goggly Spy P.I. Gold Dexter Goldface Goldiebites Goldie Terrortwirl Goldito Favrito Goldpin Baller Gold Punch Grampiano Grandma Crinkles Grave Rave The Great Mumbo Bumblo Greeny Rex Grrr'illa Grimes Halloween Knight Handy Dandy Hashtagz Hawt Diggity Hawt Mess Highroller Hiptoast Ice Sight Javasaurus Rex Jet Setter Knotzel Latte Spice Whirl Leafmeat Alone Loadoutsky Lolly Licks Lolly Mints Miss Mixed Movie Munchster Ms. Take Must Turd Nanny McBag Nomaste Nope Soap Ol' Tic Toc Ollie Bite Outta Order Overpack Pop N. Lock Pop O' Gold Pressure Punk Professor Scope Rebugnant Roarista Sandy Shades Scribby Sheriff Sugarfeet Shifty Gifty Sippyberry Sippy Slurps Skippy Dippy Disc Slappyhappy Smooth Shaker Smore N' More Sour Wing Starscope Sticky McGee Sugar Saddle Super Bubs Sweet Cheat Technotic Sonic Terror Tale Torch Tidy Trunksky Tricitrustops Tropic Guzzlerush Tutu Puffz Twerple Burple Unilla Icequeencone Venus Frogtrap Vigitente Waddlepop Wasabi Breath Whirlderful Whoopsie Cushion Wristocrat
35 notes
·
View notes
Text
0 notes
Photo
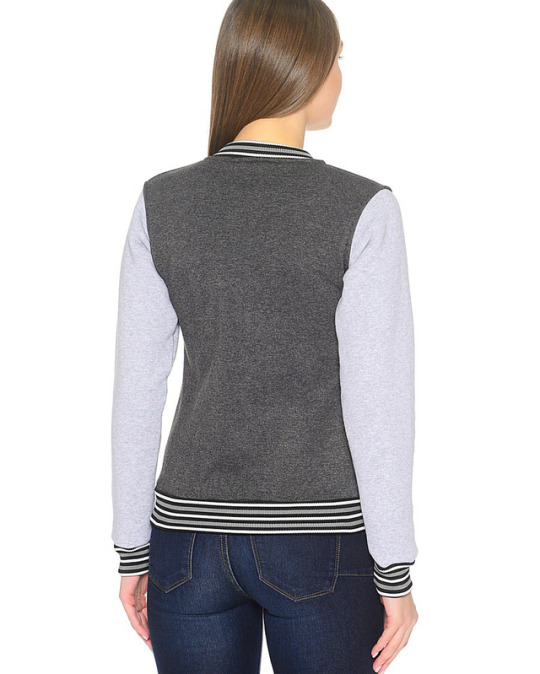
• Жен. бомбер Stella - G86_003 • Подробная информация - azimuthsport.ru/view_goods/zhenskij-bomber-stella-g-86-001-pid-208875 ❗️Оплата после примерки❗️ ______________________________________________________ Как сделать заказ - azimuthsport.ru/page_roznica Отзывы - azimuthsport.ru/index.php?route=product/testimonial ______________________________________________________ Мы в соц.сетях😀 1️⃣ vk.com/azimuth_sport 2️⃣ instagram.com/azimuthsport.ru 3️⃣ ok.ru/group/53542813696118 4️⃣ facebook.com/goryk2015 5️⃣ youtu.be/YOVJZTRnCME 6️⃣ t.me/azimuth_sport ______________________________________________________ . . . . . . . . . . . . #azimuthsport #azimuth #azimuth_official #bogner #roxy #quiksilver #whs #burton #stella #gsousnow #азимут #богнер #рокси #вхс #бертон #стелла #москва #садовод #оптом #чм2018 #футбол #футболка #хлопок #качество #сделановроссии #бомба #бомбер #теплая #хочу #лайки (at ТК Садовод)
#roxy#azimuthsport#azimuth#сделановроссии#садовод#бомба#stella#оптом#богнер#футбол#whs#качество#москва#теплая#burton#бомбер#бертон#quiksilver#стелла#азимут#вхс#хочу#рокси#чм2018#футболка#лайки#bogner#azimuth_official#хлопок#gsousnow
1 note
·
View note
Video
youtube
A type depilator
Features and advantages: 1.The frame is made of stainless steel 2.Adopt A-shaped bracket, the dehairing box can be opened and closed and esay to maintain 3.The angle and azimuth of the hair removal box can be adjusted freely so that flexibale operation speed will reduce accidental damage to polutry skin to the minmum 4.The box body automatically aligned and reached. 5.The capacity is optional and large for people in need. Wechat/whatsapp: 86 132 1320 3466 Email: [email protected] http://www.hnjoyshine.com
#A Type depilator#chicken hair removal#chicken plucker#best plucker for poultry#duck depilator#chicken plucker for sale
0 notes
Text
Promise of a Solar Storm & Satellite Environment Check-In | Space Weather News 08.22.2019
youtube
//pagead2.googlesyndication.com/pagead/js/adsbygoogle.js
(adsbygoogle = window.adsbygoogle || []).push({});
Antenna
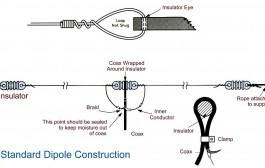
Why dipole antennas are a half wave long ?
January 13, 2015 No comments
Why dipole antennas are a half wave long ? Read more
Green Heron Rotator Controller RT-21azel
October 02, 2014 No comments
Rotator Controller RT-21 Azimuth/Elevation Two separate control boards for independent Azimuth and Elevation New crisp OLED displays Separate Point-an… Read more
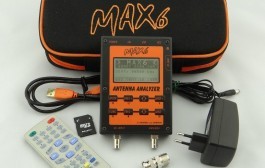
MAX6 -Antenna Analyzers
December 03, 2015 No comments
Antenna Analyzers MAX6 antenna analyzer is not just an ordinary meter for antenna – it’s much more! Built-in radio signal meter allows you to… Read more
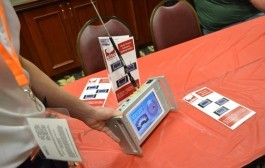
The Siru Innovatios SDR20 adds new features
August 04, 2015 No comments
New features * Smooth zoom in FFT/waterfall view * Adaptive menu * Frequency memory with snapshot pictures of signals * Sliding effect between views *… Read more
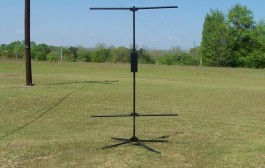
TransWorld Basic Antenna
February 12, 2016 No comments
These two folding arm units are ready for connecting to the array box of your choice, and attaching to either the sturdy Quadrastand or Permanent Moun… Read more
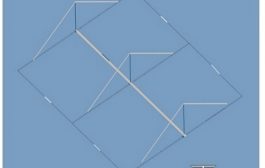
MONO 3 40 MOXON
January 14, 2017 No comments
MONO 3 40 moxon is every contester’s dream, a rugged monoband yagi, compact design and large bandwidth. Specifications Frequency MHz: 7~7,3 Active ele… Read more
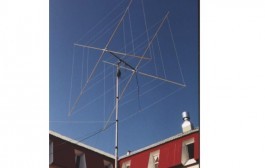
Two element QUAD antenna Model RQ-26H
October 31, 2015 No comments
Antenna RQ-26H- preset “Cubical Quad” on the bands 6, 10, 12, 15, 17, 20 m with heavy duty design. It uses the most advanced composite mat… Read more
News
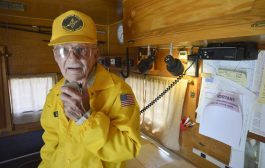
Efforts Continue to Enhance ARES Program, Add Resources
August 23, 2019 No comments
The ARRL Board of Directors, committees, and Headquarters administrative staff are continuing efforts to enhance the venerable Amateur Radio Emergency… Read more
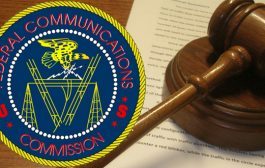
FCC issues $39,000 + Forfeiture Order against man from North Carolina
August 22, 2019 No comments
The WIA report the FCC has issued a $39,000 + Forfeiture Order against a man from North Carolina, for intentional misuse of a local public safety radi… Read more
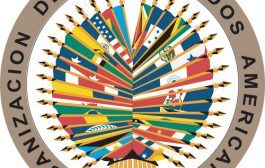
Inter-American Proposal Removes 47 – 47.2 GHz from Bands under Study for 5G Services
August 21, 2019 No comments
The 34th meeting of the Inter-American Telecommunication Commission (CITEL) Permanent Consultative Committee II (PCC.II) concluded a week of meetings… Read more
ARRL Contest and DXCC Rules Now Prohibit Automated Contacts
August 20, 2019 No comments
Following the direction of the ARRL Board of Directors, ARRL has incorporated changes to the rules for all ARRL-sponsored contests and DXCC, prohibiti… Read more
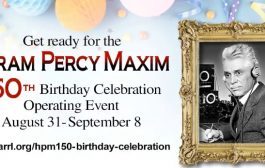
Happy 150! The Hiram Percy Maxim Birthday Celebration!
August 20, 2019 No comments
As announced in September 2019 QST – page 86 This year marks the 150th anniversary of the birth of the League’s first president and… Read more
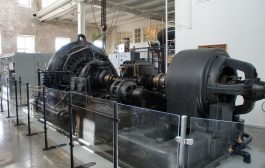
Alexanderson Alternator Station SAQ Hails “Incredible” Number of Listener Reports
August 20, 2019 No comments
Sweden’s Alexanderson Alternator station SAQ says it received 438 listener reports — “an incredible amount” — for its June 30 Alexanderson Day transmi… Read more
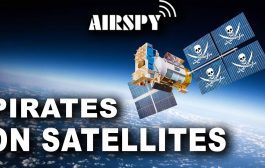
Pirates On US Navy Satellites – UHF SatCom
August 18, 2019 No comments
Here we take a look at how to listen to the Brazillian Pirates on UHF SatCom Read more
//pagead2.googlesyndication.com/pagead/js/adsbygoogle.js
(adsbygoogle = window.adsbygoogle || []).push({});
Review
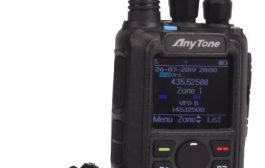
Unboxing the AnyTone 878PLUS by David Casler
August 22, 2019 No comments
Read more
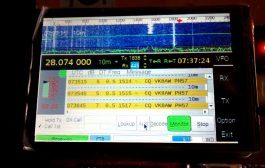
Yaesu 891 + WSJT + FT8 Digital on Raspberry Pi 4
August 17, 2019 No comments
Read more
Wouxun KG-UV980P Quad Band First Look
August 16, 2019 No comments
Read more
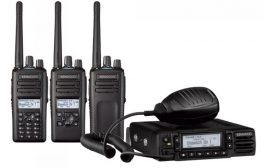
NX-3000 Series lightbar and custom voice prompts | Kenwood Comms
August 15, 2019 No comments
The lightbar and custom voice prompts are shown in operation on the NX-3000 series heandheld walkie talkie radio, these features are a big advantage f… Read more
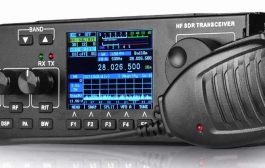
The RS-918 clone of a clone of an outstanding open source SDR transceiver, the mcHF
August 14, 2019 No comments
RS-918 10WATT HF SDR Transceiver RX:1.8-30MHz TX:All HAM HF BANDS,Full Modes: SSB(J3E),CW,AM(RX Only),,FM, FREE-DV Features: Spectrum Dynamic Waterf… Read more
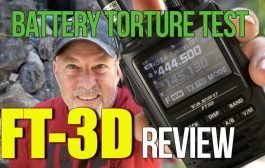
Yaesu FT3D Full Review and Battery Torture Test
August 13, 2019 No comments
“This is it. The first complete unbiased review of the new Yaesu FT3D dual band Fusion handheld ham radio with built in GPS and APRS. Watch this… Read more
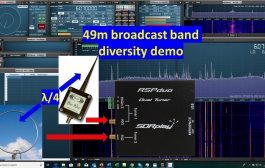
SDRplay HF Diversity Demo
August 12, 2019 No comments
Read more
App – Mobile
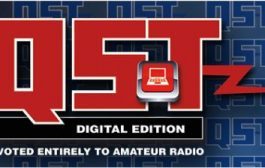
New APP for digital QST
March 25, 2019 No comments
Apple has released a new app for digital QST (version 5.1) readers that use that platform. A long-standing problem involved the inability of some Appl… Read more
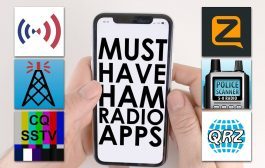
6 Must Have Amateur Radio Apps!
December 24, 2018 No comments
Read more
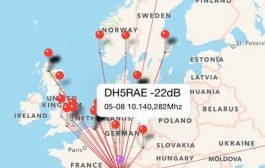
iWSPR TX – WSPR for iOS version 2.8 now available
October 16, 2018 No comments
WSPR WSPR implements a protocol designed for probing potential propagation paths with low-power transmissions. Normal transmissions carry a station’s… Read more
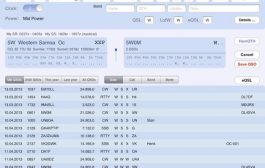
RUMlogNG2Go for iOS version 3.5.2 now available
August 19, 2018 No comments
Thomas Lindner, DL2RUM, is happy to announce the availability of version 3.5.2 of his RUMlogNG2Go logger for the iPhone and iPad. RUMlogNG2Go can be … Read more
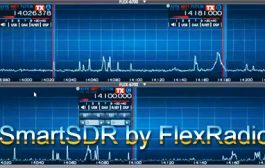
SmartSDR for iOS v2.6.2 Now Available
August 12, 2018 No comments
FlexRadio Systems announces the release of SmartSDR for iOS v2.6.2 is now available for download or update from the Apple App Store. This App requires… Read more
QRZ.com App for Android now in Beta Testing
May 07, 2018 No comments
The updates to QRZ’s Android App, formerly known as QRZDroid have been released and are available to those who are interested in trying it out…. Read more
EchoLink for iOS version 2.10.18 is now available
May 07, 2018 No comments
EchoLink for iOS version 2.10.18 is now available in the App Store. This is a 64-bit build that is fully compatible with iOS 10 and modern iOS devices… Read more
The post Promise of a Solar Storm & Satellite Environment Check-In | Space Weather News 08.22.2019 appeared first on QRZ NOW – Ham Radio News.
