#which scatters shorter wavelengths of light (such as blue and green) and allows longer wavelengths (such as red and orange) to dominate the
Explore tagged Tumblr posts
Text
"Crimson Horizons: Behold the Enchanting Red Sunset in Sweden's Breathtaking Landscapes"
"Captivating Beauty: Witness the Majestic Red Sunset in Sweden and Immerse Yourself in Nature's Radiant Splendor"

#Red Sunset Sweden#User#Sunset in Sweden red#casting hues of red#orange#pink#and purple. The phenomenon of a red sunset is caused by the scattering of light in the Earth's atmosphere. When the sun is low on the horiz#its light has to pass through a thicker portion of the atmosphere#which scatters shorter wavelengths of light (such as blue and green) and allows longer wavelengths (such as red and orange) to dominate the#Sweden#with its vast landscapes and scenic views#the fiery colors of a red sunset can create a truly memorable experience. The exact timing and intensity of a red sunset can vary depending#the time of year#âCaptivating Beauty: Witness the Majestic Red Sunset in Sweden and Immerse Yourself in Nature's Radiant Splendorâ#Title#ScarletSkies#20 hashtag#RedSunset#SwedenSunset#VibrantSkies
3 notes
¡
View notes
Text
Hereâs something from NASA since I have no interest in Instagram, https://eclipse2017.nasa.gov/what-color-sun
As with all matter, the sun emits a âblack body spectrumâ that is defined by its surface temperature. A black body spectrum is the continuum of radiation at many different wavelengths that is emitted by any body with a temperature above absolute zero. For our sun, this black body curve or âPlank Functionâ is a smooth almost bell shaped curve involving electromagnetic (EM) radiation at many different wavelengths from very long infrared to very short ultraviolet wavelengths. During very hot, explosive, high energy solar flare events, the sun emits huge amounts of x-ray and gamma ray radiation as well, up to over 100 MeV energies and up to 1032 ergs of energy over only a few seconds or tens of seconds! These massive solar flares are huge explosions in the sunâs atmosphere caused by the sudden release of magnetic field energy and tend to occur near solar maximum. The flares also accelerate charged particle plasmas to high speeds resulting in radio emission. So, the sun actually emits energy at all wavelengths from radio to gamma ray. But, as can be seen in the image above, it emits most of its energy around 500 nm, which is close to blue-green light. So one might say that the sun is blue-green! This maximum radiation frequency is governed by the sunâs surface temperature, around 5,800K. A higher surface temperature would result in a shorter maximum wavelength and our sun might peak in the blue or violet part of the spectrum (or even the ultra violet!). A lower surface temperature, and our sunâs spectrum might peak in the yellow or orange or even red part of the spectrum. But this is physics, not perception. I must note here another spectral signature from the sun, the photon flux. If we use the relationship found by Max Planck, E = hf (that is Energy = the Planck Constant times the Frequency) and convert the solar irradiance into photon counts, the spectral signature across visible wavelengths is much flatter and the sun is perceived as more yellow.
If we were above the atmosphere, say on the International Space Station and looked at the sun (through our filtered visor), the sun would appear white! Why? Because though the sun emits strongest in the green part of the spectrum, it also emits strongly in all the visible colors â red through blue (400nm to 600nm). Our eyes which have three color cone cell receptors, report to the brain that each color receptor is completely saturated with significant colors being received at all visible wavelengths. Our brains then integrate these signals into a perceived white color.
Here on Earth, the atmosphere plays a role in the color of the sun. Since shorter wavelength blue light is scattered more efficiently than longer wavelength red light, we lose some of the blue tint of the sun as sunlight passes through the atmosphere. In addition, all wavelengths of visible light passing through our atmosphere are attenuated so that the light that reaches our eyes does not immediately saturate the cone receptors. This allows the brain to perceive color from the image with a little less blue â yellow. Though it does not affect what our eyes see, all x-ray and gamma ray radiation is filtered out before it comes close to the ground. Most UV is absorbed by stratospheric ozone (above 10km) and most IR is absorbed by water vapor and other molecules with non - zero dipole moments.
Of course, when sunlight passes through a lot of atmosphere as is the case with sunrises and sunsets, even more blue light is scattered and a much greater percentage of the longest wavelength (red) light makes it to our eyes.
So, you see, there is no simple answer to this question, but the good news is that you can defend almost any answer!

https://www.instagram.com/reel/Cs9Z8vbsFky/?igshid=ZjUwM2YwMzA3MA==
3 notes
¡
View notes
Text
TAFAKKUR: Part 71
Blue Light, Daytime Sleep and the Prophetic Tradition
Sleep is a blessing from God. Humans spend around one third of their lives sleeping. Sleep is essential for the performance of important daily acts such as speech, memory, and original or flexible thinking. At the end of a busy day our body looks forward to relaxing and restoring its energy for the next day. Although sleep plays a significant role in our daily life, most of us rarely find and use suitable periods for a good sleep. There is no fixed amount of time that everyone needs to sleep, since this varies from person to person depending on several different factors, such as genetic background, age, and daily activities. For an adult individual the average time spent sleeping can vary from six to eight hours.1 We need to make some time for rest and recovery.
In reality, people who habitually go to sleep very late at night have difficulty waking up in the morning. This happens because of disturbances in the bodyâs internal clock or circadian rhythms. Circadian rhythms are important in determining the sleeping and feeding patterns of all creatures.
There are clear patterns of brainwave activity, hormone production, adjustments to body temperature, blood pressure and many other biological activities linked to this daily cycle.
It is known that melatonin, a hormone with a high level of concentration at night and low levels during daytime, regulates the circadian rhythm. However, there are other factors that activate or inhibit the internal body clock and several studies have demonstrated that light on the eyeâs retina is a more powerful influence on human circadian rhythms.
