#optically anisotropic crystal
Explore tagged Tumblr posts
Text
Pleochroism in gem quality zoisite var. tanzanite
Apparently we're doing this...
*my pictures, not my crystal
Ever wondered why the colour of some gemstones seems to shift as you view them from different angles? Perhaps are you were looking at a pleochroic gemstone!
Pleochroism is a body colour effect seen in transparent to translucent, optically anisotropic* crystalline material caused by differential absorption of the polarised light rays following different paths through the gemstone, and causing a change to perceived body colour when the stone is viewed from different directions.
...Okay, but what does that mean?
Pleochroism means 'many coloured'. When light passes through a crystal, it is sometimes split into two rays - each ray takes a slightly different path, and because it encounters different atoms within the crystal structure, it is modified to a different colour.
When both different coloured rays of light exit the crystal, your eye resolves them as a single colour. Clever, right? :D

The four photos above are of the same crystal. The top photos show the crystal from two different angles - one looks distinctly more purplish than the other, right? That is eye-visible pleochroism!
Even gems that show pleochroism don't always show it strongly enough to see with the naked eye. But this tanzanite crystal does.
The bottom photos show the same crystals viewed through a type of polarising filter called a dichroscope. The dichroscope is made of polarising film where each half lets through only one of the rays of coloured light - meaning you can see each of the two colours at the same time, one in each half of the filter!
But wait!- I hear you cry. Why are there THREE different colours??
Hmm well this is getting into a deeper discussion of crystal habits and their optical behaviours, so for now let's say that depending on the symmetry of the crystal, an optically anisotropic* stone can show two or three colours - but only ever two in one direction! You have to turn the crystal to see the third colour.
If a stone shows two colours we say it is dichroic, if it shows three it is trichroic. This is the maximum number of colours you will see.
When you view the tanzanite crystal in the position in the left photo, the light rays being transmitted are blue and reddish-purple. Your eyes resolve this to show a purpley-blue colour. Neat!
But in the position from the right photo, it's transmitting blue and yellow. Wild! The yellow ray dilutes some of the intensity of the blue, so the colour you see is a weaker blue tone.
The photo I'm missing from my set is the crystal viewed from top-down, which would then show a purple and yellow split through the dichroscope!
So turning the stone, or moving your head position relative to the position of the stone, really does change its colour, because you are perceiving a different set of coloured light rays being transmitted through the stone!
When fashioning a rough crystal, a lapidary (that's a stone cutter) would to orient the crystal so the best colour is face-up - ie. when you look at the finished polished stone, you will see the most attractive balance of colour. What is considered ideal varies with the stone - you might cut a very dark crystal to show its lighter colour, or a pale one to show the most intense colour it is able to!
Fun additional fact - tanzanite can be heated to improve its colour. It does this by removing the yellow element entirely, meaning you will see intense bluey-purple tones from all angles! The wonders of stone treatments!
*Edit I am misleading you by saying you will see bluey-purple in all directions... in two directions (as tanzanite is a biaxial gemstone) you will find an optic axis, which is a direction of optical isotropy in an otherwise optically anisotropic* gemstone. So in this direction, you will only have a single transmitted ray of light, not two!
*I use the phrase optically anisotropic a couple of times and it's hard to explain without a whole separate citizen lecture on crystallography, but the quick summary is that it means a crystal in which light behaves differently as it travels in different directions - such as the differential absorption resulting in different colours in pleochroism! This is as opposed to an optically isotropic crystal, where light behaves the same way in all directions of travel.
And that is a recited-from-memory summary of pleochroism! :D (please don't tell me off if I got any of it wrong I didn't go upstairs to check my notes ;_;) (I mean I didn't get any of it wrong but my quoted definition probably wasn't word perfect ^^;)
@royallykt thank you so much for your interest i hope you enjoyed learning about all this :)
#in my tech era#infodumping#gemmology#pleochroism#tanzanite#zoisite#optically anisotropic crystal#well it didn't get my fic written#but i did enjoy it :)#you say that#i actually cracked the scene that had been troubling me yesterday#so clearly i could write about gemmology#as a little treat#can provide more photos of pleochroic stones under the dichroscope if desired#tourmaline tends to be a good one#i'll have a synthetic ruby somewhere which shows a orangey-red/purpley-red split#and i have an iolite which shows brilliant diagnostic trichroism#anyway#^^;#hope you enjoyed!#i had another go at uploading the video and this time it worked! yaay!
14 notes
·
View notes
Text
Israeli air strike in Gaza kills prominent scientist Sufyan Tayeh, Palestinian ministry says
Reuters
Dec 2 An Israeli air strike targeting the neighborhood of Al-Faluja, 30 km (18 miles) northeast of Gaza City, has killed prominent Palestinian scientist Sufyan Tayeh and his family, the Palestinian Higher Education ministry announced on Saturday. Tayeh, who was president of the Islamic University of Gaza, was a leading researcher in physics and applied mathematics.
In 2005, he was arrested by Israeli occupation forces at the Rafah border crossing while he was heading to Egypt to complete the procedures for submitting his PhD dissertation at Ain Shams University. Over the period from 2008 to 2011, he assumed the position of Head of Physics Department at the Islamic University and he was appointed President of the University in August 2023.
Academic activity One of Dr. Tayeh’s research works was a proposal for scientific research in the field of optical sensors submitted to the University of Montreal, Canada. With the participation of Professor Ahmed Hamdan, Dr. Tayeh presented a research study entitled “Sensitivity enhancement in optical waveguide sensors”. The research paper aimed to explore multi-layer waveguide structures (more than 3 layers) for the purpose of optical biosensing, and to study the effect of anisotropic materials on the sensitivity of plate waveguide structures, in addition to studying more photonic crystals (two- and three-layer) with different compositions as well as the number of biosensing various layers.
Awards and honors Dr. Tayeh was winner of the Palestine Islamic Bank Award for Scientific Research for years 2019 and 2020. In March 2023, he was appointed holder of the UNESCO Chair for Physical, Astrophysical and Space Sciences in Palestine. He was recipient of the Abdul Hameed Shoman Award for Young Arab Scientists; and the winner of the Islamic University Award for Scientific Research for the year 2021. He was also ranked among the top 2% of researchers around the world in 2021. source
More than 1300 scientists from 40 countries, including physicists, mathematicians, biologists, chemists, medical doctors, engineers, and social scientists, working in academia and in industry, are compelled by the events in Gaza to call for an immediate permanent ceasefire and take a stand against war and the destructive use of science in an “International Convention Against War and Destructive Use of Science: Scientists Against Israeli Apartheid and Genocide in Gaza” on 9th December 2023. Prof. Richard A Falk, Emeritus Professor of International Law at Princeton University and Former UN Special Rapporteur for Palestine, was the keynote speaker in the Convention. He spoke ‘On the situation in Gaza.’ The Convention was moderated by Dr. Manabendra Nath Bera, a quantum information scientist from India. Dr. Flavio del Santo, a scientist from Switzerland, Dr. Niatalya Dinat, a medical doctor from South Africa, Prof. Josh Dubnau, from Sony Brook University, USA, and Prof. Assaf Kfoury, Boston University, USA, discussed possible amendments proposed by participants on the draft of the Declaration by the scientists. In the end, the amended Declaration was adopted after voting, with an absolute majority (97%).
The adopted Declaration embodies scientists’ protest against the destructive use and militarisation of science, Israeli occupation, apartheid, and genocide in Gaza, and call for an academic and scientific boycott and to stand by the Palestinian professors, scientists, researchers, scholars, and students.
8 notes
·
View notes
Text

Sapphire #sri Lanka
General
Category Oxide mineral
Formula(repeating unit)
Aluminium oxide, Al2O3
Crystal system
Trigonal
Crystal class
Hexagonal scalenohedral (3m)
H-M symbol: (32/m)
Space group
R3c
Identification
Color
Typically blue, but varies
Crystal habit
As crystals, massive and granular
Twinning
Both growth twins (in various orientations) and polysynthetic glide twinning on the rhombohedron [1011
Cleavage
Poor
Fracture
Conchoidal, splintery
Mohs scale hardness
9.0
Luster
Vitreous
Streak
Colorless
Diaphaneity
Transparent to nearly opaque
Specific gravity
3.98–4.06
Optical properties
Abbe number 72.2
Refractive index
nω = 1.768–1.772
nε = 1.760–1.763,
birefringence 0.008
Pleochroism
Strong
Melting point
2,030–2,050 °C
Fusibility
Infusible
Solubility
Insoluble
Other characteristics
Coefficient of thermal expansion (5.0–6.6)×10−6/K[citation needed]
relative permittivity at 20 °C
ε = 8.9–11.1 (anisotropic)
2 notes
·
View notes
Text
What have I been up to? Well more or less more optics.
I started to learn about the Index Ellipsoid, and I am beginning to understand how we can quantify a materials allowed polarizations using the "indicatrix" (not going to lie this is a hard word to spell lol)
I now understand that there are two possible electric flux densities (basically the quadratic equations +/- gives two roots, so when you solve for two possible refractive indices, you also get two D solutions) and that these two fluxes are orthogonal.
The index Ellipsoid let's us characterize anisotropic crystals into biaxial (two defined optical axes) and uniaxial (two axes are equal)
But honestly, I'm hitting a bit of a wall here. I just want to be familiar with the material before grad school starts. Since I'm self studying now, I decided to venture off into a related topic: Computational electromagnetics!!
I took numerical methods this winter so when I heard about Finite Difference Method I didn't look like a 🦌 staring at 🚗 lol (honestly I find Runge-Kutta methods scarier haha)
So I learned a bit about collocated grids, recalled Dirichlet boundary conditions, periodic boundary conditions as well as Neuman boundary conditions.
My plan is to use COMSOL multi physics to do a little simulation for my masters thesis, so I'm betting that having a grasp on the numerical side of emag is something I need to accomplish to bear fruitful results.
And of course I get hit with the realization exactly *why* plane waves are used to teach us optics: because they are simply a stepping stone towards us learning about Gaussian Beams!
The 🐇 hole just keeps getting deeper and deeper... 🕳️
#anisotropy#optics#physics#math#electromagnetics#gaussian beam#boundary conditions#Neumann#Dirichlet#COMSOL multiphysics#numerical electromagnetics#Finite Difference Methods#SoundCloud
0 notes
Text
Global Top 7 Companies Accounted for 83% of total Anisotropic Conductive Film market (QYResearch, 2021)
Anisotropic conductive film (ACF) is a lead-free and environmentally friendly adhesive interconnect system that is commonly used in liquid crystal display manufacturing to make the electrical and mechanical connections from the driver electronics to the glass substrates of the LCD.
Anisotropic conductive adhesives are prepared in two forms: films and pastes. In the film form, Anisotropic conductive adhesives are called Anisotropic conductive film (ACF), while they are known as anisotropic conductive pest (ACP) in the paste form. This report studies the Anisotropic conductive film (ACF) market, from angles of Company, regions, product types and end industries, to analyze the status and the future.
According to the new market research report “Global Anisotropic Conductive Film Market Report 2023-2029”, published by QYResearch, the global Anisotropic Conductive Film market size is projected to reach USD 0.8 billion by 2029, at a CAGR of 4.8% during the forecast period.
Figure. Global Anisotropic Conductive Film Market Size (US$ Million), 2018-2029
Figure. Global Anisotropic Conductive Film Top 7 Players Ranking and Market Share(Based on data of 2021, Continually updated)
The global key manufacturers of Anisotropic Conductive Film include Showa Denko Materials, Dexerials, etc. In 2021, the global top three players had a share approximately 83.0% in terms of revenue.
About QYResearch
QYResearch founded in California, USA in 2007.It is a leading global market research and consulting company. With over 16 years’ experience and professional research team in various cities over the world QY Research focuses on management consulting, database and seminar services, IPO consulting, industry chain research and customized research to help our clients in providing non-linear revenue model and make them successful. We are globally recognized for our expansive portfolio of services, good corporate citizenship, and our strong commitment to sustainability. Up to now, we have cooperated with more than 60,000 clients across five continents. Let’s work closely with you and build a bold and better future.
QYResearch is a world-renowned large-scale consulting company. The industry covers various high-tech industry chain market segments, spanning the semiconductor industry chain (semiconductor equipment and parts, semiconductor materials, ICs, Foundry, packaging and testing, discrete devices, sensors, optoelectronic devices), photovoltaic industry chain (equipment, cells, modules, auxiliary material brackets, inverters, power station terminals), new energy automobile industry chain (batteries and materials, auto parts, batteries, motors, electronic control, automotive semiconductors, etc.), communication industry chain (communication system equipment, terminal equipment, electronic components, RF front-end, optical modules, 4G/5G/6G, broadband, IoT, digital economy, AI), advanced materials industry Chain (metal materials, polymer materials, ceramic materials, nano materials, etc.), machinery manufacturing industry chain (CNC machine tools, construction machinery, electrical machinery, 3C automation, industrial robots, lasers, industrial control, drones), food, beverages and pharmaceuticals, medical equipment, agriculture, etc.
0 notes
Text
Birefringent Crystals
Birefringence is an optical property exhibited by materials whose refractive index varies with the polarization and direction of light propagation. These optically anisotropic crystal materials are said to be birefringent crystals. OST Photonics offers several birefringent crystals such as YVO4 and a-BBO crystals, etc.
What Are the Applications of Birefringent Crystal?
Birefringent crystal is an important photoelectric functional crystal material, which is widely used in the field of optical polarizer, optical modulation and nonlinear optical technology.
FAQS about Birefringent Crystals
What Is Birefringence?
When a beam of light strikes an interface of crystal, it typically generates two refracted beams, which is known as the phenomenon of birefringence.
Which Crystals Have Birefringence?
Calcite(CaCO3), YVO4, Alpha-BBO, Quartz, MgF2 and LiNbO3 crystals exhibit birefringence.
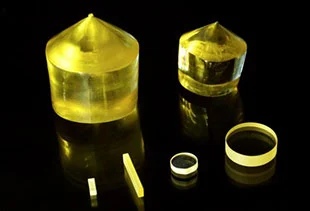
1 note
·
View note
Photo

