#microfluidics technology
Explore tagged Tumblr posts
Text
What Is Microfluidics? An Introduction to Lab-on-Chip Technology
Discover how microfluidics and Lab-on-Chip technology are revolutionising diagnostics, research, and environmental monitoring. Learn about their precision, cost-effectiveness, and applications in healthcare, genomics, and food safety. Bring innovation to your business with cutting-edge solutions today.
#microfluidics technology#lab-on-chip devices#portable diagnostics#microfluidic applications UK#lab-on-chip healthcare#microfluidics environmental monitoring#microfluidics DNA analysis#microfluidic cost-effective testing#advanced lab-on-chip solutions#microfluidics sustainability#microfluidic drug development#LOC portable testing devices#A-Gas Electronic Materials
0 notes
Text
youtube
#implantable drug delivery system#on-demand therapy#digital electronics-free devices#biocompatible materials#localized drug delivery#shape-memory alloys#hydrogel technology#microfluidics#smart materials#chronic pain management#cancer treatment innovation#personalized medicine#non-electronic implants#biofeedback loops#sustainable healthcare#precision drug delivery#hormonal therapies#advanced medical devices#therapeutic innovation#future of medicine#Youtube
0 notes
Link
#bloodtesting#diagnostics#healthcare#innovation#technology#POCT#moleculardiagnostics#digitalpathology#microfluidics#automation#precisionmedicine#healthcareaccess#globalheal...
0 notes
Text
The Science of Lab on a Chip
By Arjuwan Lakkdawala
Ink in the Internet
Science nowadays looks so much like The Jetsons cartoon, where lots of technology would be folded into a little chip or box. The technological leaps have been phenomenal, and what makes this more fascinating in this era, than past breakthrough discoveries, is that the Internet has given us a front row seat of the entire show as it unfolds.
That is not all, we are so connected that it's possible to contribute progressive ideas to science or any project or situation. There is always someone reading and posts go viral faster than we can say cheese.
While this attribute of the Internet makes it very entertaining. There is a serious aspect to the science itself. The aspect of saving lives. The scientists of the world are heroes, innovating not only to progress science but also to invent impactful devices and medication methods in developing countries, we in the privileged first world countries may not think often about the suffering of third world countries. But the elites in academia have made them a priority. Still, despite these efforts there are several issues in getting the proper aid to those countries.
In African regions there is the problem of counterfeit medicines that cause annually thousands of deaths. So a messaging system to scan the barcodes on the medication that could be sent to a website for verification was developed.
Another crisis in developing countries is that women often die during childbirth from blood loss. Due to lack of available medical equipment and blood donors, the same blood lost would be transfused back into the patient using kitchen things and guize to filter the blood - which done in this way is unhygienic and dangerous. For this the Sisu Global Health developed the Hemafuse for hygienic and steril blood transfusion.
Other inventions like 3D printers are also of significant impact in developing countries. Some examples for instance include; printing prosthetics, setting up field hospitals, or printing a lab-in-a-box.
In this regard is one very interesting invention, which is the lab-on-a-chip. It may sound impossible for a whole lab to be on a chip that can be anywhere from the size of your fingernail to a few inches. This product hasn't been industrialised but it is being used in several medical settings, and constant improved models are in the works.
As technology advances devices are getting miniature and miniature. This is to make it conveniently possible to pack more technology in smaller spaces. But other benefits as scientists emphasis is that it reduces human error. This is more crucial in the medical field than anywhere else.
So how are devices getting so miniature that we can have a lab-on-a-chip?
How did they do it. How does it work. I researched these questions and the answer is complex science, but I will give a detailed overview.
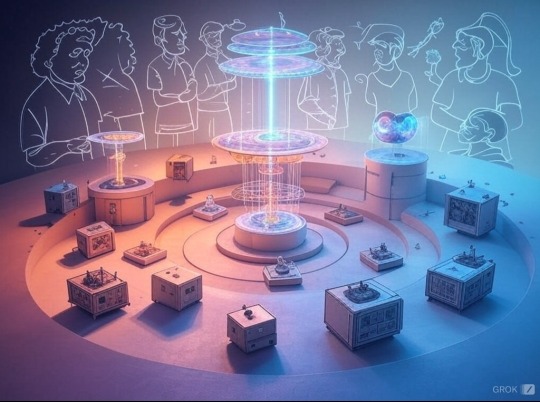
Let's start with the science of chips (not the eating ones) a computer chip is the brain of every type of artificial intelligence. Chips are made mostly from silicon (hence the name Silicon Valley.)
