#cryogenic liquid propulsion engines
Explore tagged Tumblr posts
Text
youtube
#general knowledge in tamil#gk on board#gkonboard#general knowledge#pothu arivu#kalanjiyam#behind earth#unsigned#tamil pokkisham#madan gowri#mr gk#seythikkural#building rockets#space travel#Agnikul Cosmos#Srinath Ravichandran#Moin SPM#aerospace#small rockets#launch vehicles#satellite launches#IIT Madras#3D printing#rocket engines#mobile pads#ISRO#3D printing process#two-stage rocket#cryogenic liquid propulsion engines#space exploration
1 note
¡
View note
Text
Hydrogen Rockets: The Key to Sustainable Space Exploration
Introduction to Hydrogen Rocket Engine
In the realm of space exploration, the quest for efficient propulsion systems has led to the development and utilization of hydrogen rocket engines. These engines harness the power of hydrogen, the most abundant element in the universe, to propel spacecraft into the cosmos with remarkable efficiency and power.
History of Hydrogen Rocket Engine Development
The concept of using hydrogen as a propellant dates back to the early days of rocketry. However, it wasn't until the mid-20th century that significant advancements were made in the development of hydrogen rocket engines. Pioneering work by scientists and engineers paved the way for the modern hydrogen propulsion systems we see today.
How Hydrogen Rocket Engines Work
Fuel Combustion Process
Hydrogen rocket engines operate on the principle of combustion. Liquid hydrogen is combined with liquid oxygen in a combustion chamber, where it undergoes a controlled explosion. This rapid combustion generates intense heat and pressure, producing a powerful stream of hot gases.
Thrust Generation
The expulsion of these hot gases through a nozzle at the rear of the rocket creates thrust according to Newton's third law of motion. This thrust propels the rocket forward, overcoming the forces of gravity and atmospheric resistance.
Advantages of Hydrogen Rocket Engines
Hydrogen rocket engines offer several key advantages over conventional propulsion systems:
High Efficiency: Hydrogen boasts one of the highest specific impulse values among rocket propellants, making it extremely efficient in terms of thrust per unit of propellant mass.
Clean Combustion: The combustion of hydrogen with oxygen produces water vapor as a byproduct, resulting in cleaner emissions compared to traditional rocket fuels.
Abundant Resource: Hydrogen is abundant in the universe, making it a sustainable and readily available resource for space exploration endeavors.
Challenges and Limitations
Despite its many advantages, hydrogen rocket technology also faces significant challenges and limitations.
Cryogenic Storage
One of the primary challenges associated with hydrogen rocket engines is the need for cryogenic storage. Liquid hydrogen must be kept at extremely low temperatures to remain in a liquid state, requiring specialized storage and handling systems.
Cost and Infrastructure
The infrastructure required to produce, store, and transport liquid hydrogen adds to the overall cost of hydrogen rocket technology. Additionally, the development of hydrogen propulsion systems necessitates substantial investments in research and development.
Applications of Hydrogen Rocket Engines
Hydrogen rocket engines find a wide range of applications in space exploration and satellite deployment missions.
Space Exploration
Hydrogen-powered rockets enable spacecraft to travel vast distances across the solar system, facilitating missions to explore distant planets, moons, and celestial bodies.
Satellite Deployment
The high efficiency and reliability of hydrogen rocket engines make them ideal for launching satellites into orbit around the Earth and beyond.
Comparison with Traditional Rocket Engines
Compared to traditional rocket engines fueled by kerosene or solid propellants, hydrogen rocket engines offer superior performance and environmental benefits. They deliver higher specific impulse and produce cleaner emissions, contributing to a more sustainable approach to space exploration.
Environmental Impact and Sustainability
The environmental impact of hydrogen rocket engines is relatively minimal compared to conventional propulsion systems. The use of hydrogen as a fuel results in cleaner combustion and reduced greenhouse gas emissions, aligning with efforts to mitigate the environmental footprint of space exploration activities.