Facet controllable synthesis of two-dimensional rare earth oxides
Since graphene was awarded the Nobel Prize in 2010, two-dimensional (2D) materials have continued to attract researchers' attention in logic, storage, optoelectronic and photonic 2D device manufacturing fields because of the atomic thickness and excellent performance. Based on the research of graphene, scientists have discovered some other 2D materials, such as layered transition metal dichalcogenides (TMDs), hexagonal boron nitride (h-BN), and non-layered III–V group semiconductors.
Recently, 2D rare earth oxides (REOs) have arisen as a unique and promising non-layered material family. The unfilled 4f orbitals of rare earth elements are shielded by the fully filled outer shell, thus the unpaired 4f electrons of rare earth ions are typically not involved in chemical reactions, leading to promising properties in luminescence, magnetic, electronics and catalytic activities. 2D REOs combine the unique properties of rare earth elements and have been widely used in optics, magnetism, high-efficiency catalysts, transistors, biomedicine and other fields.
Besides, it is reported that the crystal facet also has a certain influence on the properties of the 2D materials. Therefore, it is very significant to controllably synthesize 2D materials with specific facets. However, due to its non-layered structure, it is challenging to control the 2D anisotropic growth of the material. Moreover, since 2D materials will expose the most stable facet with the lowest energy, it is particularly important to control the thermodynamics of the materials.
Read more.
18 notes
·
View notes
Text
Special optical fibers: the overview
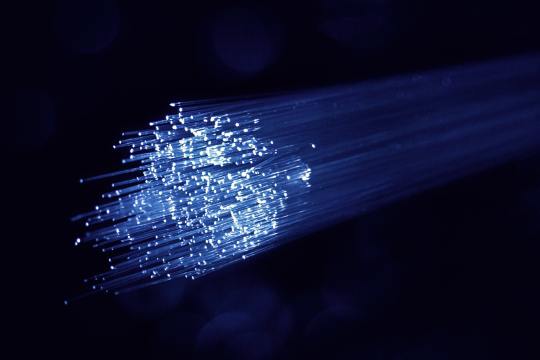
At present, optical fibers are widely used not only in fiber-optic data transmission lines but also in various fiber optic cables and sensors of physical quantities and other fiber-based devices. The specifics of this application require the creation of optical fibers with special properties.
The main purpose of special optical fibers is to perform various operations with light signals (amplification, modulation, filtration, etc.), as well as the operation of fibers in special modes and conditions (for example, under high mechanical loads – shock or
static, high temperature, radiation, humidity, UV, average IR, and far-IR ranges), so the requirements for optical losses in such fibers fade into the background.
The typical length of special optical fibers is not kilometers, as, in the case of long-distance fiber cables, it achieves from one to several tens of meters. Today, manufacturers of fiber optic solutions note a growing interest in specialized fibers for use in optical components.
For example, global consumption of special optical fibers in 2007 amounted to more than $ 1.2 billion. Many manufacturers of special optical fibers are expanding their customers in the field of biomedicine, aviation, input/output, and military industries. Other manufacturers see more opportunities for using special fiber optic cables in sensors and fiber optic gyroscopes.
Nevertheless, the use of special optical fibers in communication systems has made more significant progress and promises many new opportunities. It is already clear that in any case of further development, special fiber cables will be used in the equipment of next-generation communication networks.
Currently, there are about twenty types of special optical fibers that differ in their design characteristics and basic properties. The following basic information about some of the widely used special optical fibers is provided based on the most important areas of their application in communications.
Optical fiber for lasers and amplifiers
Ytterbium fiber with a double-clad is used in high-power radiation sources and amplifiers. These fiber optic cables are designed to meet the requirements for high-power amplifiers, industrial and military lasers, and infrared sources.
The optical fibers are specifically designed to effectively combine a single-mode signal and high pumping power from a multimode diode into a passive double-clad fiber. The combination of low-cost, high-output multimode diodes with these fibers allows for easily achieving multi-watt power levels with an effective ratio of electrical power to optical power.
These fiber cables have a multimode core that corresponds in size to the diameter of the inner clad of the ytterbium fiber used as an active element for fiber lasers and amplifiers. They are used to transfer radiation energy from the optical pump source of a fiber laser (or amplifier) to its active element and deliver laser output radiation for various applications.
Optical fibers for optical multiplexers and demultiplexers
Optical multiplexers and demultiplexers of an input/output are typically created with the use of photosensitive fibers. The ability of an optical fiber to change the refractive index of the core under the influence of light is called the fiber's photosensitivity.
Photosensitive fibers are used to create fiber Bragg gratings, which are the main component of radiation input-output multiplexers and demultiplexers. A fiber Bragg grating is an optical fiber with a periodic change in the refractive index along with its core.
By irradiating a photosensitive fiber with a laser beam through a phase mask, a fiber Bragg grating can be created. The main property of this grating is the reflection of light propagating through the fiber in a narrow band that is centered around the Bragg wavelength.
Optical fibers for modulators
There are two types of optical waveguide modulators: planar and fiber. Both types are most often phase modulators. Thus, both polarizing fibers and conventional optical fibers are used in these modulators.
Optical fibers for filters
Currently, there are numerous types of optical fiber filters: filters on diffraction or Bragg gratings, Fabry–Perot and Mach–Zander filters, etc.
For example, a Bragg filter is a photosensitive optical fiber with a Bragg grating formed on part of it. If you change (control) the period of the FBG filter, it becomes a tunable filter. The grating period can be changed by heating or mechanical stresses.
Optical fibers for dispersion compensation
Dispersion compensation can be performed using several methods. For example, special fiber cables or dispersion-compensating modules can be used.
These fiber optic cables have a large negative dispersion, as well as a negative slope of the dispersion curve. A wide range of operations can be performed using fibers that compensate for dispersion. The second example of dispersion compensation is fiber Bragg gratings with a variable period.
Optical fibers for supercontinuum sources
Photonic crystal fibers are a special example of special optical fibers. Thanks to the appearance of a series of unique properties, they are used not only in optical communication, but also in high-power transmission, sensitive sensors, non-linear devices, and other areas.
Manipulating the type of grating, its step, the shape of the air channels, and the refractive index of the glass allows for obtaining properties that do not exist in conventional fiber optic cables. For example, nonlinear properties make photon-crystal fibers capable of generating a supercontinuum, i.e. converting light of a certain wavelength into light with longer and shorter waves. Thus, it is possible to create broadband light sources based on new principles.
Fiber optic amplifiers
It is known that the optical signal is attenuated by 10-20 dB at every 50-100 km of fiber optic cables. This fact requires compensation. Previously, the only way to compensate for losses in the line was the use of regenerators in the existing communication lines.
Currently, three types of optical amplifiers have been developed for fiber optic systems: semiconductor optical amplifiers, fiber amplifiers based on rare-earth ions (for example, erbium), and Raman fiber amplifiers.
The most widespread use is currently found in optical fiber amplifiers. The current level of technology development allows for employing various impurities into quartz fiber, in particular, rare earth elements. Erbium optical fiber amplifiers are the most common at present.
Advantages of erbium fiber amplifiers include:
– high energy transfer from the pump to signal > 50 %;
- simultaneous amplification over a wide range of wavelengths, i.e. they are suitable for WDM systems;
- output limit greater than 10-25 dB / m;
– the gain time constant is large enough for overcoming modulation interference;
- low noise factor;
– polarization independence (which reduces loss);
- the opportunity to use these optical fibers in remote systems;
- the erbium amplifier can also operate in the S and L ranges.
The disadvantages of erbium fiber amplifiers include:
- large dimensions of the erbium amplifier module;
- the inability to integrate with semiconductor devices;
- amplified spontaneous emission (ASE);
– crosstalk;
- gain limit.
Raman fiber amplifier
Raman amplifiers are promising for use in fiber optic systems due to their following fundamental advantages: they can amplify at any wavelength; the fiber light guide itself can be used as the active medium of Raman amplifiers; the gain spectrum of these amplifiers depends on the pump spectrum (wavelength), so the selection of pump sources can form a very wide (more than 100 nm) gain band; Raman amplifiers have a low noise level.
The main disadvantage of Raman amplifiers is their low conversion efficiency, which requires the use of a fairly powerful continuous pump radiation to obtain the typical signal gain of 30 dB for fiber optic systems.
Double-clad activated optical fibers
An appropriate pump is required for any laser to work. In particular, fiber lasers use optical fiber pumping. It is proposed to use double-clad optical fibers to increase the output power of fiber lasers and simplify the input of radiation from semiconductor laser diodes into the fiber light guide.
Photonic crystal activated fibers
Recently, photonic crystal fiber-based lasers have been rapidly developed. Photonic crystal waveguides and optical fibers are a new type of waveguides. Their appearance is associated with the creation and research of new fiber optic systems – photonic crystals. They have the following distinctive features in comparison to conventional fibers:
- high numerical aperture;
- large core diameter, which can support the single-mode operation. As a result, high pumping powers and generation without noticeable heating can be realized in photonic crystal fibers;
- the absence of non-linear effects;
- high anisotropy of the optical fiber structure, allowing transmission of radiation with a high degree of polarization.
Anisotropic single-mode fiber cables
Along with the long-distance lines, fiber optic cables are widely used in a wide variety of measurement, diagnostic, and highly sensitive monitoring and control systems. Anisotropic single-mode optical fibers promote the development of sensors for measuring various physical quantities and such unique devices as fiber optic gyroscopes.
Many manufacturers of special optical fibers are expanding their customers in the field of biomedicine, aviation, and military industries. Other manufacturers see more opportunities for using special fiber optic cables in sensors and fiber optic gyroscopes. Nevertheless, the use of special optical fibers in communication systems has made more significant progress and promises many new opportunities. It is already clear that in any case of further development, special fiber cables will be used in the equipment of next-generation communication networks. If you would like to obtain an optical fiber product, you should choose the Optromix company. Optromix is a provider of top quality special fibers and broad spectra optical fiber solutions. The company delivers the best quality special fibers and fiber optic cables, fiber optic bundles, spectroscopy fiber optic probes, probe couplers, and accessories for process spectroscopy to clients. If you have any questions or would like to buy an optical fiber, please contact us at [email protected]
1 note
·
View note
Link
The human body is an exquisite specimen of an intricate, functioning quantum mechanism that has evolved successful interfaces to decode and utilize quantum inputs from the environment. But what happens when the primary interface is challenged by a sudden change in quantum inputs?
Vitamin C is probably one of the most well-known nutrients. Abundantly found in fruits and most vegetables, vitamin C confer myriads of health benefits, the most famous being its antioxidant, free radical scavenging attributes. Today, I will take you on a unique journey to discover the real role ascorbic acid plays in our bodies at the quantum level.
Ascorbate is Ubiquitous in all Eukaryotes
Ascorbate, also referred to as L-ascorbic acid or vitamin C, is essential for all eukaryoticalgae, plants and animals, but not for prokaryotic bacteria. All photosynthetic plants synthesize ascorbate, while some animals, including primates, cannot synthesize ascorbate due to the loss of a terminal enzyme in the GULO pathway that is responsible for the conversion of ascorbate from glucose. [1]
In the beginning, all eukaryotes used the GULO (l-gulonolactone oxidase) pathway for ascorbate biosynthesis. As time passed, photosynthetic organisms whose biological activities are solely dependent upon light for the conversion of light energy into chemical energy, evolved a more efficient GLDH (l-galactonolactone dehydrogenase) pathway that uncoupled hydrogen peroxide generation during ascorbate biosynthesis. [2] Ascorbate auxotrophs, unable to synthesize ascorbic acid, must obtain this essential nutrient from their diet. Primates and guinea pigs get their ascorbate from land plants; bats’ sources are land plants, insects and blood; whereas marine animals like teleost fish and crustaceans obtain their ascorbate from zooplankton and phytoplanktons. [1]
There are many speculations as to why ascorbate auxotrophs lost the ability to synthesize ascorbate. Some believed the successful evolution of the GLDH pathway in photosynthetic plants provided ample and constant supply of ascorbate. Primates in the wild that are unable to synthesize ascorbates consume high amounts of ascorbate. For example, gorillas (Gorilla gorilla) consume 20-30 mg/kg/day, howler monkeys (Alouatta palliata) consume 88 mg/kg/day, and spider monkeys (Ateles geoffroyi) consume 106 mg/kg/day. At that amount, it is equivalent to 8.46 g for a human weighing 80 kg! [27]. Others believed that a reduction in the "cost" of production, which is the generation of the reactive oxygen species, H2O2, balanced out the lost of production capability. [3] I believe there is another reason why a required nutrient in humans is not synthesized by our bodies because the role of ascorbate extends far beyond its redox capacities. It is also the same reason why prokaryotes do not synthesize ascorbate. Light.
Prokaryotes are Light Emitters
