#isostatic pressing applications
Explore tagged Tumblr posts
Text
Shaping the Future: Insights into the Isostatic Pressing Market
The global isostatic pressing market is projected to be USD 13,568.1 million by 2030 growing at a CAGR of 7.0% during the forecast period. sectors including energy, aerospace, medical devices, automotive, and manufacturing regularly use isostatic pressing. In this regard, the requirement for such technology is significantly impacted by the growing requirement for contemporary materials and…
View On WordPress
#advanced forming technology#advanced materials#aerospace industry#automotive components#ceramic components#engineering ceramics#high-pressure manufacturing#industrial manufacturing#industry trends#isostatic pressing#isostatic pressing applications#isostatic pressing process#manufacturing efficiency#market growth#material compaction#metal parts production#powdered metals#precision engineering
0 notes
Text
Future of Powder Metallurgy Market: Insights from Industry Experts
The global powder metallurgy market size is estimated to reach USD 6.36 billion by 2030, according to a new report by Grand View Research, Inc., expanding at a CAGR of 12.9% over the forecast period. Increasing initiatives to reduce the weight of aerospace parts by using additive manufacturing along with a rise in demand for lightweight auto parts from the automotive industry drives the market growth.
The aircraft manufacturers are focusing on saving the cost and weight of aircraft parts, which increases the demand for 3D printing materials to deliver high-performance and cost-effective aircraft elements. An increase in the number of 3D printers in terms of feature, size, and material compatibility in the long run coupled with strong competitive strategies to expand through various partnerships and joint ventures is further expected to drive the market growth.
The growing demand from the healthcare industry for personalized prosthetics along with increasing usage of medical devices propels the sales mostly from the metal additive manufacturing market space. In the industrial sector, rapid prototyping and on-site production are estimated to offer promising growth opportunities to market vendors over the projected period.
The COVID-19 pandemic led to the shutdown of manufacturing and metallurgy industries and affected both upsides and downsides in the year 2020. The key end-use sectors of the powder metallurgy industry, including the automotive, aerospace, and consumer goods sectors, have observed a negative trend in FY 2020. For instance, in April 2021, Toyota Motor Corp. experienced a decline in worldwide sales by 5.1% in the fiscal year ending March 2021. This has stalled automotive and other industrial production activities and caused a severe decline in demand for powder metals, such as iron, steel, and aluminum.
Gather more insights about the market drivers, restrains and growth of the Powder Metallurgy Market
Powder Metallurgy Market Report Highlights
• The steel material segment is estimated to witness a CAGR of 12.0%, in terms of revenue, over the projected period. The low cost and easy availability of steel are expected to drive the segment growth
• The Metal Injection Molding (MIM) process segment led the global market in 2020 and is projected to witness steady growth over the coming years owing to the significance of the process in the components manufacturing industry
• The aerospace & defense application segment accounted for the largest revenue share of more than 51.0% in 2022. The aerospace & defense industry is highly focused on adapting new technologies with a high investment budget, which is the key driving factor for the segment
• Large-scale OEMs, generally from the aircraft industry, are investing significantly in metal 3D printing to manufacture aeronautical parts, which is expected to drive the OEMs end-use segment at the fastest CAGR over the forecast years
• North America accounted for the highest share of more than 34.0% in 2022. Increased funding for the R&D and standardization in the technology segment in leading European countries is expected to propel the market growth
Powder Metallurgy Market Segmentation
Grand View Research has segmented the global powder metallurgy market on the basis of material, process, application, end-use, and region:
Powder Metallurgy Material Outlook (Volume, Tons; Revenue, USD Billion, 2018 - 2030)
• Titanium
• Nickel
• Steel
• Aluminum
• Cobalt
• Others
Powder Metallurgy Process Outlook (Volume, Tons; Revenue, USD Billion, 2018 - 2030)
• Additive Manufacturing
• Powder Metal Hot Isostatic Pressing
• Metal Injection Molding
Powder Metallurgy Application Outlook (Volume, Tons; Revenue, USD Billion, 2018 - 2030)
• Aerospace & Defense
• Automotive
• Oil & Gas
• Industrial
• Medical & Dental
Powder Metallurgy End-use Outlook (Revenue, USD Billion; Volume, Tons, 2018 - 2030)
• OEMs
• AM Operators
Powder Metallurgy Regional Outlook (Volume, Tons; Revenue, USD Billion, 2018 - 2030)
• North America
o U.S.
• Europe
o Germany
o France
o U.K.
• Asia Pacific
o China
o Japan
• Central & South America
o Brazil
• Middle East & Africa
Order a free sample PDF of the Powder Metallurgy Market Intelligence Study, published by Grand View Research.
#Powder Metallurgy Market#Powder Metallurgy Market Size#Powder Metallurgy Market Share#Powder Metallurgy Market Analysis#Powder Metallurgy Market Growth
0 notes
Text
Isostatic Pressing Market Demand, Trends, Report 2023-2030
BlueWeave Consulting, a leading strategic consulting and market research firm, in its recent study, estimated Global Isostatic Pressing Market size at USD 7.75 billion in 2023. During the forecast period between 2024 and 2030, BlueWeave expects Global Isostatic Pressing Market size to expand at a significant CAGR of 8.08% reaching a value of USD 12.35 billion by 2030. The growth of Global Isostatic Pressing Market is influenced by an increasing demand for advanced materials, including ceramics and composites, drives market growth as isostatic pressing is used in their production. Also, the aerospace and automotive industries rely on the isostatic pressing technology for manufacturing precision components, contributing to market expansion. Further, the growing energy sector fuels the demand for isostatic pressing in manufacturing parts for power generation equipment. Also, the medical and semiconductor industries benefit from their applications in manufacturing high-precision components. On top of that, technological advancements and innovations in isostatic pressing techniques further propel the market's growth by enhancing efficiency and versatility.
Sample Request: https://www.blueweaveconsulting.com/report/isostatic-pressing-market/report-sample
Opportunity - Rising Demand for Low Cost Titanium and Alloys
Global Isostatic Pressing Market is projected to record significant growth from a spurring demand for low cost titanium and alloys from the automotive sector. Isostatic pressing is a manufacturing process used to shape and consolidate powdered materials into desired forms. The process is ideal for producing complex and precise shapes, such as ceramics, metal parts, and composites. Isostatic pressing is generally used in automotive industry to create high-density, high-strength components with minimal defects and uniform properties. Thus, the process of isostatic pressing enables automobile manufacturers to produce low cost titanium and alloys.
Impact of Escalating Geopolitical Tensions on Global Isostatic Pressing Market
Global Isostatic Pressing Market witnesses notable impacts from the increasing geopolitical tensions across the world. As wars and conflicts result in economic and trade sanctions and supply chain disruptions, demand for isostatic pressing machinery and services could decline across various industries, including aerospace and automotive, resulting in reduced production and postponed capital investments. However, Global Isostatic Pressing Market also saw some resilience due to an increased demand for isostatic pressing in the medical and pharmaceutical sectors for manufacturing critical components, such as ventilator parts and medical devices.