Silicon is made from sand and it's the most abundant natural resource on earth after oxygen.
As we know computers started very large and got smaller, now we have handheld devices that are so slick they are practically flat, and are packed with compute power and huge memory storage.
This is mainly the magic of microchips and transistors. As chips got smaller so did transistors, and they are what gives the chips their compute power. In a powerful optimized chip the size of your fingernail there could be 50 billion transistors.
Transistors have shrunk to the size of atoms.
(Basically the size of atoms, I did extensive research to understand this, and this is what I make of it. The "transistor" is simply layers of silicone, no wires, or hardware, then atoms with an electric charge (ions) are split into negative and positive charged ones known as cations (positive) and anions (negative) and these are fired into the silicone layers, changing their atomic composition, and hence creating an electrical circuit the size of atoms without wires. We know atoms are so small we cannot see them even with a microscope, and this is how devices are getting miniature and compute power is getting bigger. A single transistor circuit can hold two stages of the binary commands by which computer software is programmed. That is 1 and 0. So 50 billion transistors can hold a very large memory capacity and compute power. An electrical current by battery or a direct powerline activates the transistor configuration of postive and negative atoms, which are controlled by the three layers of each transistor.)
The next question is how is it possible for machines to engineer at such nano and microscales. This is the great secret behind such technological leaps - I like to give it a layman term 'light engineering,' and not so much a layman term 'chemical engineering.'
The silicone used in chips and the materials (polymers) used in lab-on-a-chip are modified chemically to be sensitive to different wavelengths of light and even waves of sound, and these techniques are used in a major part of such miniature engineering.
A chip starts basically as an ingot of silicone, then it is sliced into strips technically called 'wafers' this is coated with photo sensitive material, and then it is put in a lithography machine that has a blueprint known as the 'mask' that gets imprinted on it. This is repeated on layers of silicone; building an electrical circuit for the transistors. Then atoms of different charges are blasted in the electrical patterns made on the silicone. This process takes place in a clean room, dust is hazardous to it for it can damage the chips.
After a set of other modifications the chips are tested and ready to be used in devices. Depending on the tech used in the manufacturing, there could be a few billion to 50 billion transistors nearly the size of atoms in the chip.
The material widely used in lab-on-a-chip are polymers, and they are fabricated with the technology of microfluidics. This means that the lab-chips will have microchannels and chambers where test fluids will be pushed through or stored as the tests go on. Fluids at such microscales behave very differently than what we are used to in observations in large volumes. The science of microfluidics explores and takes advantage of the change in fluid dynamics of the microscale. The microchannels and chambers are etched on the chip using lithography - the same technology used in manufacturing microchips and transistors.
Those lab-chips could have high-tech analytical tools like spectrometers and gas chromography attached to them, and in built pressure systems to move the fluids.
There are also organs-on-a-chip, these mimic microenvironments and the effect on specific organ micro simulation, and are more precise than traditional culture tests. Lab-chips are said to be faster than normal conventional labs, cost much less, reduce human error, and being portable can be of significant impact were medical care is needed but a lab or hospital is not possible.
Lab-on-a-chip are used in blood tests, diagnostics of bacteria and viruses, DNA/RNA and HIV tests, and more applications are being developed.
Micro technology had a revolution in the medical field in the 70s, described as "scientists first had only a street light, where testing was not possible of more than a single gnome at a time, and then we had a floodlight."
This happened after scientific methods of studying atoms in physics was applied to molecular biology, and then chemical engineering branched out into biochemical and biomedical engineering. Scientists made technological breakthroughs and discovered that the human body worked like a very high-tech sophisticated biological machine, and it was possible to apply engineering methods to it - resulting in the science of biochemical engineering and systems biology, etc. Scientists started to look for ways to merge biology with mechanical engineering like prosthetics, artificial organs, and so on.