All living organisms release biophotons. Humans, animals and plants emit ultra-weak biophotons at intensities between 10 to 100 photons/cm2 /sec wavelengths between 200 nm and 800 nm. Prokaryotic bacteria emit over 1000 photons/cm2 /sec. The intensity is dramatically increased during their growth phase. It is well known that bacteria use quorum sensing communication signals to regulate their physiological activities, including symbiosis, virulence, conjugation, motility, sporulation, and biofilm formation. [4] One of these communication signals used by quorum sensing microbes is electromagnetic radiation in the visible (400–750 nm) and near-infrared (750–2500 nm) regions. [5] So what happens when you add ascorbate to quorum sensing bacteria like Escherichia coli or Klebsiella pneumoniae that uses light for cell to cell communication? Death is the result. [6] The ability of ascorbate to inhibit growth, sporulation and enterotoxin production is due to its anti-quorum sensing ability. [7] How does ascorbate disrupt quorum sensing?
Birefringence, Depolarization and Quantum Decoherence
Vitamin C, or ascorbate, is a birefringent molecule that is optically active.[7, 8] The word birefringence comes from the Latin ‘bi’ meaning ‘twice’, and ‘refringere’ meaning ‘to break up’. Birefringent and optically active materials are anisotropic, where the index of refraction varies with polarization direction. This quality is the exact opposite of isotropic materials like glass, liquids and amorphous mediums where direction of polarization does not matter. When a ray of light enters a birefringent material like calcium carbonate or ascorbate, it will be broken up into two rays going in different directions and velocities upon exit. That is why when you look through a birefringent crystal, you will see double. [9] After light passes through a birefringent medium, it will also be depolarized, meaning there is a reduction or even a complete loss of polarization. [10]
Can Light from the Sun be Depolarized?
We all know light from the sun is unpolarized. What exactly does the term ‘unpolarized’ mean?
The atoms on the surface of a heated surface generates light and these atoms act independently of one another. The electromagnetic waves emanating from the heated surface will each have its own polarization direction. The sum of these random orientations result in a wave whose direction of polarization changes rapidly and randomly. Such a wave is said to be unpolarized. Common sources of light, including the sun, incandescent, fluorescent lights, LEDs (Light Emitting Diodes), and flames, all produce unpolarized light. However, these unpolarized light often become partially polarized due to multiple scatterings and reflections. When sunlight passes through the Earth’s atmosphere, it is scattered by air molecules, rendering the light that reaches the ground partially linearly polarized. The reason the sky looks blue is because the blue spectrum being at a higher frequency, is scattered more by air molecules. [11] So one can say that due to the scattering effects, all light that passes through a birefringent medium will be depolarized upon exit to a certain extent.
Photons & Quantum Decoherence
Photons carry information. How much information is carried depends on whether you believe in a holographic, fractal universe.
Quantum information is commonly encoded in the polarization of single photons. When light passes through birefringent mediums, the depolarization of the photons is equivalent to the decoherence of the quantum information they encode. Depolarization of such photons acts as quantum noise on the stored information. [12] Quantum decoherence has been proven to be equivalent to the classical depolarization experienced by light. [13] Decoherence is regarded as a loss of information into the environment, [14]and decoherence can also degrade or terminate entanglement. [15]
Is it becoming clearer to you why quorum sensing prokaryotic bacteria do not synthesize ascorbate? Ascorbate is birefringent. The light used by bacteria for cell to cell communication will be depolarized and rendered decoherent by ascorbate. That is how ascorbate disrupts quorum sensing in prokaryotic bacteria. [7] How important is electromagnetic radiation (EMR) depolarization in the human body? I believe EMR depolarization is critical for maintaining optimum health, especially when you consider the fact that 33% of all the protein in the human body is collagen, and collagen is also birefringent!
Birefringence & Depolarization are Tissue Health Indicators
As early as 1975, birefringence or the ability to depolarize light, was already used to differentiate the state of health of underlying tissues being examined. In general, healthy tissues are more birefringent, whereas diseased or necrotic tissues display little to no birefringence, depending on the nature and degree of damage. When tendons were stressed in forced training exercises, the birefringence and the resistance to tear were both decreased. As tendons became completely detached due to stress, birefringence was completely lost. [16] Tissue birefringence is dependent on collagen fiber organisation and orientation. In osteoarthritis, the loss of birefringence is linked with the early stages of cartilage degeneration. The greater degree of loss in birefringence, the greater the increase in cartilage degeneration. [37]
Today, information about tissue structure can be extracted from how light is depolarized as it passes through the tissue being examined. The pathology of a tissue is correlated to the decrease of birefringence in the tissue. For example, myocardial infarction results in a decrease in the birefringence signals generated in the area of infarction, due to disorganization of collagen fibers formed in scar tissues [17]. This decrease in birefringence in unhealthy, damaged tissues, and the subsequent reduction in depolarization of light, can also be detected as an increase in biophoton emissions. In plants, unhealthy or injured areas display higher biophoton emission than healthy, uninjured areas. [18] In humans, cancerous cells emitted higher intensity of photons than non-cancerous cells mostly in the ultraviolet and blue wavelengths (370 nm, 420 nm, 500 nm), whereas non-cancerous, healthy cells emitted more biophotons than cancer cells in the infrared range (620 nm, 950 nm). [19]
Collagen Birefringence and Infrared Light
Healthy non-cancerous cells emit more biophotons in the infrared frequencies, yet contrary to unhealthy, necrotic cells that displayed reduced birefringence, these healthy tissues still exhibit strong birefringence and large degrees of depolarization. Why do healthy cells emit infrared range biophotons and still remain birefringent? Because infrared light has been proven and demonstrated to induce birefringence [20], and higher birefringence is associated with healthy tissues. In the regeneration of third degree burns in the skin of mice, areas treated with 632.8 nm low intensity laser showed higher birefringence than non-treated control areas in the same subjects. [21] The degree of depolarization is also dependent upon frequency of wavelengths. Pig skin, similar to human skin, is strongly birefringent. The degree of depolarization is strongest at longer infrared wavelengths, and decreases as wavelength is shortened. When pig skin is damaged by gamma ray irradiation, the ability of the skin to depolarize light (tested at 450 nm to 675 nm) is further reduced. [22]
The triple helix structure of collagen makes it optically birefringent. This birefringence is ubiquitous in all biological tissues. [23] However, the ability to depolarize light is dependent upon various factors, the most important one being the depth or thickness of the tissue involved. [24]
Infrared wavelengths between 600 and 1500 nm can penetrate up to 5 mm on the human skin, which would be slightly below the dermis. Whereas light waves in the ultraviolet frequencies generally do not penetrate beyond the epidermis of, or under 1 mm in tissues. [22] The ability of collagen to depolarize light at shorter wavelengths is affected by how the collagen fibers are aligned and limited by the depth of the tissues probed. Due to this limitation, collagen on its own is not the ideal primary interface with quantum signals from our environment. But combined with ascorbate, or vitamin C, nature has created the perfect quantum interface for eukaryotes.
Collagen & Ascorbate, an Exquisite Entanglement
Collagen cannot be synthesized without ascorbate. Collagen requires ascorbate for hydroxylation, a process that allows the molecules to achieve the best configuration, rendering structural stability to the collagen fibers, making them stronger and less susceptible to damage. When human skin cultures are exposed to ascorbate, collagen synthesis is increased by eightfold with no increase in other proteins. In human skin, collagen accounts for up to 75% of the weight of the dermis which is below the epidermis where infrared frequencies can penetrate. [23] Even though the concentration of collagen is lower in the epidermis, this is the very layer of our skin where the highest concentration of ascorbate is found, and the epidermis is also where ultraviolet wavelengths can penetrate.
Normal skin contains high quantities of ascorbate, well above plasma levels and comparable to other body tissues. The concentration in the epidermis is between 2 to 5-fold higherthan that found in the dermis. Ascorbate is found to be depleted in aged or photodamaged skin, and skin that has been exposed to pollution or irradiation. [26] Is this depletion of ascorbate in the epidermis cause or effect? To answer that question, first we need to understand why there is so much ascorbate in the epidermis.
Ascorbate is a Quantum Workhorse
The sodium-dependent vitamin C transporter 2, or SVCT2 for ascorbate is found in every cell of the human body, even in mitochondria. [74] This transporter is one of the most evolutionarily conserved molecules and no species lacks this key protein. [28] The SVCT2 transporter will pick up and transport ascorbate even when the concentration available is extremely low (high affinity), in contrast to the SVCT1 ascorbate transporter, which is high capacity, but low affinity. [74] Together, these two transporters with different capacities and affinities ensure adequate ascorbate levels in all cells in the body. The knockout of the SVCT2 high affinity transporter in mice is lethal on day 1 of life, and when the SVCT1 transporter is deleted, half of the mice without the SVCT1 transporter do not survive to weaning. [36] Most of the time cells have only one form of the transporters. In the epidermis, both forms of the transporters are expressed. [26] Why does nature want to make sure there is more than adequate ascorbate in the epidermis?
Science has been relentlessly uncovering the endless roles undertaken by the epidermis in critical biological functions. It is well established that the skin is the largest organ of the body, with neural, endocrine and immune functions that regulate local and global homeostasis of the various systems involved. [29] Just recently (October 2018), circadian clocks stronger than those in the blood have been identified in the human epidermis. [30]
Ascorbate, the Primary Quantum Interface
Ascorbate is birefringent. It will depolarize light and render the incoming photons decoherent. You can think of ascorbate as the first interface where incoming photon signals are being decoded before secondary interfaces receive and act upon those quantum signals. How does quantum biology interpret the properties of ascorbate? So far, ascorbate has been associated with three quantum properties. It is able to absorb UV-B photons and suppress fluorescence (or fluorescence quenching) of radiation below roughly 310 nm. The absorption maximum of ascorbate is below 270 nm, with some studies showing a peak at 220 nm. Ascorbate is also able to lower excitation signals and transform short UV-B wavelengths between 280 nm to 320 nm into longer UV-A radiation in the 320 nm to 400 nm range. With fluorescence quenching ascorbate is able to substantially reduce emissions to the UV-A range of between 320 nm to 400 nm. These mechanisms effectively shift high-energy light waves into longer wavelengths emitting lower energies. [31] These quantum effects exert immeasurable influence on all aspects of essential biological functions, most of which begin in the epidermis of our skin.
Ascorbate and Vitamin D Synthesis
The epidermis is an active site for hormone synthesis, the most important and well known is that of Vitamin D. It is well established that upon exposure to UVB radiation, the sterol 7-dehydrocholesterol (7-DHC) is converted to pre-vitamin D3, which then is isomerized into vitamin D3. The biologically inert vitamin D3 then enters circulation and undergoes two successive hydroxylations in the liver and kidney to form the hormonally active metabolite calcitriol. [32]
The highest concentration of the sterol 7-DHC is found in the deepest two layers of the epidermis, the stratum spinosum and the stratum basale. These two layers also have the highest capacity for the conversion of 7-DHC into pre-vitamin D3. [33] The epidermis consists mostly of keratinocytes, and the bottom layer, stratum basale, is also where keratinocytes and melanocytes are formed. Ascorbate is highly concentrated in all layers of the epidermis. However, concentration of ascorbate has actually been found to increase with depth in the uppermost layer, the stratum corneum. [26] The stratum corneum is ABOVE the stratum spinosum and the stratum basale where 7-DHC is concentrated. So why is there such a high requirement for ascorbate in the epidermis that it expresses both forms of the SVCT transporters?
The sterol 7-DHC, precursor to vitamin D3, is extremely reactive to chain oxidationwhen exposed to exogenous radicals and oxygen. Oxysterols formed from the free radical chain oxidation of 7-DHC are cytotoxic. [33] Cholesterol and most oxysterols are excited by ultraviolet wavelengths below 200 nm. Ascorbate’s unique quantum ability to absorb UV-B and UV-C frequencies, and also to transform high energy short UV wavelengths into longer less energetic wavelengths is critical for metabolic processes in keratinocytes. [31] Why?
Keratinocytes Metabolize Calcitriol
Keratinocytes contain the entire metabolic pathway for the conversion of vitamin D3 to its active metabolite calcitriol, 1,25(OH)2D3, via the vitamin D-25 hydroxylase (CYP27A) and the 25OHD-1α-hydroxylase (CYP27B1). These are the same genes found in the liver and kidney that are responsible for the hydroxylation of vitamin D3 and its conversion into the active form calcitriol. In fact, the expression of CYP27B1 is HIGHER in keratinocytes than in any other cell in the body, including the cells of the proximal renal tubule in the kidney. CYP27B1 expression is the highest in the stratum basale of the epidermis in vivo. [34] The conversion of the highly reactive 7-DHC to calcitriol in keratinocytes is only activated in the presence of ultraviolet radiation in the ranges of 285 nm to 315 nm. [35] It is therefore reasonable to assume that the presence of the ascorbate as quantum interface would ensure minimal reactive chain oxidation by 7-DHC during the conversion processes.
In addition to negative feedback loops in keratinocytes, ascorbate also controls the level of calcitriol that can enter into circulation when the production by the liver and kidney is intact. [34] Acorbate not only has the ability to modulate the differentiation of keratinocytes [26], it is able to control the level of calcitriol production in keratinocytes via its effects on melanin synthesis.
L-DOPA, Melanin & Ascorbate