Blue light
As light that is radiated from the sun travels through the atmosphere, some shorter wavelengths of light, such as violet and blue, are absorbed by gas molecules and then scattered all around the sky. This gives the sky a blue appearance. According to Dr. Mark Rea, director of the Lighting Research Center, our daily rhythms are in fact blue sky detectors, and he notes, âBlue sky is ideal for stimulating the circadian system because itâs the right color and intensity, and itâs âonâ at the correct time for the right duration, the entire day.â
Researchers have used light for jet-setters, sleep-troubled patients, and shift workers to set their body clocks and solve sleep problems. Moreover, they claim the dogma that the 24-hour biological clock is set by light alone is a misconception that many scientists have believed for years. Steven Lockley of Brigham and Women's Hospital, a Harvard research and teaching affiliate says, âThe visual system in humans is most sensitive to green light, but when we exposed twelve healthy young men and women to the same amount of either green or blue light, their 24-hour rhythms shifted twice as much with blue than with green.â After exposure to blue light, their body clocks were rearranged by three hours, whereas the effect of green light was a change of about 1.5 hours. So, if your eyelids get heavy around 11 p.m., 6.5 hours of exposure to blue light will keep you up until 2 a.m. Since television and computer screens produce blue light, watching them too much at night may inappropriately affect the sleep cycle. However, if a change in shift makes you sleepless at 4 a.m., proper blue light therapy might help you sleep three hours longer.
To reduce the impact of synthetic light on our biological system, lighting manufacturers have been developing dynamic lighting control led by computer based systems. For example, to perk people up in the morning, the lamp increases the blue light concentration. After lunch, it again produces more blue light to counter afternoon sleepiness. In Europe, such systems have already been installed in some places including some hospitals, business offices, town halls and traffic-control centers.
Keeping all this in mind, we see how blue light affects our daily life. So, sleeping in some parts of the daytime can have advantages and disadvantages for sustaining optimal performance.
Daytime sleep in Prophetic tradition
If we turn our attention to the life of the Prophet Muhammad, (peace and blessings be upon him) we see how he successfully set an example for his Companions. It was the practice of the Prophet and his Companions not to sleep after the dawn prayer, the first of the five daily prayers, as it is a time of blessing. He would read some parts from the Qurâan and reflect on the universe and its Creator at a time when concentration is at its peak and the mind is clear of daily worries. Once dawn broke, the Prophet would pray and then speak with people who stayed behind for some time. Therefore, some of the early Muslims disliked the idea of sleeping immediately after dawn because being awake at this time was encouraged.
The first is âghaylula.â This is from pre-dawn to forty minutes or so after the sun has risen, the time when prayer is lawful but reprehensible. Sleep at this time is contrary to the practices of the Prophet, since according to hadiths, it leads to a decrease in oneâs livelihood and to its being unfruitful. The time most appropriate for preparing to labor for oneâs sustenance is when it is cool. When this time has passed, lethargy descends. It has been established through numerous experiences that just as this is detrimental to that dayâs labor and indirectly to oneâs livelihood, so also is it the cause of unfruitfulness.
Based on our limited knowledge about blue light, by sleeping at this time we may cause our biological rhythms to delay because of missing the right blue intensity at the correct time, which can result in a shift in sleep period and other shifts in daily cycles.
The second is âfaylula.â This is from the afternoon prayer till sunset. This sleep leads to a diminution of life, that is, it makes life that day shorter and makes it pass in a state of semi-sleep due to drowsiness, thus causing a physical deficiency to life. So too in an immaterial aspect, since most of the results of that day, material and immaterial, become apparent after the afternoon prayer, to pass that time in sleep as though prevents those results being seen and makes the day as though not lived.
The third is âqaylula,â which is in accordance with the Sunna of the Prophet. It is from mid-morning to just past noon. This sleep is part of the Sunna since it allows a person to rise at night to pray. So also in the Arabian Peninsula to rest from work at noon when it is intensely hot is the custom of the people and of the area, so has further strengthened this Practice of the Prophet. This sleep increases both life and sustenance. For half an hourâs qaylula sleep is the equivalent of two hoursâ sleep at night. That means it adds one and a half hours to a personâs life every day. It saves one and a half hours from the hand of sleep, the brother of death, and makes it live, adding it to the time of working for oneâs livelihood.
Recently, this type of sleep or nap has been strongly advised for health and for enhancing daily performance. âWhen I was at NASA we gave the pilots a planned nap in the cockpit,â says Rosekind, a board member of the National Sleep Foundation. âWhile two pilots flew the plane, the third would have forty minutes to nap. We found they would sleep for twenty-six minutes, which boosted their performance by 34% and their alertness by 54%.â9 He explains that the pilots were able to improve their performance significantly, and he adds that the effects of a short nap of less than a half hour lasted for two to three hours. Similar research shows that in this way you can make yourself more alert, reduce stress, and improve cognitive functioning, which means more patience, less stress, better reaction time, increased learning, and more efficiency.
Each day our daily life gets faster and we unintentionally ignore our body and its need for rest, which leads to sleep deprived eyes, disturbed sleep cycles, stress, and so on. Sleeping well keeps us alive longer. But this does not mean sleeping for a long time, which increases the risk of death. What we learn from the Prophetic tradition and research into the effects of light on our biological clocks can help us to find and to arrange proper times for sleeping and coordinating our daily activities. Once we achieve this, benefits in spiritual life, health, social interactions, learning and thinking clearly will follow.