Future Prospects and Developments
As technology advances and our understanding of hydrogen propulsion deepens, the future holds great promise for hydrogen rocket engines. Ongoing research and development efforts aim to enhance efficiency, reduce costs, and overcome existing limitations, paving the way for new frontiers in space exploration.
Conclusion
Hydrogen rocket engines represent a cornerstone of modern space exploration, offering unparalleled efficiency, reliability, and environmental sustainability. While challenges remain, ongoing advancements in technology and infrastructure continue to expand the horizons of human spaceflight and scientific discovery.
FAQs
Are hydrogen rocket engines more powerful than traditional rocket engines? Hydrogen rocket engines typically offer higher specific impulse values, making them more efficient in terms of thrust per unit of propellant mass.
What are the main challenges associated with hydrogen rocket technology? Cryogenic storage and infrastructure costs are among the primary challenges facing hydrogen rocket technology.
What are the environmental benefits of hydrogen rocket engines? Hydrogen combustion produces cleaner emissions compared to traditional rocket fuels, contributing to reduced environmental impact.
What are the primary applications of hydrogen rocket engines? Hydrogen rocket engines are used in space exploration missions and satellite deployment operations.
What does the future hold for hydrogen rocket technology? Ongoing research and development efforts aim to improve efficiency, reduce costs, and expand the capabilities of hydrogen rocket engines.
#Hydrogen propulsion#Rocket engine technology#Space exploration#Liquid hydrogen#Rocket propulsion systems#Sustainable propulsion#Cryogenic storage#Spacecraft propulsion#Rocket engine efficiency#Environmental sustainability
0 notes
Text


Completed experiments on International Space Station to help answer how boiling and condensation work in space
After a decade of preparation and two years of active experiments in space, a facility that Purdue University and NASA's Glenn Research Center in Cleveland designed, built and tested has completed its test campaign on the International Space Station.
The Flow Boiling and Condensation Experiment (FBCE), led by Purdue professor Issam Mudawar, gathered vital data for engineering innovations to help astronauts travel farther into space and conduct long-duration missions on the moon or Mars. The data will also support the design of future spacecraft propulsion, thermal management systems, in-space refueling and vapor compression heat pumps for planetary bases.
"We were asked to create an experiment to test flow boiling in microgravity, and I'm thrilled to see that we've delivered in a big way," said Mudawar, the Betty Ruth and Milton B. Hollander Family Professor of Mechanical Engineering and FBCE's principal investigator. "The amount of data coming out of the FBCE is just absolutely enormous, and that's exactly what we want."
Mudawar's space-based experiment tackles a simple question: How do we keep future vehicles and facilities cool in space's harsh environment? For example, the moon's surface fluctuates wildly between minus 410 degrees and 250 degrees Fahrenheit; to create a human habitat there, reliable thermal management will be essential.
Traveling farther into space requires cryogenic fuel depots, but the flow physics of cryogenic liquids in microgravity haven't been fully understood. Even future propulsion systems, such as nuclear fission or Rankine cycle heat engines, will also require efficient cooling systems capable of operating in a zero-gravity environment.
For all these reasons, NASA solicited research proposals on the topic and selected a proposal submitted by Mudawar, who is one of the most cited researchers on flow boiling and condensation, and Glenn's Mojib Hasan.
In flow boiling, heat is removed by flowing a liquid that then boils, phase-changing into a gas, and then later condensing back into a liquid. While this efficient thermal management solution has been studied in-depth on Earth, no one knew if it was feasible in space.
"We have developed over a hundred years' worth of understanding of how heating and cooling systems work in Earth's gravity," Mudawar said, "but we haven't known how they work in weightlessness."
Enter the FBCE, which started operating on the space station in 2022. About the size of a refrigerator, FBCE contains two Purdue-designed test modulesâone for flow boiling and one for condensationâintegrated with a fluid system that Purdue researchers and NASA Glenn designed together. NASA Glenn built the fluid system along with avionics modules. Sitting in the space station's Fluids Integrated Rack, the fluid system enables researchers to conduct space station experiments autonomously from the ground.