Large Sized Isostatic Pressing Segment Leads Global Market by HIP Capacity
The large sized pressing segment holds a major share in Global Isostatic Pressing Market by HIP (hot-isostatic pressing) capacity. The segment encompasses a wide range of industrial applications that require substantial force and pressure for shaping materials. Large-sized pressing equipment is utilized in various industries, including aerospace, automotive, and manufacturing, to create components and products that demand high precision and strength. The demand for large-sized pressing equipment is often driven by the need for efficiently producing sizable parts or achieving intricate designs in critical applications, making it a prominent and significant segment in Global Isostatic Pressing Market by hip capacity.
Competitive Landscape
Global Isostatic Pressing Market is fiercely competitive. Major companies in the market include American Isostatic Presses (API), DORST Technologies GmbH & Co.KG, Bodycote PLC, Engineered Pressure Systems (EPSI), Fluitron, Inc., Kobe Steel, Ltd, Nikkiso Co. Ltd, Pressure Technology, Inc., Shanxi Golden Kaiyuan Co. Ltd, Kittyhawk Products, Quad City Manufacturing Lab, Aerosint SA, Höganäs AB, Kobe Steel Ltd, and Quintus Technologies AB. These companies use various strategies, including increasing investments in their R&D activities, mergers, and acquisitions, joint ventures, collaborations, licensing agreements, and new product and service releases to further strengthen their position in Global Isostatic Pressing Market.
Contact Us:
BlueWeave Consulting & Research Pvt. Ltd
+1 866 658 6826 | +1 425 320 4776 | +44 1865 60 0662
0 notes
Text
Exploring the Versatility of Tungsten Rods in Industrial Applications
Are you looking for an incredibly strong, ductile, and heavy metal to use in your industrial applications? Then tungsten rods could be just the option for you. Known as one of the hardest metals on Earth, tungsten is not only versatile but also incredibly durable – making it suitable for a wide range of uses. From its many impressive mechanical properties to its incredible resistance to wear and corrosion – it’s no wonder that tungsten has become a favorite choice amongst industry professionals worldwide. In this blog post, we'll explore how these unique rods can benefit various industries and why they may be the perfect component for your next project!

The Unique Properties of Tungsten Rods and Their Industrial Uses
Tungsten rods have become increasingly popular in various industries due to their unique properties and applications. One of the main features setting tungsten rods apart is their remarkable hardness and durability, making them capable of withstanding even the highest levels of heat and pressure. This characteristic ensures their widespread use in industries that require high-performance materials such as aerospace engineering, automotive manufacturing, and construction. Additionally, tungsten rods possess high thermal and electrical conductivity, making them ideal for applications where heat or electricity may be involved. From light bulb filaments to radiation shields, tungsten rods have become a staple material in many modern industries, proving to be a valuable asset in the world of engineering and technology.
Advancements in Tungsten Rod Manufacturing Techniques
Tungsten, known for its remarkable physical and chemical properties, is crucial for high-tech industries. The advancements in tungsten rod manufacturing techniques have been nothing short of revolutionary in recent years. The more traditional methods, such as powder metallurgy, have paved the way for newer techniques such as Liquid Phase Sintering, Electro-Slag Remelting, and Hot Isostatic Pressing which have brought about a shorter production time, increased toughness, and better machinability. These developments have increased the demand for tungsten in the tech industry. As a testament to the progress achieved, it is interesting to note that tungsten rods today are constructed with better precision, purity, and performance than ever before, allowing them to be used in constantly demanding conditions.
Customizing Tungsten Rods for Specific Industrial Needs
Whether it's for aerospace, automotive, or medical applications, industrial components are required to operate at their highest efficiency levels at all times. That's why tungsten rods have established themselves as one of the most preferred materials for various manufacturing processes, due to their ability to withstand high temperatures and pressure. However, these tungsten rods cannot be used naturally as they need to be tailored to specific industrial needs. Customizing tungsten rods involves a series of steps that alter their structure and composition, which can affect their mechanical, thermal, and electrical properties. Therefore, it's important to consult experienced technicians who can help you customize your tungsten rods to meet your specific industrial needs.
In conclusion, tungsten rods have many unique and beneficial properties that make them ideal for various industrial applications. Manufacturing techniques for these rods are evolving to meet the demands of industry, and with continued advances in this area, customized solutions can be created to meet specific industrial needs. Tungsten rods can be a cost-effective and reliable solution to a wide variety of industrial scenarios. It’s no wonder that they’ve become so important in today’s world. Investigate further if you think tungsten rods could be right for your project or desire to learn more about their unique properties. There are plenty of resources out there that discuss the characteristics of tungsten rods and how they could contribute to your particular needs. Get started now – it just might change your industrial operations for the better!
0 notes
Text
Features of porous titanium

Porous titanium, also named as sintered titanium and titanium foams, is sintered from titanium powder. It has pore structure exhibit high specific strength, high energy absorption, excellent corrosion resistance and biocompatibility. Generally, porous titanium processed from irregular titanium or titanium alloy irregular powder. It can be isostatically pressed, molded, and extruded.
The main process for porous titanium (including tube and sheet) is made of high-purity titanium powder as raw material, after sieved, cold isostatic pressing, high temperature, high vacuum sintering. The porous titanium is made up of its high-tech materials and special molding process, which makes it have unique and excellent performance:
-Uniform structure, narrow pore size distribution and high separation efficiency.
-High porosity, low filtration resistance and high penetration efficiency.
-High temperature resistance, generally can be used below 280 degrees.
-Good chemical stability, acid and alkali corrosion resistance, with antioxidant properties.
-Good mechanical properties.
-Low pressure difference, small footprint, large flow.
-Strong anti-microbial ability, does not interact with microorganisms.
Porous titanium has a wide variety of unique and important applications, included filtration, gas absorbing, gas sparging, separation, catalyst supporting, current collection, medical implantation and etc.
0 notes
Text
0 notes
Text
Powder Metallurgy Market - Forecast(2024 - 2030)
Powder Metallurgy Market Overview

Request Sample Report :
COVID-19 Impact
The onset of Covid-19 in the entire nation had a negative impact on the automotive industry. There was an overall revenue impact across the automotive industry. The pandemic also impacted all the stakeholders present in the value chain for the short as well as medium term. Shortage of raw material, shifting of production to other countries, liquidity crunch to delays in availability of models, deferred launches, and shrinkage in consumer demand were some of the main issues faced by the automotive stakeholders, owing to which the production and demand of automobiles had significantly fallen. For instance, according to the China Passenger Car Association (CPCA), China’s passenger car sales in June fell 6.5% year on year to 1.68 million units. The unstable automotive production and demand have significantly impacted the market of powder metallurgy as the demand for powder metal was also uncertain during the period. A prolonged truncation of consumer demand due to the lockdown has significantly affected auto manufacturers’ revenues and cash flows. Even after the restriction is eased in 2021, with discretionary spending taking a backseat, further declines in the market for passenger vehicles are expected.
Powder Metallurgy Market Report Coverage
The report: “Powder Metallurgy Market — Forecast (2021–2026)”, by IndustryARC, covers an in-depth analysis of the following segments of the powder metallurgy Industry.