#allah#god#muhammad#prophet#sunnah#hadith#quran#ayat#islam#muslim#muslimah#hijab#help#revert#convert#religion#reminder#dua#salah#pray#prayer#welcome to islam#how to convert islam#new muslim#new revert#new convert#revert help#convert help#islam help#muslim help
6 notes
¡
View notes
Link
Origin of Light
Solar energy results from nuclear fusion in the sunâs core, which creates an enormous, continuous flow of radiant energy that travels through- out the solar system. The energy that reaches us from all the stars twinkling in our galaxy can- not compare with what we receive from the sun, the only significant source of energy for Earthâs atmosphere.
This radiant energy released in the form of electromagnetic waves, which travel unimpeded from the sun in straight lines at the speed of light-186,000 miles a second. Although the intensity of the energy diminishes as it travels the 93 million miles from the sun to Earthâs
outer atmosphere, the amount of energy received by Earth from the sun in ten seconds still is equivalent to all the electricity generated on the planet in one week. Electromagnetic waves vary in length, which is measured as the distance between the crests of waves
Different types of Light
All the types of these waves together constitute the electromagnetic spectrum, which ranges from extremely short ultraviolet waves to very long radio waves. Visible light, which occupies a narrow band of that spectrum, comprises colors that vary in wavelength from short to long.
Shorter wavelengths scatter more effectively in the atmosphere, which is why the sky is blue-a color with a relatively short wavelength-on a sunny day. At dawn and dusk, light must pass through more atmosphere, allowing the longer orange and red light waves to predominate over the widely scattered blue waves.
The multicolored arc of a rainbow is produced by sunlight striking raindrops beneath a rain cloud. Light refracts-bends-when it passes through drops of water. Each color of light refracts at a different angle: Violet bends more than blue, which bends more than green, and so on, with red refracting the least. If sunlight enters a raindrop at just the proper angle, it refracts, and its many colors spread into a visible array.
Sunlight refracted through millions of raindrops forms a rainbow. On a primary rainbow, red is the outside color and violet the inside color. Occasionally a secondary rain- bow appears slightly higher in the sky, and in it the colors of the rainbow are reversed (opposite). A rainbowâs position in the sky depends on the sunâs altitude above the horizon-the lower the sun, the higher the rainbow appears.
For more read click here: Â https://shouts.site/light/
0 notes
Link
White Sunblock is a Thing of the Past
by Joel Pedersen
As a child, I spent many summer days at the beach in southern California. I remember playing in the surf, collecting shells, watching sea lions, and seeing the white noses of the lifeguards. In those days, lifeguards smeared thick, white zinc oxide paste on their noses to protect themselves from getting sunburned

My nose, smeared in Zinka Sunblock, which contains zinc oxide particles
When my kids were young there was a brief period when colored zinc oxide sunblock seemed to be in vogue
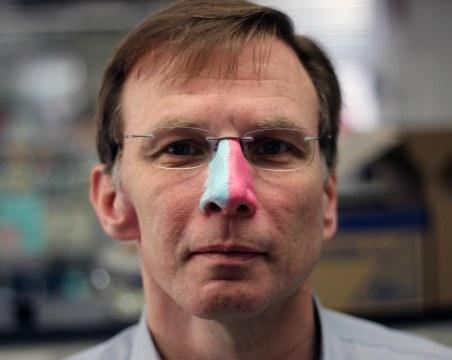
My nose, smeared in colored Zinka Sunblock
Nowadays, we still use zinc oxide in sunblocks, but itâs no longer white or colored; itâs transparent! How can this be? Itâs the same material!!
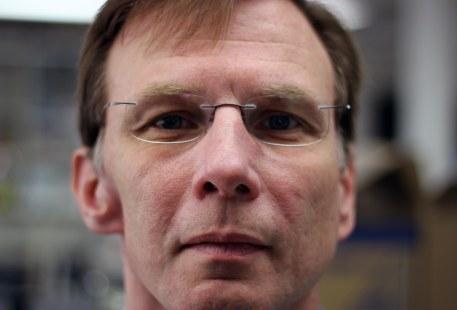
My nose, smeared in Blue Lizard sunblock, which contains zinc oxide nanoparticles
White pasty sunblocks protect your skin by absorbing and scattering away the harmful ultraviolet radiation. They also happen to scatter the less energetic form of radiation, visible light. They appear white in color because they scatter all colors of visible light roughly equally, so the white light that hits the sunblock is still white light when it hits our eyes. The colored sunblocks had pigments added that absorb some wavelengths of visible light.
Nano-enabled zinc oxide sunblock appears transparent because the smaller particles in it do not scatter light as effectively as their larger pasty counterparts. This might seem like a problem. If they donât scatter light as effectively, wouldnât that mean they would allow more harmful ultraviolet radiation through to your skin, unscattered? In fact, they do not! But Iâm getting ahead of myself. First, letâs discuss why nano-sized zinc oxide particles are transparent while the larger sized particles in the older sunblocks appear white.
Different particle sizes scatter light to varying degrees.(1) As particles become so small that their diameters are equivalent to roughly half the wavelength of light, the intensity of scattered light falls steeply. This means, if you can make particles smaller than about half the wavelength of visible light (390 to 700 nanometers), they will no longer be able to scatter that visible light efficiently! So, nanoparticles smaller than roughly 200 nanometers are practically transparent.
Now that weâve solved the problem of light scattering by zinc oxide particles in sunblock, letâs return to our earlier question. By making particles that donât scatter visible light, have we created another problem? Arenât we allowing harmful ultraviolet radiation to reach the skin on the lifeguardsâ noses since weâre not scattering it away. Fortunately not! Nano-scale zinc oxide remains effective in sunblock because it efficiently absorbs ultraviolet light, preventing it from interacting with the skin.
Light scattering also depends on wavelength. For particles much smaller than the wavelength of light, those particles scatter shorter wavelength light (for example UV light) much more efficiently than longer wavelength light (for example, visible light). More precisely, for particles in this size range, scattering is inversely proportional to the fourth power of the wavelength. This means that UV light at, say, 350 nanometers is scattered nearly 5Ă more efficiently than green light at 520 nanometers, all else being equal. (The wavelength-dependent scattering is the reason the sky is blue, but I digress.)