Arjuwan Lakkdawala is an author and independent researcher in science.
X/Twitter/Instagram: Spellrainia Email: [email protected]
Copyright ©️ Arjuwan Lakkdawala 2024
Sources:
Yale School of Medicine, Yale Medicine Magazine - human body as machine
AIChe, The Global Home of Chemical Engineers - chemical engineers are advancing biomedicine
University of Alberta, Faculty of Engineering, Department of Chemical and Materials Engineering - biochemical and biomedical engineering
ElveFlow, An ELVESYS Brand - introduction to lab-on-a-chip
ASML, Our Technology, lithography - using light to print tiny patterns on silicon - is a fundamental step in mass producing microchips
News-Medical, Life Sciences - what us microfluidics? by susha cheriyedath, M.Sc., reviewed by Afsaneh khetrapal BSc
ElveFlow, An ELVESYS Brand - definition of microfluidics
Semiconductor Engineering, Deep Insights for the Tech Industry - understanding memory by Alex yoon
Homepage.cs.uri.edu - how computers work the CPU and memory
Royal Society of Chemistry - microfluidic diagnostics for the developing world, xiaole mao and tony jun huang
The Borgen Project, blog latest news - health technologies for developing countries
University Wafer - how to fabricate a computer chip from silicon
Nano Werk - transistors explained - what they are and what they do
#Arjuwan Lakkdawala#ink in the Internet#developing countries#lab on a chip#microfluidics#silicone#microchips#transistors#chemical engineering#lithography#science#technology#health
0 notes
Text
How is Asia-Pacific Shaping the Future of the Microfluidics Market? Exploring Strategies, Innovations, and Key Developments

Introduction
The Microfluidics Market is experiencing significant growth worldwide, with the Asia-Pacific region showing a steady rise due to several critical factors. The increasing prevalence of chronic diseases, particularly chronic obstructive pulmonary disease (COPD), alongside a growing emphasis on healthcare innovation, is driving this expansion. According to the World Health Organization (WHO), China alone accounts for 100 million people living with COPD, representing 25% of all COPD cases globally. This article delves into the factors behind Asia-Pacific's emergence as a key player in the microfluidics market, highlighting strategies, innovations, and developments that are shaping the future of this dynamic sector.
Download FREE Sample : https://www.nextmsc.com/microfluidics-market/request-sample
Asia-Pacific’s Strategic Edge in Microfluidics
High Prevalence of Chronic Diseases: The Asia-Pacific region faces a high burden of chronic diseases, including COPD, diabetes, and cardiovascular conditions. The need for effective diagnostics and treatment solutions is driving the demand for microfluidic technologies, which offer rapid, accurate, and cost-effective testing and monitoring.
Healthcare Infrastructure Development: Many countries in Asia-Pacific are investing heavily in healthcare infrastructure to improve accessibility and quality of care. Governments and private sectors are collaborating to build advanced healthcare facilities equipped with the latest technologies, including microfluidic devices.
Technological Advancements and Innovation: The region is witnessing rapid advancements in microfluidic technologies, driven by a strong emphasis on research and development. Universities, research institutions, and companies are working together to innovate and commercialize new microfluidic applications.
Supportive Government Policies: Several Asia-Pacific countries have implemented policies that support the growth of the biotechnology and medical device industries. These policies include funding for research, tax incentives for technology development, and streamlined regulatory processes for new medical technologies.
Emerging Innovations in Microfluidics Technology
Point-of-Care Diagnostics: One of the most significant innovations in the microfluidics market is the development of point-of-care diagnostic devices. These portable and easy-to-use devices enable healthcare providers to conduct rapid tests at the patient’s location, improving the speed and accuracy of diagnosis and treatment.
Lab-on-a-Chip Devices: Lab-on-a-chip technology is revolutionizing the field by integrating multiple laboratory functions onto a single chip. These devices can perform complex analyses with minimal sample volumes and faster processing times, making them ideal for applications in diagnostics, drug development, and environmental monitoring.