By Material: Ferrous (Iron, Steel, and Stainless Steel), Non-Ferrous (Copper, Aluminium, Cobalt, Tin, Nickel, Magnesium, Zinc, Titanium, Molybdenum, and Others By Process: Conventional Process, Metal Injection Moulding, Hot Isostatic Pressing, and Metal Additive Manufacturing By Application: Porous Products, Bearing and Bushes, Filters (Ceramic Filter, Fiber Metal Filter, and Others), Refractory Metal Composites, Electric Motors, Cemented Carbides, Machinery Parts (Gears, Sprockets, Rotors, and Others), Tungsten Wires, Medical Implants, Magnetic Materials, Cutting Tools and Dies, and Others By End-Use Industry: Medical & Healthcare, Automobile (Transmission, Engine Parts, and others), Oil & Gas, Electrical and Electronics (Refrigerators, Vacuum Cleaners, Circuit Breakers, Electric Motors, Sewing Machines, and Others), Industrial (Hydraulics, Motors/Controls, and Others), Aerospace (Aero-engine, Land-based Gas Turbine, Airframes, and Others), Household Appliances, Recreation and Leisure, and Others By Geography: North America (USA, Canada, and Mexico), Europe (UK, Germany, France, Italy, Netherlands, Spain, Russia, Belgium, and Rest of Europe), Asia-Pacific (China, Japan, India, South Korea, Australia and New Zealand, Indonesia, Taiwan, Malaysia, and Rest of APAC), South America (Brazil, Argentina, Colombia, Chile, and Rest of South America), Rest of the World (Middle East, and Africa)
Inquiry Before Buying :
Key Takeaways
Asia-Pacific dominates the powder metallurgy market, owing to the increasing demand and production of lightweight vehicles in the region. For instance, according to OICA, the production of passenger cars has increased by 2.6 % in Malaysia in 2019.
There are many cases in which powder metallurgy over casting has a strong benefit. The benefits of the powder metallurgy process are particularly apparent when dealing with high-value and high-melting-point materials, owing to which there is increasing adoption of powder metallurgy over die casting, which will propel the market growth.
There are growing interests in producing customized medical implants using additive manufacturing and in producing porous implant structures (to match bone stiffness and to aid osteointegration) by powder metallurgy processing, which may boost the market growth.
For More Details on This Report — Request for Sample
Powder Metallurgy Market Segment Analysis — By Material
The ferrous segment held the largest share in the powder metallurgy market in 2020. Ferrous powdered metals such as iron, steel, and more offer the highest level of mechanical properties. Ferrous metal powders are also known for their extensive characteristics such as durability, hardness, tensile strength, lower costs, broad flexibility, and more. The ferrous powdered metal is largely employed in the automotive industry for designing bearings, gears, or other auto parts, owing to its good strength and flexibility. Furthermore, ferrous metals are also widely utilized in various other end-use industries such as construction, piping, aerospace, electronics, and more. Thus, it is anticipated that these extensive characteristics and wide applications of ferrous powder metallurgy are the major factors driving its demand during the forecast period.
Powder Metallurgy Market Segment Analysis — By Application
The bearings segment held the largest share in the powder metallurgy market in 2020 and is growing at a CAGR of 7.4% during 2021–2026, owing to its self-lubricating property. Bearings that are made from powder metallurgy are known as self bearings of sintered metal. They are economical, suitable for high production rates, and precision tolerances can be produced. The majorities of porous-metal bearings consist of either bronze or iron with pores that are interconnected. These voids take up 10 percent of the total volume to 35 percent. In operation, lubricating oil is deposited in these voids and feeds to the bearing surface through the interconnected pores. Sintered-metal self-lubricating bearings are widely used in home appliances, small motors, machine tools, aircraft, and automotive accessories, business machines, instruments, and farm and construction equipment, owing to which it holds a prominent share in the powder metallurgy application segment.
Schedule A Call :
Powder Metallurgy Market Segment Analysis — By End-Use Industry
The automotive segment held the largest share in the powder metallurgy market in 2020 and is growing at a CAGR of 8.6% during 2021–2026. Powder metal parts display excellent controlled porosity and self-lubricating properties that allow gases and liquids to be filtered. Powder metallurgy is also a strongly recommended method in the manufacture of components involving complex bends, depressions, and projections. Flexibility in the development of mechanical parts of different compositions, such as metal-non-metal and metal-metal hybrids, allows high dimensional precision in the production of automobile parts and ensures consistent properties and measurements with very little scrap and waste of material. The most popular vehicle parts that are manufactured through the method of powder metallurgy are the bearings and gears. A variety of metals, including ferrous, and non-ferrous are used in automotive components including chassis, steering, exhaust, transmission, shock absorber parts, engine, battery, seats, air cleaners, brake disc, and more. The powder metallurgy is often used in these components as it improves the net shape, utilizes heat treatment, enhances surface treatment, and improves the precision of these components. Thus, the demand for powder metallurgy in the automotive sector is growing due to such factors.
Powder Metallurgy Market Segment Analysis — By Geography
Asia-Pacific region held the largest share in the powder metallurgy market in 2020 up to 45%, owing to the increasing automotive manufacturing coupled with population growth in the region. China is the world’s largest vehicle market, according to the International Trade Administration (ITA), and the Chinese government expects automobile production to reach 35 million by 2025. In 2019, according to OICA, the automotive production in Malaysia and Vietnam has increased up to 571632, and 250000, i.e., 1.2%, and 5.5%. India’s annual production in 2019 was 30.91 million vehicles, according to Invest India, compared to 29.08 million in 2018, recording a healthy 6.26 percent growth. Also, by 2026, the US$118 billion Indian car industry is projected to cross US$300 billion. The increasing automation production in the Asia Pacific will eventually boost the demand for powder metallurgy to manufacture various automobile components, which will likely influence the growth of the powder metallurgy market in the APAC region.
Powder Metallurgy Market Drivers
Flourishing Aerospace Industry
Powder metallurgy is used extensively in aerospace, because of its advantages of high strength/weight ratio, high heat capacity, and high modulus of elasticity. Within aerospace, powder metallurgy finds its most significant application in turbine engines, compressors, fan sections, discs, airframes, fasteners, and landing gear. Tungsten metallurgy based tungsten carbide has received considerable attention in the aerospace industry because of its high strength at very high temperatures. Tungsten carbide is sintered through a selective laser sintering process based on the additive manufacturing process. In 2019, China was the second-largest civil aerospace and aviation services market in the world and one of the fastest-growing markets, according to the International Trade Administration (ITA). China will need 7,690 new aircraft over the next 20 years, valued at US$1.2 trillion, according to Boeing (Commercial Market Outlook 2018–2037). China also currently accounts for 15 percent of the world’s commercial aircraft fleet, and it will be almost 20 percent by 2037. According to Boeing, the demand for 2,300 airplanes worth US$320 billion is projected in India over the coming 20 years. Boeing’s current market outlook (BMO) forecasts demand for 2,520 new aircraft in the Middle East by 2030. With the flourishing aerospace industry, the demand for aircraft components will also gradually increase, which will drive the market growth.