In conclusion, we have seen that for scattering light, size matters! Zinc oxide nanoparticles can be made in sizes smaller that approximately 200 nanometers, so we no longer have to smear unsightly white paste on our noses to take advantage of the sun-protective effect of zinc oxide.
 Shop Schmidt Clothing for all your summer clothing
 // <![CDATA[ (function () { var scriptURL = 'https://sdks.shopifycdn.com/buy-button/latest/buy-button-storefront.min.js'; if (window.ShopifyBuy) { if (window.ShopifyBuy.UI) { ShopifyBuyInit(); } else { loadScript(); } } else { loadScript(); } function loadScript() { var script = document.createElement('script'); script.async = true; script.src = scriptURL; (document.getElementsByTagName('head')[0] || document.getElementsByTagName('body')[0]).appendChild(script); script.onload = ShopifyBuyInit; } function ShopifyBuyInit() { var client = ShopifyBuy.buildClient({ domain: 'schmidt-clothing.myshopify.com', storefrontAccessToken: '04e3f2c2f256c599ae4e274d76cc6246', }); ShopifyBuy.UI.onReady(client).then(function (ui) { ui.createComponent('collection', { id: '188621521034', node: document.getElementById('collection-component-1593789762986'), moneyFormat: '%24%7B%7Bamount%7D%7D', options: { "product": { "styles": { "product": { "@media (min-width: 601px)": { "max-width": "calc(25% - 20px)", "margin-left": "20px", "margin-bottom": "50px", "width": "calc(25% - 20px)" }, "img": { "height": "calc(100% - 15px)", "position": "absolute", "left": "0", "right": "0", "top": "0" }, "imgWrapper": { "padding-top": "calc(75% + 15px)", "position": "relative", "height": "0" } }, "button": { ":hover": { "background-color": "#0363b3" }, "background-color": "#023a69", ":focus": { "background-color": "#0363b3" }, "border-radius": "14px", "padding-left": "46px", "padding-right": "46px" } }, "buttonDestination": "checkout", "text": { "button": "Buy now" } }, "productSet": { "styles": { "products": { "@media (min-width: 601px)": { "margin-left": "-20px" } } } }, "modalProduct": { "contents": { "img": false, "imgWithCarousel": true, "button": false, "buttonWithQuantity": true }, "styles": { "product": { "@media (min-width: 601px)": { "max-width": "100%", "margin-left": "0px", "margin-bottom": "0px" } }, "button": { ":hover": { "background-color": "#0363b3" }, "background-color": "#023a69", ":focus": { "background-color": "#0363b3" }, "border-radius": "14px", "padding-left": "46px", "padding-right": "46px" } }, "text": { "button": "Add to cart" } }, "cart": { "styles": { "button": { ":hover": { "background-color": "#0363b3" }, "background-color": "#023a69", ":focus": { "background-color": "#0363b3" }, "border-radius": "14px" } }, "text": { "total": "Subtotal", "button": "Checkout" } }, "toggle": { "styles": { "toggle": { "background-color": "#023a69", ":hover": { "background-color": "#0363b3" }, ":focus": { "background-color": "#0363b3" } } } } }, }); }); } })(); // ]]>
0 notes
Text
Arcane Realm Races: Manikins
Overview

Manikins are a species of diminutive humans from the planet Kaf. Their physiology is adapted to high altitudes like mountains with their native settlements at heights above 2,500 meters (8,200 ft) to 6,500 meters (21,300 ft).
They also take pride in their handiwork, with some of the worldâs most advanced and cutting edge technology being developed in their urban centers.
Biology
Quick Species Profile
(Homo sapiens mannchen)
Life Expectancy
Typical: 65 - 75 years Oldest: 145 years
Height:Â 109 - 135 cm (3'7" - 4'5")
Weight:Â 27 â 41 kg (70 â 90 lbs)
Creature Type: Natural Humanoid
Appearance
Of the natural humanoid races of Kaf, a Manikin looks a lot a modern human of Earth. There are two key differences, first is they are smaller, typically a foot or more shorter in height but the same proportions. The other key difference is that they are very frequently seen with a wide range of hair and eye colors which are naturally impossible in real humans, including purple, blue, green, and countless other colors.
One area in which Manikins do not diverge significantly from modern humans is skin color which ranges from pale to dark brown.
Physiological Traits
Low Oxygen Resistance: Manikins have the ability to maintain relatively low levels of hemoglobin, which is the chemical complex for transporting oxygen in the blood. Instead, they inhale more air with each breath and breathe more rapidly than sea-level populations throughout their lifetime which enables them to inhale larger amounts of air per unit of time to compensate for low oxygen levels. They also show a sustained increase in cerebral blood flow and less susceptibility to chronic mountain sickness than regular humans. They have ten times more nitric oxide and double the forearm blood flow of low-altitude dwellers. Nitric oxide causes dilation of blood vessels allowing blood to flow more freely to the extremities and aids the release of oxygen to tissues.
Heavy Birthweight: Manikin women are known to give birth to heavier-weight infants with higher blood oxygen levels than women of lowland humanoids.
Large Lung Capacity:Â Manikins have larger lung volumes proportional to other humanoids throughout life, and a high capacity for physical exercise.
Cold Resistance: Manikins hands and feet are have more small arteries and veins suppling blood than similar sized humanoids. This makes their extremities much less likely to freeze and suffer from frostbite species adapted to sea level temperatures.
