#postcentral gyrus
Explore tagged Tumblr posts
Text

The Science Research Notebooks of S. Sunkavally, p 382.
#receptor layer of the retina#pigmented epithelium#analgesia#tapetum lucidum#blood clotting#calcium#postcentral gyrus#scarring#tribal practise#ascorbic acid requirements#scar#aeration of a liquid medium#peptides#nocturnal migration of sealife#satyendra sunkavally#manuscripts#notebooks#diaries#theoretical biology
0 notes
Note
I've been fed with your posts :)
You shall no longer be hunted for your Precentral gyrus, Central sulcus, Postcentral gyrus, and Limbic lobe!! ^^
Isn't that great?!? :3
I just saw this
YAY!!! IM SO HWPOY!!! NO MORE DEATH FOR ME!!!
2 notes
·
View notes
Text
While right-handedness is considered the “default”, or what is “correct”, left-handedness is quite common, with about 10% of the population being left-handed. However, this estimate is uncertain, as many people have been forced to predominantly use their right hand. Handedness is not a coincidence, but rather has a neurological explanation behind it.
The brain is split into two sides, the right and the left, and each side of the brain controls the opposite side of the body. The left hemisphere is often referred to as the logical, straightforward side, while the right is the artistic and emotional side. The left hemisphere of the brain controls movements of the right hand and vice versa. The biggest theory behind hand preference argues that evolutionary natural selection resulted in the majority of individuals with speech and language control in the left hemisphere of the brain. Because of the need to produce written language, evolutionary development resulted in a preference for right-handedness, as the left hemisphere controls speech and language.
There are genetic theories behind hand preference. It has been proposed there are two genes that are associated with handedness, one called the D gene for dextral, or right hand, and the other called the C gene for chance. The D gene, which is more frequent in populations, results in right hand preference, whereas the C gene, which is less common, results in individuals with a 50% chance of being right-handed and a 50% chance of being left-handed. However, genetic linkage analyses in families with a large number of left-handed members have not found convincing evidence for a single major gene. It is known there is a genetic component. For example, one study of over 25,000 pairs of twins revealed that the preferred hand for writing or drawing is a weak genetic trait with a heritability of 24%. This supports the genetic component of handedness, but also confirms there are environmental components as well. Although there are single gene theories that correlate with data on the prevalence of handedness, studies have failed to identify a single gene locus responsible for handedness. Additionally, genome-wide association studies (GWASs) for handedness have found no statistically significant associations.

When looking at the brain structures of a left-handed versus right-handed person, brain hemispheres tend to be more symmetrical in left-handed and ambidextrous individuals. Out of right-handed individuals, 95% have brains that strictly divide up tasks—the left hemisphere almost exclusively handles language and speech, whereas the right hemisphere handles emotion and image processing. However, in left-handed individuals, only 20% have brains that divide up these tasks so concretely. This is likely due to the correlation between written language and speech and language as controlled through the brain, with language control shifting to the right-hemisphere in left-handed individuals. One study looked at asymmetry between different handed persons using MRIs. When looking at cortical thickness—the thickness of the cortex, a region of the brain—asymmetries, there were two clusters where left-handed individuals differed on average from right-handers, one on the postcentral gyrus, and one on the inferior occipital gyrus. For all surface areas and thickness asymmetries within these significant clusters, left-handed persons had lower average asymmetry indexes than right-handers. To further explain this, if a cluster in right-handed persons was more asymmetrical to the right, it had an even stronger rightward asymmetry in left-handed persons. And if a cluster in right-handed persons was more asymmetrical to the left, it had a weaker leftward asymmetry in left-handers. This study concluded that left-handedness is associated with a neural shift to the dominant right hemisphere for hand motor control, within all the significant cortical clusters associated with handedness.
Another study looked to identify structural brain differences in different handed individuals. They looked at 21 right-handed people and 21 non right-handed people (left-handed or ambidextrous). Measures of the cortex such as thickness, surface area, volume, and curvature, as well as volumes of subcortical structures—regions below the cortex—such as the amygdala, caudate, hippocampus, globus pallidus, putamen, and thalamus, were were compared between the two groups using whole brain 3D T1 weighted MRI. They found the volumes of the right putamen and left globus pallidus in non right‐handed participants were significantly larger than those who were right‐handed. They concluded that there were significant differences in brain morphology between right‐handed and non right‐handed individuals, especially in the basal ganglia, which could produce differences in motor control according to handedness. The basal ganglia are a group of subcortical nuclei within the brain that are primarily responsible for motor control. Thus differences in these nuclei in right versus left-handed individuals could explain the differences in motor control.
While a specific gene has not been discovered to be responsible for handedness, it is clear that handedness is a result of brain development related to speech and language. Clear symmetry differences of brain hemispheres are present between right and left-handed individuals, as are brain morphology differences in general.
2 notes
·
View notes
Text
Hypothetical Neuroanatomy Vernacular
Mapping the brain and renaming its crucial areas, such as each gyrus, with the names of cathedrals and basilicas from the Catholic religion is an imaginative way to reframe neuroscience with cultural and architectural references. Here's how this could look conceptually:
Frontal Lobe: Precentral Gyrus (Motor Cortex): St. Peter's Basilica (Vatican City) – This gyrus is essential for voluntary motor movements, much like how St. Peter's is a central figure in the Vatican, symbolizing leadership. Superior Frontal Gyrus: Notre-Dame Cathedral (Paris, France) – Known for cognitive functions like self-awareness, similar to the iconic and reflective grandeur of Notre-Dame. Middle Frontal Gyrus: St. Paul's Cathedral (London, UK) – Associated with complex thinking and decision-making, just as St. Paul's is a historic site for public discourse and debate. Inferior Frontal Gyrus: Sagrada Família (Barcelona, Spain) – Tied to language processing and creativity, which aligns with the artistic and unique design of Sagrada Família.
Parietal Lobe: Postcentral Gyrus (Somatosensory Cortex): Cathedral of Santiago de Compostela (Spain) – This area processes sensory information, akin to the pilgrimages to Santiago de Compostela, symbolizing a gathering of experiences. Superior Parietal Lobule: Cologne Cathedral (Germany) – Involved in spatial orientation and perception, reminiscent of Cologne's towering structure, which dominates the city's skyline. Inferior Parietal Lobule: Duomo di Milano (Milan Cathedral) (Italy) – Functions in integrating sensory information, paralleling the complex and intricate design of Milan Cathedral. Temporal Lobe: Superior Temporal Gyrus: Basilica of the National Shrine of the Immaculate Conception (Washington, D.C., USA) – Important for auditory processing, like how this basilica represents a hub of sound, liturgy, and music. Middle Temporal Gyrus: St. Mark's Basilica (Venice, Italy) – Plays a role in semantic memory, just as St. Mark's is central to the cultural and historical memory of Venice. Inferior Temporal Gyrus: Basilica of Guadalupe (Mexico City, Mexico) – Related to visual processing, echoing the iconic image of the Virgin of Guadalupe, an important visual symbol in Catholicism.