Melanin are chromophores that inhibit the conversion of 7-DHC by competing for the absorption of UV-B photons. An increase in melanin in human skin will increase the time required for exposure to UV radiation in the formation of pre-vitamin D3. [32]
Melanin is produced by melanocytes in the stratum basale, the last layer of the epidermis. Melanin is synthesized from its precursor L-tyrosine, with the aid of enzymatic reactions by tyrosinase. [38] Human keratinocytes have been demonstrated to synthesize catecholamines from L-tyrosine, and the human epidermis actually has the capacity for TOTAL catecholamine biosynthesis. Scientists have identified in keratinocytes, all the key enzymes for catecholamine synthesis including tyrosine hydroxylase, the rate limiting enzyme in the conversion of tyrosine into L-DOPA which is the important precursor to neurotransmitters like dopamine and norepinephrine. [39, 40] When L-DOPA is oxidized, melanin is formed. [41]
Ascorbate controls the formation of melanin by inhibiting the oxidation of L-DOPA. The presence of ascorbate can also increase the amount of L-DOPA synthesized by tyrosine in the presence or absence of UV-B irradiation. However, the amount of L-DOPA synthesized in the dark in the presence of ascorbate after exposure to ultraviolet is markedly higher than non-exposure to ultraviolet. Most importantly, ascorbate has the ability to reduce melanin. [42] The reduction of melanin is an area that is underexplored, but it has huge implications, considering fungi can use melanin to convert radiation into chemical energy.
Radiosynthesis & Ascorbate
Many eukaryotic fungal species produce melanin chromophores. These species have been found to be able to thrive in high-radiation environments, including the damaged nuclear reactor at Chernobyl. Melanin has the known capacity to absorb a wide spectrum of electromagnetic radiation and transduce these radiation into biologically useful energy. This phenomenon of radiation induced growth is called radiosynthesis. [43] The ability to transform radiation into electrical energy in fungi is substantially enhanced in the presence of ascorbate, or vitamin C. The oxidation of melanin as a result of irradiation produces electric current. The ability of ascorbate to reduce melanin through the transfer of electrons optimizes the redox capacity of melanin, resulting in enhanced electrical current synthesis. [44] Imagine a future world where humans have the capacity to turn electromagnetic radiation from natural and artificial sources into biologically useful energy by using our melanin biosynthetic pathways! But don’t get too excited over this prospect. Humans are ascorbate auxotrophs, meaning they are eukaryotes who have lost the ability to synthesize ascorbate. As such, our melanin biosynthetic pathways are different from that of fungi and far from perfect.
Melanoma, Melanin & Ascorbate
Melanoma is a dangerous form of skin cancer. The irradiation of melanin by ultraviolet rays has been shown to enhance free radical formation. Although melanin is protective at longer wavelengths, melanin enhances cell damage by radiation at shorter wavelengths. The induction of melanoma by ultraviolet A in the range between 320-400 nm requires the presence of melanin pigment, and is associated with oxidative DNA damage in melanocytes. On the other hand, no pigmentation is involved in the initiation of melanoma by ultraviolet B radiation in the range between 280-320 nm. This perhaps resolves the long standing conundrum why African albinos who have melanocytes but lack melanin, are highly susceptible to non-melanoma skin cancer, yet these same Africans are resistant to cutaneous malignant melanoma. [45] So how does the lack of ascorbate contribute to the melanoma-melanin equation? Why don’t we take a look at the difference between fish that can biosynthesize ascorbate and those that cannot.
Teleost are bony fish that have lost the ability to synthesize ascorbate. [46] Common examples of teleost are salmon, trout, cod, perch, herrings, catfish, carp, and minnows, to name a few. Teleosts produce melanin pigments and when exposed to high levels of ultraviolet radiation, these fish develop melanoma. The lesions on the skins of these fish with melanoma contained more melanin than in healthy cells. [47] Cartilaginous fish like hammerhead sharks, are able to biosynthesize ascorbate. [46] They too can ‘tan’ remarkably well under UV exposure. But unlike teleost fish, ascorbate producing sharks do not develop melanoma. “The juvenile shark's skin responded similarly to that observed in humans and other vertebrates in response to direct sunlight, turning from brown to black. Although a similar melanin response was seen in this study (i.e. increased melanin concentration), the sharks in this previous study showed no visible lesions or growths and were therefore not shown to contract melanomas or dermal carcinomas.” [47] The reduction of melanin by ascorbate in the skin of sharks could very well be the reason why sharks do not develop melanoma.
The epidermis is the first receptor of quantum signals from the environment. It makes perfect sense that ascorbate, the ultimate quantum interface, is found there in such high concentrations. The eye is the other important receptor for environmental quantum signals. How much birefringent ascorbate and collagen do you think can be found there?
Eye, Collagen and Ascorbate
The cornea has the highest concentration of ascorbate of all tissues in the body. As a primary quantum signal receptor for the body, the eye is where critical quantum entanglements are established. Quantum signals from light must be properly depolarized before they can be utilized by secondary biologic chromophore interfaces like melanopsin, melanin and hemoglobin. In humans, melanopsin is found in neurons in the retina, BEHIND the cornea. Before electromagnetic signals can reach chromophores and opsins like melanopsin, they are first depolarized by ascorbate and collagen in the cornea. The concentration of ascorbate in cornea is 14 times of that in the aqueous humor. The aqueous humor has 20 times the ascorbate in plasma. By this calculation, cornea would have about 300 times the amount of ascorbate when compared to plasma! The concentration of ascorbate in the corneal epithelium is perhaps the highest of any known and reported tissue in the human body. [48] It is interesting that of different animals examined, the highest concentration of ascorbate in the corneal epithelium are found in those diurnal species that encounter the highest environmental levels of ultraviolet radiation exposure. [48] And if this is not enough, don’t forget that collagen is also birefringent. The middle layer of the cornea is the stroma and accounts for nearly 90% of corneal thickness. The stroma is 71% collagen by dry weight, and contains three different types of collagen, [49] probably each with different birefringent qualities. How does the cornea get its ascorbate? Have you ever noticed that you may tear a lot when you are faced with strong sunlight? Tears also contain extremely high ascorbate, and it is believed that tears provide a continuous source of ascorbate for the corneal epithelium. [50]
It is without question that our high technology world is placing ever increasing demands on ascorbate availability. Humans can increase their ascorbate intake to keep up with demand, unlike plants and animals whose level of ascorbate biosynthesis may not keep pace with advances in technology.
Ascorbate in a 5G World
Human are unable to synthesize ascorbate. But our the ability to recycle certain amounts of ascorbate [51] combined with dietary intake allowed for optimal survival in environments that did not have excessive levels of artificial electromagnetic frequencies. Since the advent of technology, this ability to interface and depolarize ever-increasing electromagnetic frequencies began to decline exponentially in all eukaryotic plants and animals. For organisms that biosynthesize ascorbate, endogenous ascorbate production simply cannot keep up with the intensity of increasing radiation from man-made radio waves. For organisms that must obtain their ascorbate from diets, the decreased ascorbate levels in the food chain compounds the issue of deficiency.
A perfect example of this phenomena is the collapse of bee colonies as a result of increased electromagnetic frequencies. Increasing scientific evidence strongly support the theory of colony collapse disorder (CCD) among honey bees due to electromagnetic radiation from cell phones and cell towers. The massive amount of radiation produced by towers and mobile phones negatively affect honey bee behavior and biology. [52] Bee colonies are known to sustain a marked increase in loss rates during the winter as a result of decreased food sources that contain ascorbate. [53] Even though bees are able to biosynthesize ascorbate, the levels produced are obviously not enough to counter the effects of increased electromagnetic radiation that causes a significant reduction in endogenous antioxidants such as glutathione and catalase. The supplementation of ascorbate in diet of bees however, rescued colony loss rates during the winter by 33%, in parallel with increased antioxidant defense with elevated glutathione transferase and catalase activities. [54]
Birds on the other hand, appear to fare slightly better. Even though most birds do not synthesize ascorbate [46], breeding and migrating birds do not seem to be highly affected by electromagnetic radio waves.[55] Why? Feathers are made of keratin, and keratin is highly birefringent. [57] Feathers, therefore are able to depolarize electromagnetic radiation. The way birefringent feathers are arranged on the body of a bird is simply an exquisite display of nature at one of her better moments. [56]
How do plants protect themselves since they are exposed all the time to cosmic rays, irradiation from the sun and in the past half century, increased onslaught of electromagnetic frequencies in the form of artificial lights and Radiofrequency (RF) electromagnetic radiation (EMR) from a wide spectrum of radio waves? Plants, if you remember, have evolved a successful ascorbate biosynthetic pathway that does not generate reactive oxygen species. When exposed to varying levels of light intensities, plants usually respond by adjusting their ascorbate concentrations. [58] But the question remains, is the endogenous ascorbate production enough to counter the effects of EMR from cell towers? Trees grown in the direct vicinities of mobile phone base stations were found to show signs of damage that included stunted growth, brown leaves, irregular growth, dead branches, and color changes. Damage was usually the highest in locations with high radiation exposure and starts on the sides facing the source of radiofrequency radiation. Over time, the damage was extended to the entire plant. [59] A review of an extensive number of studies on the damaging effects of weak radio frequencies on plants showed mostly inhibition or reduced growth rates, whereas some studies actually did not find any negative response upon electromagnetic radiation (EMR) exposure. [60] How does EMR exposure reduce or inhibit growth in plants?
Ascorbate, Solar Energy Photoinhibition & Depolarization of Electromagnetic Radiation
Plants suffer reduced growth rates under photoinhibition when exposed to excessive solar irradiation. [61] Ascorbate provides photoinhibition protection to photosystem II in eukaryotic algae, plants, and prokaryotic cyanobacteria. Cyanobacteria do not use light for quorum sensing, instead they use acyl homoserine lactones (AHLs) as chemical signals in quorum sensing [62]. I propose the damage suffered by plants when exposed to EMR is a manifestation of reactions that are similar to solar irradiation induced photoinhibition. Both EMR and solar irradiation are electromagnetic frequencies, and both have been found to reduce photosynthetic capacities. Ascorbate attenuates photoinhibition by dissipating excess electrons and excitation energy via the water-water cycle in plants. [63] Ascorbate is now being viewed by science as indispensable for plant growth. [64] Ascorbate is birefringent. Birefringence causes depolarization. Electromagnetic radiation can be depolarized in the same way light is depolarized by birefringent mediums. When EMR undergoes depolarization, there is a redistribution of wave energy leading to a loss of the wave field energy. [65] Since radio waves can be depolarized, increased birefringence from ascorbate could very well protect plants that are affected by EMR exposure. Can ascorbate protect animals also?
Of Mice & Men, the Ascorbate Connection
In November 2018, the National Toxicology Program (NTP) released a report that demonstrated clear evidence that male rats exposed to high levels of radio frequency radiation (RFR) similar to that used in 2G and 3G cell phones developed cancerous heart tumors. The interesting part about this report is that although all rats received radiation across their entire bodies, female rats did NOT develop any tumor. In addition, longer lifespans were detected among MALE rats. [66] Are you intrigued? The explanation is really quite simple. It all has to do with how the birefringent, depolarizing ascorbate interfaces with the RFR on a quantum level in the male and female rats. In male rats, the level of ascorbate in heart muscle is low (5-10 mg per 100 g) when compared to the highest concentrations found in their adrenals (280-400 mg per 100g. If you will recall from the earlier section on birefringence and tissue health, lower birefringence equals less healthy tissues. In addition, the plasma content of ascorbate in male rats is an abysmal 1.6 mg per 100 g. At such low plasma ascorbate levels, very little ascorbate can be delivered to the epidermis to counter the high intensity radiation insults. [67] Female rats on the other hand, have extremely high levels of ascorbate in their plasma, ranging from 150 mg per 100 g to 165 mg per 100 g. [68] In comparison with ascorbate plasma level of male rats, that is a almost a 100-fold increase. With this level of circulating ascorbate in the plasma, the epidermis of the female rats should have more than adequate ascorbate to counter the effects of any amount of electromagnetic radiation. This is exactly what happened in the study. There was a complete absence of any tumor growth in the female rats treated with high intensity RFR. As for the inexplicable longevity observed in some male rats, the radiation exposure probably decreased ascorbate levels throughout the body. Decreased ascorbate is linked to the decrease in growth hormones [69 , 70], and a decrease in growth hormones has been linked to longevity [71].
The evidence for the birefringent ascorbate as the ultimate quantum interface certainly appears convincing. For those who may still be wondering, perhaps we should take a final look at how ascorbate relates to the one place where all quantum entanglements begin and end.
Mitochondria is an Ascorbate Hog
All eukaryotes have mitochondria. Mitochondria sustain life by providing energy. Being the final destination of all quantum interactions, it is no wonder ascorbate is found there in such high concentrations. However, judging by how mitochondria take in ascorbate, it is perhaps reasonable to assume that mitochondria are the first and last places where one would find ascorbate.