Buy Now :
Expanding Electrical and Electronics Sector
The powder metallurgy method offers the opportunity to cost-effectively produce net form components from a variety of materials. A market segment that has exhibited the ability to take advantage of powder metallurgy’s flexibility has been in electromagnetic applications such as household appliances, industrial applications, and more. Magnetic materials are essential elements in the electronic industry in recent times. From the motors and turbines that provide the power for the industry to the high-frequency transformers that power computers, magnetic materials are becoming increasingly important for consumers. The electronic sector is booming in various regions, which will further drive the market growth as there is an increasing demand for powder metallurgy from the electrical and electronics sector. For instance, the consumer electronics and appliances sector in India is expected to become the fifth-largest in the world by 2025, according to Invest India. India could create an US$800 billion to US$1 trillion digital economy by 2025, and India’s digital economy could fuel 18–23 percent of overall economic activity by 2025. According to the Government of Canada, revenues in the ICT sector reached an estimated US$210 billion in 2019. ICT sector revenues grew from US$158 billion to US$200 billion from 2013 to 2018, a 26.9 percent increase.
Powder Metallurgy Market Challenges
Various Drawbacks Associated with Powder Metallurgy
The cost of metal powders compared to the cost of raw material used for casting or forging a component is relatively higher. At the time the cost of tooling and equipment is also higher. When production volumes are limited, this is especially a limitation. Also, low melting point metal powders such as zinc, tin, and cadmium give thermal difficulties during sintering operation, as most oxides of these metals cannot be reduced at temperatures below the melting point. Furthermore, large or complex-shaped parts, and uniformly high — density products are difficult to produce by the powder metallurgy process. Without any degradation, a few powders are also difficult to store. All these drawbacks associated with the powder metallurgy may hinder the market growth during the forecast period.
Powder Metallurgy Market Landscape
Technology launches, acquisitions, and R&D activities are key strategies adopted by players in the powder metallurgy market. Major players in the powder metallurgy market are Arcam AB, Carpenter Technology Corp., ExOne GmbH, GKN Plc, Höganäs AB, Materialize NV, Melrose Industries PLC, Sumitomo Electric Industries, Ltd., and Hitachi Chemical Co., Ltd.
Acquisitions/Technology Launches
In May 2019, Epson Atmix Corp. installed a new production line at its Kita-Inter Plant in Japan for producing amorphous alloy powder. The company invested around ¥800 million (USD 7.43 million) for the installation of a new line and it plans to further increase its production capacity in stages and reach 6,000 tons per year by 2023.
In October 2019, GKN Powder Metallurgy a leading metal powder and parts manufacturers acquired specialist polymer 3D printing service provider Forecast 3D. The acquisition presents a significant expansion of GKN powder metallurgy’s additive capabilities.
Relevant Reports
Powder Coatings Market — Forecast (2021–2026)
Report Code: CMR 0113
Ferromanganese Market — Forecast (2021–2026)
Report Code: CMR 0382
For more Chemicals and Materials Market reports, please click here
#PowderMetallurgy#Metalworking#AdvancedManufacturing#PowderMetals#AdditiveManufacturing#MetalPowder#IndustrialMaterials
0 notes
Text
Introduction to Aluminum Nitride Ceramics
Aluminum nitride ceramics are ceramics with aluminum nitride as the main crystal phase. It is a covalent bond compound, belongs to the hexagonal crystal system, has a fibrillar crystal structure, and is white or grayish-white.

Aluminum nitride ceramics processing
The manufacturing methods of aluminum nitride ceramics include hot pressing sintering, atmospheric pressure sintering, and reaction sintering. The first two are usually used. Because AlN will hydrolyze, it cannot be slurry injection molding. It can be molded and isostatically pressed. When AlN powder is granulated, polyvinyl alcohol (PVA) is used as a binder because PVA is insoluble in anhydrous ethanol.
The hot pressing sintering process is to heat one side and pressurize the other side at a high temperature of 1800~2000℃, and Al2O3, MgO, and SiO2 additives may or may not be added.
The atmospheric pressure sintering process is to add additives Y2O3, Al2O3, SiO2, Be0, CaO, etc. at a high temperature of 1800~1900℃.
Performance and application of aluminum nitride ceramics
Aluminum nitride ceramics have a high melting point of 2450℃, good stability in high-temperature non-oxidizing atmospheres within 2000℃, and high thermal conductivity, which is 10 times that of alumina ceramics. Similar to beryllium oxide ceramics, its thermal expansion coefficient is identical to that of silicon, with high electrical insulation resistance, excellent dielectric constant and low dielectric loss, good mechanical properties, corrosion resistance, and strong light transmittance.
In addition, aluminum nitride is not eroded by molten metals such as molten aluminum and gallium arsenide, especially because it has excellent corrosion resistance to molten aluminum liquid. However, the high temperature (>800℃) oxidation resistance of AIN ceramics is poor, and it is easy to absorb moisture and hydrolyze in the atmosphere, which should attract people's attention.
The use of aluminum nitride ceramics has the characteristics of high strength at room temperature and high temperature, small expansion coefficient, and good thermal conductivity. It can be used as high-temperature components, heat exchanger materials, etc.
It has the ability to resist the dissolution of metals and alloys such as iron and aluminum and can be used as crucibles and casting mold materials for smelting metals such as Al, Cu, Ag, and Pb.
It has excellent high-temperature resistance under a special atmosphere (about 2000℃) and can be used as the furnace body material of a non-oxidizing electric furnace.
Aluminum nitride ceramics have the characteristics of high thermal conductivity and high insulation resistance and can be used as heat sinks and insulating substrates for semiconductors. Aluminum nitride film can be made into high-frequency piezoelectric components, and ultra-large-scale integrated circuit substrates are one of the most important uses of aluminum nitride ceramics.
0 notes
Text
Isostatic Pressing Market Strategies and Resources to Grow Your Company, 2032
Isostatic pressing is a highly effective manufacturing process used to shape and densify materials, such as metals and ceramics, through uniform pressure application. This technique is particularly valuable in producing complex geometries with high density and minimal porosity. By ensuring that pressure is applied uniformly from all directions, isostatic pressing enhances the mechanical properties of materials, making it a preferred choice in industries like aerospace, automotive, and electronics.
The isostatic pressing process can be categorized into two main types: hot isostatic pressing (HIP) and cold isostatic pressing (CIP). HIP involves heating the material during the pressing process, which promotes densification and improves material properties, while CIP is performed at room temperature. Both methods are crucial for achieving high-quality components that meet the demanding specifications of various applications.
The Isostatic Pressing Market is on the rise due to its applications in producing high-density materials across various industries, including aerospace, automotive, and electronics. The demand for advanced manufacturing techniques that ensure uniform pressure distribution is driving innovations in isostatic pressing technologies, leading to improved material properties and performance.
Future Scope
The future of isostatic pressing is promising, as advancements in materials science and manufacturing technologies continue to evolve. The demand for high-performance components with intricate designs is expected to drive the adoption of isostatic pressing across various sectors. Innovations in automation and process control will further enhance efficiency and precision, making isostatic pressing a cornerstone of advanced manufacturing practices.