Occipital Lobe: Cuneus: Chartres Cathedral (France) – Involved in basic visual processing, akin to Chartres’ famous stained glass, symbolizing the transmission of light and vision. Lingual Gyrus: Basilica di San Francesco (Assisi) (Italy) – Tied to visual recognition, similar to the frescoes of Assisi, which convey visual narratives and stories.
Limbic System: Cingulate Gyrus: Cathedral of Brasília (Brazil) – Associated with emotion and behavior regulation, comparable to the modern and innovative design of Brasília's cathedral, symbolizing new directions and forms of expression. Parahippocampal Gyrus: Basilica of the Sacred Heart (Montmartre, Paris) – Involved in memory encoding, like how the basilica's hilltop location provides a panoramic memory of Paris. Hippocampus: St. Stephen's Cathedral (Vienna, Austria) – Essential for memory and learning, much like St. Stephen’s serves as a historical and cultural repository. Insula: Cathedral of Santa Maria del Fiore (Florence) (Italy) – Involved in emotional processing and bodily awareness, much like the dome of Santa Maria del Fiore, which is an architectural marvel inspiring awe and emotional response.
Cerebellum: Westminster Cathedral (London, UK) – Known for motor coordination and balance, comparable to Westminster's architectural balance and historical significance.
Brainstem: Basilica di San Giovanni in Laterano (Rome) (Italy) – A vital structure for life support functions, just as San Giovanni in Laterano is one of the most ancient and essential basilicas in Catholicism, serving as the ecclesiastical seat of the Pope.
Corpus Callosum: Cathedral of Seville (Spain) – Facilitates communication between brain hemispheres, similar to Seville's cathedral, which bridges Gothic, Renaissance, and Moorish architectural styles.
This thematic naming not only assigns each area of the brain a cultural landmark but also allows for a creative representation of their function and significance, blending neuroscience with art, history, and spirituality.
0 notes
Quote
The dorsal column-medial lemniscus pathway (DCML) is a sensory pathway of the central nervous system. It conveys sensation of fine touch, vibration, pressure, two-point discrimination and proprioception (position) from the skin and joints. Also known as the posterior column - medial lemniscus pathway, it consists of two parts. The dorsal (posterior) column, which runs from the spinal cord to the medulla, and the medial lemniscus which runs as a continuation of the dorsal column, from the medulla to the cortex. In the cortex the DCML pathway projects onto the primary somatosensory cortex of the postcentral gyrus. Here sensation location is 'mapped' using a somatotopic arrangement, represented as a homunculus.
Dorsal column-medial lemniscus (DCML) pathway: Anatomy | Kenhub
0 notes
Text
Gross anatomy See also: Evolution of the brain § Evolution of the human brain, and Neuroscience of sex differences The adult human brain weighs on average about 1.2–1.4 kg (2.6–3.1 lb) which is about 2% of the total body weight,[2][3] with a volume of around 1260 cm3 in men and 1130 cm3 in women.[4] There is substantial individual variation,[4] with the standard reference range for men being 1,180–1,620 g (2.60–3.57 lb)[5] and for women 1,030–1,400 g (2.27–3.09 lb).[6]
The cerebrum, consisting of the cerebral hemispheres, forms the largest part of the brain and overlies the other brain structures.[7] The outer region of the hemispheres, the cerebral cortex, is grey matter, consisting of cortical layers of neurons. Each hemisphere is divided into four main lobes – the frontal lobe, parietal lobe, temporal lobe, and occipital lobe.[8] Three other lobes are included by some sources which are a central lobe, a limbic lobe, and an insular lobe.[9] The central lobe comprises the precentral gyrus and the postcentral gyrus and is included since it forms a distinct functional role.[9][10]
The brainstem, resembling a stalk, attaches to and leaves the cerebrum at the start of the midbrain area. The brainstem includes the midbrain, the pons, and the medulla oblongata. Behind the brainstem is the cerebellum (Latin: little brain).[7]
The cerebrum, brainstem, cerebellum, and spinal cord are covered by four[11] membranes called meninges. The membranes are the tough dura mater; the middle arachnoid mater and the more delicate inner pia mater. Between the arachnoid mater and the pia mater is the subarachnoid space and subarachnoid cisterns, which contain the cerebrospinal fluid.[12] The outermost membrane of the cerebral cortex is the basement membrane of the pia mater called the glia limitans and is an important part of the blood–brain barrier.[13] The living brain is very soft, having a gel-like consistency similar to soft tofu.[14] The cortical layers of neurons constitute much of the cerebral grey matter, while the deeper subcortical regions of myelinated axons, make up the white matter.[7] The white matter of the brain makes up about half of the total brain volume.[15]
Structural and functional areas of the human brain A diagram showing various structures within the human brain Human brain bisected in the sagittal plane, showing the white matter of the corpus callosum A diagram of the functional areas of the human brain Functional areas of the human brain. Dashed areas shown are commonly left hemisphere dominant. Cerebrum Main articles: Cerebrum and Cerebral cortex
Major gyri and sulci on the lateral surface of the cortex
Lobes of the brain The cerebrum is the largest part of the brain and is divided into nearly symmetrical left and right hemispheres by a deep groove, the longitudinal fissure.[16] Asymmetry between the lobes is noted as a petalia.[17] The hemispheres are connected by five commissures that span the longitudinal fissure, the largest of these is the corpus callosum.[7] Each hemisphere is conventionally divided into four main lobes; the frontal lobe, parietal lobe, temporal lobe, and occipital lobe, named according to the skull bones that overlie them.[8] Each lobe is associated with one or two specialised functions though there is some functional overlap between them.[18] The surface of the brain is folded into ridges (gyri) and grooves (sulci), many of which are named, usually according to their position, such as the frontal gyrus of the frontal lobe or the central sulcus separating the central regions of the hemispheres. There are many small variations in the secondary and tertiary folds.[19]
The outer part of the cerebrum is the cerebral cortex, made up of grey matter arranged in layers. It is 2 to 4 millimetres (0.079 to 0.157 in) thick, and deeply folded to give a convoluted appearance.[20] Beneath the cortex is the cerebral white matter. The largest part of the cerebral cortex is the neocortex, which has six neuronal layers. The rest of the cortex is of allocortex, which has three or four layers.[7]
The cortex is mapped by divisions into about fifty different functional areas known as Brodmann's areas. These areas are distinctly different when seen under a microscope.[21] The cortex is divided into two main functional areas – a motor cortex and a sensory cortex.[22] The primary motor cortex, which sends axons down to motor neurons in the brainstem and spinal cord, occupies the rear portion of the frontal lobe, directly in front of the somatosensory area. The primary sensory areas receive signals from the sensory nerves and tracts by way of relay nuclei in the thalamus. Primary sensory areas include the visual cortex of the occipital lobe, the auditory cortex in parts of the temporal lobe and insular cortex, and the somatosensory cortex in the parietal lobe. The remaining parts of the cortex are called the association areas. These areas receive input from the sensory areas and lower parts of the brain and are involved in the complex cognitive processes of perception, thought, and decision-making.[23] The main functions of the frontal lobe are to control attention, abstract thinking, behaviour, problem-solving tasks, and physical reactions and personality.[24][25] The occipital lobe is the smallest lobe; its main functions are visual reception, visual-spatial processing, movement, and colour recognition.[24][25] There is a smaller occipital lobule in the lobe known as the cuneus. The temporal lobe controls auditory and visual memories, language, and some hearing and speech.[24]