"The FBCE experiments are crucial in two ways," Mudawar said. "Not only do they generate fundamental data that would be difficult for us to gather on Earth, but they also serve to validate theoretical models that we have spent years developing. Once the models are validated, it becomes possible for NASA to more accurately predict the fluid physics of lower-gravity environments, such as the moon or Mars."
Boiler up
Mudawar started this project in 2011 when NASA selected his proposal after a decadal survey from the National Academies emphasized the need for this research.
"Every 10 years, the National Academies conduct a survey that advises NASA what specific aspects they should focus on," Mudawar said. "This report indicated a lack of fundamental models of how fluids behave in microgravity. That's when I began work with NASA Glenn to create an experimental facility to study fluid flow and phase change in space."
The first prototype FBCE flew on parabolic airplane flights with Zero Gravity Corp. (ZERO-G). Purdue students and NASA Glenn-qualified operators conducted experiments on that flight during 15-second periods of microgravity. After promising results and years of hardware development, testing and flight qualification, the final version of the facility came together at NASA Glenn.
After passing NASA's design, safety and readiness reviews, FBCE's flow boiling module was launched to the space station in August 2021 and began operations in early 2022. Last August, the condensation module of FBCE launched, replacing the flow boiling module. Purdue completed its test campaign in July.
The mountains of data gathered from FBCE's experiments will form the basis of fundamental models and future research for years to come.
"This is among NASA's largest and most complex experiments for fluid physics research," Mudawar said. "We have published more than 70 papers on reduced gravity and fluid flow since the project's inception and even contributed to the most recent National Academies decadal survey based on our findings. Collectively, these papers are really almost like a textbook for how boiling and condensation works in space."
Cool future
While the Purdue test campaign has ended, FBCE isn't going anywhere. The facility will remain on the space station for other researchers who want to experiment with fluid physics in space.
"My original proposal established that FBCE would eventually become a national resource," Mudawar said. "Other investigators have already started using it since our campaign ended. It's my hope that the data coming from FBCE will help establish the science of cooling spacecraft for years to come."
TOP IMAGE: NASA astronaut Frank Rubio services the Flow Boiling and Condensation Experiment (FBCE) on the International Space Station, designed, built and tested by Purdue and NASA's Glenn Research Center. While its official test campaign has concluded, FBCE is staying in space and is available for other organizations to conduct zero-gravity fluid dynamics experiments. Credit: NASA
LOWER IMAGE: The second module of the Flow Boiling and Condensation Experiment launched to the International Space Station last August among other cargo for NASA. Credit: NASA/Danielle Johnson
3 notes
¡
View notes
Text
Hydrogen Storage Market is valued at approximately USD 8,356.06 million and is projected to grow at a compound annual growth rate (CAGR) of 23% over the forecast period, reaching around USD 15,595 million by 2032.The hydrogen storage market is at the forefront of the global transition to sustainable energy. With the increasing emphasis on decarbonization and renewable energy sources, hydrogen has emerged as a critical energy carrier. Hydrogen storage solutions are essential for facilitating the adoption of hydrogen in applications spanning transportation, power generation, and industrial processes. This market is experiencing rapid growth, fueled by advancements in storage technologies, supportive government policies, and rising investments in green hydrogen projects.
Browse the full report https://www.credenceresearch.com/report/hydrogen-storage-market
Market Dynamics
Market Growth and Potential The hydrogen storage market is projected to witness significant growth over the next decade. This expansion is driven by the rising demand for hydrogen as a clean fuel alternative and the need for robust storage solutions that support its safe and efficient utilization. The development of large-scale green hydrogen production facilities is further propelling the demand for advanced storage technologies. As nations aim to achieve their net-zero emissions targets, hydrogen storage infrastructure is becoming a vital component of their energy strategies.