Colorful Hair & Eyes: Manikins have a wide range of hair and eye colors which are naturally impossible in modern humans, including purple, blue, green, etc. Most of these colors arenât caused by pigments, but by the way light waves are scattered by the proteins in their hair or eyes. Because of the layered structure of the hair or iris, light waves entering it bounce back at different times and thus collide, or âinterfereâ with each other and certain wavelengths get cancelled out while others become reinforced, resulting in a rainbow of structural colors.
Sorcery
Passive Traits
Active Abilities
Manaplasm Conjuration: Being natural humanoids, when manikins channel mana from the environment into magic, the energy generates a substance known as manaplasm. Their magic involves manipulation of manaplasm to do various things such as act as a projectile, barrier, mimic other substances, or alter preexisting magical effects.
General Diet
Manikins are opportunistic omnivores, capable of consuming a wide variety of plant and animal material. They are partial to food that contains a high sugar, fat, or salt content.
The healthiest diet for a manikin is very balanced and varied, ranging from plant matter like grains, fruits, and vegetables, to animal meat and their products.
Manikins are one of the few races of Kaf that are able to consume dairy products into adulthood due to their history of raising animals for milk.
ReproductionÂ
Manikin females are fertile year round and have a menstrual cycle roughly every month. Their women will also go through menopause, typically after 50 years of age, in which they will no longer bare children but males remain fertile much longer, many well into their 70âs.
As with other mammals, manikin reproduction takes place as internal fertilization by sexual intercourse between an opposite sex couple to produce offspring. While developing in their motherâs womb, the child gives her a distinctive âbaby bumpâ accompanied by a significant weight gain. Being mammals, the females give birth to live young, either one or several at a time.The delivery itself normally takes place after nine months of pregnancy and is a painful process that can take as much as ten hours. In some circumstances, women could die during childbirth, although it became less common in places with access to top-notch medical technologies. During their first months of existence, manikin babies are usually fed with milk from their motherâs breasts.
Lifespan & Growth
Manikin infants tend to have higher birth weight and higher blood oxygen levels than most humanoid races. The manikins also follow the typical stages of the human life span like infancy, childhood, adolescence,  young adulthood, adulthood and old age. The main difference from earth humans being the lack of a growth spurt during puberty.
Manikins hit their peak of physical and mental capacity from the years of 25 to 35, after a decade or so from their bodies may deteriorate in quality without a specific diet or exercises. A manikin without advanced medical treatments can likely live roughly half a century, recent advances able to extend this to possibly a century and a half.
Regardless of physiological and psychological changes, the legal transition from childhood to adulthood depends on culture and can be anywhere from 16 to 25 years old.
Habitat
Mountainside, Highlands, High Alt Cities, Layered Cities
#arcane realm worldbuilding#fantasy#worldbuilding#fantasy race#manikins#natural humanoid#high altitude
1 note
¡
View note
Text
Recreating the Look of 1850s Tintypes in Digital with Math and Science
While I was visiting San Francisco, Kristy Headley, a dear friend and fellow engineer, showed me her studio. There I was lucky enough to sit for her while she did some vintage tintyping. Tintyping was one of the earliest forms of photography, popular in the 1850s.
It was a kind of incredibly inconvenient Polaroid â the photos were exposed instantly after a quick wash on plates of iron or aluminum (never actually tin). All you needed was a very, very large camera, plates treated with âcollodionâ to make a light-reactive surface, and a sizable collection of chemicals. Unfortunately, the genuine process has a high risk of explosion, creating cyanide gas, incurring blindness from silver halide splashback, or getting whacked out on ether.
âDustyâ. Tintype/ferrotype by Quinn Jacobson and licensed under CC BY-SA 3.0
Kristy showed me each painstaking step, and I was enamored with the result. With her mastery of lighting techniques, sheâd produced tintype photographs where every detail popped â every freckle, hair, and eyelash seemed to stand out. Yet, these looked very different from modern photography. There was a certain, seemingly unquantifiable trait to them. Why?
I sought out to quantify this olâ timey look and the fashion by which one could reproduce it with a digital camera. Perhaps I could share in this delight without keeping jugs of volatile chemicals in my room.
Look to the Spectrum!
Kristy had told me that her exposure plates were only sensitive to certain colors: UV, blue, and some green. There was a specific range of wavelengths this collodion emulsion was sensitive to â one that doesnât match human vision.
Human vision, as we can see above, is a sensitivity to the electromagnetic spectrum. Electromagnetic waves between about 400 and 700 nanometers will be perceived as light to us â the seven colors of the rainbow! (Interesting article about this to come later) If we dip to wavelengths shorter than 400, we get imperceivable ultraviolet (UV) light; rise to wavelengths above 700, we get imperceivable infrared (IR) light.
But What Do Vintage Photographic Emulsion Surfaces See?
Engineer Niles Lund seems to be the only person who has researched the spectral sensitivity of collodion, the emulsion chemical stew used to make wet-plate and tintype photographs in the mid-1800s. After exchanging a few emails, Niles sent me this updated spectral chart.
Spectral sensitivity of cadmium bromide, potassium iodide, and collodion consisting of the two. Available here.
So now we know what to recreate if we want âdigital collodionâ! We need to cut out the upper half of the visible spectrum allowing nothing above about 520 nanometers into the camera.
Iâve done much work in this field, so I thought this would be a great time to blend science and art to explore this concept and compare it to other hyper-spectral imagery!