Cortical folds and white matter in horizontal bisection of head The cerebrum contains the ventricles where the cerebrospinal fluid is produced and circulated. Below the corpus callosum is the septum pellucidum, a membrane that separates the lateral ventricles. Beneath the lateral ventricles is the thalamus and to the front and below is the hypothalamus. The hypothalamus leads on to the pituitary gland. At the back of the thalamus is the brainstem.[26]
The basal ganglia, also called basal nuclei, are a set of structures deep within the hemispheres involved in behaviour and movement regulation.[27] The largest component is the striatum, others are the globus pallidus, the substantia nigra and the subthalamic nucleus.[27] The striatum is divided into a ventral striatum, and dorsal striatum, subdivisions that are based upon function and connections. The ventral striatum consists of the nucleus accumbens and the olfactory tubercle whereas the dorsal striatum consists of the caudate nucleus and the putamen. The putamen and the globus pallidus lie separated from the lateral ventricles and thalamus by the internal capsule, whereas the caudate nucleus stretches around and abuts the lateral ventricles on their outer sides.[28] At the deepest part of the lateral sulcus between the insular cortex and the striatum is a thin neuronal sheet called the claustrum.[29]
Below and in front of the striatum are a number of basal forebrain structures. These include the nucleus basalis, diagonal band of Broca, substantia innominata, and the medial septal nucleus. These structures are important in producing the neurotransmitter, acetylcholine, which is then distributed widely throughout the brain. The basal forebrain, in particular the nucleus basalis, is considered to be the major cholinergic output of the central nervous system to the striatum and neocortex.[30]
Cerebellum
Human brain viewed from below, showing cerebellum and brainstem Main article: Cerebellum The cerebellum is divided into an anterior lobe, a posterior lobe, and the flocculonodular lobe.[31] The anterior and posterior lobes are connected in the middle by the vermis.[32] Compared to the cerebral cortex, the cerebellum has a much thinner outer cortex that is narrowly furrowed into numerous curved transverse fissures.[32] Viewed from underneath between the two lobes is the third lobe the flocculonodular lobe.[33] The cerebellum rests at the back of the cranial cavity, lying beneath the occipital lobes, and is separated from these by the cerebellar tentorium, a sheet of fibre.[34]
It is connected to the brainstem by three pairs of nerve tracts called cerebellar peduncles. The superior pair connects to the midbrain; the middle pair connects to the medulla, and the inferior pair connects to the pons.[32] The cerebellum consists of an inner medulla of white matter and an outer cortex of richly folded grey matter.[34] The cerebellum's anterior and posterior lobes appear to play a role in the coordination and smoothing of complex motor movements, and the flocculonodular lobe in the maintenance of balance[35] although debate exists as to its cognitive, behavioural and motor functions.[36]
Brainstem Main article: Brainstem The brainstem lies beneath the cerebrum and consists of the midbrain, pons and medulla. It lies in the back part of the skull, resting on the part of the base known as the clivus, and ends at the foramen magnum, a large opening in the occipital bone. The brainstem continues below this as the spinal cord,[37] protected by the vertebral column.
Ten of the twelve pairs of cranial nerves[a] emerge directly from the brainstem.[37] The brainstem also contains many cranial nerve nuclei and nuclei of peripheral nerves, as well as nuclei involved in the regulation of many essential processes including breathing, control of eye movements and balance.[38][37] The reticular formation, a network of nuclei of ill-defined formation, is present within and along the length of the brainstem.[37] Many nerve tracts, which transmit information to and from the cerebral cortex to the rest of the body, pass through the brainstem.[37]
Microanatomy The human brain is primarily composed of neurons, glial cells, neural stem cells, and blood vessels. Types of neuron include interneurons, pyramidal cells including Betz cells, motor neurons (upper and lower motor neurons), and cerebellar Purkinje cells. Betz cells are the largest cells (by size of cell body) in the nervous system.[39] The adult human brain is estimated to contain 86±8 billion neurons, with a roughly equal number (85±10 billion) of non-neuronal cells.[40] Out of these neurons, 16 billion (19%) are located in the cerebral cortex, and 69 billion (80%) are in the cerebellum.[3][40]
Types of glial cell are astrocytes (including Bergmann glia), oligodendrocytes, ependymal cells (including tanycytes), radial glial cells, microglia, and a subtype of oligodendrocyte progenitor cells. Astrocytes are the largest of the glial cells. They are stellate cells with many processes radiating from their cell bodies. Some of these processes end as perivascular end-feet on capillary walls.[41] The glia limitans of the cortex is made up of astrocyte foot processes that serve in part to contain the cells of the brain.[13]
Mast cells are white blood cells that interact in the neuroimmune system in the brain.[42] Mast cells in the central nervous system are present in a number of structures including the meninges;[42] they mediate neuroimmune responses in inflammatory conditions and help to maintain the blood–brain barrier, particularly in brain regions where the barrier is absent.[42][43] Mast cells serve the same general functions in the body and central nervous system, such as effecting or regulating allergic responses, innate and adaptive immunity, autoimmunity, and inflammation.[42] Mast cells serve as the main effector cell through which pathogens can affect the biochemical signaling that takes place between the gastrointestinal tract and the central nervous system.[44][45]
Some 400 genes are shown to be brain-specific. In all neurons, ELAVL3 is expressed, and in pyramidal neurons, NRGN and REEP2 are also expressed. GAD1 – essential for the biosynthesis of the neurotransmitter GABA – is expressed in interneurons. Proteins expressed in glial cells include astrocyte markers GFAP and S100B whereas myelin basic protein and the transcription factor OLIG2 are expressed in oligodendrocytes.[46]
Cerebrospinal fluid
Cerebrospinal fluid circulates in spaces around and within the brain Main article: Cerebrospinal fluid Cerebrospinal fluid is a clear, colourless transcellular fluid that circulates around the brain in the subarachnoid space, in the ventricular system, and in the central canal of the spinal cord. It also fills some gaps in the subarachnoid space, known as subarachnoid cisterns.[47] The four ventricles, two lateral, a third, and a fourth ventricle, all contain a choroid plexus that produces cerebrospinal fluid.[48] The third ventricle lies in the midline and is connected to the lateral ventricles.[47] A single duct, the cerebral aqueduct between the pons and the cerebellum, connects the third ventricle to the fourth ventricle.[49] Three separate openings, the middle and two lateral apertures, drain the cerebrospinal fluid from the fourth ventricle to the cisterna magna, one of the major cisterns. From here, cerebrospinal fluid circulates around the brain and spinal cord in the subarachnoid space, between the arachnoid mater and pia mater.[47] At any one time, there is about 150mL of cerebrospinal fluid – most within the subarachnoid space. It is constantly being regenerated and absorbed, and is replaced about once every 5–6 hours.[47]
A glymphatic system has been described[50][51][52] as the lymphatic drainage system of the brain. The brain-wide glymphatic pathway includes drainage routes from the cerebrospinal fluid, and from the meningeal lymphatic vessels that are associated with the dural sinuses, and run alongside the cerebral blood vessels.[53][54] The pathway drains interstitial fluid from the tissue of the brain.[54]