Technological Advancements Innovations in storage technologies are transforming the hydrogen market landscape. Storage methods such as compressed gas, liquid hydrogen, and solid-state storage materials are gaining traction due to their efficiency and scalability. Compressed hydrogen, for example, is widely used in fuel cell vehicles and portable power systems. Meanwhile, advancements in cryogenic storage have enhanced the feasibility of liquid hydrogen for large-scale transportation and aerospace applications. Solid-state hydrogen storage, leveraging metal hydrides and chemical compounds, offers promising potential for long-term storage with high energy densities.
Key Market Drivers
Decarbonization Initiatives and Energy Transition Governments and industries worldwide are prioritizing decarbonization to combat climate change. Hydrogen is a versatile energy carrier that can be utilized in hard-to-abate sectors such as heavy industry, shipping, and aviation. Investments in hydrogen storage systems are being driven by the need to ensure a steady supply of hydrogen to meet growing demand, particularly in renewable energy integration and industrial applications.
Expansion of Fuel Cell Electric Vehicles (FCEVs) The growing adoption of FCEVs has intensified the demand for hydrogen storage solutions. Fuel cell technology relies on stored hydrogen to generate electricity for propulsion, offering a clean alternative to conventional internal combustion engines. Major automotive manufacturers and governments are investing in hydrogen refueling infrastructure, further boosting the market for hydrogen storage.
Government Support and Policies Supportive policies and funding initiatives are playing a pivotal role in accelerating hydrogen storage deployment. Governments are implementing subsidies, grants, and tax incentives to encourage the adoption of hydrogen technologies. For instance, the European Unionâs Hydrogen Strategy aims to establish a comprehensive hydrogen ecosystem, while countries like Japan and South Korea have set ambitious targets for hydrogen storage and utilization.
Challenges and Opportunities
Despite its promising outlook, the hydrogen storage market faces challenges such as high costs and safety concerns. Developing cost-effective and scalable storage solutions remains a priority for stakeholders. The industry is also addressing safety issues related to hydrogenâs flammability and high-pressure storage requirements by advancing materials and system designs.
Opportunities lie in expanding infrastructure for hydrogen refueling and storage. Innovations in modular and transportable storage systems are enabling flexible deployment in remote areas and off-grid applications. Furthermore, the integration of hydrogen storage with renewable energy sources, such as wind and solar, presents a compelling use case for balancing energy supply and demand.
Future Outlook
The future of the hydrogen storage market looks promising, with continued advancements in technology and supportive policy frameworks. By 2030, the market is expected to witness substantial growth, driven by widespread adoption across various sectors. Emerging trends, such as the development of hydrogen-powered aviation and marine transportation, will further propel the demand for innovative storage solutions.
Key Players:
Air Products and Chemicals, Inc.
Linde plc
Nel ASA
Plug Power Inc.
Ballard Power Systems Inc.
Cummins Inc.
Toyota Motor Corporation
Siemens AG
Hexagon Composites ASA
Hyzon Motors Inc.
Segmentations:
Based on Product Type:
Compressed Hydrogen Storage
Liquid Hydrogen Storage
Solid-State Hydrogen Storage
Based on Technology:
Physical Storage
Chemical Storage
Hybrid Systems
Based on End-User:
Transportation
Power Generation
Industrial Processes
Residential Applications
Based on Region:
North America
United States
Canada
Europe
United Kingdom
Germany
France
Italy
Asia-Pacific
China
India
Australia
Japan
Latin America
Brazil
Mexico
Argentina
Middle East and Africa
South Africa
United Arab Emirates
Israel
Browse the full report https://www.credenceresearch.com/report/hydrogen-storage-market
Contact:
Credence Research
Please contact us at +91 6232 49 3207
Email:Â [email protected]
Website:Â www.credenceresearch.com
0 notes
Text
The Important Role of Orbital Welding in the Aerospace Industry
What is Orbital Welding?