As industries strive for more sustainable and efficient manufacturing processes, isostatic pressing is well-positioned to meet these needs. The ability to produce components with minimal waste and high yield makes it an attractive option for manufacturers looking to reduce their environmental footprint. Additionally, the ongoing development of new materials, including advanced ceramics and composites, will expand the range of applications for isostatic pressing.
Trends
Current trends in isostatic pressing include the integration of additive manufacturing techniques, enabling the production of complex shapes that were previously challenging to achieve. This hybrid approach allows manufacturers to combine the benefits of additive and subtractive manufacturing, resulting in improved design flexibility and reduced lead times.
There is also an increasing focus on sustainable practices, with efforts to optimize material usage and minimize waste during the pressing process. Manufacturers are exploring ways to recycle and repurpose materials, further enhancing the sustainability of isostatic pressing. Additionally, advancements in simulation and modeling technologies are improving process design and outcomes, allowing for more precise control over the pressing parameters.
Application
Isostatic pressing is widely used across various sectors, including aerospace for producing lightweight, high-strength components, automotive for manufacturing parts with stringent quality requirements, and electronics for creating high-performance substrates and capacitors. Its ability to produce dense, high-quality materials makes it indispensable in applications that demand superior mechanical properties.
In aerospace applications, isostatic pressing is used to manufacture components such as turbine blades and structural elements that require exceptional strength-to-weight ratios. In the automotive industry, it plays a critical role in producing high-performance components, such as brake discs and engine parts, that must withstand extreme conditions. In electronics, isostatic pressing is essential for creating substrates used in advanced circuit boards and capacitors, ensuring reliable performance in electronic devices.
Key Points
Effective for shaping and densifying materials through uniform pressure.
Increasing demand for high-performance components drives adoption.
Trends include integration with additive manufacturing and sustainable practices.
Applied in aerospace, automotive, and electronics sectors.
Read More Details: https://www.snsinsider.com/reports/isostatic-pressing-market-4528
Contact Us:
Akash Anand — Head of Business Development & Strategy
Email: [email protected]
Phone: +1–415–230–0044 (US) | +91–7798602273 (IND)
0 notes
Text
Durability and Wear Resistance of Ceramic Valve Cores
The ceramic valve core, a critical component in various fluid control systems, is renowned for its good performance characteristics. Among these, its wear resistance stands out as a key feature that significantly contributes to its longevity and reliability. This article delves into the intricacies of the ceramic valve core's wear resistance, examining the factors that contribute to its durability and the implications for its use in various applications.

The ceramic valve core's resistance to wear is a result of the material's inherent properties. Ceramics, being a non-metallic material, is composed of compounds such as oxides, carbides, and nitrides. These compounds provide the ceramic valve core with a high level of hardness, which is a critical factor in determining its ability to withstand wear. The hardness of ceramic materials is typically measured on the Mohs scale, and ceramic valve cores often fall within the range of 7 to 9, indicating a high resistance to abrasive wear.
One of the primary reasons for the ceramic valve core's good wear resistance is its low coefficient of friction. When compared to metal counterparts, ceramic valve cores exhibit a significantly reduced tendency to generate friction, which is a major cause of wear in moving parts. This low frictional property is particularly beneficial in applications where the valve core is subjected to frequent operation, as it reduces the wear and tear that would otherwise occur over time.
The manufacturing process of ceramic valve cores also plays a significant role in enhancing their wear resistance. Advanced techniques such as sintering and hot isostatic pressing are used to achieve high-density ceramic structures. These processes ensure that the ceramic valve core is free from porosity and micro-cracks, which are common sources of weakness in materials and can cause premature wear.
Moreover, the ceramic valve core's resistance to corrosion is another factor that contributes to its wear resistance. In environments where corrosive fluids are present, metal valve cores can degrade over time, causing increased wear and eventual failure. Ceramic valve cores, on the other hand, are chemically inert and do not react with most chemicals, thus maintaining their structural integrity and wear resistance even in harsh conditions.
The application of advanced surface treatments and coatings on ceramic valve cores further enhances their wear resistance. These treatments can include the application of diamond-like carbon (DLC) coatings, which are known for their extreme hardness and low friction properties. By applying such coatings, the ceramic valve core's surface is protected from abrasive wear, and its service life is significantly extended.
In practical applications, the ceramic valve core's wear resistance is put to the test in various ways. For instance, in water control systems, the valve core is subjected to the abrasive action of sand and other particulates present in the water. Similarly, in industrial processes involving the handling of slurries or abrasive materials, the valve core must withstand the constant wear caused by these materials. The ceramic valve core's ability to maintain its performance in such conditions is a testament to its good wear resistance.
However, it is important to note that while the ceramic valve core is highly resistant to wear, it is not immune to damage. Impact forces, thermal shock, and improper installation can still be causing the failure of the valve core. Therefore, proper handling, installation, and maintenance are crucial to ensure the suitable performance and longevity of the ceramic valve core.
In conclusion, the ceramic valve core's wear resistance is a multifaceted attribute that stems from its material properties, manufacturing processes, and surface treatments. Its high hardness, low coefficient of friction, and chemical inertness make it an ideal choice for applications where durability and reliability are paramount. As technology continues to advance, the ceramic valve core's wear resistance will likely be further improved, solidifying its position as a preferred choice in fluid control systems across various industries.
0 notes
Text
What is the Application of Isostatic Pressing in Aerospace Industry?
The method of isostatic pressing was initiated during the mid-1950s and has gradually developed from a research interest to a practical tool of manufacturing. Various sectors utilize this method for powder consolidation or casting defect healing. The procedure is utilized for various materials, such as metals, ceramics, composites, carbon, and plastics. Isostatic pressing enforced a constant,…
View On WordPress
#advanced materials#industrial applications#isostatic pressing innovations#isostatic pressing market#isostatic pressing technology#Market Analysis#Market dynamics#market trends#material forming#material shaping
0 notes
Text
Unveiling the Potential of Silicon Carbide Tubes: A Comprehensive Guide
Silicon Carbide (SiC) tubes are at the forefront of advanced ceramic technology, offering unmatched performance in numerous industrial applications. Known for their exceptional strength, thermal stability, and chemical resistance, SiC tubes are indispensable in environments where conventional materials fail. This article provides an in-depth exploration of silicon carbide tubes, covering their properties, manufacturing processes, applications, and advantages.
What are Silicon Carbide Tubes?
Silicon Carbide tubes are cylindrical structures made from silicon carbide, a compound of silicon and carbon. These tubes exhibit extraordinary properties that make them suitable for extreme conditions, including high temperatures, corrosive environments, and high-stress applications. SiC tubes are used in a variety of industries, ranging from chemical processing to power generation and semiconductor manufacturing.
Properties of Silicon Carbide Tubes
Silicon carbide tube possess a unique set of properties that make them ideal for demanding applications:
High Hardness:
SiC tubes are extremely hard, ranking just below diamond on the Mohs hardness scale. This attribute provides excellent wear resistance, making them highly durable.
Thermal Stability:
SiC tubes can withstand very high temperatures, often exceeding 1,600°C. This thermal stability ensures their performance in high-temperature applications.
Chemical Resistance:
The material is highly resistant to chemical corrosion, including acids, alkalis, and oxidizing environments. This makes SiC tubes suitable for harsh chemical processing conditions.