The study of mitochondria ascorbate uptake, regeneration and recycling is still an ongoing effort, with new ascorbate transporters, like the orphan transporter SVCT3, are being discovered. [72] The general consensus to date is that the high affinity SVCT2 transporter is used by mitochondria for uptake of ascorbate across all cells, tissues and species. More recently, mitochondria were demonstrated in the U937 cell to take up exponential amounts of ascorbate at the expense of cytosolic uptake. A fifteen minute exposure of 3 micromolar extracellular ascorbate concentration leads to an increase in ascorbate concentration in the cytosol to 45 micromolar. However, the mitochondria matrix concentration of ascorbate is 5000 micromolar. This means that during uptake the increase in the cytosolic concentration of ascorbate is disrupted by a more efficient mitochondrial uptake via the high affinity SVCT2 transporter. [73] On top of that, the requirement for transport across the SVCT2 transporter is set to a bare minimum in mitochondria. Unlike SVCT2s in plasma membrane which require 2 sodium ions per ascorbate molecule, sodium ion requirement is 100 fold less in mitochondria SVCT2 transporters. Ascorbate SVCT2 transporters in plasma membrane also require the presence of calcium and magnesium for transport of ascorbate. Mitochondria SVCT2 can take up ascorbate in the ABSENCE of calcium and magnesium. [74]
In plants, ascorbate is found to be synthesized by mitochondria between Complex III and Complex IV. [75] Mitochondria are where all quantum entanglements begin and end. Since ascorbate is the ultimate quantum interface, mitochondria will never be found without ascorbate in any living organism, plant nor animal.
The overwhelming evidence on ascorbate I have presented to you today is but a microscopic fraction of the portion of the iceberg that is visible to us currently. This iceberg is without doubt an important piece of the puzzle that will help us understand how we are entangled with our environment, natural or artificial. I cannot say whether ascorbate will be ‘the’ answer for survival and adaptation in the modern high tech world man has created. Having a better understanding of how the birefringent quantum properties of ascorbate can affect our adaptation is an excellent start though. Thank you for joining me on this important and exciting discovery. It would assist me greatly if you will take a moment to leave your impression or comments so I can plan my next article on ascorbate for you.
References:
Evolution of alternative biosynthetic pathways for vitamin C following plastid acquisition in photosynthetic eukaryotes https://www.ncbi.nlm.nih.gov/pmc/articles/PMC4396506/
OP20 - The evolution of vitamin C biosynthetic pathways in plants and algae https://www.sciencedirect.com/science/article/pii/S0891584915003718
The Mystery of Vitamin C https://www.nature.com/scitable/topicpage/the-mystery-of-vitamin-c-14167861
Quorum sensing in bacteria https://www.ncbi.nlm.nih.gov/pubmed/11544353
When microbial conversations get physical https://www.ncbi.nlm.nih.gov/pmc/articles/PMC3057284/#BX1
Antimicrobial activity of Vitamin C demonstrated on uropathogenic Escherichia coliand Klebsiella pneumoniae http://www.jcrsmed.org/article.asp?issn=2455-3069;year=2017;volume=3;issue=2;spage=88;epage=93;aulast=Verghese#ref25
Evaluation of Ascorbic Acid as a Quorum‐sensing Analogue to Control Growth, Sporulation, and Enterotoxin Production in Clostridium perfringens https://onlinelibrary.wiley.com/doi/abs/10.1111/j.1365-2621.2004.tb13374.x
Polarimetry and stereochemistry: the optical rotation of Vitamin C as a function of pH https://www.sciencedirect.com/science/article/pii/S0187893X14705361
Birefringence https://hamptonresearch.com/tip_detail.aspx?id=195
Depolarization index and the average degree of polarization https://www.osapublishing.org/ao/abstract.cfm?uri=ao-44-13-2490
Unpolarized light https://www.britannica.com/science/light/Unpolarized-light
Realizing controllable noise in photonic quantum information channels https://arxiv.org/pdf/1006.5795.pdf
Quantum decoherence versus classical depolarization in nanohole arrays https://openaccess.leidenuniv.nl/bitstream/handle/1887/61262/Quantum_decoherence_versus_classical_depolarization_in_nanohole_arrays.pdf?sequence=1
Quantum Decoherence https://en.wikipedia.org/wiki/Quantum_decoherence
Potecting entanglement from decoherence using weak measurement and quantum measurement reversal https://www.nature.com/articles/nphys2178
POLARIZED LIGHT MICROSCOPY AS A TOOL OF DIAGNOSTIC PATHOLOGY http://journals.sagepub.com/doi/pdf/10.1177/23.1.1090645
Polarization birefringence measurements for characterizing the myocardium, including healthy, infarcted, and stem-cell-regenerated tissues https://www.spiedigitallibrary.org/journals/Journal-of-Biomedical-Optics/volume-15/issue-04/047009/Polarization-birefringence-measurements-for-characterizing-the-myocardium-including-healthy-infarcted/10.1117/1.3469844.full?SSO=1
Biophoton interaction in biological systems: evidence of photonic info-energy transfer? http://www.u.arizona.edu/~kcreath/pdf/pubs/2005_KC_GES_SPIE_v5866p338.pdf
Biophotonic markers of malignancy: Discriminating cancers using wavelength-specific biophotons https://www.ncbi.nlm.nih.gov/pmc/articles/PMC5699883/
Anisotropy, birefringence, and optical phase retardation related to intersubband transitions in multiple quantum well structures https://aip.scitation.org/doi/10.1063/1.111568
Collagen birefringence in skin repair in response to red polarized-laser therapy https://www.ncbi.nlm.nih.gov/pubmed/16674192
Analysis of the depolarizing properties of irradiated pig skin http://iopscience.iop.org/article/10.1088/1464-4258/7/1/003
Effect of vitamin C on collagen biosynthesis and degree of birefringence in polarization sensitive optical coherence tomography (PS-OCT) https://academicjournals.org/journal/AJB/how-to-cite-article/A12EC627514
Imaging skin pathology with polarized light https://www.ncbi.nlm.nih.gov/pubmed/12175282
Birefringence and second harmonic generation on tendon collagen following red linearly polarized laser irradiation https://www.ncbi.nlm.nih.gov/pubmed/23247985
The Roles of Vitamin C in Skin Health https://www.ncbi.nlm.nih.gov/pmc/articles/PMC5579659/
Ascorbic acid content of neotropical plant parts available to wild monkeys and bats https://www.ncbi.nlm.nih.gov/pubmed/3104078
https://www.ncbi.nlm.nih.gov/pubmed/24594434
Ascorbic Acid and Gene Expression: Another Example of Regulation of Gene Expression by Small Molecules? https://www.ncbi.nlm.nih.gov/pmc/articles/PMC2851117/
Sensing the environment: Regulation of local and global homeostasis by the skin neuroendocrine system https://www.ncbi.nlm.nih.gov/pmc/articles/PMC3422784/
Population-level rhythms in human skin with implications for circadian medicine http://www.pnas.org/content/early/2018/10/29/1809442115
The significance of ascorbate in the aqueous humour protection against UV-A and UV-B https://www.ncbi.nlm.nih.gov/pubmed/8690035
The Cutaneous Photosynthesis of Previtamin D3: A Unique Photoendocrine System https://www.jidonline.org/article/S0022-202X(15)46131-7/pdf
Oxysterols from Free Radical Chain Oxidation of 7-Dehydrocholesterol: Product and Mechanistic Studies https://pubs.acs.org/doi/abs/10.1021/ja9080265
Vitamin D Metabolism and Function in the Skin https://www.ncbi.nlm.nih.gov/pmc/articles/PMC3188673/
UVB-Induced Conversion of 7-Dehydrocholesterol to 1α,25-Dihydroxyvitamin D3 in an In Vitro Human Skin Equivalent Model https://www.jidonline.org/article/S0022-202X(15)41438-1/fulltext
The SLC23 family of ascorbate transporters: ensuring that you get and keep your daily dose of vitamin C https://www.ncbi.nlm.nih.gov/pmc/articles/PMC3246704/
Quantifying birefringence in the bovine model of early osteoarthritis using polarisation-sensitive optical coherence tomography and mechanical indentation https://www.nature.com/articles/s41598-018-25982-9
Keratinocytes regulate the function of melanocytes https://www.sciencedirect.com/science/article/pii/S1027811714000238
Tyrosine Hydroxylase and Regulation of Dopamine Synthesis https://www.ncbi.nlm.nih.gov/pmc/articles/PMC3065393/
Catecholantines in Hutnan Keratinocyte Differentiation https://www.jidonline.org/article/S0022-202X(15)42202-X/pdf
Melanin Synthesis Pathways http://www.skinwhiteningscience.com/melanin_synthesis_pathways.html
Influence of Ascorbic Acid on Oxidation of Tyrosine by Ultraviolet Light http://journals.sagepub.com/doi/abs/10.3181/00379727-45-11579P?journalCode=ebma
Melanin, Radiation, and Energy Transduction in Fungi https://www.ncbi.nlm.nih.gov/pubmed/28256187
Gamma radiation interacts with melanin to alter its oxidation–reduction potential and results in electric current production https://www.ncbi.nlm.nih.gov/pubmed/21632287
Melanoma induction by ultraviolet A but not ultraviolet B radiation requires melanin pigment https://www.nature.com/articles/ncomms1893
The Genetics of Vitamin C Loss in Vertebrates https://www.ncbi.nlm.nih.gov/pmc/articles/PMC3145266/
Evidence of Melanoma in Wild Marine Fish Population https://journals.plos.org/plosone/article?id=10.1371/journal.pone.0041989
Ascorbic Acid Content of Human Corneal Epithelium https://iovs.arvojournals.org/article.aspx?articleid=2123569
Human corneal stroma contains three distinct collagens https://iovs.arvojournals.org/article.aspx?articleid=2176380
Tear ascorbic acid levels and the total antioxidant status in contact lens wearers: A pilot study https://www.ncbi.nlm.nih.gov/pmc/articles/PMC2712698/
Mechanisms of ascorbic acid recycling in human erythrocytes https://www.ncbi.nlm.nih.gov/pubmed/11687303
Effect of electromagnetic radiation of cell phone tower on foraging behaviour of Asiatic honey bee, Apis cerana F. (Hymenoptera: Apidae) http://www.entomoljournal.com/archives/2017/vol5issue3/PartU/5-3-142-590.pdf
Ascorbate Metabolism in Mature Pollen Grains of Dasypyrum villosum (L.) Borb. during Imbibition https://www.sciencedirect.com/science/article/pii/S0176161711801860
Supplementation of the honey bee diet with vitamin C: The effect on the antioxidative system of Apis mellifera carnica brood at different stages https://www.tandfonline.com/doi/pdf/10.3896/IBRA.1.51.3.07
Response of Breeding And Migrating Birds to Extremely Low Frequency Electromagnetic Fields https://www.jstor.org/stable/2269494?seq=1#page_scan_tab_contents
DEVELOPMENT OF FEATHER KERATIN DURING EMBRYOGENESIS OF THE CHICK http://jcb.rupress.org/content/jcb/16/2/215.full.pdf
Birefringence and Elasticity in Keratin Fibres https://www.nature.com/articles/172675a0
Dynamic compartment specific changes in glutathione and ascorbate levels in Arabidopsis plants exposed to different light intensities https://www.ncbi.nlm.nih.gov/pmc/articles/PMC3728233/
Radiofrequency radiation injures trees around mobile phone base stations https://www.baubiologie.de/downloads/wug/rf-radiation-injures-trees-2016.pdf
Review: Weak radiofrequency radiation exposure from mobile phone radiation on plants https://www.ncbi.nlm.nih.gov/pubmed/27650031
Photoinhibition of photosynthesis in needles of two cypress (Cupressus sempervirens) clones https://www.ncbi.nlm.nih.gov/pubmed/15929934
Quorum sensing in Cyanobacteria: N-octanoyl-homoserine lactone release and response, by the epilithic colonial cyanobacterium Gloeothece PCC6909 https://www.nature.com/articles/ismej200868
The water-water cycle as alternative photon and electron sinks https://www.ncbi.nlm.nih.gov/pmc/articles/PMC1692883/
Ascorbate-mediated regulation of growth, photoprotection, and photoinhibition in Arabidopsis thaliana https://academic.oup.com/jxb/article/69/11/2823/4991886
Depolarization effects of radio wave propagation in various land built-up environments https://ieeexplore.ieee.org/abstract/document/6904806
High exposure to radio frequency radiation associated with cancer in male rats https://www.nih.gov/news-events/news-releases/high-exposure-radio-frequency-radiation-associated-cancer-male-rats
DISTRIBUTION OF ASCORBIC ACID, METABOLITES AND ANALOGUES IN MAN AND ANIMALS https://nyaspubs.onlinelibrary.wiley.com/doi/abs/10.1111/j.1749-6632.1975.tb29271.x
Evaluation of tissue ascorbic acid status in different hormonal states of female rat https://www.sciencedirect.com/science/article/abs/pii/002432059390111F
The Effect of Vitamin C on Growth Hormone Secretion https://clinicaltrials.gov/ct2/show/NCT01537094
The Association of Macro- and Micronutrient Intake with Growth Hormone Secretion https://www.ncbi.nlm.nih.gov/pmc/articles/PMC3392357/
Life Span Extension by Reduction in Growth Hormone-Insulin-Like Growth Factor-1 Axis in a Transgenic Rat Model https://www.ncbi.nlm.nih.gov/pmc/articles/PMC1850833/
The sodium-dependent ascorbic acid transporter family SLC23 https://www.ncbi.nlm.nih.gov/pubmed/23506882
Mitochondrial Uptake and Accumulation of Vitamin C: What Can We Learn from Cell Culture Studies? https://www.ncbi.nlm.nih.gov/pubmed/28699359
The mitochondrial transporter of ascorbic acid functions with high affinity in the presence of low millimolar concentrations of sodium and in the absence of calcium and magnesium https://www.sciencedirect.com/science/article/pii/S0005273615000887?via%3Dihub
Ascorbate Biosynthesis in Mitochondria Is Linked to the Electron Transport Chain between Complexes III and IV https://www.ncbi.nlm.nih.gov/pmc/articles/PMC59007/
#Vitamin C#quantum biology#quantum physics#quantum consciousness#vitamins#integrative medicine#mitochondria#ascorbic acid
4 notes
·
View notes
Text
Stress Birefringence
The birefringence effect caused by the internal stress meter of optical components will affect the polarization state of light, which can not be tolerated in micro-lithography, laser optics and astronomy. In general, the requirements for accurate measurement of small stress birefringence are very demanding. The image polarization measuring instrument, which can give the spatial distribution and direction of stress birefringence at the same time, has solved this problem well.
Under less stringent conditions, optical glass can generally be considered to be homogeneous, with the refractive index being equal everywhere in all directions. However, the stress caused by the material or the production process will deform the structure of the material, resulting in local density differences along the axial direction. The propagation speed of light in a medium is related to the density of the material. The change of the local density leads to the difference of the speed of light propagating in the medium and the change of the refractive index related to the direction. The birefringence phenomenon of the medium under the action of stress is the so-called stress birefringence (SBR).
In addition to optically isotropic materials, there are also many naturally occurring optically anisotropic materials, also known as birefringent materials, such as calcite and quartz crystals. For these materials, changes in the refractive index ratio can also be seen under mechanical stress, which can be so large as to cause damage to the crystalline material. Even small changes in the local refractive index can have a negative impact on the imaging quality of the optical element, thereby affecting its functionality. In addition, birefringence changes the polarization state of the transmitted light, which is detrimental in applications such as metrology. Therefore, it is very important to accurately determine the stress birefringence and its spatial distribution in the manufacture of optical materials and components.