Orbital welding is a process where the welding arc is rotated circumferentially around a workpiece, typically a tube or pipe, in a continuous motion. The process is usually automated and highly controlled, ensuring that each weld is consistent, accurate, and free from defects. Orbital welding systems consist of a welding power supply, control system, and an orbital head that rotates the welding tool around the workpiece. It was initially developed in the 1960s for the aerospace sector to meet the stringent demands for weld precision and reliability.
The Growing Need for Orbital Welding in Aerospace
The aerospace industry is marked by the production of high-performance components that must withstand extreme conditions, including high temperatures, pressure fluctuations, and mechanical stresses. As aircraft and spacecraft technology has evolved, so too have the demands for greater fuel efficiency, lighter materials, and stronger structures. Orbital welding fits into this environment by providing a welding process that meets these high demands in several critical ways:
Precision and Accuracy: Aerospace components require incredibly tight tolerances and welds with minimal error margins. Orbital welding offers a high degree of precision, which is essential in the aerospace sector where even the slightest defect in a weld could lead to catastrophic failure.
Repeatability and Consistency: In automated orbital welding, every weld is identical, ensuring that each component fabricated in a series maintains the same level of integrity. This consistency is crucial for mass production of aerospace parts, ensuring that each part performs as expected.
Weld Quality: Aerospace applications require welds to be extremely strong and free from imperfections such as cracks, voids, or inclusions. Orbital welding, being a controlled process, minimizes the risk of these defects by maintaining consistent heat input, travel speed, and weld positioning throughout the entire operation.
Safety: Given the extreme environments that aerospace components are subjected to, safety is of utmost importance. Failures in weld joints can lead to dangerous consequences, especially in space applications or in flight. Orbital welding significantly enhances the reliability and safety of the welded joints.
Applications of Orbital Welding in Aerospace
Orbital welding has a wide range of applications in the aerospace industry, from building aircraft fuselages to assembling rocket propulsion systems. Some of the most notable applications include:
Tubes and Piping Systems: Aircraft and spacecraft are equipped with complex piping systems used for fuel delivery, hydraulic systems, and cooling systems. Orbital welding is ideal for joining thin-walled tubes made from materials such as stainless steel, titanium, and nickel alloys that are commonly used in these systems. The precision and control offered by orbital welding ensures that the piping systems can withstand high pressures and temperatures.
Propulsion Systems: Rocket engines and jet engines involve intricate pipework and components that must be able to withstand extreme conditions. Orbital welding is used extensively in the construction of these systems due to its ability to produce clean, high-strength welds. The automated nature of orbital welding also minimizes the risk of human error in these critical components.
Structural Components: The frames and structural components of spacecraft, satellites, and airplanes often require high-quality welds that provide strength without adding unnecessary weight. Orbital welding allows for the creation of lighter, stronger structures by ensuring consistent, high-strength joints across various sections.
Fuel and Cryogenic Systems: Orbital welding is also used to manufacture fuel systems, including cryogenic tanks that store liquid hydrogen and oxygen in space missions. These tanks and their associated piping must be leak-proof and able to operate under ultra-cold temperatures, making orbital welding the preferred method due to its precision.
Maintenance and Repair: In addition to manufacturing, orbital welding is also employed in the maintenance and repair of aerospace systems. For example, when tubing in fuel delivery or hydraulic systems requires repair, orbital welding can be used to precisely weld the replacement parts, ensuring that the integrity of the system is maintained.
Advantages of Orbital Welding in Aerospace
The advantages of orbital welding extend far beyond its precision and consistency. Several other benefits make it the welding method of choice for many aerospace applications:
Automation: The automated nature of orbital welding reduces the dependency on manual labor, which is particularly important in the aerospace industry where highly skilled welders are often in short supply. The automation ensures that welds are made with minimal human intervention, reducing the possibility of human error.