Setting Up an Experiment
Now we invite some friends and models to come sit for us! I asked the models to avoid skin-concealing makeup and zinc-oxide sunscreen, which is the only sunscreen to obstruct UV-A rays. They were asked to model under a broad-spectrum light source (the sun), with an additional light source of a silver reflector, and maintain a pose for a few minutes while I photograph each under the following conditions:
Visible Spectrum
This is what humans see (obviously). Using three cones, sensitive to three different ranges of wavelengths, which loosely map to red-green-blue, we see everything from violet to red. Violet is the shortest wavelength. Red is the longest. Not surprisingly, this is what digital cameras see, as well. Silicon photovoltaic sensors, whether CCD or CMOS, can take in a theoretical 290nm to 1100nm. However, it would be maddening to have your photography overexposed, blooming, and flaring due to lightwaves you cannot see, to say nothing of threats of overheating, so sensors are limited by optical filters placed directly over the sensor to only photograph, more-or-less, in the human visible spectrum. Photons that donât play nice in this range are either bounced back out of the lens or absorbed and destroyed.
Hereâs what our model Kayla looked like in the visible spectrum:
Captured on an unmodified Nikon D610 through a normal UV-limiting 50mm lens. Notice her light freckles, dark shirt, and slightly light eyes
Orthochromatic Blue (Collodion AND Squid Vision)
This could be seen as the same spectrum that octopus and squid might view in. Humans, except for rare and awesome mutants and the colorblind, are âtrichromaticâ, with three cones that allow for three different ranges of wavelengths in. Cephalopods only have one, the S cone, which is sensitive in almost the exact fashion of the spectral curve of the 1850âs collodion (except for UV). Though they might have a different mechanical use of their eyes, which could allow for more color vision, but I digressâŚ
By using Schott BG25 bandpass glass, bought off a gentleman who fits esoteric german glass into filters, on a lens that cuts UV (any normal lens) on any unmodified camera, we get a theoretical spectral sensitivity that looks like:
The âdouble bandpassâ ionically colored glass made by Schott AG in Germany nicely mimics the spectral response of the collodion.
Of course, all the photons that this can transmit that the camera canât capture become irrelevant, so on an unmodified camera, we will get a spectral response that looks like:
This means that on an unmodified camera, using a lens that doesnât block UV, weâre going to get an image where the focus is going to be more on near-UV damage to the skin â concentrations of freckles and blemishes are going to much more pronounced. Thereâs going to be a light leak of very red photons, and weâll be missing much of the UV range, but weâre very close to vintage emulsion now.
Our model, under the same lighting conditions (with an exposure compensation of +.7, calibrated from an 18% gray card):
Captured on an unmodified Nikon D610 through a UV-permitting 50mm lens and a BG25 double bandpass filter. Notice her pronounced freckles, dark shirt, and very light eyes
By gum, weâve done it! With that nanty narking, I think we can say weâve captured the olâ timey feel of collodion!
âŚbut it is not scientifically accurate. This is about the best we can do on an unmodified camera, as we need some expensive modifications to allow UV to extend into the sensor. More on that later.
Letâs see what she looks like in some other, more bizarre wavelengths!
Near Infrared and Short Wave Infrared (Snake Vision)
This is, likely, what snakes see. This is the orange-and-red human-visible spectrum (near infrared, or NIR) and the infrared waves closer to the human spectrum. This is what I like to photograph foliage in because it can be false-colored and remapped using the color channels that your camera knows how to deliver data in. I have a Nikon D80 that has been modified to shoot in this wavelength, with an upper infrared spectrum bound (unmeasured) limited only by the physical limitations of a silicon CCD.
For some reason, for the duration of this experiment, this setup absolutely refused to focus properly. This camera does not have live-view, and it is very difficult to assess these images until theyâve been processed on a computer. Nonetheless, these blurry images were interestingâŚ
Captured on a modified Nikon D80 through normal 50mm lens, no filter. Notice there are no freckles, and her shirt appears grey.
Short Wave Infrared
I donât think anything âseesâ solely in this spectrum, though it is a difficult concept to explore, so perhaps time will tell. To capture the full breadth of short wave infrared, or to move into longer wave infrared, we would have to use a special sensor made out of indium gallium arsenide. Itâs interesting to see that any sun damage and melanin deposits, such as freckles and tans, will not appear in this wavelength. The IR photons do a good deal more sub-surface scattering in the skin than visible light, rendering each person as a waxen figure.
Captured on a modified Nikon D80 through a normal 50mm lens and a BG25 double bandpass filter. Notice there are no freckles, her shirt now appears white, her eyes have darkened considerably, and all of her skin appears waxen and soft.
Polishing these photos up a bit in a vintage style split-toning
You might have noticed these are not delivered in monochromatic black and white, as in the style of a silver gelatin process. Instead, I chose to render them out with a bit of split-toning. Since the birth of photography, this was a common practice, using various methods to bring color to the highlights and to the shadows.
In this case, I made the highlights gold to mimic the flame-gilding of the mid-1800s, which âfixedâ the highlights of a photograph using a gold chloride solution and an open flame. As ambrotypes caught on in the 1860s, ârubyâ hued backs became more common in photography, so I enjoy using a red tint in my lowlights.
Photo Results
Kaitlin
Visible
Collodion
Kevin
Visible
Collodion
Christine
Visible
Collodion
Xach
Visible
Collodion
Caitlin
Visible
Collodion
Kayla
Visible
Collodion
Next Steps to Take it Further
In order to fully and accurately capture the collodion sensitivity range, I need to not only use a lens that allows ultraviolet photons to pass, as used in this experiment, but also a camera with a sensor modified to receive UV light. Because this is not a fast or cheap prospect, Iâve been dragging my feet to see if this could be done in conjunction with another modificationâŚ
As photographing humans draws no benefit from presenting false color, hyperspectral photography (such as UV) is best delivered in black and white. Having a color sensor actually hurts collodion-spectrum portraiture â if the construction of the image relies on a missing red channel, then we need to have a monochromatic sensor to gather an image using the filters described in this process with due sharpness.