https://www.ptc-stress.com/stress-birefringence/
0 notes
Text
It's Zircon Time!
(If you are the same age as me you may well have read that like you were a Power Ranger saying "It's Morphin' Time" and you wouldn't be incorrect because we're going to talk about how zircon slowly degrades from crystalline to amorphous structure over millions of years... so...)
I'm excited to tell you about gem-quality zircon!

@theproblemwithstardust I have no idea if you're still interested but heads up I got carried away I think I wrote over 3k words about this you may need snacks and an interval
Zircon & Double Refraction
Zircon is a naturally occurring gem mineral, chemical formula zirconium silicate and crystallising in the tetragonal system. It is a uniaxial optically anisotropic gemstone (remember, optically anisotropic means light is split into two when passing through the crystal) and in its rough form occurs as elongate to squad tetrahedral crystal with bipyramidal terminations.
(What does that mean? It means it's a rectangle with two triangles at the ends, and the rectangular section might be long or short!)

Even as a rough crystal zircon will show some of the features it is well known for - such as its sub-adamantine lustre (meaning the near-diamond-like brightness of surface reflection of white light) and (if the crystal is transparent enough) a high birefringence value means internal features are viewed as doubled.

Woah... you just chucked a LOAD of science terms at me! What does any of it mean?
Let me tell you about double refraction in zircon! I'm so excited by it!
I'll break these terms down one at a time:-
Refraction - the bending of light as it passes through the crystal
Double refraction - two rays of light get bent at slightly different angles! (Remember that as an optically anisotropic material, zircon splits light into two rays)
Birefringence - the difference in the amount the two rays of light are bent by
You know how when you stick a straw in a glass of water, it looks as though it is slightly bent? That's refraction at play! When light passes between two mediums of different optical densities (for example from air into water, or air into a gemstone) the light is bent. The angle is it bent by is related to the substance it passes through. We use refraction in gemmology to identify gemstones, because every stone refracts (bends) light by a different amount.
In an optically anisotropic gemstone the two rays of light are both bent by different amounts. We can measure how much each ray of light is bent by, and the difference between them, to help identify them!
Fun fact, the 2 rays of light are referred to as the ordinary ray and the extraordinary ray - that is important for identifying stones by their RI but I'm getting off topic for zircon!
The important thing in zircon is that it has Very High Birefringence. That means that the two rays of light are bent by so much that when they leave the gemstone and reach the viewer (that's you!) you see a doubled image of whatever is inside the stone - double vision!


(If like me you wear glasses, it feels like looking at the gemstone without your glasses on - everything just sliiightly out of focus...)
If a zircon has inclusions, each of these will appear to be doubled when viewed through the crystal. Even easier to spot, and present even in an inclusion-free stone, is the doubling of the back facets of the gemstone! That's right, when you look through the stone at the pattern of facets on the other side, they will appear to be doubled.
Haang on a sec - what about the Optic Axis, I hear you ask?
An Optic Axis is a direction in an optically anisotropic gemstone in which light behaves as though it is passing through an optically isotropic material. That's a material where light travels as a single ray rather than splitting into two - so when viewed from the right angle, a zircon crystal will let light pass through as a single ray, and you won't see any double refraction at all!

Fun fact! In a uniaxial crystal, there is one optic axis and it is always parallel to the c-axis (the long dimension of the crystal).
This is all pretty neat, right? If you want your colourless zircon crystal to pass as a diamond imitation, it should be cut with the table perpendicular to the c-axis. That way, when you look straight down at the top of the stone, you won't see any of that dizzying eye-visible double-refraction - diamonds are optically isotropic, so they only ever transmit a single ray of light :)
(I mean there are tons of other ways to differentiate diamond and zircon, but at a glance, it would make for a better imitation...)
Metamict Zircon
Wouldn't it be great if zircon were always so easily identifiable in part due to its high birefringence? Not a lot of stones that have eye or loupe visible birefringence - most of them are much smaller values (ie. the difference in the angle the two rays of light are bent at is much smaller)
Sad news for you friends, but zircon does not always stay in this nicely ordered highly crystalline state, behaving as a tetragonal optically anisotropic crystal should.
You see, the thing that gives zircon it's colour is Uranium. That's right, radioactive uranium!

And even when it is only present as a few ppm (parts per million) in the zircon structure, the radiation emitted by those atoms is enough to start breaking through the bonds between other atoms in the zirconium silicate structure, and slowly but surely the structure of zircon is transformed from highly organised crystalline bonds, to irregular and disorganised amorphous atomic arrangement.
Why am I talking about this off the back of birefringence values?
Because amorphous materials are optically isotropic - that is, light behaves the same in all directions. No more double refraction!

(In case you haven't noticed yet you are actually taking a stroll through the gemmology section of my mind and encountering Related Thoughts in the exact order in which they are stacked and catalogued in my Mental Shelves, I hope you are keeping up but hit me up in the comments or reblogs if you need me to circle back to anything?)
But! I hear you cry - if you identify zircon by it's birefringence, how do you tell it's zircon when it's altering to an amorphous state?
When zircon becomes amorphous - also known as becoming metamict, it doesn't suddenly change all at once. There will be areas of crystalline structure interspersed with amorphous areas. When viewed with a 10x loupe, this gives the stone a hazy or grainy internal appearance. Although many gemstones show various types of zoning, the hazy zoning associated with metamict zircon is quite distinctive.
The colour of zircon also changes during this breakdown of the crystal structure, becoming a greenish colour instead of the usual browns, reds, yellows.

Heated zircon can be colourless or even bright blue - I kinda assume they would follow the same path when becoming metamict but can't say for sure! That said, I know from my own experimentation that heated blue zircon goes a murky grey-brown on exposure to UV, so perhaps it really is all the same process :)
Diagnostic Absorption Spectra in Zircon
What if the zircon is completely metamict? What if there is no birefringence at all, and you can't be certain that the haziness inside the stone is identifiable as hazy zoning?
Well, don't forget that a lot of gemmological identification involves combining different observations and test results - it's very rare to be able to do a single test and say "THIS. It's definitely THIS."
That said... there is a test we can do on zircon which provides a diagnostic result, even in the absence of all other tests!
We can check the Absorption Spectrum!
Holllllld up... better go over what we mean by Absorption Spectra.
When white light passes through a gemstone, it is modified via absorption...
Wait, go back a bit further.
White light is actually made up of light of all coloured wavelengths from 400nm (violet) to 700nm (red) - this is called the 'visible light spectrum' as these are the wavelengths the human eye can detect!

When all colours of light at once reach the human eye, the mind interprets it as 'white' light.
When the colour is modified because one or more wavelengths are removed, we then start to perceive colour.
For example, when light passes into a corundum crystal coloured by chromium, most violet, some blue, and all green and yellow and some orange light is absorbed. The resulting colour you see, made up from transmitted red and a little blue light, is red!
By the way, the absorption I just described is for ruby! :)
BACK TO ZIRCON!
The uranium in zircon causes a unique absorption which can be viewed with a spectroscope. Many gemstones, even colourless ones, show various absorption spectra. Relatively few show a diagnostic absorption spectra, which has a pattern of absorption unique to that gem species and colouring element. Although you should always back up your gemstone identifications with multiple pieces of evidence, with a diagnostic feature you can make a positive identification of gem species even in the absence of other information.
So what does zircon's diagnostic absorption spectrum look like?
Well, that can vary depending on whether it is high (crystalline) zircon or low (metamict) zircon, and whether it has been heat-treated to alter it's colour!
The defining feature in all types of zircon is a diagnostic absorption line at 653nm (about half-way along the red area of the spectrum).

In heat-treated colourless or blue zircon, this may be the only absorption line present.

In other zircons, as well as the 653nm line, you will see up to 40 other absorption lines or bands scattered throughout the spectrum.
(Oh backtrack a moment again! When viewing the absorption spectra, the black lines are the wavelengths that are being absorbed :) Please do remind me to tell you this stuff, I forget because I Thought It Was Obvious (as Tech would say) but I'm doing my best to cover the basic science as well as gemmology here)

Metamict zircon still shows a diagnostic absorption spectra, including the line at 653nm. However, many of the absorption lines and bands have become distinctly fuzzy-looking - this is another helpful piece of evidence to determine whether you are looking at high zircon or metamict zircon.
(Still here, team? I have been writing for 2 hours and we're 1700 words deep, so take a break if you need it, hydrate or diedrate... save this post for later... close the tab and back slowly away from the screen because you didn't realise you were getting into all this when you clicked below the read-more...)
Toughness and Dispersion
Other features of zircon that are important when considering its use as a gemstone are it's relatively high hardness, but low toughness.
Hardness and toughness? Aren't they the same thing?
No :D
Hardness (in gemmological terms) relates to the ability of a material to resist being scratched when the sharp point of another material is dragged across the surface. Zircon has a Moh's Relative Hardness rating of 8/10 - that's pretty good resistance to being scratched!
However, it has Low toughness. Toughness relates to the ability of a material to resist being fractured or cleaved as a result of physical impact. In short, zircon chips easily. When faceted as a gemstone, it tends to chip along the sharp edges between the facets, accumulating numerous small fractures which are described cumulatively as 'nibbled facet edges'.

Remember when I said there were numerous other ways to tell the difference between diamond and zircon? This is one of them! You would not expect to see wear like this to a diamond - however, unless your zircon has been very well cared for, you would expect the facet edges to show a certain amount of abrasion due to its low toughness... and yes, if you view those chips through the crystal, they will appear to be doubled due to the high birefringence :)
Other features of zircon include a high Dispersion Index.
Dispersion is the splitting of white light into its spectral wavelengths when passing through two inclined surfaces of a transparent material (think the Pink Floyd Dark Side of the Moon prism splitting the single ray of white light into the rainbow!)

Guess what? When you facet a gemstone, it almost always has two inclined surfaces of transparent material! In gemstones with a high Dispersion Index, you will see more dispersion - that is, when you tilt them, you will see more flashes of coloured light (blue, green, red) sparkling back at you from the facets than a gemstone with a low dispersion index.
You can see dispersion even in gemstones with a body colour - they don't have to be colourless to see it! That said, it can be harder to spot some of those dispersed colours against the body colour - so this feature may be more prominent in colourless or light coloured zircons than in deeper colour samples.

Aaaaaaaand I think that's it? At least, I think that's everything I wanted to cover with you about gem-quality zircon today!
BUT WAIT!
The fun's not over :P
I'm pretty sure I promised a comparison between naturally occurring zircon, and common artificial material cubic zirconia!
(Just when you thought you were free...)
Natural vs. Artificial
Zircon is a naturally occurring, crystalline, inorganic mineral formed by natural processes. Don't forget, the chemical formula is Zirconium Silicate and it crystallises in the tetragonal system.
Cubic Zirconia (shortened to CZ) is an artificial crystalline material grown by man. The chemical formula is Zirconium Oxide and it crystallises in the cubic system.
Notice how I say artificial, not synthetic? That's an important distinction.
Synthetic materials have a direct comparison in nature. All synthetic gemstones are artificially grown, but not all artificial materials are considered synthetic.
To be classified as a synthetic gemstone, the result of the artificial growth process must be chemically, physically and optically identical to the naturally occurring mineral.
Cubic Zirconia has no natural counterpart. It is completely artificially created. Hence, it can be referred to as artificial or as a simulant (meaning it is simulating/imitating another natural material).
Cubic Zirconia
CZ is grown via the incredibly metal sounding process of SKULL MELTING
Fun fact! CZ has a higher melting point than any material you could make a crucible of to melt it in. So when we make it, we have to melt it inside a skin, or 'skull', of solid CZ, with a liquid inside. We can go into the science another time if you like, but the quick version is it's like heating up lasagne in the microwave, and the middle gets hot whilst the edges are still cold... that's how we melt the centre of the CZ mix whilst keeping in in a cooled skull of its own solid material :)

Above a certain temperature (I want to say off the top of my head with a melting point in excess of 2600 degrees Celsius), zirconium oxide adopts a cubic arrangement - nice and symmetrical, and optically isotropic (light moves as a single ray and behaves the same in all directions). However below temperatures of around 2000 degrees Celsius, zirconium oxide would crystallise in the monoclinic system. Woaaah! That's not terribly symmetrical! We want it to be cubic zirconia, the same in all directions.
To stop the zirconium oxide mix from changing to a monoclinic arrangement as it cools, we need to introduce a stabilising element. Most often this is a rare earth element such as yttrium, which bonds with the zirconium oxide structure and forces it to retain its cubic arrangement even at lower temperatures. Cool, right?
(ahaha I didn't mean to make that joke... cool... cos we're cooling the mixture... it's late and I've been typing for a long time now I hope you're still with me...)
Fun fact, due to it's super high melting point, you actually need to start the melting and recrystallisation process by inserting a thin wafer of pure zirconium into the ingredient mix inside the Skull. This can then be melted, which will then oxidise, and start a chain reaction of melting and bonding with the surrounding material :)

CZ Optical Behaviours
CZ is cubic, meaning it is optically isotropic, so you will only have single refraction (a single ray of light transmitted) no matter which direction you view the stone in.
This is enough to differentiate it from zircon, which is optically anisotropic with high birefringence, but what about diamond, the gemstone that CZ so frequently imitates?
Don't worry - we can again look at features such as the absorption spectra, and hardness/toughness as well as lustre to tell the difference.
The lustre (surface reflection of white light) of CZ is lower than that of diamond (adamantine) or zircon (sub-adamantine). I want to say CZ is bright vitreous (bright glasslike)? So with practice you will learn when the surface reflection just doesn't seem quite bright enough to be diamond...
(It's bothering me that I skipped the formal lustre definition earlier so here it is now: lustre is the quality and quantity of white light returned via reflection from the surface of the gem material towards the viewer)
CZ has a really high dispersion index - that's the splitting of white light into its spectral colours, remember, the coloured sparkles you see when you move the stone! CZ has a dispersion index even higher than diamond, so if the stone seems to be returning too many sparkles of colour... it's probably too good to be true.