Blood supply Main article: Cerebral circulation
Two circulations joining at the circle of Willis (inferior view)
Diagram showing features of cerebral outer membranes and supply of blood vessels The internal carotid arteries supply oxygenated blood to the front of the brain and the vertebral arteries supply blood to the back of the brain.[55] These two circulations join in the circle of Willis, a ring of connected arteries that lies in the interpeduncular cistern between the midbrain and pons.[56]
The internal carotid arteries are branches of the common carotid arteries. They enter the cranium through the carotid canal, travel through the cavernous sinus and enter the subarachnoid space.[57] They then enter the circle of Willis, with two branches, the anterior cerebral arteries emerging. These branches travel forward and then upward along the longitudinal fissure, and supply the front and midline parts of the brain.[58] One or more small anterior communicating arteries join the two anterior cerebral arteries shortly after they emerge as branches.[58] The internal carotid arteries continue forward as the middle cerebral arteries. They travel sideways along the sphenoid bone of the eye socket, then upwards through the insula cortex, where final branches arise. The middle cerebral arteries send branches along their length.[57]
The vertebral arteries emerge as branches of the left and right subclavian arteries. They travel upward through transverse foramina which are spaces in the cervical vertebrae. Each side enters the cranial cavity through the foramen magnum along the corresponding side of the medulla.[57] They give off one of the three cerebellar branches. The vertebral arteries join in front of the middle part of the medulla to form the larger basilar artery, which sends multiple branches to supply the medulla and pons, and the two other anterior and superior cerebellar branches.[59] Finally, the basilar artery divides into two posterior cerebral arteries. These travel outwards, around the superior cerebellar peduncles, and along the top of the cerebellar tentorium, where it sends branches to supply the temporal and occipital lobes.[59] Each posterior cerebral artery sends a small posterior communicating artery to join with the internal carotid arteries.
Blood drainage Cerebral veins drain deoxygenated blood from the brain. The brain has two main networks of veins: an exterior or superficial network, on the surface of the cerebrum that has three branches, and an interior network. These two networks communicate via anastomosing (joining) veins.[60] The veins of the brain drain into larger cavities of the dural venous sinuses usually situated between the dura mater and the covering of the skull.[61] Blood from the cerebellum and midbrain drains into the great cerebral vein. Blood from the medulla and pons of the brainstem have a variable pattern of drainage, either into the spinal veins or into adjacent cerebral veins.[60]
The blood in the deep part of the brain drains, through a venous plexus into the cavernous sinus at the front, and the superior and inferior petrosal sinuses at the sides, and the inferior sagittal sinus at the back.[61] Blood drains from the outer brain into the large superior sagittal sinus, which rests in the midline on top of the brain. Blood from here joins with blood from the straight sinus at the confluence of sinuses.[61]
Blood from here drains into the left and right transverse sinuses.[61] These then drain into the sigmoid sinuses, which receive blood from the cavernous sinus and superior and inferior petrosal sinuses. The sigmoid drains into the large internal jugular veins.[61][60]
The blood–brain barrier The larger arteries throughout the brain supply blood to smaller capillaries. These smallest of blood vessels in the brain, are lined with cells joined by tight junctions and so fluids do not seep in or leak out to the same degree as they do in other capillaries; this creates the blood–brain barrier.[43] Pericytes play a major role in the formation of the tight junctions.[62] The barrier is less permeable to larger molecules, but is still permeable to water, carbon dioxide, oxygen, and most fat-soluble substances (including anaesthetics and alcohol).[43] The blood-brain barrier is not present in the circumventricular organs—which are structures in the brain that may need to respond to changes in body fluids—such as the pineal gland, area postrema, and some areas of the hypothalamus.[43] There is a similar blood–cerebrospinal fluid barrier, which serves the same purpose as the blood–brain barrier, but facilitates the transport of different substances into the brain due to the distinct structural characteristics between the two barrier systems.[43][63]
Development Main article: Development of the nervous system in humans Further information: Development of the human brain
Neurulation and neural crest cells Simple drawing of the lateral view of the three primary vesicle stage of the three to four week old embryo shown in different colors, and the five secondary vesicle stage of the five week old embryo shown in different colors and a lateral view of this Primary and secondary vesicle stages of development in the early embryo to the fifth week Very simple drawing of the front end of a human embryo, showing each vesicle of the developing brain in a different color. Brain of a human embryo in the sixth week of development At the beginning of the third week of development, the embryonic ectoderm forms a thickened strip called the neural plate.[64] By the fourth week of development the neural plate has widened to give a broad cephalic end, a less broad middle part and a narrow caudal end. These swellings are known as the primary brain vesicles and represent the beginnings of the forebrain (prosencephalon), midbrain (mesencephalon), and hindbrain (rhombencephalon).[65]
Neural crest cells (derived from the ectoderm) populate the lateral edges of the plate at the neural folds. In the fourth week—during the neurulation stage—the neural folds close to form the neural tube, bringing together the neural crest cells at the neural crest.[66] The neural crest runs the length of the tube with cranial neural crest cells at the cephalic end and caudal neural crest cells at the tail. Cells detach from the crest and migrate in a craniocaudal (head to tail) wave inside the tube.[66] Cells at the cephalic end give rise to the brain, and cells at the caudal end give rise to the spinal cord.[67]
The tube flexes as it grows, forming the crescent-shaped cerebral hemispheres at the head. The cerebral hemispheres first appear on day 32.[68] Early in the fourth week, the cephalic part bends sharply forward in a cephalic flexure.[66] This flexed part becomes the forebrain (prosencephalon); the adjoining curving part becomes the midbrain (mesencephalon) and the part caudal to the flexure becomes the hindbrain (rhombencephalon). These areas are formed as swellings known as the three primary brain vesicles. In the fifth week of development five secondary brain vesicles have formed.[69] The forebrain separates into two vesicles – an anterior telencephalon and a posterior diencephalon. The telencephalon gives rise to the cerebral cortex, basal ganglia, and related structures. The diencephalon gives rise to the thalamus and hypothalamus. The hindbrain also splits into two areas – the metencephalon and the myelencephalon. The metencephalon gives rise to the cerebellum and pons. The myelencephalon gives rise to the medulla oblongata.[70] Also during the fifth week, the brain divides into repeating segments called neuromeres.[65][71] In the hindbrain these are known as rhombomeres.[72]