from RSSMix.com Mix ID 8899291 https://ift.tt/2L0VwXC via IFTTT
from WordPress https://ift.tt/2KSKWTm via IFTTT
0 notes
Text
Eastmain Strengthens the Percival Discovery at Clearwater with 1.84 g/t Au over 22.2 m
TORONTO — Eastmain Resources Inc. (“Eastmain” or the “Company” – TSX:ER, OTCQX:EANRF) is pleased to report results for the first two drill holes (674 metres (“m”)) of the 2019 winter program at the Percival discovery (“Percival”), on the 100%-owned Clearwater Property (the “Property”) in James Bay, Québec (see FIGURES 1-5).
Drilling Highlights:
ER19-832: 1.86 grams per tonne gold (“g/t Au”) over 52.7 m (vertical depth of 25 m), including 4.89 g/t Au over 11.0 m zone (silicified breccia).
Hole ER19-832 was drilled east to west through the discovery hole section (holes ER18-822 and ER18-823) confirming mineralization extends along predicted plunge and testing an interpreted NNW fault; silicified breccias of the main Percival zone were cut from surface to a 55 m downhole depth, and a second mineralized interval was cut between 115 m to 140 m.
ER19-833: 1.84 g/t Au over 22.2 m (vertical depth of 86 m), including 13.4 g/t Au over 1.0 m (silicified breccia).
Hole ER19-833 targeted a wide EM anomaly associated with the projected eastern extension of Percival, intersecting silicified breccia mineralization from 100 m to 125 m downhole; a second interval has been intersected between 145 m and 180 m.
Claude Lemasson, Eastmain President and CEO commented: “With the help of some new key targeting tools and further interpretation of the results of our initial drill campaign, our winter drilling program is off to a great start. We have now intersected the targeted mineralization 100 m to the east of the discovery holes, in what we believe is the extension of the same geophysical anomaly. The VTEM and ground MaxMin surveys clearly illustrate an extension of Percival, its associated anomaly, and provides additional guidance to the faulting that has occurred in the area. We believe this current drilling program will continue to expand the Percival discovery, while targeting this and other preeminent geophysical anomalies.”
Table 1: Significant Intercepts
Location Drill Hole From (m) To (m) Length (m) Grade (Au g/t) Vertical Depth (m) Host Lithology Percival ER19-832 1.3 54.0 52.7 1.86 25 Silicified Breccia incl. 1.3 31.0 29.7 2.81 also incl. 12.0 23.0 11.0 4.89 114.0 150.0 36.0 0.67 126 Silicified Mudstone Breccia incl. 143.5 150.0 6.5 1.52 244.0 247.5 3.5 0.98 234
Altered Mudstone Breccia
Percival ER19-833 101.8 124.0 22.2 1.84 86 Silicified Breccia incl. 102.6 111.0 8.4 3.76 also incl. 109.0 110.0 1.0 13.4 incl. 123.0 124.0 1.0 4.00 147.3 179.3 32.0 0.79 116 Silicified Breccia and Silicified Mudstone Breccia incl. 147.3 148.3 1.0 3.86 incl. 154.3 155.3 1.0 4.76 incl. 165.3 169.3 4.0 1.40 Incl. 177.3 179.3 2.0 2.7 200.0 204.0 4.0 1.52 143 Silicified Mudstone Breccia
Intervals are presented in core length; holes are generally planned to intersect mineralization as close to perpendicular to strike as possible; true widths are estimated to be 75% of downhole length when hole and dips of the mineralized horizons are considered.
Assays results presented are not capped. Intercepts occur within geological confines of major zones but have not been correlated to individual structures/horizons within these zones at this time.
Vertical depth is measured from the surface to the mid-point of the reported interval.
Geophysical Surveys
In January, Eastmain completed 16 line-kms of MaxMin Horizontal Loop Electro-Magnetic (HLEM) survey, on 50 m line spacings, centered over the discovery holes (see FIGURE 5). This survey was successful at identifying strong shallow EM responses to assist with drill collar placement in the Percival area. The MaxMin grid is being expanded in February to 32 line-kms, extending coverage to 1 km east and west of the Percival discovery holes, reaching westward to the Knight showing. Percival mineralization consists of significant concentration of sulphide (pyrrhotite + pyrite) mineralization which appears to respond well to the ground HLEM tool and will be used in concert with airborne VTEM and magnetic data to further stratigraphic interpretation near the discovery holes. Review and interpretation of the KS Horizon Helicopter-borne VTEM™ survey completed January 1, 2019, is ongoing with results anticipated to be incorporated in the Clearwater summer/fall exploration campaign.
Drilling Results
Hole ER19-832 was drilled east to west through the section of the Percival discovery holes ER18-822 and ER18-823, tracing Percival mineralization along plunge and seeking to intercept an interpreted fault located west of the discovery holes (see FIGURES 2 and 3). Drilling intersected silicified breccias from surface to 55 m downhole depth. This interval shows strong bedding and foliation sub-parallel to the core orientation. A minor fault structure was intersected at 58 m downhole. Silicified siltstone units are found on both sides of the fault which continues to have an inferred north strike with a steep dip to the NE. The presence of significant ductile deformation in core suggests that local folding and transposition may play a more important role in distribution of mineralization than late faulting. Gold mineralization was intersected in a second silicified mudstone breccia interval located west of the fault structure between 115 m and 140 m downhole. This mineralization is interpreted to be the extension of mineralization intersected in hole ER18-830 and ER18-831 (see press release Jan. 15, 2019).
Hole ER19-833 was collared 100 m east of the discovery holes to evaluate a strong ground electromagnetic HLEM conductor along trend and east of the Percival discovery holes. It is the easternmost drill hole to date at Percival, some 50 m east of holes ER18-826 and ER18-827 (see FIGURES 2 and 4). This hole intersected strongly silicified breccia mineralization 101 m to 128 m downhole and may represent the continuation of the Percival mineralization. A second interval of more variably mineralized, less silicified breccia and silicified mudstone breccia, was intersected from 145 m to 180 m downhole and is interpreted to correspond to a second, more northerly horizon of mineralized breccias previously intersected in holes ER18-825 (1.05 g/t Au over 6 m) and ER18-827 (0.50 g/t Au over 22.5 m) (see press release Dec. 20 2018).
The Percival Discovery
Percival is located 14 km ESE of the Company’s million-ounce Eau Claire gold deposit(1), within the 20-km long Cannard Deformation Zone (see FIGURE 1) and within the southern segment of the KS Horizon. Percival is a hydrothermal gold system hosted in a thick metasedimentary sequence with locally interbedded intermediate to felsic volcaniclastic rocks. Results from the latest drilling continue to provide further understanding of the mineralization controls, namely by defining lithological and structural settings, within the host stratigraphy while identifying at least two gold mineralized zones within the metasedimentary package (see FIGURES 2-4).
2019 Project Program and Objectives
2019 Winter Drill Program: A three-month, 20-hole (5,500 m), focused drilling campaign began in January; the program is designed to expand Percival while identifying new gold mineralization within the argillite-mudstone sedimentary package; building on the winter program results, a second extensive campaign will be planned for the second half of 2019 and will include additional drilling at the discovery area and test targets delineated along the KS Horizon using newly acquired geophysical and soil geochemistry information.
Improved interpretation at Percival: Focused drill testing of the Percival gold bearing zone along the KS Horizon, using stratigraphic and structural interpretation, to identify and improve predictability of potential mineralization offsets; detailed mineralogy and petrology to define the gold association as a further vector for drilling.
Geophysical Targeting: Use MaxMin HLEM to select and follow strong conductive trends related to gold mineralization; use results from the completed Helicopter-borne VTEM™ and Horizontal Magnetic Gradiometer Geophysical Survey (VTEM Plus), performed by Geotech along the KS Horizon, to help focus future work at Percival and on other prospective targets along the KS Horizon (see FIGURE 1).
Table 2: Drill Hole Information
Target Zone Drill Hole UTM Coordinates Zone 18 Azimuth Dip Total Length Elevation Number Easting Northing Degrees Degrees (m) (m) Percival ER19-832 457660 5781800 241.5 -72 301 336 Percival ER19-833 457750 5781775 360 -45 373 335
For additional information on the Geology of the Percival Discovery and the KS Horizon, please visit: http://www.eastmain.com/projects/clearwaterexploration/.
To view Figures 1-5, please click on the following link: http://www.eastmain.com/_resources/news/Images/ER-190225-Percival.pdf.
This press release was compiled and reviewed by William McGuinty, P.Geo., Eastmain’s VP Exploration, a Qualified Person under National Instrument 43-101.
(1) A total of 1,001,200 oz of contained gold in the combined open pit and underground diluted production schedule, as defined in Eastmain’s “Technical Report: Updated Mineral Resource Estimate and Preliminary Economic Assessment on the Eau Claire Gold Deposit, Clearwater Property, Quebec, Canada”. Effective date, February 4, 2018 and issued July 4, 2018. Contained ounces are derived from a combined open pit and underground mineral resource estimate of 853,000 oz Au (4.29 Mt at an average grade of 6.18 g/t Au) Measured & Indicated, and 500,000 oz Au (2.38 Mt at an average grade of 6.53 g/t Au) Inferred.
Quality Assurance and Quality Control (QA/QC)
The design of the Eastmain Resources’ drilling programs, Quality Assurance/Quality Control and interpretation of results is under the control of Eastmain’s geological staff, including qualified persons employing a strict QA/QC program consistent with NI 43-101 and industry best practices. The Clearwater project is supervised by Eastmain’s Project Geologist, Michel Leblanc P.Geo.
Drill core is logged and split with half-core samples packaged and delivered to ALS Minerals laboratory. Samples are dried and subsequently crushed to 70% passing a 2 mm mesh screen. A 1,000 grams subsample is pulverized to a nominal 85% passing 75-micron mesh screen. The remaining crushed sample (reject) and pulverized sample (pulp) are retained for further analysis and quality control. All samples are analysed by Fire Assay with an Atomic Absorption (AA) finish using a 50 g aliquot of pulverized material. Assays exceeding 5 g/t Au are re‐assayed by Fire Assay with a Gravimetric Finish. Eastmain regularly inserts 3rd party reference control samples and blank samples in the sample stream to monitor assay performance and performs duplicate sampling at a second certified laboratory. Approximately 10% of samples submitted are part of the Company’s laboratory sample control protocols.
About Eastmain Resources Inc. (TSX:ER) www.eastmain.com
Eastmain is a Canadian exploration company advancing three high-grade gold assets in the emerging James Bay gold camp in Québec. The Company holds a 100%-interest in the Clearwater Property, host of the Eau Claire Project, for which it issued a Preliminary Economic Assessment (“PEA”) in May 2018, and the Percival Discovery made in November 2018. Eastmain is also the operator of the Éléonore South Joint Venture, located immediately south of Goldcorp Inc.’s Éléonore Mine, which hosts the Moni/Contact Trend Discovery (2017). In addition, the Company has a 100% interest in the Eastmain Mine Project where the Company prepared a NI 43-101 Mineral Resource Estimate in January 2018, and a pipeline of exploration projects in this favourable mining jurisdiction with nearby infrastructure.