High Thermal Conductivity:
Silicon carbide has excellent thermal conductivity, which allows for efficient heat transfer. This property is critical in applications requiring rapid temperature changes.
Low Thermal Expansion:
SiC tubes exhibit low thermal expansion, reducing the risk of cracking or deformation under thermal cycling.
Mechanical Strength:
The high strength of SiC tubes allows them to withstand significant mechanical stress without breaking or deforming.
Manufacturing Process of Silicon Carbide Tubes
The production of silicon carbide tubes involves several precise steps to ensure the material's superior properties:
Powder Preparation:
High-purity silicon carbide powder is prepared, often with specific additives to enhance certain properties.
Shaping:
The SiC powder is shaped into tubes using techniques such as extrusion, isostatic pressing, or slip casting. This step involves forming the powder into a cohesive green body.
Sintering:
The green body is then subjected to a high-temperature sintering process, typically in a controlled atmosphere. During sintering, the silicon carbide particles bond together to form a dense, solid structure.
Machining:
After sintering, the tubes may undergo additional machining to achieve precise dimensions and surface finishes. This step is crucial for applications requiring tight tolerances.
Quality Control:
The final tubes are rigorously tested for mechanical strength, thermal properties, and chemical resistance to ensure they meet the required specifications.
Applications of Silicon Carbide Tubes
Due to their exceptional properties, silicon carbide tubes are used in a wide range of applications across various industries:
Chemical Processing:
SiC tubes are ideal for use in reactors, heat exchangers, and piping systems in chemical processing plants. Their resistance to corrosion and high temperatures ensures long-term reliability.
Power Generation:
In power plants, silicon carbide tubes are used in high-temperature heat exchangers and gas turbines. Their thermal stability and conductivity enhance the efficiency of these systems.
Semiconductor Manufacturing:
SiC tubes play a crucial role in semiconductor fabrication equipment, where they are used in diffusion furnaces and chemical vapor deposition (CVD) processes. Their purity and thermal properties are essential for producing high-quality semiconductors.
Aerospace and Defense:
The aerospace and defense industries use SiC tubes in applications such as missile nozzles, rocket engines, and thermal protection systems. Their strength and thermal stability are vital for performance in extreme conditions.
Industrial Furnaces:
SiC tubes are employed in industrial furnaces for applications like metal melting, powder metallurgy, and sintering. Their ability to withstand high temperatures and thermal cycling makes them ideal for these environments.
Energy Storage and Conversion:
In energy storage systems, SiC tubes are used in components like fuel cells and batteries. Their chemical resistance and thermal management capabilities improve the efficiency and longevity of these systems.
Advantages of Silicon Carbide Tubes
Silicon carbide tubes offer several advantages over traditional materials:
Durability:
The high hardness and wear resistance of SiC tubes ensure a longer lifespan, reducing the need for frequent replacements and maintenance.
Efficiency:
The excellent thermal conductivity of SiC tubes allows for efficient heat transfer, improving the performance of thermal systems.
Cost-Effectiveness:
Although the initial cost of SiC tubes may be higher, their durability and low maintenance requirements make them cost-effective in the long run.
Versatility:
SiC tubes can be used in a variety of applications, from chemical processing to aerospace, making them highly versatile.
Performance in Extreme Conditions:
SiC tubes maintain their properties under extreme temperatures and corrosive environments, ensuring reliable performance in challenging conditions.
Challenges and Considerations
Despite their numerous advantages, there are some challenges associated with the use of silicon carbide tubes:
Manufacturing Complexity:
The production of SiC tubes requires precise control over the manufacturing process, making it more complex and costly compared to other materials.
Machining Difficulty:
The hardness of SiC makes it challenging to machine, requiring specialized equipment and expertise.
Initial Cost:
The initial cost of SiC tubes can be higher than that of other materials, which may be a consideration for some applications.
Conclusion
Silicon carbide tubes represent a pinnacle of advanced ceramic technology, offering a unique combination of strength, thermal stability, and chemical resistance. Their exceptional properties make them ideal for a wide range of demanding applications, from chemical processing and power generation to semiconductor manufacturing and aerospace. As industries continue to push the boundaries of performance and efficiency, the demand for SiC tubes is expected to grow, driving further innovation and development in this field. Whether in extreme temperatures, corrosive environments, or high-stress applications, silicon carbide tubes stand out as a reliable and high-performing solution.

0 notes
Text
Alumina Ceramic: Engineering Marvel with Unmatched Versatility and Performance
Alumina ceramic, scientifically termed aluminum oxide (Al2O3), stands as a paragon of modern engineering materials. It is widely recognized for its exceptional properties and versatility, making it indispensable in numerous high-performance applications. This comprehensive exploration delves into the unique attributes, manufacturing processes, and diverse applications of alumina ceramic, underscoring its pivotal role in advancing technology and industry.
The Exceptional Properties of Alumina Ceramic
Alumina ceramic is celebrated for its remarkable combination of physical, chemical, and mechanical properties, which include:
High Hardness: Alumina ceramic possesses a hardness of 9 on the Mohs scale, nearly as hard as diamond. This high hardness translates to excellent wear resistance, making it ideal for applications where durability is paramount.
Chemical Inertness: It exhibits outstanding chemical stability, resisting corrosion and degradation in harsh environments, including acidic and alkaline conditions. This property is critical for applications in chemical processing and biomedical fields.
Thermal Stability: Alumina ceramic maintains its structural integrity at high temperatures, with a melting point of approximately 2050°C (3722°F). This thermal stability makes it suitable for high-temperature applications such as furnace components and thermal insulators.
Electrical Insulation: Alumina is an excellent electrical insulator, with a high dielectric strength. This property is crucial for its use in electronic substrates, insulators, and components.
Mechanical Strength and Fracture Toughness: While alumina ceramic is inherently brittle, it exhibits high compressive strength and moderate fracture toughness. Advances in material science have enhanced its toughness, expanding its range of applications.
Biocompatibility: Alumina ceramic’s inert nature makes it biocompatible, suitable for medical implants and prosthetics. It does not provoke adverse reactions in the human body, ensuring its safety in biomedical applications.
Manufacturing Processes of Alumina Ceramic
The production of alumina ceramic involves several precise steps, each critical to achieving the desired properties and performance. The key stages in the manufacturing process include:
Raw Material Preparation: The primary raw material, high-purity alumina powder, is derived from bauxite ore through the Bayer process. The purity of the alumina powder is essential, especially for high-performance applications, as impurities can significantly affect the final properties.
Powder Processing: The alumina powder is mixed with additives to enhance its properties, such as sintering behavior and mechanical strength. This mixture is then granulated to form a uniform and flowable powder, suitable for shaping.
Shaping: Various techniques are employed to shape the alumina powder into the desired form. Common methods include uniaxial pressing, isostatic pressing, injection molding, and extrusion. The choice of shaping method depends on the complexity and size of the final component.
Sintering: The shaped green bodies are subjected to high temperatures (typically between 1400°C and 1800°C) in a controlled atmosphere during the sintering process. Sintering densifies the material, reducing porosity and enhancing its mechanical and thermal properties.