CZ also grows free of inclusions, and the absence of internal features is a huge warning sign that you're looking at an imitation rather than a diamond!
As well as these optical features, CZ will also show surface features to differentiate it from zircon or diamond. The facet edges even in well-cut stones are typically rounded and soft, and although they don't tend to accumulate the 'nibbled' look of chipped zircon, the facets can be scratched - typically picking up a 'frosted' look if they are particularly heavily worn.
Remember when I mentioned using yttrium or a similar element to force the zirconium oxide mix to retain it's cubic structure? That's what causes the absorption spectra in CZ!
CZ shows a REE (rare earth element) spectra. It's not diagnostic like a zircon spectra is, but it is highly characteristic. Both coloured CZ and colourless can show it - REE spectra typically manifest as clusters of many fine absorption lines mostly distributed in the yellow-green area of the spectrum.
Iiiiiii think I might actually be done now (except the zircon vs CZ comparison strayed into grounds of differences between diamond and its common simulants so I'm trying not to get doubly triply side-tracked into synthetic moissanite which is ALSO doubly refractive with loupe-visible doubling of internal features due to high birefringence and only gets to old the term 'synthetic' on a technicality because naturally it is almost impossible to find gem-quality moissanite outside of rare meteoric impact events...)
(Guess what synthetic moissanite is silicon carbide crystallising in the hexagonal system and is created by a process known as sublimation - where a material goes from solid to gaseous or plasma state (or back) without passing through a liquid state in between!)
Hmm I realise by the time I post this I will have added photos but I think I'll save as a draft for now and maybe sleep before I do that... **Photos added now! Microscope still not set up so you have to make do with my internet searching, sorry
I hope you have enjoyed today's citizen science gemstone lecture brought to you by the ability to recall memorised information without checking my notes and the parasocial relationship I have projected onto you, the Tumblrites, hoping that you will love the science side of gemstones as much as I do!
just-thoughts-about-gems (this is the place to ask your gemstone questions)
#questions in a comment or reblog and i'll do my best to answer :)#gemstones#gemmology#gemology#zircon#cubic zirconia#i feel i should declare#that for all i talk about refractive indexes and birefringence as identifying measurements in gemstones#the gem species we are talking about today have RI values too high to be measured by a standard gemmological refractometer ^^;#you're relying on that eye-visible birefingence#and other features#for identification#that said#someone mentioned apatite!#can't get much further in the birefringence scale from zircon than that#without being optically isotropic#and therefore having no birefringence value#apatite birefringence of 0.003-0.005#that is super small#barely able to measure it without the aid of polarising filters to differentiate the two rays of light and read their RI values#refractive index#and birefringence#have no units#they are relative values#not absolute#i can remember lots#but i do have to get a book to look up zircon's RI and birefringence#(maybe because i can't measure it on the refractometer anyway so there was no merit to memorising it)#i HAVE to go to bed#goodnight lovely gemstone friends
20 notes
·
View notes
Text
Blue Sapphire - Neelam Gemstone
Natural Blue sapphire, also Called Neelam, Neelamani, Indraneel or the Neela Pukhraj, Neelam, It goes on the Corundum mineral household as well as also the Vedic astrology deems it since the fastest acting bead gift currently.

Astrological Benefits
Blue sapphire is dominated by the powerful lord Saturn and gets the capability to flourish the life span of the wearer immediately. However, a wrong use of this gem can ruin him also. This stone is famous to bestow the wearers with fantastic benefits in his profession or business by appeasing Saturn and eliminating its own damaging movements in the research. Other astrological advantages of Blue Tooth include boundless fame and fortune to the wearer within his creative efforts or in his political aspirations.
Healing Properties
Blue sapphire is Associated with love and innocence. It enriches the wearer's throat and assists the individual's communication abilities. Moreover, it encourages the wearer to keep on the religious path and experience the greatest healing of body and mind by unplugging all of the blockages.
Blue Sapphire Origins
Mines of Blue the blue sapphires located in Kashmir are ranked the highest concerning quality, due to their royal blue color and a velvety texture.
Birthstone
Gemstone that communicates a birth. Every one of those twelve weeks is associated with multiple or single diamonds. In early times, it had been considered that this rock safeguards your loved ones from any sort of harm. Additionally, it represented trust and loyalty.
Four Variables to Select Greatest Blue Sapphires
While Choosing the Finest blue sapphire to buy, you need to consider these four major aspects.
· Cut -- A great deal depends upon the cut and also the form of the Neelam stone. A nice cut can boost the brightness of the blue sapphire many times. Further, an oblong or oblong shaped blue sapphire stone is more affordable than a square or additional custom shaped kinds.
· Clarity -- Locating a totally clear Stone is very rare, because, visible inclusions are consistently current in them because of geological outcomes. Thus, a crystal blue pearl is extremely pricey. These vividly bright diamonds are more expensive when compared with blue sapphires that are overly dark or too grey.
· Carat weight it’s a rare occasion to obtain this rock which has equally high quality weight and higher clarity simultaneously. Therefore for these Neelams, the purchase price can vary in tens of thousands of dollars.
Blue Sapphire Works with Gold or Silver Metal.
Expert astrologers Suggest sporting a blue sapphire with just silver or gold alloy. It's ideal to receive it studded at a silver or gold ring and put it at the middle finger of the ideal hand. It is also possible to personalize it into a necklace or a necklace, in accordance with your precise requirements. Most of all, the blue sapphire should touch the wearer's skin so as to avail its own benefits.
Wearing Process
It's of utmost significance to wear almost any gemstone in its own prescribed Manner in order to prevent its negative outcomes. Is as follows-
· The most important thing to be taken prior to sporting a gemstone, particularly a Blue horoscope. Wearing a Blue Tooth in you can cause accidents and sick luck.
· After your Astrologer offers you a flag that is green, you can go right ahead and buy an Authorized and legitimate Blue Tooth gemstone as a fictitious stone won't bring out the desirable benefits.
· Following the Purchase of this gemstone, the upcoming important point to understand is your most suitable metal to repair the Blue Sapphire right into. Blue Sapphire could be worn in.
· It's thought that the impact of the stone is dependent upon its weight. Heavier the Stone, more successful it could be. Usually, an Individual can wear a Blue Tooth
· Saturday Evening is thought to be the most felicitous to put on a Blue Tooth / Neelam.
· It's advised to dip the ring Holy water or milk prior to wearing it to eliminate all of the impurities which the ring may have.
· Following the Ring is washed, keep it on a dark colored fabric where Saturn Yantra is drawn with Kumkum.
· The ring can currently be worn at the middle finger of their left or right hand, based on the sex. Men will wear the Blue Sapphire within their right hand while the females may wear it in their hand. The rock, generally, loses its Result in 4.5 to 5 decades and has to be changed afterwards.
· Make sure to wash out the ring of dust and dirt regular.
Properties of Blue Sapphire
Hardness: 9 (Moh’s scale of hardness)
Optic Character: Anisotropic
Refractive Index (RI): 1.760 – 1.778.
Birefringence: 0.007 – 0.010.
Specific Gravity: 3.99 – 4.01.
For More Information about Blue Sapphire Visit Here.
0 notes
Text
Laser writing enables practical flat optics and data storage in glass
https://sciencespies.com/physics/laser-writing-enables-practical-flat-optics-and-data-storage-in-glass/
Laser writing enables practical flat optics and data storage in glass