Forward- Looking Statements – Certain information set forth in this news release may contain forward-looking statements that involve substantial known and unknown risks and uncertainties. Forward-looking statements consist of statements that are not purely historical, including statements regarding beliefs, plans, expectations or timing of future plans, and include, but not limited to, statements with respect to the potential success of the Company’s future exploration and development strategies. These forward-looking statements are subject to numerous risks and uncertainties, certain of which are beyond the control of Eastmain, including, but not limited to the impact of general economic conditions, industry conditions, dependence upon regulatory approvals, the availability of financing, ýtimely completion of proposed studies and technical reports, and risks associated with the exploration, development and mining industry generally such as economic factors as they affect exploration, future commodity prices, changes in interest rates, safety and security, political, social or economic developments, environmental risks, insurance risks, capital expenditures, operating or technical difficulties in connection with development activities, personnel relations, the speculative nature of gold exploration and development, including the risks of diminishing quantities of grades of Mineral Resources, contests over property title, and changes in project parameters as plans continue to be refined. Readers are cautioned that the assumptions used in the preparation of such information, although considered reasonable at the time of preparation, may prove to be imprecise and, as such, undue reliance should not be placed on forward-looking statements. The Company assumes no obligation to update such information, except as may be required by law.
View source version on businesswire.com: https://www.businesswire.com/news/home/20190225005255/en/
Contacts
Claude Lemasson, President and CEO +1 647-347-3765 [email protected]
Alison Dwoskin, Manager, Investor Relations +1 647-347-3735 [email protected]
from Financial Post https://ift.tt/2tz6afK via IFTTT Blogger Mortgage Tumblr Mortgage Evernote Mortgage Wordpress Mortgage href="https://www.diigo.com/user/gelsi11">Diigo Mortgage
0 notes
Text
Forth Ports Places Order For Next Generation Damen ASD Tug 2312
Damen Shipyards Group has signed a contract with Forth Ports Limited for an ASD Tug 2312. The signing ceremony took place in Tilbury Docks, UK on 26th February.
Representing Damen was Area Director Frank de Lange, while Forth Ports was represented by COO Stuart Wallace. The new vessel will operate as part of the Forth Estuary Towage operation, conducting towage work at various Forth Ports locations as well as providing towage services to other external customers in Scotland.
Forth Ports has a range of existing operations in the Forth and Tay Estuaries that will benefit from the acquisition of this new vessel, however, the new vessel will also provide opportunities to service new emerging markets such as decommissioning and offshore wind.
Image Credits: damen.com
The ASD 2312 is well suited to operating in such an environment with such vessels; compact, but, with 70 tonnes bollard pull, extremely powerful.
She’s manoeuvrable too – thanks to her twin fin skeg and dual azimuth thrusters. The ASD Tug 2312 is a state-of-the-art vessel, one of Damen’s next generation tugs. The evolved character of the vessel is clearly visible in its safety features. The wheelhouse, for example, offers 86% window coverage, using impact resistant safety glass.
Meanwhile, the deck is obstacle free, reducing tripping hazards. The winch is located under the superstructure, which not only frees up space, but also facilitates towing operations both forward and aft. The tug is prepared for the future, being IMO Tierr III ready. Emissions are further reduced thanks to the tug’s smooth rounded bilge, an efficient profile that cuts fuel consumption. Damen has prepared a NOx Reduction System that can be applied to IMO Tier III ready vessels at any stage for full compliance.
Meanwhile, smart layouts and compliance with MLC 20006 crew accommodation and comfort regulations ensure the six-person crew are on top form at all times.
As a result of Damen’s philosophy of standardised shipbuilding, the ASD Tug 2312 was already under construction when Forth Ports placed their order with Damen, ensuring rapid delivery of the vessel.
While a standard platform, like all vessels in Damen’s portfolio, the ASD Tug 2312 is customisable via modular options. Forth Ports has selected a number of options for its vessel, including remote monitoring. This, ultimately, can significantly lower the total cost of ownership of the vessel. Amongst other things it provides feedback on performance so that sailing can be optimally efficient. It also informs whenever a part or component needs replacing, so that preventative maintenance can be carried out in order to maximise vessel uptime.
Furthermore, Damen will outfit Forth Ports’ ASD Tug 2312 with CCTV, FiFi capabilities, additional fendering at the bow and a winterisation package, amongst other options.
Damen Sales Manager UK Mike Besijn said, “We are delighted with this order from Forth Ports. The ASD Tug 2312 is a tug for the future that I am sure will serve them well in the years to come. Forth Ports has carefully evaluated their vessel requirements for both their current and future plans around fleet renewal and we are delighted that they have selected Damen for this replacement tug and we look forward to a long and growing relationship with Forth Ports.”
Alan McPherson, Chief Harbour Master at Forth Ports said, “We are very pleased to be working with Damen on the delivery of the 2312 to Forth Estuary Towage as we replace older tonnage and significantly increase our towage fleet capability. The team at Damen have worked closely with us to deliver a very capable vessel for the operations we undertake and our staff are looking forward to this type of tug within our fleet. I look forward to building our relationship with Damen in the coming years.”
As Forth Ports required their new tug to be operational as soon as possible, Damen Marine Services (DMS) stepped into to assist. While the new ASD Tug 2312 is under construction, DMS is providing ASD Tug 2811 Pioneer to operate with Forth Ports.
Reference: damen.com
Report an Error
from Storage Containers https://www.marineinsight.com/shipping-news/forth-ports-places-order-for-next-generation-damen-asd-tug-2312/ via http://www.rssmix.com/
0 notes
Text
Why India’s ASAT Test Was Reckless
India conducted its first successful anti-satellite (ASAT) test, dubbed “Mission Shakti,” on March 27, 2019. Using a so-called PDV Mark II missile, a modified version of India’s Prithvi Defense Vehicle (PDV) anti-ballistic-missile interceptor, India’s Defense Research and Development Organization (DRDO) destroyed the Microsat-r satellite orbiting at 285 kilometers in altitude. Microsat-r (COSPAR designation 2019-006A) was a 740 kilogram satellite launched by India two months earlier to serve as a target for the test.
In the aftermath of the test, accusations quickly emerged — including from NASA administrator Jim Bridenstine — that the debris generated by the test endangered other satellites. India was quick to claim it had acted “responsibly.” The Indian government pointed out that the test was performed at low altitude, below 300 km, in order to avoid creating debris at the altitudes of operational satellites in Low Earth Orbit (many of which orbit at altitudes between 400 and 1200 km).
In a press conference, DRDO chairman Dr. Sateesh Reddy, in addition, claimed that the kill-vehicle hit Microsat-r in what he described as an “almost direct hit in the same plane,” i.e. head-on. This was done — or so it was claimed — in order to minimize the ejection of debris fragments into higher orbits. The Indian government maintains that the test yielded a negligible risk to operational satellites at higher altitudes. It has claimed that most debris fragments re-entered into the Earth’s atmosphere within two days of the test, and that all of it would re-enter within 45 days.
Enjoying this article? Click here to subscribe for full access. Just $5 a month.
But are these Indian government claims true? An in-depth analysis of publicly available data from both DRDO and U.S. military sources shows that this test wasn’t conducted as “responsibly” as the Indian government claims. Debris did end up orbiting at higher altitudes well within the altitude range of operational satellites, including the International Space Station (ISS). The debris fragments currently being tracked by the U.S. military’s tracking network will take considerably more than 45 days to re-enter into the atmosphere. Moreover, telemetry data included in a video released by the Indian DRDO indicates that the kill vehicle did not hit the target satellite head-on as the DRDO claims, but under a clear upwards angle, which would eject fragments to higher orbits.
The Timing of the ASAT Test
From information released by the DRDO, we know that the PDV-Mk II missile was launched from the missile test range at Abdul Kalam island (formerly known as Wheeler island) on the Indian coast around 11:09 a.m. Indian Standard Time (5:39 a.m. GMT). This time was independently confirmed by U.S. military sources, likely based on observations by their space-based infrared system (SBIRS) early warning satellites. Indian sources say the missile had a flight-time of 168 seconds. From a video released by DRDO showing the kill vehicle’s impact into Microsat-r as imaged by an earth-based infrared camera on the Indian coast, we can pinpoint the intercept time at 5:42:15.5 GMT. This would place the launch as having taken place around 5:39:27 GMT. The orbital position of the target satellite at 5:42:15.5 GMT, the moment of intercept, was within a kilometer of 18°.715 N, 87°.450 E at an altitude of 284 km.
Fig. 1: map showing the approach trajectory of the target satellite (white), the missile launch site and intercept location (yellow), and the hazard area for the test (red) from a Maritime Area Warning. Map by the author.
Fig. 2: 3D reconstruction of the approximate intercept geometry, created by the author using STK. This reconstruction modelled a direct ballistic ascent.
An OSINT Treasure Trove
Shortly after the test, DRDO released a propaganda video that contains two highly interesting fragments of video footage from the actual test. One is a short sequence of images from the infrared target seeking camera on the missile itself. The other is a sequence of footage taken by an earth-based infrared camera on the Indian coast.
It is the latter footage that contains a lot of information useful for the purpose of this analysis and is, in fact, an OSINT treasure trove. A clock running in the footage yields an accurate time of the kill vehicle impact. But more importantly, the footage appears to contain telemetry information from the missile: azimuth (direction), elevation, and range (distance) to the camera. The footage covers the last 2.7 seconds of the missile’s flight up to impact, and some 2 seconds after the impact as well, showing the expanding debris cloud.
Fig 3: still frame from DRDO released video footage of the intercept, as captured by an infrared camera on the Indian coast. Yellow annotations indicate missile telemetry data in the video frame.
Based on U.S. tracking data from U.S. Strategic Command’s Combined Space Operations Center (CSpSOC), we know the position of Microsat-r, the target satellite, at the time of impact. We therefore can use the missile’s azimuth, elevation, and range information in the video frame showing the impact moment to find the location of the earth-based camera. This camera was located on the Indian coast near 21°.34 N, 86°.91 E, some 15 km southwest of Chandipur ITR. This location is near the point where the forward prolonged trajectory of the target satellite crossed the Indian coast. As the satellite position has an approximately 1 km uncertainty inherent to orbital elements in CSpOC’s two-line element (TLE) set format, there is a similar uncertainty in the camera location.
Fig 4: reconstructed location of the infrared camera.
Knowing the camera location, we can next use the telemetry data in the frames to reconstruct the trajectory of the missile during the last 2.7 seconds prior to impact with Microsat-r, and compare it to the trajectory of Microsat-r itself.
In order to do this, we can create a flat plane through the camera sensor, tangent to the earth surface at the camera location. Azimuth, elevation and range data from the footage then give us Cartesian positions and elevations with respect to this reference plane. In this way, we don’t have to be bothered by the earth’s curvature, and it greatly simplifies calculations. We are only interested in the relative geometry of the satellite and missile tracks, so this approach is sufficient.
Fig 5: graphical representation of the reference frame used, a flat plane through the sensor location tangent to the earth surface.
In the diagrams that follow, positions are expressed as delta X and delta Y (in km) in the reference plane with respect to the camera location, and altitude over the reference plane (note: this is not the same as the altitude above the earth’s surface). The Y-axis points north, the X-axis east. As we had to work with limited resolution frames extracted from a YouTube video, occasional errors in deciphering the telemetry numbers cause some small occasional jitters in the reconstructed missile trajectory. Nevertheless, the results are very consistent.
The first diagram shows the missile and satellite trajectories when viewed top-down (i.e. “from above”): black is the missile and red the satellite. The solid lines give the trajectories up to the moment of impact, the dashed lines give post-impact trajectories. The approximately 1 km positional error in the satellite’s trajectory is indicated in grey.
Seen from this perspective, the hit appears to be almost head-on, with the missile first crossing the satellite trajectory from the east and then impacting while homing in slightly from the west, relative to the satellite’s movement vector. The latter is, as we will see, in keeping with two other lines of evidence.
Fig 6: missile trajectory in the horizontal plane, reconstructed from telemetry data in the DRDO video.
When we look at the missile trajectory from a vertical perspective, i.e. “from the side”, the situation looks quite different, with interesting implications. It becomes clear that, far from hitting “head-on,” the missile hit with a clear upwards angle of approximately 48 degrees with the horizontal (or 135 degrees with respect to the satellite’s direction of movement).
This runs counter to DRDO chairman Sateesh Reddy’s assertion of a “head-on” hit designed to reduce the risk of debris being ejected into higher orbits. An impact coming from below increases the risk of fragments being ejected to altitudes above the target satellite’s original orbit.
Fig 7: missile trajectory in the vertical plane, reconstructed from telemetry data in the DRDO video.
A second line of evidence — footage from the infrared target seeking camera on the missile itself — also indicates a hit coming from below, in an upwards direction. The last frames of this footage show the infrared silhouette of the satellite, including its solar panels. They appear to show the latter full-on:
Fig 8: final image of the target seeking camera on the missile, showing the solar panels of the target satellite, from the DRDO video.
Would the impact have been “head-on,” i.e. moving into a direction opposite to the satellite’s movement vector, the solar panels should not have been visible full-on, but edge-on, given the sun-satellite-missile geometry at that moment. Solar panels are normally pointed toward the sun. A hit under an upwards angle, by contrast, would show the solar panels almost head-on, as in the footage. Hence, like the earth-based footage, the footage from the missile target seeking camera contradicts a “head-on” hit. These two lines of evidence are therefore consistent, supporting the same conclusion: that DRDO’s claims about the nature of the intercept were not entirely true.