Personalized Medicine: Microfluidic devices are playing a crucial role in the advancement of personalized medicine. These devices enable the analysis of individual patient samples to tailor treatments based on specific genetic, biochemical, and physiological characteristics, improving treatment efficacy and patient outcomes.
Integration with Digital Health: The integration of microfluidics with digital health technologies, such as mobile health applications and telemedicine platforms, is enhancing the utility and accessibility of these devices. Digital health solutions facilitate remote monitoring, data collection, and real-time analysis, supporting better patient management and care.
Inquire before buying : https://www.nextmsc.com/microfluidics-market/inquire-before-buying
Key Developments in the Microfluidics Market
Expansion of Manufacturing Capabilities: To meet the growing demand for microfluidic devices, companies in Asia-Pacific are expanding their manufacturing capabilities. This includes building new production facilities and scaling up existing ones to increase output and reduce costs.
Strategic Collaborations and Partnerships: Collaborations between companies, research institutions, and healthcare providers are driving innovation and commercialization in the microfluidics market. These partnerships enable knowledge sharing, resource pooling, and accelerated development of new technologies.
Regulatory Approvals and Standards: Obtaining regulatory approvals and adhering to international standards are critical for the successful commercialization of microfluidic devices. Asia-Pacific countries are working towards harmonizing their regulatory frameworks with global standards to facilitate market entry and adoption of new technologies.
Commercialization of New Products: The continuous launch of new and advanced microfluidic devices is propelling market growth. Companies are introducing products that address specific healthcare needs, such as rapid COVID-19 testing kits, cancer diagnostics, and personalized drug delivery systems.
Impact on Global Healthcare and Diagnostics
Enhanced Diagnostic Capabilities: Microfluidic devices offer enhanced diagnostic capabilities by providing rapid, accurate, and cost-effective analyses. These devices can detect diseases at early stages, monitor health conditions, and provide real-time data, improving patient outcomes and reducing healthcare costs.
Accelerated Drug Development: Microfluidics is playing a vital role in drug development by enabling high-throughput screening, toxicity testing, and personalized drug formulation. This accelerates the drug development process and reduces costs, bringing new therapies to market faster.
Improved Access to Healthcare: The portability and ease of use of microfluidic devices enhance access to healthcare, particularly in remote and underserved areas. Point-of-care testing devices can be used in various settings, from rural clinics to emergency rooms, ensuring timely diagnosis and treatment.
Future Outlook and Strategic Imperatives
Looking ahead, Asia-Pacific’s growth in the microfluidics market is poised to continue, driven by several strategic imperatives:
Continued Investment in Research and Development: Ongoing investment in R&D is crucial for advancing microfluidic technologies. Companies and research institutions must focus on developing next-generation devices that offer greater accuracy, faster processing times, and broader applications.
Expansion of Applications: The potential applications of microfluidics extend beyond healthcare and diagnostics to fields such as environmental monitoring, food safety, and industrial processes. Expanding the use of microfluidic technologies in these areas can drive market growth and innovation.
Regulatory Support and Compliance: Ensuring compliance with regulatory standards is essential for the successful commercialization of microfluidic devices. Companies must navigate regulatory requirements to ensure the safety and efficacy of their products, gaining the trust of healthcare providers and patients.
Leveraging Digital Health Platforms: The integration of microfluidics with digital health platforms can enhance the utility and accessibility of these devices. Digital platforms can facilitate remote monitoring, telemedicine, and data analytics, providing comprehensive healthcare solutions.
Conclusion
Asia-Pacific's emergence as a dominant player in the microfluidics market is driven by a high prevalence of chronic diseases, significant healthcare investments, and a strong focus on technological innovation. The region's commitment to advancing microfluidic technologies is transforming healthcare and diagnostics, improving patient outcomes, and reducing costs. As the demand for rapid, accurate, and personalized healthcare solutions continues to grow, Asia-Pacific’s leadership in the microfluidics market is poised to strengthen, paving the way for a future where microfluidic devices play a pivotal role in global healthcare.