A characteristic of the brain is the cortical folding known as gyrification. For just over five months of prenatal development the cortex is smooth. By the gestational age of 24 weeks, the wrinkled morphology showing the fissures that begin to mark out the lobes of the brain is evident.[73] Why the cortex wrinkles and folds is not well-understood, but gyrification has been linked to intelligence and neurological disorders, and a number of gyrification theories have been proposed.[73] These theories include those based on mechanical buckling,[74][18] axonal tension,[75] and differential tangential expansion.[74] What is clear is that gyrification is not a random process, but rather a complex developmentally predetermined process which generates patterns of folds that are consistent between individuals and most species.[74][76]
The first groove to appear in the fourth month is the lateral cerebral fossa.[68] The expanding caudal end of the hemisphere has to curve over in a forward direction to fit into the restricted space. This covers the fossa and turns it into a much deeper ridge known as the lateral sulcus and this marks out the temporal lobe.[68] By the sixth month other sulci have formed that demarcate the frontal, parietal, and occipital lobes.[68] A gene present in the human genome (ARHGAP11B) may play a major role in gyrification and encephalisation.[77]
0 notes
Text
All About the Forebrain
End Brain
Cortex
Corpus callosum
Limbic system
Basal ganglia
Olfactory bulb
Cortex
derived from Latin: “bark”
thin (3-4 mm) surface layer of the cerebral hemispheres
appears gray because of high concentration of cell bodies
cells are arranged in six layers
Other structures of the cortex:
Sulcus (plural sulci): a groove in the brain matter.
Central sulcus separates frontal lobe from parietal lobe.
Lateral sulcus separates temporal lobe from frontal and parietal lobe.
Gyrus (plural gyri): a small protrusion or bump formed by the folding of the cerebral cortex.
Physiological Divisions of the Cortex
Three “types”:
A. Primary Motor Cortex: precentral gyrus in frontal lobe
B. Primary Sensory Cortex:
somatosensory (touch, balance, joint position, pain): postcentral gyrus in parietal lobe
auditory (hearing): in temporal lobe
visual: in occipital lobe
C. Association cortex (in all lobes): accounts for about 90% of total cortex
Prefrontal Cortex
- Association cortex
- Part of frontal lobe
- Functions:
planning actions
decision making
emotional control
- Impaired in many psychiatric disorders
Corpus Callosum
- Latin for “tough body”
- Connects the two cerebal hemispheres
- Consists of white matter (neuronal fibers)
- Can be cut to treat severe epilepsy
Limbic System
- from the Latin limbus: “border, edge”
- Group of structures between the cortex and brain stem
- Principal structures:
amygdala
hippocampus
cingulate cortex
- Functions:
emotion
memory (hippocampus)
Basal Ganglia
- Latin: “large balls at the bottom”
- accumulations of the gray matter inside the hemispheres below the cortex
- Striatum (Latin: “striped mass”), globus pallidus (Latin: “pale ball”)
movement control (automatic movements, adjustment of movements)
Parkinson’s disease, Tourette’s syndrome
Nucleus accumbens (”adjacent” nucleus)
Reward, pleasure
Addiction
Between Brain AKA Diencephalon
Thalamus
Hypothalamus
Pineal gland
Thalamus
- Greek: “chamber”
- Gateway for channeling sensory information to the cortex
- Allows cortex to focus on selective sensory signals and diminishes significance of others
- Processing of visual, auditory, somatosensory, and gustatory information
Hypothalamus
- Greek: “under” thalamus
- Regulates body temperature, salt/water balance, hunger, thirst, energy metabolism, reproductive behaviors, and emotional responses
- Controls both the autonomic nervous system & the endocrine system.
- Releases hormones through connections with pituitary gland = hypophysis
drugs = exogenous chemicals
endogenous chemicals =
hormones: carried by blood, control body organs, work from a distance
neurotransmitters: work in the synaptic cleft, help neuronal cells communicate
enzymes: change hormones & neurotransmitters
More About the Hypothalamus
- The paraventricular nucleus in the hypothalamus regulates hormonal response to stress.
- HPA axis: stress response depends on the interaction of the hypothalamus, pituitary gland, and adrenal gland.
- Excessive stress response (cortisol) leads to damaging changes in brain & other body systems.
Pineal gland
- sleep control
- releases melatonin
5 notes
·
View notes
Text
A while back, this post happened and I mentioned an excessively detailed response, and here it is, presenting a (more or less) physiologically reasonable process for the entire function and breakdown of Garak's implant in “The Wire”. This breaks down into three main parts: how the implant is supposed to function, what went wrong, and how it's fixed. I'll add a TLDR as well. There's a lot of assumptions made (namely that Cardassian physiology is more or less functioning like Earth mammalian physiology), determinations that characters don't necessarily know exactly what they're talking about, simplification of exceedingly complicated things, and terminology.
Before we get into headcanoning and speculating, let's cover what we know of the implant from the episode. The implant is situated in the “postcentral gyrus and has filaments that connect it to [the] entire central nervous system” and “was designed to stimulate the pleasure centres of [the] brain to trigger the production of vast amounts of natural endorphins” in case of torture. The first symptoms include clammy skin*, dyspnea (difficulty breathing), miosis (contracted pupils), high level of pain (specifically a headache, although potentially in other areas as well), and “some type of seizure”. Later on, there is mention of deterioration of the “cranial nerve cluster”, bradycardia (a slow heart rate, likely since treatment was a stimulant and cardiostimulator), and toxins accumulating in lymphatic tissues caused by an altered leukocyte molecular structure. After turning off the implant, synthetic leukocytes are required to cure lingering effects (the aforementioned toxins and altered leukocytes). (*Clammy skin refers to cool, pale, and sweaty skin, so if one is looking at a purely canon view, this does mean that Cardassians sweat. However, the idea of ectothermic Cardassians that thus don't sweat is pretty common, so it's possible that one could interpret this as thus referring solely to cool and pale skin, or, since Bashir is basing his assessment on solely visual examination, just paleness, likely in the mucous membranes of the conjunctiva and gum tissue)
Let's break this down a little, starting by getting some of the nonsense out of the way. Endorphins (which are by definition natural) aren't produced by any 'pleasure centers' of the brain, they come from the pituitary gland; 'cranial nerve cluster' is essentially meaningless (although I suppose that the brain could be considered a nerve cluster); and, unlike the episode suggests, leukocytes aren't molecules – they're cells and thus made out of countless molecules. Moving onto breaking down terminology that has actual meaning, the postcentral gyrus is a part of the brain (roughly the middle top) that contains the primary somatosensory cortex, which helps the brain know where sensory signals are coming from, including pain. So, good job writers, you did mention a brain region associated with pain sensing! The central nervous system (CNS) is the brain and spinal cord. Endorphins are a type of what are called endogenous opioids, opioids produced by the body itself. They have more or less the same effects as other opioids like morphine, so that's probably the best starting point for research on side-effects and withdrawal symptoms for anyone who wants to look into that. Most relevant is that they're an analgesic (pain killer) and increase the production and release of dopamine, the “happy chemical”. Lymphatic tissues are just the tissues of the lymphatic system, which is kind of like an extra liquid collection system and aids in immune responses by producing, processing, and storing immune cells (and fat transport, but that's extraneous here). Leukocyte is a generic umbrella term equivalent to 'white blood cell' and thus contains a lot of different types of cells doing different things. Dr. Bashir really should have been more specific there.