We have different options: Buy a monochromatic camera, which can run between 3,000 and 50,000 dollars, or do a âmono-modâ to scrape the Bayer filter off of an existing camera. Conversion shops have assured me this is impossible on the Nikon cameras that I like to use, but I hold out hope for a âfull spectrumâ monochromatic modification. Alternatively, I can also look into intelligent upscaling, possibly using AI to regain resolution lost in the process.
Final Thoughts
As I used attractive models in this experiment, instead of the lab tools one should use to characterize optics, the spectral range of each photograph is an educated guess at best, based on theoretical readings. Lab characterizations will follow.
Trust the math. I know things look underexposed and incorrect, but if youâve set your exposure compensation based off an 18% gray card â or, realistically, ignored that and just set it to +.7, then you can trust your lightmeter shooting in âorthochromatic blueâ.
Sometimes, thereâs no substitute for the real thing â recreating wet-plate collodion and tintypes is severely limited by digital consideration â but limitation is the mother of invention!
Editorâs note: A longer and more comprehensive version of this article can be found on Warrenâs website. In it, Warren also discusses challenges he encountered with Bayer filters and how he wrote his own demosaic algorithm script to overcome them.
About the author: Phil Warren is a photographer, engineer, explorer, and adventurer. The opinions expressed in this article are solely those of the author. You can find more of Warrenâs work and writing on his website and Instagram.
source https://petapixel.com/2019/04/05/recreating-the-look-of-1850s-tintypes-in-digital-with-math-and-science/
0 notes
Text
Recreating the Look of 1850s Tintypes in Digital with Math and Science
While I was visiting San Francisco, Kristy Headley, a dear friend and fellow engineer, showed me her studio. There I was lucky enough to sit for her while she did some vintage tintyping. Tintyping was one of the earliest forms of photography, popular in the 1850s.
It was a kind of incredibly inconvenient Polaroid â the photos were exposed instantly after a quick wash on plates of iron or aluminum (never actually tin). All you needed was a very, very large camera, plates treated with âcollodionâ to make a light-reactive surface, and a sizable collection of chemicals. Unfortunately, the genuine process has a high risk of explosion, creating cyanide gas, incurring blindness from silver halide splashback, or getting whacked out on ether.
âDustyâ. Tintype/ferrotype by Quinn Jacobson and licensed under CC BY-SA 3.0
Kristy showed me each painstaking step, and I was enamored with the result. With her mastery of lighting techniques, sheâd produced tintype photographs where every detail popped â every freckle, hair, and eyelash seemed to stand out. Yet, these looked very different from modern photography. There was a certain, seemingly unquantifiable trait to them. Why?
I sought out to quantify this olâ timey look and the fashion by which one could reproduce it with a digital camera. Perhaps I could share in this delight without keeping jugs of volatile chemicals in my room.
Look to the Spectrum!
Kristy had told me that her exposure plates were only sensitive to certain colors: UV, blue, and some green. There was a specific range of wavelengths this collodion emulsion was sensitive to â one that doesnât match human vision.
Human vision, as we can see above, is a sensitivity to the electromagnetic spectrum. Electromagnetic waves between about 400 and 700 nanometers will be perceived as light to us â the seven colors of the rainbow! (Interesting article about this to come later) If we dip to wavelengths shorter than 400, we get imperceivable ultraviolet (UV) light; rise to wavelengths above 700, we get imperceivable infrared (IR) light.
But What Do Vintage Photographic Emulsion Surfaces See?
Engineer Niles Lund seems to be the only person who has researched the spectral sensitivity of collodion, the emulsion chemical stew used to make wet-plate and tintype photographs in the mid-1800s. After exchanging a few emails, Niles sent me this updated spectral chart.
Spectral sensitivity of cadmium bromide, potassium iodide, and collodion consisting of the two. Available here.
So now we know what to recreate if we want âdigital collodionâ! We need to cut out the upper half of the visible spectrum allowing nothing above about 520 nanometers into the camera.
Iâve done much work in this field, so I thought this would be a great time to blend science and art to explore this concept and compare it to other hyper-spectral imagery!
Setting Up an Experiment
Now we invite some friends and models to come sit for us! I asked the models to avoid skin-concealing makeup and zinc-oxide sunscreen, which is the only sunscreen to obstruct UV-A rays. They were asked to model under a broad-spectrum light source (the sun), with an additional light source of a silver reflector, and maintain a pose for a few minutes while I photograph each under the following conditions:
Visible Spectrum
This is what humans see (obviously). Using three cones, sensitive to three different ranges of wavelengths, which loosely map to red-green-blue, we see everything from violet to red. Violet is the shortest wavelength. Red is the longest. Not surprisingly, this is what digital cameras see, as well. Silicon photovoltaic sensors, whether CCD or CMOS, can take in a theoretical 290nm to 1100nm. However, it would be maddening to have your photography overexposed, blooming, and flaring due to lightwaves you cannot see, to say nothing of threats of overheating, so sensors are limited by optical filters placed directly over the sensor to only photograph, more-or-less, in the human visible spectrum. Photons that donât play nice in this range are either bounced back out of the lens or absorbed and destroyed.
Hereâs what our model Kayla looked like in the visible spectrum:
Captured on an unmodified Nikon D610 through a normal UV-limiting 50mm lens. Notice her light freckles, dark shirt, and slightly light eyes
Orthochromatic Blue (Collodion AND Squid Vision)
This could be seen as the same spectrum that octopus and squid might view in. Humans, except for rare and awesome mutants and the colorblind, are âtrichromaticâ, with three cones that allow for three different ranges of wavelengths in. Cephalopods only have one, the S cone, which is sensitive in almost the exact fashion of the spectral curve of the 1850âs collodion (except for UV). Though they might have a different mechanical use of their eyes, which could allow for more color vision, but I digressâŚ
By using Schott BG25 bandpass glass, bought off a gentleman who fits esoteric german glass into filters, on a lens that cuts UV (any normal lens) on any unmodified camera, we get a theoretical spectral sensitivity that looks like:
The âdouble bandpassâ ionically colored glass made by Schott AG in Germany nicely mimics the spectral response of the collodion.