Fig 9: Geometry reconstructions for two intercept scenarios by the author, using STK. One shows a “head-on”hit, the other a hit coming under an upwards angle from below. Note the differing views of the solar panels in both scenarios.
Interestingly enough, and perhaps unintentionally telling, the DRDO propaganda video has an animation of the intercept that at one point also features a steep upwards angle, consistent with a direct ballistic ascent.
Fig 10: Still image from DRDO video showing a graphical depiction of the missile trajectory. Compare to the author’s reconstruction.
Expanding Debris Cloud
After the kill vehicle hit the satellite, an expanding debris cloud was created that quickly spread. Knowing the position of the camera, we can actually plot the trajectory of the target satellite on the infrared imagery of this rapidly expanding debris cloud. We see the cloud expanding along the satellite track, notably in a forward direction (which is logical, as with an impact coming from below, the forward momentum from the 7.8 km/s moving satellite is the largest contributor to debris fragment speed vectors).
Fig 11: satellite trajectory as seen from the camera location, as overlaid on a frame from the DRDO video showing the expanding debris cloud. Satellite movement was from bottom to top.
Relative Speed of Impact
From the telemetry-based trajectory reconstruction, the missile reached a speed on the order of 3.1 to 3.4 km/s. Taking into account the 7.8 km/s orbital speed of the satellite, as well as the directions of their movement vectors, a relative speed in the order of 9.9-10.0 km/s on impact is indicated. This is close to the 9.8 km/s value given by U.S. military sources. It is less than the relative speed indicated in the missile’s target seeking camera footage (10.45 km/s).
Post-Impact Trajectory
Interestingly enough, the footage also includes two seconds of missile telemetry after the impact, suggesting part of the missile survived the impact. This post-impact trajectory is indicated by the dashed line in the trajectory reconstructions. In the vertical plane, we see the missile rip through the position of the satellite, the missile remnant continuing its upwards movement, perhaps under a slightly reduced angle. In the horizontal plane (i.e. seen from “above”) we see the missile remnant being clearly deflected westwards by the force of the impact, pretty much like a billiards ball hitting another ball under an oblique angle. This indicates a hit which, seen in the horizontal plane, came under a slight angle with the satellite’s movement vector. This matches information gleaned from tracked debris fragments, as I will outline below.
Debris Fragments Created
The U.S. military’s satellite tracking network, run by CSpOC, has reported it is tracking several hundreds of debris fragments created by the test. At the date of writing, CSpOC has published orbital elements for 84 of these fragments. As these 84 fragments concerns a subset of larger, well tracked fragments out of a much larger not-well or not-at-all tracked fragment population only, these orbits constitute the tip of an iceberg. The orbital elements illustrate the large spread in orbital altitudes of the debris fragments created by the test. The image below shows the orbits of these fragments (red), with the orbit of the ISS (white) shown as comparison:
Fig 12: orbits of debris fragments (red), with the orbit of the International Space Station (white) as a comparison.
One insightful way to present the orbital altitudes of these fragments and assess these at a glance is through a so-called “Gabbard” diagram. Such a diagram shows, for each fragment, the lowest point in its elliptical orbit (its perigee, in red) and the highest point in its orbit (its apogee, blue), as well as the time it takes to complete one orbit around the earth.
While the perigees of the debris fragments are distributed near the altitude of the ASAT test (285 km), the apogees spread over a large range in altitude, up to as high as 2,250 km. Some 79 percent of the tracked fragments have apogee altitudes above the orbital altitude of the International Space Station. The strongest concentration is between 200 km and 900 km altitude (see also the bar diagram), well into the realm of the orbital altitudes of many commercial as well as scientific and military satellites. Hence, these fragments clearly are an impact-threat to other satellites, in contrast to Indian government claims of minimal risks. Close approach tools like SOCRATES show that several debris fragments already have had close approaches to other satellites since the test, and that such potentially dangerous close approaches will continue to happen over the coming weeks and months.
Fig 13: Gabbard diagram showing apogee and perigee altitudes of debris fragments. ISS orbital altitude indicated as comparison.
Fig 14: bar diagram of the distribution of debris fragment apogee altitudes. ISS orbital altitude indicated as comparison.
These results should not come as a surprise, to India or anyone else. In 2008, the United States conducted “Operation Burnt Frost,” an ASAT demonstration on the malfunctioned USA 193 satellite. That intercept, like the Indian ASAT test, was at low altitude (247 km), but a significant number of fragments nevertheless ended up in much higher orbits.
Orbital Lifetimes of the Fragments
How long will it take before most of the debris created by the Indian ASAT test is cleared from orbit? The Indian government claims most fragments re-entered within hours to days, and that the rest will re-enter within 45 days of the test.
The vast majority of very small fragments created by the ASAT test will likely indeed have re-entered within hours to days of the test. But as we already have seen, there are still plenty that did not. For these, we can estimate orbital lifetimes from the decay parameters of their orbits and an educated guess of future solar activity (solar activity influences the density of the outer layers of the atmosphere). I used SatEvo software and current solar activity values to estimate the re-entry dates of the currently tracked fragments. It suggests that a significant number — almost 50 percent — of the tracked fragments have orbital lifetimes well beyond the 45 days claimed by the Indian government, as the diagram below clearly shows. Rather than 45 days, a better estimate for the maximum debris lifetime would be 45 weeks. Some fragments might even linger on until almost two years after the test.
Fig 15: orbital lifetime estimates of the currently tracked debris fragments.
Ejection Velocities
The orbital data for the 84 debris fragments tracked by CSpOC can be used to calculate the ejection velocities of these fragments. The ejection velocity, or delta V, is the extra velocity that was required to eject these fragments into their current orbits, and can be calculated from the change in orbital altitude and orbital inclination for each fragment. For the 84 fragments for which orbital elements are currently available, I calculate ejection velocities in the range of 10 to 300 meter/second, with a few having ejection speeds up to 500 meter/second. The range of ejection velocities is similar to that of the 2008 ASAT demonstration on USA 193 by the Americans. The peak in the delta V distribution of the Indian ASAT test is, however, shifted toward higher ejection velocities compared to the 2008 American ASAT demonstration: the distribution peaks near 100 m/s, while for the 2008 USA 193 intercept it peaks near 40 m/s. On average, the ejection speeds in the Indian ASAT test hence appear to have been somewhat higher than in the 2008 American test, creating peak debris densities at somewhat higher altitudes as a result. The difference might result from a difference in impact angle: the American ASAT test reportedly featured an impact on a downward angle, while the Indian test featured an upwards impact angle, as the above analysis shows. Some caution is in order here, though, as instrumental detection bias might have an unknown influence on the range of orbital altitudes mapped so far.
Fig 16: delta V distribution for the currently tracked debris fragments.
Fig 17: delta V distribution kernel density curves for the Indian ASAT test fragments, compared to a similar curve for the 2008 American ASAT demonstration on USA 193. Note the difference in peak position and fall-off slope.
Orbital Inclinations
As seen in a horizontal plane (i.e. from “above”), the kill vehicle was not exactly moving counter to the satellite, but under a very slight angle, with the kill vehicle coming slightly from the west with regard to the satellite’s movement vector. The clear westward deflection of the missile remnant after the impact, also points to this.
This hit under a small angle from the west is also born out by the orbits of the resulting debris fragments. The distribution of orbital inclinations of these fragments (the angle their orbits make with the equator) shows a shift towards smaller inclinations, by 0.5 degrees or more, compared to the original Microsat-r orbit. This again points to an impact coming from slightly west of the satellite’s vector of movement.
Fig 18: distribution of orbital inclination of the debris fragments, compared to the orbital inclination of the target satellite Microsat-r.
Conclusions
We can draw a number of conclusions from this analysis. The main conclusion is that the ASAT test was conducted in a less responsible way than originally claimed by the Indian government. First, the missile hit the target satellite on a clear upwards angle, rather than “head-on” as claimed by DRDO. Second and third, the test generated debris with much longer orbital lifetimes (up to 10 times longer), which ended up at much higher altitudes than the Indian government is willing to admit.
As much as 79 percent of the larger debris fragments tracked have apogee altitudes at or above the orbit of the International Space Station. Most of the tracked debris generated by the test orbits between 300 km and 900 km altitude, well into the range of typical orbital altitudes for satellites in Low Earth Orbit. As these debris fragments are in polar orbits, they are a potential threat to satellites in all orbital inclinations at these altitudes. Indeed, several close approaches to satellites have already happened. This threat will persist for up to half a year (rather than the 45 days claimed by the Indian government), with a few fragments lingering on (much) longer, up to almost two years.
The analysis underlines that a ‘harmless’ ASAT test involving a real intercept of an orbiting target does not exist. The Indian ASAT test and the earlier 2008 American ASAT demonstration show that even intercepts at low altitude create lots of debris that is ejected into higher orbits. And the Indian government in this case made it worse by hitting Microsat-r under an upwards angle, rather than head-on or under a downward angle. As such, this ASAT test was reckless.
Dr. Marco Langbroek is a Space Situational Awareness consultant from the Netherlands.
The post Why India’s ASAT Test Was Reckless appeared first on Defence Online.