(Left) Birefringence image of a flat lens and intensity patterns of 488 nm laser beams with different handeness circular polarizations focused and defocused by the same lens. The focal lengths are ± 208 mm. (Right) The same lens corrects short -5 D and long +5 D sightedness. Credit: by Masaaki Sakakura, Yuhao Lei, Lei Wang, Yan-Hao Yu, and Peter G. Kazansky
Femtosecond laser machining has emerged as an attractive technology enabling applications ranging from eye surgery to direct writing on the bulk of transparent materials. Scientists from the University of Southampton, UK, demonstrated a new regime of ultrafast laser writing in silica glass, which produces anisotropic nanostructures and related birefrigence with negligible transmission loss. The technology enables practical wavefront shaping with flat optics and polarization beam shaping of high power lasers from ultraviolet to infrared, as well as high-capacity optical data storage.
Conventional optics (e.g. lenses or mirrors) manipulate the phase via optical path difference by controlling thickness or refractive index of material. Recently, researchers reported that arbitrary wavefronts of light can be achieved with flat optics by spatially varying anisotropy, using geometric or Pancharatnam-Berry phase. However, despite various methods employed for anisotropy patterning, producing spatially varying birefringence with low loss, high damage threshold and durability remains a challenge.
In addition, the technologies of birefringence patterning have been also used for generating light beams with spatially variant polarization known as vector beams, in particular with radial or azimuthal polarization. Radially polarized vector beams are especially interesting due to the non-vanishing longitudinal electric field component when tightly focused, allowing superresolution imaging. Radial polarization is also the optimal choice for material processing. On the other hand, azimuthal vector beams can induce longitudinal magnetic fields with potential applications in spectroscopy and microscopy. Nonetheless, generating such beams with high efficiency is not a trivial matter.
In an article published in Light Science & Applications, scientists from the Optoelectronics Research Centre, University of Southampton, UK, demonstrated a new type of birefringent modification with ultra-low loss by ultrafast laser direct writing in silica glass. The discovered birefringent modification which is completely different from the conventional one originating from nanogratings or nanoplatelets, contains randomly distributed nanopores with elongated anisotropic shapes, aligned perpendicular to the writing polarization, which are responsible for the high transparency and controllable birefringence.
This birefringent modification enabled fabrication of ultra-low loss spatially variant birefringent optical elements including geometrical phase flat prism and lens, vector beam converters and zero-order retarders, which can be used for high power lasers. The high transmittance from UV to near-infrared and high durability of the demonstrated birefringent optical elements in silica glass overcome the limitations of geometrical phase and polarization shaping using conventional materials and fabrication methods including photo-aligned liquid crystals and meta-surfaces.
The researchers report: “We observed ultrafast laser induced modification in silica glass with the evidence of anisotropic nanopore formation representing a new type of nanoporous material. The technology of low loss polarization and geometrical phase patterning widens the applications of geometrical phase optical elements and vector beam convertors for high power lasers and visible and UV light sources. The space-selective birefringent modification with high transparency also enables high capacity multiplexed data storage in silica glass.”
Explore further
No such thing as nonlinear resolution in ultrafast laser machining
More information: Masaaki Sakakura et al, Ultralow-loss geometric phase and polarization shaping by ultrafast laser writing in silica glass, Light: Science & Applications (2020). DOI: 10.1038/s41377-020-0250-y
Provided by Chinese Academy of Sciences
Citation: Laser writing enables practical flat optics and data storage in glass (2020, February 20) retrieved 20 February 2020 from https://phys.org/news/2020-02-laser-enables-flat-optics-storage.html
This document is subject to copyright. Apart from any fair dealing for the purpose of private study or research, no part may be reproduced without the written permission. The content is provided for information purposes only.
#Physics
0 notes
Link
When electrons are confined into very small spaces, they can exhibit unusual electrical, optical and magnetic behaviour. From confining electrons in two-dimensional atomic sheet graphene -- a feat that won the Nobel Prize in physics in 2010 -- to restricting electrons even further to achieve one-dimensionality, this broad line of research is transforming the landscape of fundamental research and technological advances in physics, chemistry, energy harvesting, information and beyond.
In a study published in Nature Communications, an international team led by Aalto University researchers has now found that fibrous red phosphorus, when electrons are confined in its one-dimensional sub-units, can show large optical responses -- that is, the material shows strong photoluminescence under light irradiation. Red phosphorus, like graphene, belongs to a unique group of materials called one-dimensional van der Waals (1D vdW) materials. A 1D vdW material is a radically new type of material that was discovered only in 2017. Until now, research on 1vdW materials has focused on electrical properties.
The team uncovered the optical properties of 1D vdW fibrous red phosphorus through measurements like photoluminescence spectroscopy, where they shone laser light on the samples and measured the colour and brightness of the light emitted back. The findings show the 1D vdW material demonstrates giant anisotropic linear and non-linear optical responses -- in other words, the optical responses strongly depend on the orientation of the fibrous phosphorus crystal -- as well as emission intensity, which relates to the number of photons emitted during a specific time.
Read more.
#Materials Science#Science#Phosphorus#Red phosphorus#Optics#1D materials#Fibers#Photoluminescence#Aalto University
15 notes
·
View notes
Text
300+ TOP ENGINEERING GEOLOGY Lab Viva Questions and Answers
ENGINEERING GEOLOGY Lab Viva Questions :-
1. The shape of the Earth is: Geoid 2. Age of the Earth is 4.6 billion years 3. Sial and Sima are separated by Conrad discontinuity 4. Morhorovicic discontinuity is found between Crust and Mantle 5. Mantle and Core are separated by Gutenberg discontinuity
GEOLOGY Viva Questions 6. The term ‘NiFe’ refers to Core of the Earth 7. The plastic layer of the mantle is called Asthenosphere 8. The composition of Sial is Granitic to grano dioritic 9. The composition of Sima is Basaltic 10. The polar and equatorial diameter of the Earth is 12,713 km and 12,756 km Geomorphology : 11. The process of disintegration and decomposition is called Weathering 12. The process of Erosion includes Disintegration 13. Frost action takes place due to Freezing of water 14. Which minerals are highly susceptible to chemical weathering Ferromagnesian Minerals 15. Which are characteristic functions of the geomorphic agents Erosion, Transportation and Depositional works 16. William Morris Devis has recognised the stages involves in a cycle of river erosion are Initial stage, Youth stage, Mature stage and Old stage 17. In which stage of erosional cycle maximum changes occur Mature stage 18. Waterfalls and Gorges are characteristic features of the river in Initial stage 19. Pot holes are formed generally by Abrassion or Corrosion 20. Chemical erosion by river water is known as Corrosion 21. The transportation by Creeping and Rolling is known as Traction 22. The plain land produced by the river action is Peneplain 23. Deltas are formed in Old Stage of the River 24. The transportation through lifts and falls of materials is known as Saltation 25. Which type of drainage pattern develops in folded or tilted beds Trellis pattern 26. Canyon is A deep valley with steep near vertical sides 27. Pass is An opening between the Mountains 28. Aeolian topography is created by the geological action of Wind 29. Yardang topography associated with Wind action 30. Which instrument is used to measure the wind velocity Anemometer 31. Loess is Homogeneous and unstratified deposit of silt 32. A Crescent shaped dune with two tapering arms is known as Barchan 33. Wind ripples are generally formed by Saltation movement of sand grains 34. A normal sand-dune is characterised by Gentle windward and Steeper leeward sides 35. Blow-outs are Broad shallow depression in desserts 36. When one wing of a Barchan is missing, then it is known as Seif 37. The space between the dunes is known as Gassis 38. Chinook is a local hot wind which flows mostly in USA and Canada 39. Which abrasion is more effective in rounding the sand grains Wind abrasion 40. “Lag-Gravel” is The coarse sediments left behind where wind has removes the finer grain sizes 41. Glaciers are formed by Compaction and Re-crystallisation of snow 42. Ne’ve’ or Flrn are Granular ice mass 43. The polar glaciers are Below the freezing point throughout the year 44. The Karst topography developed due to the action of Groundwater 45. Which process is mainly responsible for development of the Karst topography Chemical Process 46. The columns of limestone that hang from the ceiling downwards are known as Stalactites 47. Stalagmites are Rising up vertically from the floor of the cavern 48. Blind valleys are found in Karst topography 49. Drip-stones are Columns formed by joining of Stalactite and Stalagmite 50. ‘Terra rossa’ is Residual red soil occurring on limestone in Karst region 51. The difference between lake and basin is Lakes commonly occur above the mean-sea level while basins have their bottoms below the water table. 52. Dhands are Small lakes of Aeolian origin 53. A narrow strip of water joining the two water bodies is called Strait 54. The ocean which is between Africa and Australia Indian Ocean Crystallography & Mineralogy : 55. The faces, edges and solid angles have a definite relationship with each other. This relationship is expressed by Euler’s formula 56. Euler’s formula is F+A = E+2 57. Centre of symmetry is Repetition is with respect to a point 58. Axis of Symmetry Repetition is with respect to a line 59. Plane of Symmetry Repetition is with respect to a plane 60. Which instrument is used to measure the interfacial-angle of crystals Goniometer 61. Which crystal system is having maximum of classes Hexagonal system 62. In which crystal system majority of minerals crystallises Monoclinic system 63. The Isometric system is characterised by 4 axes of 3 fold symmetry 64. What is the normal interfacial angle in dodecahedron form of cubic system 600 65. Gyroidal class belongs to Isometric system 66. The cleavages in twinned crystals are In different directions 67. Butterfly twinning is seen in Gypsum 68. Pericline twin is found in Plagioclase 69. The degree of transparency of a mineral is known as Diaphaneity 70. Give an example of a mineral in which cleavage is absent Quartz, Corundum 71. Hardness of human nail varies between 1.5 to 2.5 72. Hardness is which kind of property Anisotropic (A mineral may show different values in different directions.) 73. The tendency of a crystallized mineral to break along certain directions yielding more or less smooth, plane surface is Cleavage 74. The behaviour of a mineral towards the forces that tend to destroy it is called Tenacity 75. A Mineral is Naturally occurring inorganic substance with definite chemical composition 76. The external appearance of a Mineral is known as Habit/ Form 77. The powered form of a mineral is Streak 78. Shining property of a Mineral is Lustre 79. Form in which neither a crystal face nor a cleavage is seen in Amorphous 80. Quartz shows which lustre Vitreous 81. Which mineral shows silky lustre Asbestos, Gypsum 82. Mica is Flexible and elastic 83. Kyanite shows which form Bladed 84. Structure or form which depicts leaf like sheets is Lamellar 85. Muscovite mica shows which structure Foliated 86. Which form resembles human kidney Reniform 87. chromite shows which type of structure Granular 88. Colour changing phenomenon which involves oxidation is Tarnish (A phenomenon of change of original colours of minerals to some secondary colours at its surface due to oxidation at the surface) 89. Diamond shows which type of lustre Adamantine 90. Streak is an important diagnostic property of Coloured minerals 91. Generally which minerals give streak Coloured and opaque 92. Hardness of a mineral depends upon Chemical composition and atomic constitution 93. The scale of hardness is Mohs (It was in 1822 that Austrian mineralogist F. Mohs proposed a relative, broadly quantitative “scale of hardness” of minerals assigning values between 1 to 10) 94. Which mineral group is abundantly found in the Earth’s crust Feldspar group (Second abundant is Silicate or Quartz group) 95. Feldspar is found majority in which kind of rock Igneous rocks 96. Acicular habit shown in Natrolite 97. Violet colour of Amethyst is due to MnO2 98. The mineral which can be cut and powdered are known as Sectile 99. Opaque minerals indicate their origin from a Rapidly cooled silicate melt 100. Orpiment and Realgar are Sulphides of Arsenic Optical Mineralogy : 101. In natural light, the elctro-magnetic vibrations are: Always perpendicular to the direction of light-wave prorogation 102. Refractive index depends upon Nature of the substance and Kind of light used 103. The refractive index of Canada balsam is 1.54 104. Plane polarised light can be produced by Nicol prism, Reflections, Absorption 105. Backe-line method is used to determine the Refractive index 106. Which property determines the colour Wavelength 107. The wavelength varies from slightly more than …. at the red end to about …. at the violet end 7000 Å and 4000 Å respecively 108. The isotropic substance has A single refractive index 109. Double refraction phenomenon shown by Anisotropic substance 110. The angle between the reflected and refracted ray is 900, stated by Brewster’s law 111. Which crystal systems are optically uniaxial Hexagonal and Tetragonal 112. Which crystal systems have two optic axis Orthorhombic, Monoclinic and Triclinic 113. Uniaxial crystal are positive if Ordinary ray has the greater velocity than the Extraordinary ray 114. The difference between the maximum and minimum indices of a particular mineral is known as Birefringence 115. What is the birefringence of Quartz mineral 0.009 116. Birefringence is used to determine Thickness of section 117. Bereck compensator is an optical device which is made up of Calcite 118. The order of interference colour is determined by Quartz plate 119. A Polaroid is a Light filter 120. Biaxial minerals show Symmetrical extinction 121. Complete extinction occurs only when the section is Perpendicular to optic symmetry 122. Which is also known as Glimmer plate Gypsum plate 123. Amorphous substance are Anisotropic 124. Orthoclase is distinguished from Quartz in thin section by Low refractive index, Type of twinning and Negative sign 125. The Michael-Levy method is used to determine the extinction angle of Plagioclase 126. Which plate is generally used to determine the optical sign of plagioclase Selenite plate 127. The Orthopyroxenes show interference colour of 1st order 128. Which type of extinction is often shown by Quartz mineral Wavy 129. Calcite is characterised by Rhombohedral cleavage, low interference colour and optically negative character 130. Calcite and Magnesite can be distinguished from one another in thin section Magnesite is never twinned GEOLOGY Lab Viva Questions and Answers Pdf Download :: Read the full article
0 notes
Photo

Optical Properties
TA.TIL Optical Properties of Materials
First, let’s define the term optical property. An optical property of a material relates to how that material responds when exposed to electromagnetic radiation. The electromagnetic spectrum spans from gamma rays of wavelengths around 10-3 nm through x-rays, ultraviolet, visible, infrared and radio waves around 105 m. The visible spectrum, what we see every day, is between 0.4 micrometers and 0.7 micrometers with various colors corresponding to specific wavelengths. For example 0.4 micrometers is violet, 0.5 micrometers is green, and 0.65 micrometers is red.
Electromagnetic radiation can be considered two ways. The first is to consider it being wavelike and consisting of electric and magnetic field components that are perpendicular to each other and the direction of propagation. The second way to view electromagnetic radiation is from a quantum-mechanical perspective where it is composed of groups of energy called photons. This second method of evaluation relies heavily on the energy of a photon having specific values, being proportional to the frequency of the radiation or inversely proportional to the wavelength.
When this light, or radiation, travels from one medium to another (like air onto a solid) several things can happen. Light can be transmitted through the medium. It can also be absorbed by the medium or reflected at the interface between the two mediums. The intensity of the light beam that touches the surface of a solid medium must equal the sum of the intensities of the transmitted, absorbed, and reflected beams
There are various ways to describe materials based on how they respond to the light beam touching their surface. Transparent materials can transmit light with relatively little absorption and reflection. Translucent materials allow light to be transmitted diffusely, meaning the light is scattered within the interior so that objects are not distinguishable when viewed through the material. Opaque materials are impervious to the transmission of visible light and are therefore not see-through.
Some types of materials have typical traits regarding how they respond to light. All bulk metals are opaque through the entire visible spectrum meaning that all light radiation is either absorbed or reflected. Metals are opaque to all electromagnetic radiation on the low end of the frequency spectrum. This includes radio, infrared, visible and some ultra violet. However, metals are transparent for high frequency x ray and gamma ray radiation. Due to their opaqueness and high reflectivity, the perceived color of metals is determined by the wavelength distribution of the radiation that is reflected from it, not absorbed into it. Therefore, when a metal is exposed to a white light and it appears bright and silvery, it is a highly reflective metal over the entire range of the visible spectrum. Examples of this would be aluminum and silver. Copper and gold however, are red-orange and yellow in appearance. This occurs since the reflected wavelengths from these metals are the red-orange and yellow wavelengths. Nonmetal materials however, can be transparent in visible light as well as have characteristics of reflection, absorption, refraction, and transmission.
Refraction occurs when the light transmitted into the transparent material experiences a decrease in velocity and is bent at the interface. The index of refraction of a material is defined as the ratio of the velocity in a vacuum to the velocity within the medium. The magnitude of the index of refraction refers to the degree of bending and depends on the wavelength of the light. Due to each color wavelength being deflected by a different amount as the light beam goes into and out of the glass, the colors are separated from the white light in the glass prism.
Reflection occurs when light radiation passes from 1 medium to another with a different index of refraction and some light is scattered at the interface between the two media. This can occur even if both media are transparent. Since the index of refraction of air is almost unity, the higher the index of refraction of the solid, the greater the reflectivity. Reflection losses for lenses and other optical instruments are minimized by coating and reflecting the surface with very thin layers of dielectric materials.
Absorption occurs when a material captures and holds onto the energy from a radiation beam. A photon of light may be absorbed by the promotion or excitation of an electron from the nearly filled valence band across the band photon frequency.
Transmission is considered the fraction of incident light that is transmitted through the transparent material and depends on the losses that are incurred by absorption and reflection.
The color of materials is a direct relation to those wavelengths that the material absorbed. Materials that absorb all of the visible wavelengths will appear colorless such as high purity inorganic glasses, diamonds, crystal, and sapphire.
Opacity and translucency of insulators depends heavily on their internal reflectance and transmittance characteristics. Many of these materials that are intrinsically transparent may be made translucent or opaque due to interior reflection and refraction. The transmitted light beam is deflected in a direction that appears diffuse and multiple scattering events. Opacity results when scattering is so extensive that none of the incident beam is transmitted, undeflected, to the back surface. This internal scattering can result from a few sources
· Materials with anisotropic index of refractions like polycrystalline specimens
· Reflection and refraction occurring at grain boundaries due to the difference in index of refraction between the adjacent grains
· Two phase materials where the beam dispersion occurs across the phase boundaries due to different refraction indexes
· Pores within fabricated ceramics which effectively scatter light radiation
There are a few other optical properties of importance when evaluating material characteristics. This includes luminescence and photoconductivity which are important reactions that can occur when light reaches the material surface and causes a reaction.
Luminescence is the ability of some materials to absorb energy and reemit a visible light. This occurs due to the reaction between photon and electron energy levels. If the reemission of light occurs within 1 second, the phenomenon is termed fluorescence. If it is longer than 1 second it is then called phosphorescence. Many materials can be made fluorescence or phosphorescence though pure materials typically do not display these phenomena’s.
For photoconductivity, let’s first evaluate the conductivity of semiconducting materials. This conductivity depends on the number of free electrons in the conduction band and the number of available spaces in the valence band. Thermal energy within the lattice vibrations can promote electron excitation when then frees electrons and creates more available spaces within the bands. Photoconductivity occurs when light is absorbed and the conductivity increases within the material due to the photons of the light inducing electron movement and transitions.
#electromagnetic#radiation#wavelengths#optical properties#optics#optical#electromagnetic radiation#material#material color#color#color property
0 notes