That's what we get from canon, so from here on out it's pure headcanoning based on science. Let's tackle the first part: how does the implant work in the first place?
If the implant's purpose is to make one immune to pain, it needs to accomplish two main things: first, detect when pain is happening, and second, do something to stop that pain from being sensed. We're going to need to cover some basic nociception (pain sensing) here. The first step in pain detection is that a neuron called a nociceptor detects something pain causing, namely temperature (fun fact: spicy foods feel hot because some chemicals in them activate heat pain nociceptors in the mouth), chemicals (particularly chemicals released by damaged cells), and getting damaged itself. The nociceptor then releases a neurotransmitter (chemical that neurons use to communicate with each other) called Substance P. Substance P is then picked up by a secondary neuron in the spinal cord that sends the message of pain up to various parts of the brain that spread the message around and eventually cause effects, include the actual sensing of the pain, telling the brain where the pain is (that's where the primary somatosensory cortex and postcentral gyrus come in), and inducing the fight-or-flight response. One part of the brain that gets the 'we're hurting!' signal and sends a message that eventually results in the release of endogenous opioids like endorphins, which in turn prevent Substance P from being released, thus cutting off the pain signal, as well as do the other opioid things.
To accomplish the first task of detecting pain, the implant most probably has sensors for Substance P. These would probably be those filaments connecting to the entire CNS – since the Substance P is released in the spinal cord, these sensing filaments kind of need to be covering it all to detect any incoming Substance P. The second task of cutting off that pain signal then functions simply by flooding the body with endorphins (and probably other endogenous opioids). These endorphins could be sourced in three ways: via some sort of storage within the implant, via the implant causing the body to produce more, or via the implant itself producing and releasing more. Having enough stored for constant release for multiple years seems unlikely, so we'll scratch off that possibility. Garak's dialogue does suggest that the implant causes the body itself to produce more endorphins, but I'm going to go with the third option because we're going to need truly excessive amounts of endorphins to fully kill off pain signals (and it'd likely be hard to get the body to produce that much) and because it makes it easier to explain what might go wrong.
And speaking of what might go wrong, let's now look at that. The first noticeable symptoms are pale/clammy skin, dyspnea, extreme pain, and miosis. Miosis is caused by opioids, and would thus be induced by the implant and extreme pain. The pale skin and dyspnea are consistent with the heart not pumping enough blood to all the tissues. Other related symptoms include fatigue and anxiety, which are consistent with Garak's behavior. This is also consistent with the later bradycardia. This sort of heart issue is generally associated with heart attacks and other failures of the heart muscles itself, but could be caused by anything interfering with the heart's function. Since heart rate is largely controlled by the CNS, disruptions to it (such as by, say, a malfunctioning cranial implant), could induce these symptoms. We later learn that there is also deterioration of neural tissue (or at least that's how I'm interpreting Garak's 'cranial nerve cluster' comment) and damage to the lymphatic system due to toxins caused by altered leukocytes. It seems logical that these toxins are also responsible for the neural tissue deterioration and the CNS effects on the heart.
The next obvious question then is what are these toxins? Here comes the most speculative bit: the toxins are an autoimmune response caused by the implant producing malformed endorphins that bind to and affect the receptors of mature cytotoxic T-cells and cause them to bind to Class I MHC molecules in the absence of foreign antigens and result in the destruction of healthy cells. That's a lot of terminology and concepts that I just threw out, so let's break all that down. Malformed endorphins would be just what they say on the tin – after working for so long, the implant starts making making mistakes when producing and releasing the endorphins. Cytotoxic T-cells are a subtype of a subtype of leukocytes that work by destroying any cells presenting the 'red flag' that they've been invaded: a bit of something foreign to the body held out by what are called Class I MHCs, which are found on all cells. They sometimes accidentally respond and bind to plain Class I MHCs, thus resulting in an autoimmune reaction wherein the immune system attacks the body it's supposed to be protecting. Normally, these malfunctioning cytotoxic T-cells are taken care of before they mature and actually go to work, but if mature T-cells are being modified (there's your 'altered molecular structure of leukocytes'), the main bulwark against this type of autoimmune attack is side-stepped. And thus we have a malfunctioning implant killing Garak, and being able to continue to do so even when deactivated – the modified cytotoxic T-cells are still there, doing damage, even if no more new ones are being created.
This brings us to the third and final part: aside form turning off the implant, how are all its ill effects stopped? The big issue here would be the modified cytotoxic T-cells still running around, killing cells. There is one other mechanism for stopping self-attacking T-cells: regulatory T-cells. In the proposed situation, the body just doesn't have enough circulating regulatory T-cells to take care of the modified cytotoxic T-cells. Thus, the synthesized Cardassian leukocytes are specifically Cardassian regulatory T-cells that then help get rid of all the dangerous cytotoxic T-cells, thus stopping the toxins they produce from continuing to damage and kill tissues and effectively curing Garak.
So that's a lot, covering the very complicated and not entirely understood areas of neurology and immunology, so there's an amount of oversimplification, and I wouldn't be surprised if I mixed up or missed something that completely topples this entire thing.
But the TLDR is: Garak's implant makes opioids, but then it malfunctions and starts to instead make toxins that cause Garak's body to attack itself. Turning off the implant stops the production of the toxins, but Garak's body is still attacking itself until the synthesized Cardassian leukocytes are administered and fix things.