Finishing: After sintering, the ceramic components may undergo additional machining, grinding, and polishing to achieve precise dimensions and surface finishes. Advanced machining techniques are employed to produce complex geometries and tight tolerances.
Applications of Alumina Ceramic
The unique properties of alumina ceramic enable its use in a broad spectrum of applications across various industries:
Electronics and Electrical Engineering: Alumina ceramic serves as an essential material in the electronics industry, where it is used as a substrate for electronic circuits and components. Its excellent electrical insulation and thermal conductivity make it ideal for semiconductor devices, insulators, and dielectric layers in capacitors.
Mechanical and Industrial Applications: The high hardness and wear resistance of alumina ceramic make it suitable for cutting tools, bearings, seals, and pump components. It is also used as a lining material for industrial equipment to protect against wear and corrosion.
Biomedical and Dental Applications: In the medical field, alumina ceramic is used for orthopedic implants, dental crowns, and prosthetic components due to its biocompatibility and wear resistance. It is also employed in surgical instruments and diagnostic equipment.
Aerospace and Defense: The high-temperature stability and mechanical strength of alumina ceramic make it valuable in aerospace and defense applications. It is used in thermal protection systems, armor, and engine components that require resistance to extreme conditions.
Chemical Processing: Alumina ceramic’s chemical inertness makes it ideal for use in aggressive chemical environments. It is used in reactors, pipes, and vessels for chemical processing, where it provides excellent resistance to corrosion and wear.
Energy Sector: Alumina ceramic is employed in various energy applications, including insulators for high-voltage power transmission, components in nuclear reactors, and parts of fuel cells and batteries. Its electrical insulation and thermal stability are key to its performance in these applications.
Advantages of Alumina Ceramic
The widespread use of alumina ceramic is attributed to its numerous advantages:
Durability: The high hardness and wear resistance of alumina ceramic ensure a long service life and reduced maintenance costs in demanding applications.
Versatility: Its ability to be fabricated into complex shapes and sizes makes it adaptable for a wide range of applications, from simple components to intricate designs.
Cost-Effectiveness: While the initial cost of alumina ceramic components may be higher than some alternative materials, their longevity and performance often result in overall cost savings.
Environmental Resistance: The chemical stability and high-temperature resistance of alumina ceramic allow it to perform reliably in harsh environments, reducing the need for frequent replacements.
Biocompatibility: Its inert nature makes it suitable for medical applications, reducing the risk of adverse reactions and ensuring patient safety.
Future Prospects of Alumina Ceramic
The future of alumina ceramic is bright, with continuous research and development aimed at enhancing its properties and expanding its applications. Innovations in nanotechnology are expected to improve the mechanical and thermal properties of alumina ceramics, making them even more effective in advanced technologies. Additionally, advancements in additive manufacturing techniques, such as 3D printing, are poised to revolutionize the production of complex ceramic components, offering greater design flexibility and efficiency.
Conclusion
Alumina ceramic represents a pinnacle of engineering materials, combining a unique set of properties that make it indispensable across various industries. Its high hardness, wear resistance, chemical stability, and versatility ensure that it remains a material of choice for demanding applications. As technology continues to evolve, alumina ceramic is poised to play an even more critical role in advancing modern engineering and improving the performance and durability of countless products and systems.

0 notes
Text
Global Isostatic Pressing Market Report: Industry Size, Share, Statistics, Companies, and Growth Analysis – 2028
Isostatic pressing is a vital manufacturing process that plays a significant role in shaping various industries, from aerospace to automotive, ceramics to electronics. The Global Isostatic Pressing Report provides a comprehensive overview of the industry, delving into key aspects such as market size, share, statistics, prominent companies, and growth analysis.
Global Isostatic Pressing Market Size and Share:
Global isostatic pressing market report provides a comprehensive overview of the industry, delving into key aspects such as market size, share, statistics, prominent companies, and growth analysis. According to MarketsandMarkets latest research report, Isostatic pressing industry is expected to grow from USD 8.1 billion in 2023 to USD 11.7 billion by 2028, registering a CAGR of 7.6% during the forecast period.
Asia Pacific to grow at highest CAGR during the forecast period.
The robust growth of the isostatic pressing market in the Asia-Pacific region can be attributed to several key factors. These include the rapid pace of industrialization, new markets, technological advancements, the expanding automotive and aerospace sectors, the growth of electronics manufacturing, supportive government policies, and the increasing emphasis on energy and environmental technologies. Moreover, the increase in demand for low-cost and reliable metal and ceramic products for various applications and rising preference for custom implants (in medical applications) due to better and more efficient recovery are expected to propel the demand for isostatic pressing in the region.
Top Isostatic Pressing Companies – Key Market Players
The Isostatic pressing companies players have implemented various organic and inorganic growth strategies, such as product launches, collaborations, partnerships, and acquisitions, to strengthen their offerings in the market. The major players in the market are Kobe Steel, Ltd. (Japan), Bodycote (UK), Kennametal, Inc. (US), Nikkiso., Ltd. (Japan), DORST Technologies GmbH & Co. KG (Germany), American Isostatic Presses, Inc (US), EPSI (US), Pressure Technology, Inc. (US), Shanxi Golden Kaiyuan Co., Ltd. (China)
Download PDF Brochure: https://www.marketsandmarkets.com/pdfdownloadNew.asp?id=23702585
Isostatic Pressing Market Statistics and Growth Trends:
Detailed statistics are presented in the report, shedding light on the historical performance and current trends within the isostatic pressing industry. The analysis includes data on market revenue, production volume, and consumption patterns. Additionally, the report highlights growth trends, identifying key factors contributing to the expansion of the isostatic pressing market.
#Isostatic Pressing Market Report#Isostatic Pressing Industry#Global Isostatic Pressing Market#Isostatic Pressing Maret Share#Isostatic Pressing Market Size
0 notes
Text
Porous Titanium in Next-Generation PEMFCs

Porous titanium has found a wide variety of unique and important applications including filtration, separation, catalyst supporting, gas absorbing, gas sparging, current collection, and medical implantation.
Manufacture process for porous titanium
Raw titanium powder preparation—Cold isostatic pressing or rolling—Sintering in high temperature & vacuum furnace-Machined to size-Assembled to filter if required
Liquid/gas diffusion layers (LGDLs) play a crucial role in electrochemical energy technology and hydrogen production, and are expected to simultaneously transport electrons, heat, and reactants/products with minimum voltage, current, thermal, interfacial, and fluidic losses.
Porous titanium exhibits excellent properties when it is used as the anode GDL, because of its unique three-dimensional strut structure promoting highly efficient catalytic reactions. Furthermore, it shows superior corrosion resistance with almost no thickness and weight changes in the accelerated corrosion test, as opposed to considerable reductions in the weight and thickness of the conventional GDL.
Porous Titanium provides a longer-term reliability and chemical stability, which can reduce the loss of Pt catalyst and, hence, the cost of PEMFCs.