from WordPress https://defenceonline.com/2019/05/01/why-indias-asat-test-was-reckless/
0 notes
Text
Solution Manual for Elementary Surveying An Introduction to Geomatics 13th Edition by Ghilani Wolf
This is Full Solution Manual for Elementary Surveying: An Introduction to Geomatics, 13th Edition Charles D. Ghilani and Wolf
Click link bellow to view sample:
http://digitalcontentmarket.org/wp-content/uploads/2017/01/Solution-Manual-for-Elementary-Surveying-An-Introduction-to-Geomatics-13th-Edition-Charles-D-Ghilani.pdf
Origin Book information:
ISBN-10: 0132554348
ISBN-13: 978-0132554343 9780132554343
Full download link: Solution Manual for Elementary Surveying An Introduction to Geomatics 13th Edition by Ghilani Wolf
http://digitalcontentmarket.org/download/solution-manual-for-elementary-surveying-an-introduction-to-geomatics-13th-edition-charles-d-ghilani
You will be guided to the product download page immediately once you complete the payment.If you have any questions, or would like a receive a sample chapter before your purchase, please contact us via email :
Table of Contents
1 • INTRODUCTION 1 1.1 Definition of Surveying 1 1.2 Geomatics 3 1.3 History of Surveying 4 1.4 Geodetic and Plane Surveys 9 1.5 Importance of Surveying 10 1.6 Specialized Types of Surveys 11 1.7 Surveying Safety 13 1.8 Land and Geographic Information Systems 14 1.9 Federal Surveying and Mapping Agencies 15 1.10 The Surveying Profession 16 1.11 Professional Surveying Organizations 17 1.12 Surveying on the Internet 18 1.13 Future Challenges in Surveying 19 Problems 20 Bibliography 21
2 • UNITS, SIGNIFICANT FIGURES, AND FIELD NOTES 23 PART I UNITS AND SIGNIFICANT FIGURES 23 2.1 Introduction 23 2.2 Units of Measurement 23 2.3 International System of Units (SI) 25 2.4 Significant Figures 27 2.5 Rounding Off Numbers 29 PART II FIELD NOTES 30 2.6 Field Notes 30 2.7 General Requirements of Handwritten Field Notes 31 2.8 Types of Field Books 32 2.9 Kinds of Notes 33 2.10 Arrangements of Notes 33 2.11 Suggestions for Recording Notes 35 2.12 Introduction to Data Collectors 36 2.13 Transfer of Files from Data Collectors 39 2.14 Digital Data File Management 41 2.15 Advantages and Disadvantages of Data Collectors 42 Problems 43 Bibliography 44
3 • THEORY OF ERRORS IN OBSERVATIONS 45 3.1 Introduction 45 3.2 Direct and Indirect Observations 45 3.3 Errors in Measurements 46 3.4 Mistakes 46 3.5 Sources of Errors in Making Observations 47 3.6 Types of Errors 47 3.7 Precision and Accuracy 48 3.8 Eliminating Mistakes and Systematic Errors 49 3.9 Probability 49 3.10 Most Probable Value 50 3.11 Residuals 51 3.12 Occurrence of Random Errors 51 3.13 General Laws of Probability 55 3.14 Measures of Precision 55 3.15 Interpretation of Standard Deviation 58 3.16 The 50, 90, and 95 Percent Errors 58 3.17 Error Propagation 60 3.18 Applications 65 3.19 Conditional Adjustment of Observations 65 3.20 Weights of Observations 66 3.21 Least-Squares Adjustment 67 3.22 Using Software 68 Problems 69 Bibliography 71
4 • LEVELING–THEORY, METHODS, AND EQUIPMENT 73 PART I LEVELING–THEORY AND METHODS 73 4.1 Introduction 73 4.2 Definitions 73 4.3 North American Vertical Datum 75 4.4 Curvature and Refraction 76 4.5 Methods for Determining Differences in Elevation 78 PART II EQUIPMENT FOR DIFFERENTIAL LEVELING 85 4.6 Categories of Levels 85 4.7 Telescopes 86 4.8 Level Vials 87 4.9 Tilting Levels 89 4.10 Automatic Levels 90 4.11 Digital Levels 91 4.12 Tripods 93 4.13 Hand Level 93 4.14 Level Rods 94 4.15 Testing and Adjusting Levels 96 Problems 100 Bibliography 102
5 • LEVELING–FIELD PROCEDURES AND COMPUTATIONS 103 5.1 Introduction 103 5.2 Carrying and Setting Up a Level 103 5.3 Duties of a Rodperson 105 5.4 Differential Leveling 106 5.5 Precision 112 5.6 Adjustments of Simple Level Circuits 113 5.7 Reciprocal Leveling 114 5.8 Three-Wire Leveling 115 5.9 Profile Leveling 117 5.10 Grid, Cross-Section, or Borrow-Pit Leveling 121 5.11 Use of the Hand Level 122 5.12 Sources of Error in Leveling 122 5.13 Mistakes 124 5.14 Reducing Errors and Eliminating Mistakes 125 5.15 Using Software 125 Problems 127 Bibliography 129
6 • DISTANCE MEASUREMENT 131 PART I METHODS FOR MEASURING DISTANCES 131 6.1 Introduction 131 6.2 Summary of Methods for Making Linear Measurements 131 6.3 Pacing 132 6.4 Odometer Readings 132 6.5 Optical Rangefinders 133 6.6 Tacheometry 133 6.7 Subtense Bar 133 PART II DISTANCE MEASUREMENTS BY TAPING 133 6.8 Introduction to Taping 133 6.9 Taping Equipment and Accessories 134 6.10 Care of Taping Equipment 135 6.11 Taping on Level Ground 136 6.12 Horizontal Measurements on Sloping Ground 138 6.13 Slope Measurements 140 6.14 Sources of Error in Taping 141 6.15 Tape Problems 145 6.16 Combined Corrections in a Taping Problem 147 PART III ELECTRONIC DISTANCE MEASUREMENT 148 6.17 Introduction 148 6.18 Propagation of Electromagnetic Energy 149 6.19 Principles of Electronic Distance Measurement 152 6.20 Electro-Optical Instruments 153 6.21 Total Station Instruments 156 6.22 EDM Instruments Without Reflectors 157 6.23 Computing Horizontal Lengths from Slope Distances 158 6.24 Errors in Electronic Distance Measurement 160 6.25 Using Software 165 Problems 165 Bibliography 168
7 • ANGLES, AZIMUTHS, AND BEARINGS 169 7.1 Introduction 169 7.2 Units of Angle Measurement 169 7.3 Kinds of Horizontal Angles 170 7.4 Direction of a Line 171 7.5 Azimuths 172 7.6 Bearings 173 7.7 Comparison of Azimuths and Bearings 174 7.8 Computing Azimuths 175 7.9 Computing Bearings 177 7.10 The Compass and the Earth’s Magnetic Field 179 7.11 Magnetic Declination 180 7.12 Variations in Magnetic Declination 181 7.13 Software for Determining Magnetic Declination 183 7.14 Local Attraction 184 7.15 Typical Magnetic Declination Problems 185 7.16 Mistakes 187 Problems 187 Bibliography 189
8 • TOTAL STATION INSTRUMENTS; ANGLE OBSERVATIONS 191 PART I TOTAL STATION INSTRUMENTS 191 8.1 Introduction 191 8.2 Characteristics of Total Station Instruments 191 8.3 Functions Performed by Total Station Instruments 194 8.4 Parts of a Total Station Instrument 195 8.5 Handling and Setting Up a Total Station Instrument 199 8.6 Servo-Driven and Remotely Operated Total Station Instruments 201 PART II ANGLE OBSERVATIONS 203 8.7 Relationship of Angles and Distances 203 8.8 Observing Horizontal Angles with Total Station Instruments 204 8.9 Observing Horizontal Angles by the Direction Method 206 8.10 Closing the Horizon 207 8.11 Observing Deflection Angles 209 8.12 Observing Azimuths 211 8.13 Observing Vertical Angles 211 8.14 Sights and Marks 213 8.15 Prolonging a Straight Line 214 8.16 Balancing-In 216 8.17 Random Traverse 217 8.18 Total Stations for Determining Elevation Differences 218 8.19 Adjustment of Total Station Instruments and Their Accessories 219 8.20 Sources of Error in Total Station Work 222 8.21 Propagation of Random Errors in Angle Observations 228 8.22 Mistakes 228 Problems 229 Bibliography 230
9 • TRAVERSING 231 9.1 Introduction 231 9.2 Observation of Traverse Angles or Directions 233 9.3 Observation of Traverse Lengths 234 9.4 Selection of Traverse Stations 235 9.5 Referencing Traverse Stations 235 9.6 Traverse Field Notes 237 9.7 Angle Misclosure 238 9.8 Traversing with Total Station Instruments 239 9.9 Radial Traversing 240 9.10 Sources of Error in Traversing 241 9.11 Mistakes in Traversing 242 Problems 242
10 • TRAVERSE COMPUTATIONS 245 10.1 Introduction 245 10.2 Balancing Angles 246 10.3 Computation of Preliminary Azimuths or Bearings 248 10.4 Departures and Latitudes 249 10.5 Departure and Latitude Closure Conditions 251 10.6 Traverse Linear Misclosure and Relative Precision 251 10.7 Traverse Adjustment 252 10.8 Rectangular Coordinates 255 10.9 Alternative Methods for Making Traverse Computations 256 10.10 Inversing 260 10.11 Computing Final Adjusted Traverse Lengths and Directions 261 10.12 Coordinate Computations in Boundary Surveys 263 10.13 Use of Open Traverses 265 10.14 State Plane Coordinate Systems 268 10.15 Traverse Computations Using Computers 269 10.16 Locating Blunders in Traverse Observations 269 10.17 Mistakes in Traverse Computations 272 Problems 272 Bibliography 275
11 • COORDINATE GEOMETRY IN SURVEYING CALCULATIONS 277 11.1 Introduction 277 11.2 Coordinate Forms of Equations for Lines and Circles 278 11.3 Perpendicular Distance from a Point to a Line 280 11.4 Intersection of Two Lines, Both Having Known Directions 282 11.5 Intersection of a Line with a Circle 284 11.6 Intersection of Two Circles 287 11.7 Three-Point Resection 289 11.8 Two-Dimensional Conformal Coordinate Transformation 292 11.9 Inaccessible Point Problem 297 11.10 Three-Dimensional Two-Point Resection 299 11.11 Software 302 Problems 303 Bibliography 307
12 • AREA 309 12.1 Introduction 309 12.2 Methods of Measuring Area 309 12.3 Area by Division Into Simple Figures 310 12.4 Area by Offsets from Straight Lines 311 12.5 Area by Coordinates 313 12.6 Area by Double-Meridian Distance Method 317 12.7 Area of Parcels with Circular Boundaries 320 12.8 Partitioning of Lands 321 12.9 Area by Measurements from Maps 325 12.10 Software 327 12.11 Sources of Error in Determining Areas 328 12.12 Mistakes in Determining Areas 328 Problems 328 Bibliography 330
13 • GLOBAL NAVIGATION SATELLITE SYSTEMS—INTRODUCTION AND PRINCIPLES OF OPERATION 331 13.1 Introduction 331 13.2 Overview of GPS 332 13.3 The GPS Signal 335 13.4 Reference Coordinate Systems 337 13.5 Fundamentals of Satellite Positioning 345 13.6 Errors in Observations 348 13.7 Differential Positioning 356 13.8 Kinematic Methods 358 13.9 Relative Positioning 359 13.10 Other Satellite Navigation Systems 362 13.11 The Future 364 Problems 365 Bibliography 366
14 • GLOBAL NAVIGATION SATELLITE SYSTEMS—STATIC SURVEYS 367 14.1 Introduction 367 14.2 Field Procedures in Satellite Surveys 369 14.3 Planning Satellite Surveys 372 14.4 Performing Static Surveys 384 14.5 Data Processing and Analysis 386 14.6 Sources of Errors in Satellite Surveys 393 14.7 Mistakes in Satellite Surveys 395 Problems 395 Bibliography 397
15 • GLOBAL NAVIGATION SATELLITE SYSTEMS—KINEMATIC SURVEYS 399 15.1 Introduction 399 15.2 Planning of Kinematic Surveys 400 15.3 Initialization 402 15.4 Equipment Used in Kinematic Surveys 403 15.5 Methods Used in Kinematic Surveys 405 15.6 Performing Post-Processed Kinematic Surveys 408 15.7 Communication in Real-Time Kinematic Surveys 411 15.8 Real-Time Networks 412 15.9 Performing Real-Time Kinematic Surveys 413 15.10 Machine Control 414 15.11 Errors in Kinematic Surveys 418 15.12 Mistakes in Kinematic Surveys 418 Problems 418 Bibliography 419
16 • ADJUSTMENTS BY LEAST SQUARES 421 16.1 Introduction 421 16.2 Fundamental Condition of Least Squares 423 16.3 Least-Squares Adjustment by the Observation Equation Method 424 16.4 Matrix Methods in Least-Squares Adjustment 428 16.5 Matrix Equations for Precisions of Adjusted Quantities 430 16.6 Least-Squares Adjustment of Leveling Circuits 432 16.7 Propagation of Errors 436 16.8 Least-Squares Adjustment of GNSS Baseline Vectors 437 16.9 Least-Squares Adjustment of Conventional Horizontal Plane Surveys 443 16.10 The Error Ellipse 452 16.11 Adjustment Procedures 457 16.12 Other Measures of Precision for Horizontal Stations 458 16.13 Software 460 16.14 Conclusions 460 Problems 461 Bibliography 466