#microfluidics#healthcare#life science#market research#business insights#market analysis#innovation#medical technology
0 notes
Text
Miniaturizing Innovation: Exploring the Microfluidics Market
In the realm of life sciences and healthcare, the Microfluidics market is emerging as a transformative force, enabling researchers and clinicians to miniaturize laboratory processes and revolutionize diagnostics and therapeutics. This article delves into the burgeoning field of microfluidics, its applications in biomedical research and diagnostics, and its potential to reshape the healthcare landscape.
Microfluidics, the science of manipulating fluids at the microscale, offers a versatile platform for a wide range of applications, from drug discovery and genomics to point-of-care diagnostics and personalized medicine. By leveraging the unique physics and mechanics of fluid flow at small scales, microfluidic devices enable precise control over sample volumes, reaction kinetics, and experimental workflows.
The Microfluidics market encompasses an extensive array of technologies, including lab-on-a-chip devices, microfluidic pumps, valves, and sensors, as well as integrated systems for automated sample preparation and analysis. These miniaturized platforms offer numerous advantages over traditional laboratory techniques, including reduced sample and reagent consumption, faster analysis times, and increased sensitivity and throughput.
One of the key drivers propelling the growth of the Microfluidics market is the increasing demand for point-of-care diagnostics and personalized medicine. Microfluidic devices enable rapid and cost-effective analysis of biological samples, allowing for early detection of diseases, monitoring of treatment efficacy, and tailoring of therapies to individual patient needs.
In addition to healthcare applications, microfluidic technologies are transforming the landscape of biomedical research, enabling researchers to conduct experiments with unprecedented precision and scalability. From studying cellular dynamics and microorganisms to exploring complex biological phenomena, microfluidic platforms offer insights that were previously inaccessible using conventional laboratory techniques.
Moreover, the integration of microfluidics with other emerging technologies, such as artificial intelligence (AI) and advanced imaging techniques, is expanding the horizons of biomedical research and diagnostics. By combining microfluidic devices with AI-powered analytics and high-resolution imaging systems, researchers can extract valuable insights from complex biological data with unprecedented speed and accuracy.
Want to learn more? Get Sample PDF Copy
As the Microfluidics market continues to evolve, collaboration between academia, industry, and healthcare providers will be essential to realize its full potential. Addressing challenges such as standardization, scalability, and regulatory compliance will be crucial to accelerating the adoption of microfluidic technologies across diverse applications and settings.
In conclusion, the Microfluidics market represents a paradigm shift in biomedical research and diagnostics, offering a powerful platform for innovation and discovery. With its ability to miniaturize laboratory processes, enhance analytical capabilities, and enable personalized healthcare solutions, microfluidic technology is poised to shape the future of medicine and healthcare delivery, driving advances that benefit patients, researchers, and clinicians alike.
#Microfluidics#Biomedical Research#Lab-on-a-chip#Point-of-care Diagnostics#Life Sciences#Healthcare Technology
0 notes
Text
Advanced Microfluidics and Organ-On-Chip Technology
0 notes
Text
Microfluidics in Medicine
In the late 1990s and early 2000s, the Human Genome Project spent years decoding DNA from a handful of donors. The work was painstaking and slow, given DNA sequencing technology of the time. (Image credit: CDC; see also The Scientist; submitted by Marc A.) Read the full article
56 notes
·
View notes
Text
Microfluidic technology has become increasingly important in many scientific fields such as regenerative medicine, microelectronics, and environmental science. However, conventional microfabrication techniques face limitations in scale and in the construction of complex networks. These hurdles are compounded when it comes to building more intricate 3D microfluidic networks. Now, researchers from Kyushu University have developed a new and convenient technique for building such complex 3D microfluidic networks. Their tool? Plants and fungi. The team developed a 'soil' medium using nanoparticles of glass (silica) and a cellulose based binding agent, then allowed plants and fungi to grow roots into it. After the plants were removed, the glass was left with a complex 3D microfluidic network of micrometer-sized hollow holes where the roots once were.
Read more.