Of course, all the photons that this can transmit that the camera canât capture become irrelevant, so on an unmodified camera, we will get a spectral response that looks like:
This means that on an unmodified camera, using a lens that doesnât block UV, weâre going to get an image where the focus is going to be more on near-UV damage to the skin â concentrations of freckles and blemishes are going to much more pronounced. Thereâs going to be a light leak of very red photons, and weâll be missing much of the UV range, but weâre very close to vintage emulsion now.
Our model, under the same lighting conditions (with an exposure compensation of +.7, calibrated from an 18% gray card):
Captured on an unmodified Nikon D610 through a UV-permitting 50mm lens and a BG25 double bandpass filter. Notice her pronounced freckles, dark shirt, and very light eyes
By gum, weâve done it! With that nanty narking, I think we can say weâve captured the olâ timey feel of collodion!
âŚbut it is not scientifically accurate. This is about the best we can do on an unmodified camera, as we need some expensive modifications to allow UV to extend into the sensor. More on that later.
Letâs see what she looks like in some other, more bizarre wavelengths!
Near Infrared and Short Wave Infrared (Snake Vision)
This is, likely, what snakes see. This is the orange-and-red human-visible spectrum (near infrared, or NIR) and the infrared waves closer to the human spectrum. This is what I like to photograph foliage in because it can be false-colored and remapped using the color channels that your camera knows how to deliver data in. I have a Nikon D80 that has been modified to shoot in this wavelength, with an upper infrared spectrum bound (unmeasured) limited only by the physical limitations of a silicon CCD.
For some reason, for the duration of this experiment, this setup absolutely refused to focus properly. This camera does not have live-view, and it is very difficult to assess these images until theyâve been processed on a computer. Nonetheless, these blurry images were interestingâŚ
Captured on a modified Nikon D80 through normal 50mm lens, no filter. Notice there are no freckles, and her shirt appears grey.
Short Wave Infrared
I donât think anything âseesâ solely in this spectrum, though it is a difficult concept to explore, so perhaps time will tell. To capture the full breadth of short wave infrared, or to move into longer wave infrared, we would have to use a special sensor made out of indium gallium arsenide. Itâs interesting to see that any sun damage and melanin deposits, such as freckles and tans, will not appear in this wavelength. The IR photons do a good deal more sub-surface scattering in the skin than visible light, rendering each person as a waxen figure.
Captured on a modified Nikon D80 through a normal 50mm lens and a BG25 double bandpass filter. Notice there are no freckles, her shirt now appears white, her eyes have darkened considerably, and all of her skin appears waxen and soft.
Polishing these photos up a bit in a vintage style split-toning
You might have noticed these are not delivered in monochromatic black and white, as in the style of a silver gelatin process. Instead, I chose to render them out with a bit of split-toning. Since the birth of photography, this was a common practice, using various methods to bring color to the highlights and to the shadows.
In this case, I made the highlights gold to mimic the flame-gilding of the mid-1800s, which âfixedâ the highlights of a photograph using a gold chloride solution and an open flame. As ambrotypes caught on in the 1860s, ârubyâ hued backs became more common in photography, so I enjoy using a red tint in my lowlights.
Photo Results
Kaitlin
Visible
Collodion
Kevin
Visible
Collodion
Christine
Visible
Collodion
Xach
Visible
Collodion
Caitlin
Visible
Collodion
Kayla
Visible
Collodion
Next Steps to Take it Further
In order to fully and accurately capture the collodion sensitivity range, I need to not only use a lens that allows ultraviolet photons to pass, as used in this experiment, but also a camera with a sensor modified to receive UV light. Because this is not a fast or cheap prospect, Iâve been dragging my feet to see if this could be done in conjunction with another modificationâŚ
As photographing humans draws no benefit from presenting false color, hyperspectral photography (such as UV) is best delivered in black and white. Having a color sensor actually hurts collodion-spectrum portraiture â if the construction of the image relies on a missing red channel, then we need to have a monochromatic sensor to gather an image using the filters described in this process with due sharpness.
We have different options: Buy a monochromatic camera, which can run between 3,000 and 50,000 dollars, or do a âmono-modâ to scrape the Bayer filter off of an existing camera. Conversion shops have assured me this is impossible on the Nikon cameras that I like to use, but I hold out hope for a âfull spectrumâ monochromatic modification. Alternatively, I can also look into intelligent upscaling, possibly using AI to regain resolution lost in the process.
Final Thoughts
As I used attractive models in this experiment, instead of the lab tools one should use to characterize optics, the spectral range of each photograph is an educated guess at best, based on theoretical readings. Lab characterizations will follow.
Trust the math. I know things look underexposed and incorrect, but if youâve set your exposure compensation based off an 18% gray card â or, realistically, ignored that and just set it to +.7, then you can trust your lightmeter shooting in âorthochromatic blueâ.
Sometimes, thereâs no substitute for the real thing â recreating wet-plate collodion and tintypes is severely limited by digital consideration â but limitation is the mother of invention!
Editorâs note: A longer and more comprehensive version of this article can be found on Warrenâs website. In it, Warren also discusses challenges he encountered with Bayer filters and how he wrote his own demosaic algorithm script to overcome them.
About the author: Phil Warren is a photographer, engineer, explorer, and adventurer. The opinions expressed in this article are solely those of the author. You can find more of Warrenâs work and writing on his website and Instagram.
from Photography News https://petapixel.com/2019/04/05/recreating-the-look-of-1850s-tintypes-in-digital-with-math-and-science/
0 notes