17 • MAPPING SURVEYS 467 17.1 Introduction 467 17.2 Basic Methods for Performing Mapping Surveys 468 17.3 Map Scale 468 17.4 Control for Mapping Surveys 470 17.5 Contours 471 17.6 Characteristics of Contours 474 17.7 Direct and Indirect Methods of Locating Contours 474 17.8 Digital Elevation Models and Automated Contouring Systems 477 17.9 Basic Field Methods for Locating Topographic Details 479 17.10 Three-Dimensional Conformal Coordinate Transformation 488 17.11 Selection of Field Method 489 17.12 Working with Data Collectors and Field-to-Finish Software 490 17.13 Hydrographic Surveys 493 17.14 Sources of Error in Mapping Surveys 497 17.15 Mistakes in Mapping Surveys 498 Problems 498 Bibliography 500
18 • MAPPING 503 18.1 Introduction 503 18.2 Availability of Maps and Related Information 504 18.3 National Mapping Program 505 18.4 Accuracy Standards for Mapping 505 18.5 Manual and Computer-Aided Drafting Procedures 507 18.6 Map Design 508 18.7 Map Layout 510 18.8 Basic Map Plotting Procedures 512 18.9 Contour Interval 514 18.10 Plotting Contours 514 18.11 Lettering 515 18.12 Cartographic Map Elements 516 18.13 Drafting Materials 519 18.14 Automated Mapping and Computer-Aided Drafting Systems 519 18.15 Impacts of Modern Land and Geographic Information Systems on Mapping 525 18.16 Sources of Error in Mapping 526 18.17 Mistakes in Mapping 526 Problems 526 Bibliography 528
19 • CONTROL SURVEYS AND GEODETIC REDUCTIONS 529 19.1 Introduction 529 19.2 The Ellipsoid and Geoid 530 19.3 The Conventional Terrestrial Pole 532 19.4 Geodetic Position and Ellipsoidal Radii of Curvature 534 19.5 Geoid Undulation and Deflection of the Vertical 536 19.6 U.S. Reference Frames 538 19.7 Accuracy Standards and Specifications for Control Surveys 547 19.8 The National Spatial Reference System 550 19.9 Hierarchy of the National Horizontal Control Network 550 19.10 Hierarchy of the National Vertical Control Network 551 19.11 Control Point Descriptions 551 19.12 Field Procedures for Traditional Horizontal Control Surveys 554 19.13 Field Procedures for Vertical Control Surveys 559 19.14 Reduction of Field Observations to Their Geodetic Values 564 19.15 Geodetic Position Computations 577 19.16 The Local Geodetic Coordinate System 580 19.17 Three-Dimensional Coordinate Computations 581 19.18 Software 584 Problems 584 Bibliography 587
20 • STATE PLANE COORDINATES AND OTHER MAP PROJECTIONS 589 20.1 Introduction 589 20.2 Projections Used in State Plane Coordinate Systems 590 20.3 Lambert Conformal Conic Projection 593 20.4 Transverse Mercator Projection 594 20.5 State Plane Coordinates in NAD27 and NAD83 595 20.6 Computing SPCS83 Coordinates in the Lambert Conformal Conic System 596 20.7 Computing SPCS83 Coordinates in the Transverse Mercator System 601 20.8 Reduction of Distances and Angles to State Plane Coordinate Grids 608 20.9 Computing State Plane Coordinates of Traverse Stations 617 20.10 Surveys Extending from One Zone to Another 620 20.11 Conversions Between SPCS27 and SPCS83 621 20.12 The Universal Transverse Mercator Projection 622 20.13 Other Map Projections 623 20.14 Map Projection Software 627 Problems 628 Bibliography 631
21 • BOUNDARY SURVEYS 633 21.1 Introduction 633 21.2 Categories of Land Surveys 634 21.3 Historical Perspectives 635 21.4 Property Description by Metes and Bounds 636 21.5 Property Description by Block-and-Lot System 639 21.6 Property Description by Coordinates 641 21.7 Retracement Surveys 641 21.8 Subdivision Surveys 644 21.9 Partitioning Land 646 21.10 Registration of Title 647 21.11 Adverse Possession and Easements 648 21.12 Condominium Surveys 648 21.13 Geographic and Land Information Systems 655 21.14 Sources of Error in Boundary Surveys 655 21.15 Mistakes 655 Problems 656 Bibliography 658
22 • SURVEYS OF THE PUBLIC LANDS 659 22.1 Introduction 659 22.2 Instructions for Surveys of the Public Lands 660 22.3 Initial Point 663 22.4 Principal Meridian 664 22.5 Baseline 665 22.6 Standard Parallels (Correction Lines) 666 22.7 Guide Meridians 666 22.8 Township Exteriors, Meridional (Range) Lines, and Latitudinal (Township) Lines 667 22.9 Designation of Townships 668 22.10 Subdivision of a Quadrangle into Townships 668 22.11 Subdivision of a Township into Sections 670 22.12 Subdivision of Sections 671 22.13 Fractional Sections 672 22.14 Notes 672 22.15 Outline of Subdivision Steps 672 22.16 Marking Corners 674 22.17 Witness Corners 674 22.18 Meander Corners 675 22.19 Lost and Obliterated Corners 675 22.20 Accuracy of Public Lands Surveys 678 22.21 Descriptions by Township Section and Smaller Subdivision 678 22.22 BLM Land Information System 679 22.23 Sources of Error 680 22.24 Mistakes 680 Problems 681 Bibliography 683
23 • CONSTRUCTION SURVEYS 685 23.1 Introduction 685 23.2 Specialized Equipment for Construction Surveys 686 23.3 Horizontal and Vertical Control 689 23.4 Staking Out a Pipeline 691 23.5 Staking Pipeline Grades 692 23.6 Staking Out a Building 694 23.7 Staking Out Highways 698 23.8 Other Construction Surveys 703 23.9 Construction Surveys Using Total Station Instruments 704 23.10 Construction Surveys Using GNSS Equipment 706 23.11 Machine Guidance and Control 709 23.12 As-Built Surveys with Laser Scanning 710 23.13 Sources of Error in Construction Surveys 711 23.14 Mistakes 712 Problems 712 Bibliography 714
24 • HORIZONTAL CURVES 715 24.1 Introduction 715 24.2 Degree of Circular Curve 716 24.3 Definitions and Derivation of Circular Curve Formulas 718 24.4 Circular Curve Stationing 720 24.5 General Procedure of Circular Curve Layout by Deflection Angles 721 24.6 Computing Deflection Angles and Chords 723 24.7 Notes for Circular Curve Layout by Deflection Angles and Incremental Chords 725 24.8 Detailed Procedures for Circular Curve Layout by Deflection Angles and Incremental Chords 726 24.9 Setups on Curve 727 24.10 Metric Circular Curves by Deflection Angles and Incremental Chords 728 24.11 Circular Curve Layout by Deflection Angles and Total Chords 730 24.12 Computation of Coordinates on a Circular Curve 731 24.13 Circular Curve Layout by Coordinates 733 24.14 Curve Stakeout Using GNSS Receivers and Robotic Total Stations 738 24.15 Circular Curve Layout by Offsets 739 24.16 Special Circular Curve Problems 742 24.17 Compound and Reverse Curves 743 24.18 Sight Distance on Horizontal Curves 743 24.19 Spirals 744 24.20 Computation of “As-Built” Circular Alignments 749 24.21 Sources of Error in Laying Out Circular Curves 752 24.22 Mistakes 752 Problems 753 Bibliography 755
25 • VERTICAL CURVES 757 25.1 Introduction 757 25.2 General Equation of a Vertical Parabolic Curve 758 25.3 Equation of an Equal Tangent Vertical Parabolic Curve 759 25.4 High or Low Point on a Vertical Curve 761 25.5 Vertical Curve Computations Using the Tangent Offset Equation 761 25.6 Equal Tangent Property of a Parabola 765 25.7 Curve Computations by Proportion 766 25.8 Staking a Vertical Parabolic Curve 766 25.9 Machine Control in Grading Operations 767 25.10 Computations for an Unequal Tangent Vertical Curve 767 25.11 Designing a Curve to Pass Through a Fixed Point 770 25.12 Sight Distance 771 25.13 Sources of Error in Laying Out Vertical Curves 773 25.14 Mistakes 774 Problems 774 Bibliography 776
26 • VOLUMES 777 26.1 Introduction 777 26.2 Methods of Volume Measurement 777 26.3 The Cross-Section Method 778 26.4 Types of Cross Sections 779 26.5 Average-End-Area Formula 780 26.6 Determining End Areas 781 26.7 Computing Slope Intercepts 784 26.8 Prismoidal Formula 786 26.9 Volume Computations 788 26.10 Unit-Area, or Borrow-Pit, Method 790 26.11 Contour-Area Method 791 26.12 Measuring Volumes of Water Discharge 793 26.13 Software 794 26.14 Sources of Error in Determining Volumes 795 26.15 Mistakes 795 Problems 795 Bibliography 798
27 • PHOTOGRAMMETRY 799 27.1 Introduction 799 27.2 Uses of Photogrammetry 800 27.3 Aerial Cameras 801 27.4 Types of Aerial Photographs 803 27.5 Vertical Aerial Photographs 804 27.6 Scale of a Vertical Photograph 806 27.7 Ground Coordinates from a Single Vertical Photograph 810 27.8 Relief Displacement on a Vertical Photograph 811 27.9 Flying Height of a Vertical Photograph 813 27.10 Stereoscopic Parallax 814 27.11 Stereoscopic Viewing 817 27.12 Stereoscopic Measurement of Parallax 819 27.13 Analytical Photogrammetry 820 27.14 Stereoscopic Plotting Instruments 821 27.15 Orthophotos 826 27.16 Ground Control for Photogrammetry 827 27.17 Flight Planning 828 27.18 Airborne Laser-Mapping Systems 830 27.19 Remote Sensing 831 27.20 Software 837 27.21 Sources of Error in Photogrammetry 838 27.22 Mistakes 838 Problems 839 Bibliography 842
28 • INTRODUCTION TO GEOGRAPHIC INFORMATION SYSTEMS 843 28.1 Introduction 843 28.2 Land Information Systems 846 28.3 GIS Data Sources and Classifications 846 28.4 Spatial Data 846 28.5 Nonspatial Data 852 28.6 Data Format Conversions 853 28.7 Creating GIS Databases 856 28.8 Metadata 862 28.9 GIS Analytical Functions 862 28.10 GIS Applications 867 28.11 Data Sources 867 Problems 869 Bibliography 871
APPENDIX A • DUMPY LEVELS, TRANSITS, AND THEODOLITES 873 APPENDIX B • EXAMPLE NOTEFORMS 888 APPENDIX C • ASTRONOMICAL OBSERVATIONS 895 APPENDIX D • USING THE WORKSHEETS FROM THE COMPANION WEBSITE 911 APPENDIX E • INTRODUCTION TO MATRICES 917 APPENDIX F • U.S. STATE PLANE COORDINATE SYSTEM DEFINING PARAMETERS 923 APPENDIX G • ANSWERS TO SELECTED PROBLEMS 927 INDEX 933
Relate keywords
elementary surveying an introduction to geomatics 13th edition solutions pdf
elementary surveying an introduction to geomatics 13th edition pdf download
elementary surveying an introduction to geomatics thirteenth edition
elementary surveying an introduction to geomatics 13th edition
elementary surveying an introduction to geomatics 13th edition answers
elementary surveying an introduction to geomatics 13th edition solutions
elementary surveying - an introduction to geomatics 13th ed
elementary surveying an introduction to geomatics 13th edition solutions manual
elementary surveying an introduction to geomatics 13th edition pdf solutions
0 notes
Video
Can There Not Be a Lovelier Sight Than a Snow Covered Joshua Tree National Park by Mark Stevens Via Flickr: In Joshua Tree National Park at a roadside pullout looking to the east and across a snowy landscape with Joshua Trees and other desert plant-life. I liked the layered look of this national park landscape with the snow covered foreground, leading up to the hillside of rock formations that was itself also getting snow covered.
#Azimuth 86#Central and Southern California Ranges#Creosote Bush#Day 2#Desert Landscape#Desert Mountain Landscape#Desert Plant Life#DxO PhotoLab 6 Edited#Joshua Tree#Joshua Tree National Park and California#Joshua Tree Ranges#Landscape#Landscape - Scenery#Little San Bernardino Mountains#Looking East#Mojave Desert#Nature#Nikon D850#No People#Outside#Pacific Ranges#Peninsular Southern California Ranges#Project365#Roadside Pulloff#Rock Formations#Sage brush#Scenics - Nature#SnapBridge#Snow#Snow Covered
2 notes
·
View notes
Text
Solution Manual for Elementary Surveying An Introduction to Geomatics 13th Edition by Ghilani
This is Full Solution Manual for Elementary Surveying: An Introduction to Geomatics, 13th Edition Charles D. Ghilani
Click link bellow to view sample:
https://getbooksolutions.com/wp-content/uploads/2017/01/Solution-Manual-for-Elementary-Surveying-An-Introduction-to-Geomatics-13th-Edition-Charles-D-Ghilani.pdf
Origin Book information:
Charles D. Ghilani
Hardcover: 984 pages
Publisher: Prentice Hall; 13 edition (January 8, 2011)
Language: English
ISBN-10: 0132554348
ISBN-13: 978-0132554343
what is solution manual elementary surveying an introduction to geomatics 13th edition where you can download solution manual elementary surveying an introduction to geomatics 13th edition? and how you can get solution manual elementary surveying an introduction to geomatics 13th edition in fastest way?