#Materials Science#Science#Microfluidics#Biomaterials#Plants#Fungi#Sintering#Glass#Kyushu University
11 notes
·
View notes
Text
Rather than merely integrating biological concepts into computing, FinalSpark's online platform 'taps' into spherical clusters of lab-grown human brain cells called organoids. A total of 16 organoids are housed within four arrays that connect to eight electrodes each and a microfluidics system that supplies water and nutrients for the cells. The approach, known as wetware computing, in this case harnesses researchers' abilities to culture organoids in the lab, a fairly new technology that allows scientists to study what are essentially mini replicas of individual organs… While we don't have any numbers on their specific system, its energy usage, or processing power, FinalSpark's research team says that training a single large language model like GPT-3, a precursor to GPT-4, required 10 gigawatt hours or about 6,000 times the energy that one European citizen uses in a year. Meanwhile, the human brain operates its 86 billion neurons using only a fraction of that energy: just 0.3 kilowatt hours per day. Technology trends also indicate that the booming AI industry will consume 3.5 percent of global electricity by 2030. Already, the IT industry as a whole is responsible for around 2 percent of global CO2 emissions.
Looks like the "brains" are grown from stem cells and can live for about 100 days. I'm skeptical something like this could be scaled up such that everyone starts using biocomputers in like cell phones and at home, but at least people are experimenting with trying to find less energy-intensive systems?
8 notes
·
View notes
Text
Annie Jacobsen on Joe Rogan Podcast.
She wrote a book about the Area 51. In there an insider says that the roswell spaceship was made by the soviets, using technology from Nazi Germany, and the aliens were actually mutated teenagers (i dont recall how, i think they do not touch that in the book), based on the Mengele's body of research.
This the set up - The War of the worlds radio incident (exaggerated or not, the outside perception of it is what mattered). In Europe and Asia everybody was amazed. The brave americans scared by little men from mars??? How could they believe such nonsense??? The soviets developed a strategy based on that, they wanted to strike terror using a literal alien invasion, or the perception of it, but technology did not allowed them to. So, in the last days of WW2, the americans got Team Rocket, and the soviets got Team Electromagnetic. Josef Mengele wanted to move to USSR and traded his research with the Soviets. The Soviets said"Thank you. Fuck off." And things took off from there. It is a very bland book up to the insider's revelations.

48 notes
·
View notes
Text
High-Transparency Ostemer 322 Crystal Clear Polymer for Microfluidics and MEMS Applications
Discover Ostemer 322 Crystal Clear, a dual cure polymer with exceptional transparency and bonding capabilities. Ideal for microfluidic cartridges, MEMS antennas, and rapid prototyping, this material ensures high precision and reliability in advanced applications. Contact A-Gas Electronic Materials for expert solutions.
#Ostemer 322 Crystal Clear UK#dual cure polymer UK#microfluidic cartridges#MEMS antennas#hybrid material integration UK#UV cure polymer UK#heat cure polymer UK#microfluidics#rapid prototyping#A-Gas Electronic Materials#high transparency polymer UK#lab-on-chip technology UK
0 notes
Text

Driving the Tube
Applying advanced microfluidics technology, reveals the role of cells' 'handedness' – a bias for direction of growth – in vessel development
Read the published research article here
Image from work by Haokang Zhang and colleagues
Department of Biomedical Engineering, Rensselaer Polytechnic Institute, Troy, NY, USA
Image originally published with a Creative Commons Attribution 4.0 International (CC BY 4.0)
Published in Science Advances, February 2024
You can also follow BPoD on Instagram, Twitter and Facebook
#science#biomedicine#immunofluorescence#biology#chirality#vascular#vessels#microfluidics#bioengineering
12 notes
·
View notes
Link
#bloodtesting#diagnostics#healthcare#innovation#technology#POCT#moleculardiagnostics#digitalpathology#microfluidics#automation#precisionmedicine#healthcareaccess#globalheal...
0 notes
Text
DNA technology now offers both data storage and computing functions for the first time
- By Nuadox Crew -
Researchers from North Carolina State University and Johns Hopkins University have developed a technology that uses DNA for both data storage and computing functions, enabling operations like storing, retrieving, erasing, and rewriting data, which was previously impossible with DNA-based systems.
Led by Albert Keung, the project aims to overcome challenges in integrating storage and processing, common in conventional computing, within a DNA framework.