#Star Trek#DS9#The Wire#Star Trek doesn't understand biology#Elim Garak#Garak#Cardassians#Trek biotech#long post#addiction#opioids#I should probably proofread this first but eh#Also there's the blood-brain barrier that would probably be involved here but we'll just say it's passed by for some reason#Tricorder log
35 notes
·
View notes
Note
wait i think i read about that case too and he was v brain damage but could blink to communicate yes or no and smile for photos etc but he was in a wheelchair and couldn't move at all or speak . it was sad his mom said she asked him if he still wanted to die and he blinked yes :( here's the link https://www.walesonline.co.uk/news/wales-news/my-son-left-brain-damaged-17448133 is this a special case or is it a common outcome for people who attempt to hang themselves but are found before they die?
Hello there!
With neuro cases, localization is vital. The outcome of the status of the patient post-incident would depend on 1) the duration the person's brain without oxygen, 2) the extent of the damage, 3) the cerebral arteries and areas that got damaged / infarcted.
For example, if the BA 1, 2, and 3 (postcentral gyrus) got infarcted, then the patient might have a problem with localization of touch, temperature, vibration, pain, sensory perception, coordinated orofacial movement, and motor learning.
For the guy in the article, it seems like most parts of his brain got damaged.
3 notes
·
View notes
Text
CEREBRUM
The cerebrum is a large part of the brain containing the cerebral cortex (of the two cerebral hemispheres), as well as several subcortical structures, including the hippocampus, basal ganglia, and olfactory bulb. In humans, the cerebrum is the uppermost region of the central nervous system. The telencephalon is the embryonic structure from which the cerebrum develops prenatally. In mammals, the…
View On WordPress
#central sulcus#CEREBRUM#competitive exams biology important questions#frontal lobe#ibps#occipital lobe#parietal lobe#postcentral gyrus#precentral gyrus#psc#rrb#SSC#temporal lobes#UGC
0 notes
Photo
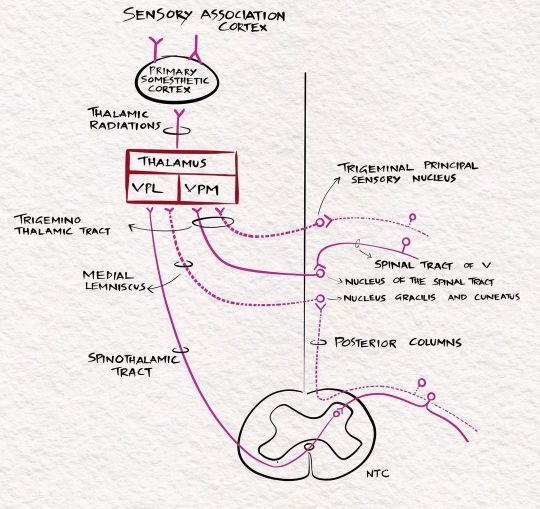
The sensory pathways. For full discussion of this image, follow 'Clinical neurology with KD' podcast episode 10 in Apple podcast, Spotify or Google podcast. Full notes are available on the neurologyteachingclub.com website. The primary modalities of sensation, including touch, pain, temperature, JPS, and vibration, are recognised at the level of the opposite thalamus. So all these sensations are carried to the opposite thalamus by the sensory pathways. The fine touch, vibration and JPS ascend in the posterior column- medial lemniscus pathway, while the pain and temperature through the lateral spinothalamic tract. From the thalamus, they are then projected to the sensory cortex in the postcentral gyrus through the posterior limb of the internal capsule. The sensory cortex helps identify the cortical sensations, namely tactile localisation, two-point discrimination, graphesthesia, stereognosis and sensory attention. #clinicalneurologywithkd #neurologyteachingclub #neurologypodcast #NTC #neurology #neurosciences #neuro #clinicalneurology #medicine #clinicalmedicine #kdpodcast #mbbs #medicos #doctors #neuroanatomy #casediscussion #medicineresidents #residency #medschool #futureneurologist #neuroimages #NEET #finalmbbs #neetpg #neetsuperspeciality #neetmedicine #eanneurology #nejm #aanbrain #neuroimage https://www.instagram.com/p/CdI1u4oP9cY/?igshid=NGJjMDIxMWI=
#clinicalneurologywithkd#neurologyteachingclub#neurologypodcast#ntc#neurology#neurosciences#neuro#clinicalneurology#medicine#clinicalmedicine#kdpodcast#mbbs#medicos#doctors#neuroanatomy#casediscussion#medicineresidents#residency#medschool#futureneurologist#neuroimages#neet#finalmbbs#neetpg#neetsuperspeciality#neetmedicine#eanneurology#nejm#aanbrain#neuroimage
1 note
·
View note
Photo

Some specific regions (gyri) of the cerebral cortex, such as the precentral gyrus (primary motor cortex) and the postcentral gyrus (primary somatosensory cortex), demonstrate topographic organization. Thus, information from the contralateral hand and arm are localized laterally, the body is represented more medially, and the lower extremity is represented along the midline and over the edge into the paracentral lobule. The face and head are represented in far lateral regions of these gyri, just above the lateral fissure. https://www.instagram.com/p/CZUZuk7jqRN/?utm_medium=tumblr
0 notes
Text
Medical Log
It has now been three days since I discovered the scar tissue in @thebestparisyouhave cerebrum and cerebellum. I believe it was caused by the Akritirian clamp which Lt. Paris received two years ago on Akritiri.
The scar tissue, which began in the frontal lobe, has spread through the precentral and postcentral gyrus and into the parietal lob. This threatens to affect Lt. Paris’s motor and sensory functions, as well as threatens to effect changes in his personality.
Because it is scar tissue, it is going to be very difficult to remove without causing damage to Lt. Paris’s brain. Because of its location, and the way it has spread, removing it could cause permanent brain damage, including but not limited to permanent loss of vision, motor control impairment, and even paralysis.
I checked @musician-in-space‘s brain the first day after I discovered the issue in Lt. Paris’s. The scar tissue has remained localized--as I expected Lt. Paris’s to--and shows no signs of growing or affecting him.
I have run dozens of simulations, but each has thus far failed to enact the results I am looking for. While Lt. Paris has told me he does not want to risk the surgery, I have faith that I will figure out how to remove the scar tissue without leaving permanent damage. I only hope that such a result will change his mind.
/End log.
13 notes
·
View notes
Photo

// Navigation
The medial entorhinal cortex is a major hub in the brain’s circuitry for spatial navigation, representing the brain’s internal compass.
This signal is referenced with respect to the environment (geocentric/allocentric), not the body's axis (egocentric).
The entorhinal (inside-rhinal) cortex is part of the medial temporal lobe or hippocampal memory system and constitutes the major gateway/interface between the hippocampal formation and the neocortex. It is related to relational organization of memory.
Grid Cells are neurons in the entorhinal cortex that provide an organism with a graph of distance travelled. They fire in triangularly tessellating patterns; this can be intepreted as a hex-grid.