You will be guided to the product download page immediately once you complete the payment. If you have any questions, or would like a receive a sample chapter before your purchase, please contact us via email :
Full download link:
https://getbooksolutions.com/download/solution-manual-for-elementary-surveying-an-introduction-to-geomatics-13th-edition-charles-d-ghilani
Table of Contents
1 • INTRODUCTION 1 1.1 Definition of Surveying 1 1.2 Geomatics 3 1.3 History of Surveying 4 1.4 Geodetic and Plane Surveys 9 1.5 Importance of Surveying 10 1.6 Specialized Types of Surveys 11 1.7 Surveying Safety 13 1.8 Land and Geographic Information Systems 14 1.9 Federal Surveying and Mapping Agencies 15 1.10 The Surveying Profession 16 1.11 Professional Surveying Organizations 17 1.12 Surveying on the Internet 18 1.13 Future Challenges in Surveying 19 Problems 20 Bibliography 21
2 • UNITS, SIGNIFICANT FIGURES, AND FIELD NOTES 23 PART I UNITS AND SIGNIFICANT FIGURES 23 2.1 Introduction 23 2.2 Units of Measurement 23 2.3 International System of Units (SI) 25 2.4 Significant Figures 27 2.5 Rounding Off Numbers 29 PART II FIELD NOTES 30 2.6 Field Notes 30 2.7 General Requirements of Handwritten Field Notes 31 2.8 Types of Field Books 32 2.9 Kinds of Notes 33 2.10 Arrangements of Notes 33 2.11 Suggestions for Recording Notes 35 2.12 Introduction to Data Collectors 36 2.13 Transfer of Files from Data Collectors 39 2.14 Digital Data File Management 41 2.15 Advantages and Disadvantages of Data Collectors 42 Problems 43 Bibliography 44
3 • THEORY OF ERRORS IN OBSERVATIONS 45 3.1 Introduction 45 3.2 Direct and Indirect Observations 45 3.3 Errors in Measurements 46 3.4 Mistakes 46 3.5 Sources of Errors in Making Observations 47 3.6 Types of Errors 47 3.7 Precision and Accuracy 48 3.8 Eliminating Mistakes and Systematic Errors 49 3.9 Probability 49 3.10 Most Probable Value 50 3.11 Residuals 51 3.12 Occurrence of Random Errors 51 3.13 General Laws of Probability 55 3.14 Measures of Precision 55 3.15 Interpretation of Standard Deviation 58 3.16 The 50, 90, and 95 Percent Errors 58 3.17 Error Propagation 60 3.18 Applications 65 3.19 Conditional Adjustment of Observations 65 3.20 Weights of Observations 66 3.21 Least-Squares Adjustment 67 3.22 Using Software 68 Problems 69 Bibliography 71
4 • LEVELING–THEORY, METHODS, AND EQUIPMENT 73 PART I LEVELING–THEORY AND METHODS 73 4.1 Introduction 73 4.2 Definitions 73 4.3 North American Vertical Datum 75 4.4 Curvature and Refraction 76 4.5 Methods for Determining Differences in Elevation 78 PART II EQUIPMENT FOR DIFFERENTIAL LEVELING 85 4.6 Categories of Levels 85 4.7 Telescopes 86 4.8 Level Vials 87 4.9 Tilting Levels 89 4.10 Automatic Levels 90 4.11 Digital Levels 91 4.12 Tripods 93 4.13 Hand Level 93 4.14 Level Rods 94 4.15 Testing and Adjusting Levels 96 Problems 100 Bibliography 102
5 • LEVELING–FIELD PROCEDURES AND COMPUTATIONS 103 5.1 Introduction 103 5.2 Carrying and Setting Up a Level 103 5.3 Duties of a Rodperson 105 5.4 Differential Leveling 106 5.5 Precision 112 5.6 Adjustments of Simple Level Circuits 113 5.7 Reciprocal Leveling 114 5.8 Three-Wire Leveling 115 5.9 Profile Leveling 117 5.10 Grid, Cross-Section, or Borrow-Pit Leveling 121 5.11 Use of the Hand Level 122 5.12 Sources of Error in Leveling 122 5.13 Mistakes 124 5.14 Reducing Errors and Eliminating Mistakes 125 5.15 Using Software 125 Problems 127 Bibliography 129
6 • DISTANCE MEASUREMENT 131 PART I METHODS FOR MEASURING DISTANCES 131 6.1 Introduction 131 6.2 Summary of Methods for Making Linear Measurements 131 6.3 Pacing 132 6.4 Odometer Readings 132 6.5 Optical Rangefinders 133 6.6 Tacheometry 133 6.7 Subtense Bar 133 PART II DISTANCE MEASUREMENTS BY TAPING 133 6.8 Introduction to Taping 133 6.9 Taping Equipment and Accessories 134 6.10 Care of Taping Equipment 135 6.11 Taping on Level Ground 136 6.12 Horizontal Measurements on Sloping Ground 138 6.13 Slope Measurements 140 6.14 Sources of Error in Taping 141 6.15 Tape Problems 145 6.16 Combined Corrections in a Taping Problem 147 PART III ELECTRONIC DISTANCE MEASUREMENT 148 6.17 Introduction 148 6.18 Propagation of Electromagnetic Energy 149 6.19 Principles of Electronic Distance Measurement 152 6.20 Electro-Optical Instruments 153 6.21 Total Station Instruments 156 6.22 EDM Instruments Without Reflectors 157 6.23 Computing Horizontal Lengths from Slope Distances 158 6.24 Errors in Electronic Distance Measurement 160 6.25 Using Software 165 Problems 165 Bibliography 168
7 • ANGLES, AZIMUTHS, AND BEARINGS 169 7.1 Introduction 169 7.2 Units of Angle Measurement 169 7.3 Kinds of Horizontal Angles 170 7.4 Direction of a Line 171 7.5 Azimuths 172 7.6 Bearings 173 7.7 Comparison of Azimuths and Bearings 174 7.8 Computing Azimuths 175 7.9 Computing Bearings 177 7.10 The Compass and the Earth’s Magnetic Field 179 7.11 Magnetic Declination 180 7.12 Variations in Magnetic Declination 181 7.13 Software for Determining Magnetic Declination 183 7.14 Local Attraction 184 7.15 Typical Magnetic Declination Problems 185 7.16 Mistakes 187 Problems 187 Bibliography 189
8 • TOTAL STATION INSTRUMENTS; ANGLE OBSERVATIONS 191 PART I TOTAL STATION INSTRUMENTS 191 8.1 Introduction 191 8.2 Characteristics of Total Station Instruments 191 8.3 Functions Performed by Total Station Instruments 194 8.4 Parts of a Total Station Instrument 195 8.5 Handling and Setting Up a Total Station Instrument 199 8.6 Servo-Driven and Remotely Operated Total Station Instruments 201 PART II ANGLE OBSERVATIONS 203 8.7 Relationship of Angles and Distances 203 8.8 Observing Horizontal Angles with Total Station Instruments 204 8.9 Observing Horizontal Angles by the Direction Method 206 8.10 Closing the Horizon 207 8.11 Observing Deflection Angles 209 8.12 Observing Azimuths 211 8.13 Observing Vertical Angles 211 8.14 Sights and Marks 213 8.15 Prolonging a Straight Line 214 8.16 Balancing-In 216 8.17 Random Traverse 217 8.18 Total Stations for Determining Elevation Differences 218 8.19 Adjustment of Total Station Instruments and Their Accessories 219 8.20 Sources of Error in Total Station Work 222 8.21 Propagation of Random Errors in Angle Observations 228 8.22 Mistakes 228 Problems 229 Bibliography 230
9 • TRAVERSING 231 9.1 Introduction 231 9.2 Observation of Traverse Angles or Directions 233 9.3 Observation of Traverse Lengths 234 9.4 Selection of Traverse Stations 235 9.5 Referencing Traverse Stations 235 9.6 Traverse Field Notes 237 9.7 Angle Misclosure 238 9.8 Traversing with Total Station Instruments 239 9.9 Radial Traversing 240 9.10 Sources of Error in Traversing 241 9.11 Mistakes in Traversing 242 Problems 242
10 • TRAVERSE COMPUTATIONS 245 10.1 Introduction 245 10.2 Balancing Angles 246 10.3 Computation of Preliminary Azimuths or Bearings 248 10.4 Departures and Latitudes 249 10.5 Departure and Latitude Closure Conditions 251 10.6 Traverse Linear Misclosure and Relative Precision 251 10.7 Traverse Adjustment 252 10.8 Rectangular Coordinates 255 10.9 Alternative Methods for Making Traverse Computations 256 10.10 Inversing 260 10.11 Computing Final Adjusted Traverse Lengths and Directions 261 10.12 Coordinate Computations in Boundary Surveys 263 10.13 Use of Open Traverses 265 10.14 State Plane Coordinate Systems 268 10.15 Traverse Computations Using Computers 269 10.16 Locating Blunders in Traverse Observations 269 10.17 Mistakes in Traverse Computations 272 Problems 272 Bibliography 275
11 • COORDINATE GEOMETRY IN SURVEYING CALCULATIONS 277 11.1 Introduction 277 11.2 Coordinate Forms of Equations for Lines and Circles 278 11.3 Perpendicular Distance from a Point to a Line 280 11.4 Intersection of Two Lines, Both Having Known Directions 282 11.5 Intersection of a Line with a Circle 284 11.6 Intersection of Two Circles 287 11.7 Three-Point Resection 289 11.8 Two-Dimensional Conformal Coordinate Transformation 292 11.9 Inaccessible Point Problem 297 11.10 Three-Dimensional Two-Point Resection 299 11.11 Software 302 Problems 303 Bibliography 307
12 • AREA 309 12.1 Introduction 309 12.2 Methods of Measuring Area 309 12.3 Area by Division Into Simple Figures 310 12.4 Area by Offsets from Straight Lines 311 12.5 Area by Coordinates 313 12.6 Area by Double-Meridian Distance Method 317 12.7 Area of Parcels with Circular Boundaries 320 12.8 Partitioning of Lands 321 12.9 Area by Measurements from Maps 325 12.10 Software 327 12.11 Sources of Error in Determining Areas 328 12.12 Mistakes in Determining Areas 328 Problems 328 Bibliography 330
13 • GLOBAL NAVIGATION SATELLITE SYSTEMS—INTRODUCTION AND PRINCIPLES OF OPERATION 331 13.1 Introduction 331 13.2 Overview of GPS 332 13.3 The GPS Signal 335 13.4 Reference Coordinate Systems 337 13.5 Fundamentals of Satellite Positioning 345 13.6 Errors in Observations 348 13.7 Differential Positioning 356 13.8 Kinematic Methods 358 13.9 Relative Positioning 359 13.10 Other Satellite Navigation Systems 362 13.11 The Future 364 Problems 365 Bibliography 366
14 • GLOBAL NAVIGATION SATELLITE SYSTEMS—STATIC SURVEYS 367 14.1 Introduction 367 14.2 Field Procedures in Satellite Surveys 369 14.3 Planning Satellite Surveys 372 14.4 Performing Static Surveys 384 14.5 Data Processing and Analysis 386 14.6 Sources of Errors in Satellite Surveys 393 14.7 Mistakes in Satellite Surveys 395 Problems 395 Bibliography 397
15 • GLOBAL NAVIGATION SATELLITE SYSTEMS—KINEMATIC SURVEYS 399 15.1 Introduction 399 15.2 Planning of Kinematic Surveys 400 15.3 Initialization 402 15.4 Equipment Used in Kinematic Surveys 403 15.5 Methods Used in Kinematic Surveys 405 15.6 Performing Post-Processed Kinematic Surveys 408 15.7 Communication in Real-Time Kinematic Surveys 411 15.8 Real-Time Networks 412 15.9 Performing Real-Time Kinematic Surveys 413 15.10 Machine Control 414 15.11 Errors in Kinematic Surveys 418 15.12 Mistakes in Kinematic Surveys 418 Problems 418 Bibliography 419
16 • ADJUSTMENTS BY LEAST SQUARES 421 16.1 Introduction 421 16.2 Fundamental Condition of Least Squares 423 16.3 Least-Squares Adjustment by the Observation Equation Method 424 16.4 Matrix Methods in Least-Squares Adjustment 428 16.5 Matrix Equations for Precisions of Adjusted Quantities 430 16.6 Least-Squares Adjustment of Leveling Circuits 432 16.7 Propagation of Errors 436 16.8 Least-Squares Adjustment of GNSS Baseline Vectors 437 16.9 Least-Squares Adjustment of Conventional Horizontal Plane Surveys 443 16.10 The Error Ellipse 452 16.11 Adjustment Procedures 457 16.12 Other Measures of Precision for Horizontal Stations 458 16.13 Software 460 16.14 Conclusions 460 Problems 461 Bibliography 466
17 • MAPPING SURVEYS 467 17.1 Introduction 467 17.2 Basic Methods for Performing Mapping Surveys 468 17.3 Map Scale 468 17.4 Control for Mapping Surveys 470 17.5 Contours 471 17.6 Characteristics of Contours 474 17.7 Direct and Indirect Methods of Locating Contours 474 17.8 Digital Elevation Models and Automated Contouring Systems 477 17.9 Basic Field Methods for Locating Topographic Details 479 17.10 Three-Dimensional Conformal Coordinate Transformation 488 17.11 Selection of Field Method 489 17.12 Working with Data Collectors and Field-to-Finish Software 490 17.13 Hydrographic Surveys 493 17.14 Sources of Error in Mapping Surveys 497 17.15 Mistakes in Mapping Surveys 498 Problems 498 Bibliography 500
18 • MAPPING 503 18.1 Introduction 503 18.2 Availability of Maps and Related Information 504 18.3 National Mapping Program 505 18.4 Accuracy Standards for Mapping 505 18.5 Manual and Computer-Aided Drafting Procedures 507 18.6 Map Design 508 18.7 Map Layout 510 18.8 Basic Map Plotting Procedures 512 18.9 Contour Interval 514 18.10 Plotting Contours 514 18.11 Lettering 515 18.12 Cartographic Map Elements 516 18.13 Drafting Materials 519 18.14 Automated Mapping and Computer-Aided Drafting Systems 519 18.15 Impacts of Modern Land and Geographic Information Systems on Mapping 525 18.16 Sources of Error in Mapping 526 18.17 Mistakes in Mapping 526 Problems 526 Bibliography 528