The breakthrough relies on soft polymer structures called dendricolloids, which support high-density DNA storage. This structure allows for key functions like copying, erasing, and rewriting DNA data without damaging it. The team successfully solved basic computational problems with this technology, demonstrating its potential to store massive amounts of data securely for long periods.
The system, dubbed a “primordial DNA store and compute engine,” is a collaborative effort involving contributions from experts in microfluidics, sequencing, and data algorithms. Researchers hope this advancement will inspire future work in molecular computing, much like early computers sparked the digital age.
The research, published in Nature Nanotechnology, was funded by the National Science Foundation (NSF), with some researchers holding interests in commercializing DNA-based systems.
Read more at NC State University
Scientific paper: Lin, K.N., Volkel, K., Cao, C. et al. A primordial DNA store and compute engine. Nat. Nanotechnol. (2024). https://doi.org/10.1038/s41565-024-01771-6
--
Other recent news
Microscopy Breakthrough: Scientists at the Fritz Haber Institute of the Max Planck Society have developed a revolutionary microscopy technique that unveils hidden worlds. This could significantly advance our understanding of microscopic structures.
3 notes
·
View notes
Text
Scientists 3D Print Self-Heating Microfluidic Devices - Technology Org
New Post has been published on https://thedigitalinsider.com/scientists-3d-print-self-heating-microfluidic-devices-technology-org/
Scientists 3D Print Self-Heating Microfluidic Devices - Technology Org
The one-step fabrication process rapidly produces miniature chemical reactors that could be used to detect diseases or analyze substances.
MIT researchers have used 3D printing to produce self-heating microfluidic devices, demonstrating a technique which could someday be used to rapidly create cheap, yet accurate, tools to detect a host of diseases.
MIT researchers developed a fabrication process to produce self-heating microfluidic devices in one step using a multi-material 3D printer. Pictured is an example of one of the devices. Illustration by the researchers / MIT
Microfluidics, miniaturized machines that manipulate fluids and facilitate chemical reactions, can be used to detect disease in tiny samples of blood or fluids. At-home test kits for Covid-19, for example, incorporate a simple type of microfluidic.
But many microfluidic applications require chemical reactions that must be performed at specific temperatures.
These more complex microfluidic devices, which are typically manufactured in a clean room, are outfitted with heating elements made from gold or platinum using a complicated and expensive fabrication process that is difficult to scale up.
Instead, the MIT team used multimaterial 3D printing to create self-heating microfluidic devices with built-in heating elements, through a single, inexpensive manufacturing process. They generated devices that can heat fluid to a specific temperature as it flows through microscopic channels inside the tiny machine.
The self-heating microfluidic devices, such as the one shown, can be made rapidly and cheaply in large numbers, and could someday help clinicians in remote parts of the world detect diseases without the need for expensive lab equipment. Credits: Courtesy of the researchers / MIT
Their technique is customizable, so an engineer could create a microfluidic that heats fluid to a certain temperature or given heating profile within a specific area of the device. The low-cost fabrication process requires about $2 of materials to generate a ready-to-use microfluidic.
The process could be especially useful in creating self-heating microfluidics for remote regions of developing countries where clinicians may not have access to the expensive lab equipment required for many diagnostic procedures.
“Clean rooms in particular, where you would usually make these devices, are incredibly expensive to build and to run. But we can make very capable self-heating microfluidic devices using additive manufacturing, and they can be made a lot faster and cheaper than with these traditional methods. This is really a way to democratize this technology,” says Luis Fernando Velásquez-García, a principal scientist in MIT’s Microsystems Technology Laboratories (MTL) and senior author of a paper describing the fabrication technique.
He is joined on the paper by lead author Jorge Cañada Pérez-Sala, an electrical engineering and computer science graduate student. The research will be presented at the PowerMEMS Conference this month.
An insulator becomes conductive
This new fabrication process utilizes a technique called multimaterial extrusion 3D printing, in which several materials can be squirted through the printer’s many nozzles to build a device layer by layer. The process is monolithic, which means the entire device can be produced in one step on the 3D printer, without the need for any post-assembly.