0 notes
Text
Argon Gases
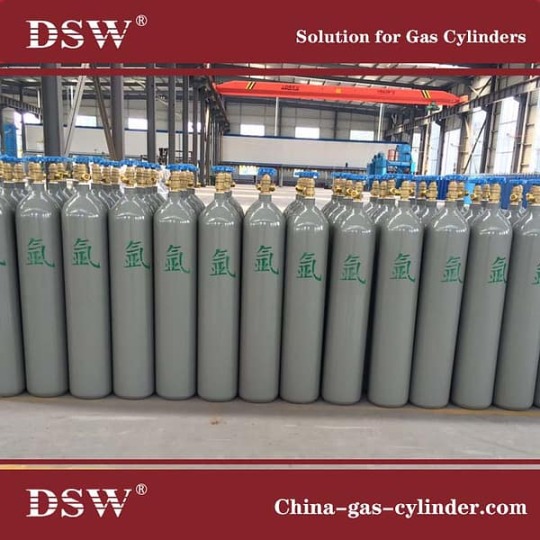
Argon Gases Supplier
Liquid Argon Gas(LAR) -Purity≧99.999% -O2 < 3ppm -N2 < 4ppm -Moisture < 3ppm Carrier gas for chromatography Sputtering in hard disk production Protecting against oxidation in viticulture DSW offers a full range of Argon Gases in various purity to suit your application and process needs with cost-effectiveness.
SKU: 99.997Categories: Argon Gas, GasesTags: argon Gas, industry argon gas
Description
Compressed Argon Gases
DSW Industry provides a wide range of argon gases in various cylinder, dewar, and cryogenic tank sizes and a variety of purity for all your industry needs. The capability of providing pure gases along with customized mixing of component gases to meet all your welding needs.
Most commonly used in the metal industry for metal production, processing, and fabrication, it can be used as a pure gas for shielding, blanketing, annealing, and hot isostatic pressing applications. Depending on the process and material, it can also be used as a mixture with other gases, particularly carbon dioxide, oxygen, nitrogen, hydrogen, or helium.
Properties of Argon
Limiting CharacteristicsCGA G-11.1TypicalType II, Grade CArgon Minimum, %99.99799.998Water10.53.5Dew Point, °F-76-90Oxygen52Nitrogen2010Hydrogen11Combined total hydrocarbons (as methane) and carbon dioxide33
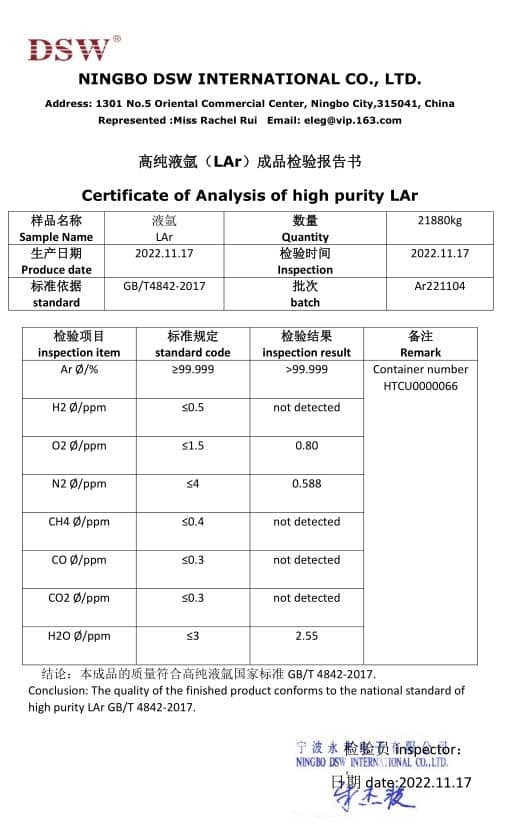
BUY COMPRESSED ARGON GAS OR LIQUID ARGON (AR)
DSW offers various sizes of argon gas in high-pressure gas cylinders and liquid argon dewars. Argon is an inert shield gas to enhance arc stability and gas metal arc welding characteristics. It is also the primary gas for the Gas Tungsten Arc Welding (GTAW) process.
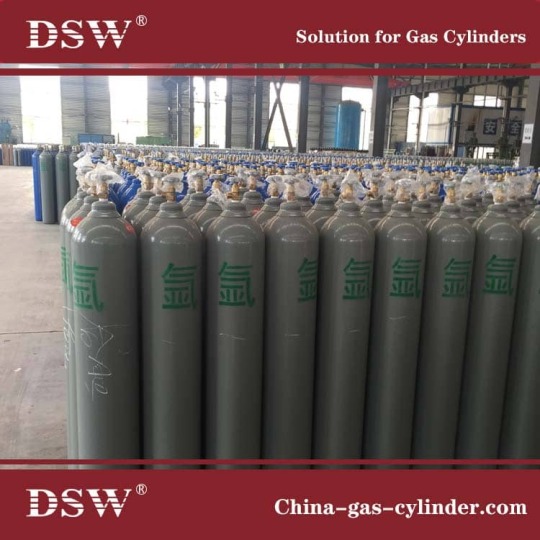
Uses of Argon
Neon Lights: Noble gases such as argon are widely used for creating neon lights, neon, and krypton. When electricity passes through an argon gas reservoir, it temporarily excites electrons in their outermost orbiting shells to jump to higher “shell” energy levels, then back again; upon their return to normal energy levels, they emit photons of light that radiate throughout space-time emitted as photons of photon light emission.
Shield Gas in Welding: Argon gas is used extensively for welding specialty alloys as well as automobile frames, mufflers, and other automotive parts. Argon serves as a shielding gas due to its nonreactive properties; Instead, it merely occupies space around where metals being welded are being assembled, thus preventing further unwanted reactions due to reactive gases like nitrogen and oxygen from happening nearby.
Heat Treating: Argon can provide an oxygen and nitrogen-free atmosphere for heat-treating processes, providing an environment free from oxygen or nitrogen contamination.
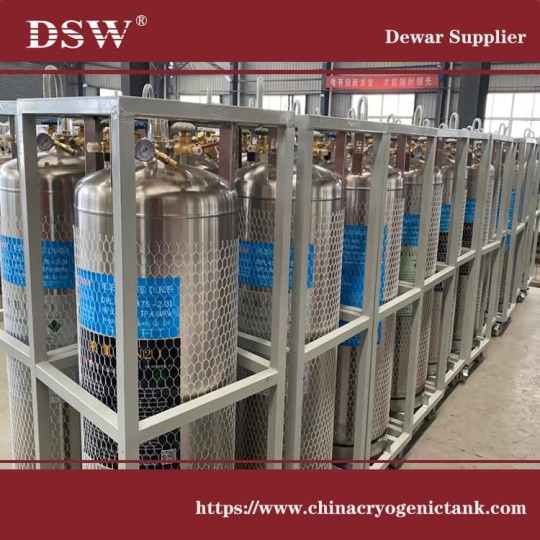
Argon may be referred to as “PLAR,” pure liquid argon, or “CLAR” (crude liquid argon), or by its chemical designation, “Ar.”
Crude argon is usually considered an intermediate product in a pure argon facility. Still, it may be a final product for some lower-capacity air separation plants, which ship it to more extensive facilities for final purification. Some crude argon is also sold as a final product for uses that do not need high-purity oxygen (e.g., some steelmaking and welding applications).
0 notes