19 • CONTROL SURVEYS AND GEODETIC REDUCTIONS 529 19.1 Introduction 529 19.2 The Ellipsoid and Geoid 530 19.3 The Conventional Terrestrial Pole 532 19.4 Geodetic Position and Ellipsoidal Radii of Curvature 534 19.5 Geoid Undulation and Deflection of the Vertical 536 19.6 U.S. Reference Frames 538 19.7 Accuracy Standards and Specifications for Control Surveys 547 19.8 The National Spatial Reference System 550 19.9 Hierarchy of the National Horizontal Control Network 550 19.10 Hierarchy of the National Vertical Control Network 551 19.11 Control Point Descriptions 551 19.12 Field Procedures for Traditional Horizontal Control Surveys 554 19.13 Field Procedures for Vertical Control Surveys 559 19.14 Reduction of Field Observations to Their Geodetic Values 564 19.15 Geodetic Position Computations 577 19.16 The Local Geodetic Coordinate System 580 19.17 Three-Dimensional Coordinate Computations 581 19.18 Software 584 Problems 584 Bibliography 587
20 • STATE PLANE COORDINATES AND OTHER MAP PROJECTIONS 589 20.1 Introduction 589 20.2 Projections Used in State Plane Coordinate Systems 590 20.3 Lambert Conformal Conic Projection 593 20.4 Transverse Mercator Projection 594 20.5 State Plane Coordinates in NAD27 and NAD83 595 20.6 Computing SPCS83 Coordinates in the Lambert Conformal Conic System 596 20.7 Computing SPCS83 Coordinates in the Transverse Mercator System 601 20.8 Reduction of Distances and Angles to State Plane Coordinate Grids 608 20.9 Computing State Plane Coordinates of Traverse Stations 617 20.10 Surveys Extending from One Zone to Another 620 20.11 Conversions Between SPCS27 and SPCS83 621 20.12 The Universal Transverse Mercator Projection 622 20.13 Other Map Projections 623 20.14 Map Projection Software 627 Problems 628 Bibliography 631
21 • BOUNDARY SURVEYS 633 21.1 Introduction 633 21.2 Categories of Land Surveys 634 21.3 Historical Perspectives 635 21.4 Property Description by Metes and Bounds 636 21.5 Property Description by Block-and-Lot System 639 21.6 Property Description by Coordinates 641 21.7 Retracement Surveys 641 21.8 Subdivision Surveys 644 21.9 Partitioning Land 646 21.10 Registration of Title 647 21.11 Adverse Possession and Easements 648 21.12 Condominium Surveys 648 21.13 Geographic and Land Information Systems 655 21.14 Sources of Error in Boundary Surveys 655 21.15 Mistakes 655 Problems 656 Bibliography 658
22 • SURVEYS OF THE PUBLIC LANDS 659 22.1 Introduction 659 22.2 Instructions for Surveys of the Public Lands 660 22.3 Initial Point 663 22.4 Principal Meridian 664 22.5 Baseline 665 22.6 Standard Parallels (Correction Lines) 666 22.7 Guide Meridians 666 22.8 Township Exteriors, Meridional (Range) Lines, and Latitudinal (Township) Lines 667 22.9 Designation of Townships 668 22.10 Subdivision of a Quadrangle into Townships 668 22.11 Subdivision of a Township into Sections 670 22.12 Subdivision of Sections 671 22.13 Fractional Sections 672 22.14 Notes 672 22.15 Outline of Subdivision Steps 672 22.16 Marking Corners 674 22.17 Witness Corners 674 22.18 Meander Corners 675 22.19 Lost and Obliterated Corners 675 22.20 Accuracy of Public Lands Surveys 678 22.21 Descriptions by Township Section and Smaller Subdivision 678 22.22 BLM Land Information System 679 22.23 Sources of Error 680 22.24 Mistakes 680 Problems 681 Bibliography 683
23 • CONSTRUCTION SURVEYS 685 23.1 Introduction 685 23.2 Specialized Equipment for Construction Surveys 686 23.3 Horizontal and Vertical Control 689 23.4 Staking Out a Pipeline 691 23.5 Staking Pipeline Grades 692 23.6 Staking Out a Building 694 23.7 Staking Out Highways 698 23.8 Other Construction Surveys 703 23.9 Construction Surveys Using Total Station Instruments 704 23.10 Construction Surveys Using GNSS Equipment 706 23.11 Machine Guidance and Control 709 23.12 As-Built Surveys with Laser Scanning 710 23.13 Sources of Error in Construction Surveys 711 23.14 Mistakes 712 Problems 712 Bibliography 714
24 • HORIZONTAL CURVES 715 24.1 Introduction 715 24.2 Degree of Circular Curve 716 24.3 Definitions and Derivation of Circular Curve Formulas 718 24.4 Circular Curve Stationing 720 24.5 General Procedure of Circular Curve Layout by Deflection Angles 721 24.6 Computing Deflection Angles and Chords 723 24.7 Notes for Circular Curve Layout by Deflection Angles and Incremental Chords 725 24.8 Detailed Procedures for Circular Curve Layout by Deflection Angles and Incremental Chords 726 24.9 Setups on Curve 727 24.10 Metric Circular Curves by Deflection Angles and Incremental Chords 728 24.11 Circular Curve Layout by Deflection Angles and Total Chords 730 24.12 Computation of Coordinates on a Circular Curve 731 24.13 Circular Curve Layout by Coordinates 733 24.14 Curve Stakeout Using GNSS Receivers and Robotic Total Stations 738 24.15 Circular Curve Layout by Offsets 739 24.16 Special Circular Curve Problems 742 24.17 Compound and Reverse Curves 743 24.18 Sight Distance on Horizontal Curves 743 24.19 Spirals 744 24.20 Computation of “As-Built” Circular Alignments 749 24.21 Sources of Error in Laying Out Circular Curves 752 24.22 Mistakes 752 Problems 753 Bibliography 755
25 • VERTICAL CURVES 757 25.1 Introduction 757 25.2 General Equation of a Vertical Parabolic Curve 758 25.3 Equation of an Equal Tangent Vertical Parabolic Curve 759 25.4 High or Low Point on a Vertical Curve 761 25.5 Vertical Curve Computations Using the Tangent Offset Equation 761 25.6 Equal Tangent Property of a Parabola 765 25.7 Curve Computations by Proportion 766 25.8 Staking a Vertical Parabolic Curve 766 25.9 Machine Control in Grading Operations 767 25.10 Computations for an Unequal Tangent Vertical Curve 767 25.11 Designing a Curve to Pass Through a Fixed Point 770 25.12 Sight Distance 771 25.13 Sources of Error in Laying Out Vertical Curves 773 25.14 Mistakes 774 Problems 774 Bibliography 776
26 • VOLUMES 777 26.1 Introduction 777 26.2 Methods of Volume Measurement 777 26.3 The Cross-Section Method 778 26.4 Types of Cross Sections 779 26.5 Average-End-Area Formula 780 26.6 Determining End Areas 781 26.7 Computing Slope Intercepts 784 26.8 Prismoidal Formula 786 26.9 Volume Computations 788 26.10 Unit-Area, or Borrow-Pit, Method 790 26.11 Contour-Area Method 791 26.12 Measuring Volumes of Water Discharge 793 26.13 Software 794 26.14 Sources of Error in Determining Volumes 795 26.15 Mistakes 795 Problems 795 Bibliography 798
27 • PHOTOGRAMMETRY 799 27.1 Introduction 799 27.2 Uses of Photogrammetry 800 27.3 Aerial Cameras 801 27.4 Types of Aerial Photographs 803 27.5 Vertical Aerial Photographs 804 27.6 Scale of a Vertical Photograph 806 27.7 Ground Coordinates from a Single Vertical Photograph 810 27.8 Relief Displacement on a Vertical Photograph 811 27.9 Flying Height of a Vertical Photograph 813 27.10 Stereoscopic Parallax 814 27.11 Stereoscopic Viewing 817 27.12 Stereoscopic Measurement of Parallax 819 27.13 Analytical Photogrammetry 820 27.14 Stereoscopic Plotting Instruments 821 27.15 Orthophotos 826 27.16 Ground Control for Photogrammetry 827 27.17 Flight Planning 828 27.18 Airborne Laser-Mapping Systems 830 27.19 Remote Sensing 831 27.20 Software 837 27.21 Sources of Error in Photogrammetry 838 27.22 Mistakes 838 Problems 839 Bibliography 842
28 • INTRODUCTION TO GEOGRAPHIC INFORMATION SYSTEMS 843 28.1 Introduction 843 28.2 Land Information Systems 846 28.3 GIS Data Sources and Classifications 846 28.4 Spatial Data 846 28.5 Nonspatial Data 852 28.6 Data Format Conversions 853 28.7 Creating GIS Databases 856 28.8 Metadata 862 28.9 GIS Analytical Functions 862 28.10 GIS Applications 867 28.11 Data Sources 867 Problems 869 Bibliography 871
APPENDIX A • DUMPY LEVELS, TRANSITS, AND THEODOLITES 873 APPENDIX B • EXAMPLE NOTEFORMS 888 APPENDIX C • ASTRONOMICAL OBSERVATIONS 895 APPENDIX D • USING THE WORKSHEETS FROM THE COMPANION WEBSITE 911 APPENDIX E • INTRODUCTION TO MATRICES 917 APPENDIX F • U.S. STATE PLANE COORDINATE SYSTEM DEFINING PARAMETERS 923 APPENDIX G • ANSWERS TO SELECTED PROBLEMS 927 INDEX 933
0 notes
Text
0 notes
Text
HMAS Adelaide (L01) : Bandera de Australia Historial Astillero: Navantia en Ferrol BAE Systems Australia en Williamstown Clase Canberra Tipo LHD Iniciado 18 de febrero de 2011 Botado:4 de julio de 20121 Asignado:4 de diciembre de 20152.
Características generales
Desplazamiento: 27 079 t Eslora: 230,82 m Manga: 32,0 m Puntal: 27,0 m Calado: 6,9 m Propulsión: CODLAG.3 (Combinad Diesel-eléctrica and Gas) 2 motores diesel Navantia-MAN de 7,86 Mw: 1 turbina de gas General Electric de 19,75 MW2 pods acimutales Navantia-Siemens de 11 MW Potencia: 30 000 cv3 Velocidad: 21,5 nudos Autonomía: 9000 nmi a 15 nudos Tripulación: 243 personas Tropas: 1200 personas Capacidad: Vehículos ligeros (nivel superior): 2046 m² Vehículos pesados (nivel inferior): 1400 m²975 m² adicionales si se emplea el espacio del dique inundable) Aeronaves: Entre 16 y 24 helicópteros 4
Equipamiento aeronaves 2 ascensores Ski-jump 12º: 1000 m² hangar configuración anfibia 3046 m² (1000 + 2046 m²) configuración aérea Coste: 400 millones de €/unidad.
———————————————————————————————————————————–
HMAS Adelaide (L01) Flags Flag of Australia History Navantia Shipyard in Ferrol BAE Systems Australia in Williamstown Class Canberra Type LHD Started February 18, 2011 Posted: July 4, 20121 Assigned: December 4, 20152.
General characteristics
Navantia-MAN Diesel engines of 7 years, with a total capacity of 7,700 t. , 86 Mw: 1 General Electric gas turbine of 19.75 MW2 Navantia-Siemens azimuthal pods of 11 MW Power: 30 000 cv3 Speed: 21.5 knots Autonomy: 9000 nmi at 15 knots Crew: 243 people Troops: 1200 people Capacity : Light vehicles (upper level): 2046 m² Heavy vehicles (lower level): 1400 m²975 additional m² when using flooded dock space) Aircraft: Between 16 and 24 helicopters 4 Aircraft equipment 2 lifts Ski-jump 12th: 1000 m² hangar Amphibian configuration 3046 m² (1000 + 2046 m²) air configuration Cost: 400 million € / unit.
HMAS Adelaide (L01) : Bandera de Australia Historial Astillero: Navantia en Ferrol Spain HMAS Adelaide (L01) : Bandera de Australia Historial Astillero: Navantia en Ferrol BAE Systems Australia en Williamstown Clase Canberra Tipo LHD Iniciado 18 de febrero de 2011 Botado:4 de julio de 20121 Asignado:4 de diciembre de 20152.
0 notes
Video
Cascading Waters of Otter Lake Waterfalls (Blue Ridge Parkway) by Mark Stevens Via Flickr: At the Otter Lake Waterfalls with a view looking to the east to the namesake waterfall. This is at a roadside pullout and recreation area along the Blue Ridge Parkway. From this creek edge viewpoint, I worked to align myself to center the waterfalls in the image, while having trees along the edges fill the frame for this national park service site location. I later worked with control points in DxO PhotoLab 7 and then made some adjustments to bring out the contrast, saturation and brightness I wanted for the final image.
#Appalachian Mountains#Azimuth 86#Blue Ridge Mountains#Blue Ridge Parkway#Blue Skies#Blues Skies with Clouds#Day 4#DxO PhotoLab 7 Edited#Forest#Forest Landscape#Landscape#Landscape - Scenery#Looking East#Nature#Nikon Z8#No People#Northern Blue Ridge#Otter Creek#Otter Lake Waterfalls#Outside#Partly Sunny#Portfolio#Project365#Scenics - Nature#Shenandoah Blue Ridge Great Smoky Mountains#SnapBridge#South Central Virginia Blue Ridge#Sunny#Travel#Trees
1 note
·
View note
Video
Can There Not Be a Lovelier Sight Than a Snow Covered Joshua Tree National Park by Mark Stevens Via Flickr: In Joshua Tree National Park at a roadside pullout looking to the east and across a snowy landscape with Joshua Trees and other desert plant-life. I liked the layered look of this national park landscape with the snow covered foreground, leading up to the hillside of rock formations that was itself also getting snow covered.
#Azimuth 86#Central and Southern California Ranges#Creosote Bush#Day 2#Desert Landscape#Desert Mountain Landscape#Desert Plant Life#DxO PhotoLab 6 Edited#Joshua Tree#Joshua Tree National Park and California#Joshua Tree Ranges#Landscape#Landscape - Scenery#Little San Bernardino Mountains#Looking East#Mojave Desert#Nature#Nikon D850#No People#Outside#Pacific Ranges#Peninsular Southern California Ranges#Project365#Roadside Pulloff#Rock Formations#Sage brush#Scenics - Nature#SnapBridge#Snow#Snow Covered
1 note
·
View note