To create self-heating microfluidics, the researchers used two materials — a biodegradable polymer known as polylactic acid (PLA) that is commonly used in 3D printing, and a modified version of PLA.
The modified PLA has mixed copper nanoparticles into the polymer, which converts this insulating material into an electrical conductor, Velásquez-García explains. When electrical current is fed into a resistor composed of this copper-doped PLA, energy is dissipated as heat.
“It is amazing when you think about it because the PLA material is a dielectric, but when you put in these nanoparticle impurities, it completely changes the physical properties. This is something we don’t fully understand yet, but it happens and it is repeatable,” he says.
Using a multimaterial 3D printer, the researchers fabricate a heating resistor from the copper-doped PLA and then print the microfluidic device, with microscopic channels through which fluid can flow, directly on top in one printing step. Because the components are made from the same base material, they have similar printing temperatures and are compatible.
Heat dissipated from the resistor will warm fluid flowing through the channels in the microfluidic.
In addition to the resistor and microfluidic, they use the printer to add a thin, continuous layer of PLA that is sandwiched between them. It is especially challenging to manufacture this layer because it must be thin enough so heat can transfer from the resistor to the microfluidic, but not so thin that fluid could leak into the resistor.
The resulting machine is about the size of a U.S. quarter and can be produced in a matter of minutes. Channels about 500 micrometers wide and 400 micrometers tall are threaded through the microfluidic to carry fluid and facilitate chemical reactions.
Importantly, the PLA material is translucent, so fluid in the device remains visible. Many processes rely on visualization or the use of light to infer what is happening during chemical reactions, Velásquez-García explains.
Customizable chemical reactors
The researchers used this one-step manufacturing process to generate a prototype that could heat fluid by 4 degrees Celsius as it flowed between the input and the output. This customizable technique could enable them to make devices which would heat fluids in certain patterns or along specific gradients.
“You can use these two materials to create chemical reactors that do exactly what you want. We can set up a particular heating profile while still having all the capabilities of the microfluidic,” he says.
However, one limitation comes from the fact that PLA can only be heated to about 50 degrees Celsius before it starts to degrade. Many chemical reactions, such as those used for polymerase chain reaction (PCR) tests, require temperatures of 90 degrees or higher. And to precisely control the temperature of the device, researchers would need to integrate a third material that enables temperature sensing.
In addition to tackling these limitations in future work, Velásquez-García wants to print magnets directly into the microfluidic device. These magnets could enable chemical reactions that require particles to be sorted or aligned.
At the same time, he and his colleagues are exploring the use of other materials that could reach higher temperatures. They are also studying PLA to better understand why it becomes conductive when certain impurities are added to the polymer.
“If we can understand the mechanism that is related to the electrical conductivity of PLA, that would greatly enhance the capability of these devices, but it is going to be a lot harder to solve than some other engineering problems,” he adds.
“In Japanese culture, it’s often said that beauty lies in simplicity. This sentiment is echoed by the work of Cañada and Velasquez-Garcia. Their proposed monolithically 3D-printed microfluidic systems embody simplicity and beauty, offering a wide array of potential derivations and applications that we foresee in the future,” says Norihisa Miki, a professor of mechanical engineering at Keio University in Tokyo, who was not involved with this work.
“Being able to directly print microfluidic chips with fluidic channels and electrical features at the same time opens up very exiting applications when processing biological samples, such as to amplify biomarkers or to actuate and mix liquids. Also, due to the fact that PLA degrades over time, one can even think of implantable applications where the chips dissolve and resorb over time,” adds Niclas Roxhed, an associate professor at Sweden’s KTH Royal Institute of Technology, who was not involved with this study.
Written by Adam Zewe
Source: Massachusetts Institute of Technology
You can offer your link to a page which is relevant to the topic of this post.
#3d#3D printing#additive manufacturing#amazing#applications#biodegradable#biomarkers#Biotechnology news#blood#chemical#chemical reactions#Chemistry & materials science news#chips#computer#Computer Science#conference#continuous#covid#Developing countries#Developments#devices#Disease#Diseases#energy#Engineer#engineering#equipment#Fabrication#Featured life sciences news#Featured technology news
2 notes
·
View notes