Head Direction Cells are neurons in the dorsal presubiculum that fire when an organism is facing a particular direction. Head direction cells in the anterodorsal thalamus anticipate the animal's future heading by about 25 msec. The subicular complex, containing the dorsal presubiculum (sometimes referred to as the postsubiculum), lies adjacent to the hippocampus.
The dorsal tegmental nuclei abundantly contain cells that are sensitive to the organism's angular head velocity. The lateral mammillary nuclei contains both angular head velocity and head direction cells. The head direction signal is projected to the anterodorsal thalamus → postsubiculum → entorhinal cortex → hippocampus. In this way directional heading information can be integrated with information about the organism's location in the hippocampus and entorhinal cortex.
The directional firing of entorhinal cells depends on signals from the head direction cells in the presubiculum.
The data are fed into Place Cells, neurons in the hippocampus that fire when an organism occupies a specific location within its environment – hence the hippocampus can be considered to contain our own internal, spatial map.
The hippocampus contains the map, the entorhinal cortex the compass.
Motor signals, whether proprioceptive feedback from muscles or efference copy signals from cortical motor systems, also play an important role in head direction signals.
// Movement
The motor cortex is an area of the frontal lobe located in the posterior precentral gyrus immediately anterior to the central sulcus.
The precentral gyrus (the motor strip) is the site of the primary motor cortex, and is a prominent structure on the surface of the posterior frontal lobe. There is a precise somatotopic representation of the different body parts in the primary motor cortex, with the leg area located medially (close to the midline), and the head and face area located laterally on the convex side of the cerebral hemisphere (cortical homunculus). The arm and hand motor area is the largest and occupies the part of precentral gyrus, located inbetween the leg and face area.
The anterior paracentral lobule is attached to the precentral and postcentral gyri and is often referred to as the supplementary motor area (SMA), located on the midline surface of the hemisphere anterior to the primary motor cortex. It is associated with preparation for movement, sensory guidance of movement, spatial guidance of reaching, direct control of proximal and trunk muscles, and coordination of the two sides of the body such as in bi-manual coordination.
The posterior parietal cortex is responsible for transforming multisensory information into motor commands, and to be responsible for some aspects of motor planning, in addition to many other non-motor functions.
The primary somatosensory cortex, especially the part called area 3a, which lies directly against the motor cortex, is sometimes considered to be functionally part of the motor control circuitry.
Other brain regions outside the cerebral cortex are also of great importance to motor function: Most notably the cerebellum, the basal ganglia, pedunculopontine nucleus and the red nucleus, as well as other subcortical motor nuclei.
8 notes
·
View notes
Text
Seizure part 3
Focal Seizures.
Okay cool cool cool, so focal seizures are known as partial seizures or partial focal seizures. With these seizures they happen in one hemisphere, or half, of the brain, in a specific cortex. Where they occur will determine what effects they have on the body, and how they are manifested. For example, an discharge of electrons (aka a seizure) in the medial aspect of the postcentral gyrus of the right side of the brain might cause a patient to have paresthesia (the weird tingly sensation) in the left leg. Remember that the left half of the brain controls the right half of the body and vice versa.
With focal seizures it is further divided into two types, simple and complex focal seizures.
With a simple focal seizure, the patient will remain conscious and alert, but might feel weird, or have unusual sensations. They might suddenly become really happy, sad, angry, etc. It might cause them to have new sensations that no one else is experiencing, might hear, smell, taste, see, or feel things that aren't actually there.
Opposite of this are complex focal seizures, wherein the patient will have a loss of consiousness or at least an alteration inhow aware they are of their surroundings, it might make them feel as if they are dreaming. It lasts anywehre from 30 seconds to 3 minutes and they go into a weird dreamlike setting, sometimes doing things that don't make sense, or doing nothing at all. Some things aren't too bad, smacking their lips, doing the same motion over and over again, but sometimes they might really fuck up and walk into traffic, take off their clothes, etc. After the seizure they will probably be tired and confused, and might not be able to return to their normal activities.
Here's where it gets even more annoying in terms of defining them. A focalized seizure starts at one side of the brain, but it doesn't have to stay there. It may actually spread to the entire brain which triggers a tonic clonic seizure. Like a shitty pokemon evolution. This is why it is hard to know which seizure is truly which. You might think your patient is having tonic-clonic (aka grand mal) seizures, but it is actually secondary (or coming after something, as a result of that something occuring) generalized seizure. There is a difference here though between the two. If a patient has Todd's Paralysis, which is a residual neurologic deficit that eventually goes away after the tonic clonic seizure, then it is actually a focal seizure that generalized secondarily, and not just a tonic clonic seizure. It's all convuluted and confusing, hence me stating earlier not to actually care too much about the differences.
There is however one seizure that I will point out due to it being unique and rather sad. Psychogenic Seizures. These seizures may resemble an epileptic seizure, and might even be diagnosed as one at first, however when EEG monitoring is done it is revealed that the brain is actually fine. Basically, the patient isn't actually having an epileptic seizure, there is no abnormal misfiring of neurons in the brain, yet they are suffering as if it were. It is actually due to some emotional or physical abuse that the brain can't deal with so it freaks out and decides that a seizure is a reasonable response. But it is actually fine.
0 notes
Text
In reality, it would depend on which part of the brain is stabbed. There are several factors to consider; does the killer intend to get rid of this person quickly, or keep them around for whatever reason? What type of weapon is the killer using? Stuff like that.
But, as every part of the brain is responsible for a different function, we can assume that those functions being damaged in such a physical way would cause specific problems for the victim. (In other words, just use your imagination 😈)
Brain stem: breathing, blood pressure, swallowing, heart rhythms, balance, facial sensations.
Cerebellum: coordination, balance, posture, motor-learning/sequence-learning, reflex memory.
Temporal lobe: auditory information, facial/object recognition, perception, and memory encoding.
Occipital lobes: visual perception, distance/depth perception, color determination, memory formation, visuospatial processing.
Parietal lobe: primary senses (taste, touch, hearing, smell, sight), space navigation, language processing.
Postcentral gyrus: sensation perception (pressure, temperature, pain, etc.)
Central sulcus: organizes all sensory and motor functions.
Precentral gyrus: controls all voluntary motor movements.
Frontal lobe: memory, emotions, impulse-control, expressive language, problem-solving, social interaction, and motor function
Sylvian fissure: sole passageway for the middle cerebral artery; helps maintain bloodflow throughout the brain.
I wonder
What is it actually like to get stabbed in the brain??
Do we go into shock? Or does our brain immediately shut down because of the damage?
Does our brain still respond with pain despite the damage dealt? Does our brain send out signals to the body despite bleeding out?
Do we freeze up in shock then collapse? Do we fall to the ground and bleed out faster because the brain is one of the organs thats needs blood the most?
Do we loose oxygen faster than we loose blood?
18 notes
·
View notes