Material Compatibility: Aerospace materials often include high-strength, temperature-resistant alloys such as Inconel, titanium, and aluminum. Orbital welding is well-suited for these materials, producing high-quality welds that meet stringent aerospace standards.
Time Efficiency: Automation also reduces welding time, as orbital welding systems can operate continuously and more rapidly than manual welding. This increase in production efficiency is vital for meeting the aerospace industryâs tight deadlines, particularly when building or maintaining large aircraft fleets or spacecraft systems.
Environmental Control: Orbital welding is often performed in environments with tight control over factors like temperature, humidity, and contaminants. This level of control is essential for aerospace welding, where even minor contaminants can compromise the integrity of a weld, potentially leading to failure during flight or in space missions.
Cost-Effectiveness: Although the initial investment in orbital welding equipment may be higher, the long-term benefits often result in lower costs. The reduced labor requirements, increased efficiency, and higher reliability of the welds help lower overall production costs. Additionally, the reduced risk of part failure due to poor weld quality can save money in terms of repairs and replacements.
Challenges and Future Prospects
Despite its many benefits, orbital welding does have some challenges. For example, the high level of orbital welding training required to operate and program orbital welding machines can present a barrier to entry. Furthermore, the initial cost of orbital welding equipment is significant, though it is often justified by the long-term savings and improved weld quality.
Looking ahead, advancements in automation and robotics are likely to further improve the capabilities of orbital welding in aerospace applications. Innovations such as artificial intelligence (AI) and machine learning may be integrated into orbital welding systems to optimize welding parameters in real-time, leading to even greater precision and efficiency. Additionally, as new aerospace materials are developed, orbital welding techniques will continue to evolve to accommodate these advanced materials.
Conclusion
Orbital welding has become an essential technology in the aerospace sector due to its unmatched precision, consistency, and quality. From rocket engines to aircraft hydraulic systems, this automated welding process ensures that critical aerospace components meet the industryâs demanding safety and performance standards. As the aerospace industry continues to evolve, so too will the importance of orbital welding, supporting the creation of increasingly advanced and reliable aircraft and spacecraft systems.
#orbital welding training#orbital welding technology#orbital welding courses#orbital welding#welding technology
0 notes
Text
Hydrogen Rocket Engine Market Development and Future Demand Analysis Report 2030
The aerospace industry is entering a revolutionary phase, with the Hydrogen Rocket Engine Market emerging as a crucial driver of future space exploration. As countries and private companies aim to push the boundaries of space travel, the demand for advanced propulsion systems is growing exponentially. Among these, hydrogen-powered rocket engines are gaining significant attention due to their efficiency, environmental sustainability, and potential to fuel long-distance space missions.
Hydrogen rocket engines use liquid hydrogen (LH2) as fuel, combined with an oxidizer, typically liquid oxygen (LOX), to produce thrust. When these two elements combust, they create a high-velocity exhaust that propels the rocket forward. What makes hydrogen-based engines unique is their high specific impulse, meaning they provide more thrust per unit of propellant compared to other types of rocket engines, such as those powered by kerosene or solid fuel.
Read More about Sample Report: https://intentmarketresearch.com/request-sample/hydrogen-rocket-engine-market-3027.html
Market Drivers: Efficiency and Sustainability
One of the main reasons for the growing interest in hydrogen rocket engines is their energy efficiency. Liquid hydrogen provides one of the highest energy-to-mass ratios among rocket fuels, enabling longer missions with less fuel. This makes hydrogen engines ideal for deep-space exploration missions, including trips to the Moon, Mars, and beyond.
Moreover, hydrogen combustion primarily produces water vapor as a byproduct, making these engines more environmentally friendly compared to traditional carbon-based rocket fuels. As environmental concerns continue to shape aerospace policies, the adoption of cleaner propulsion technologies like hydrogen engines is likely to accelerate.
Key Market Segments and Applications
Type of Engine: Liquid Hydrogen-Liquid Oxygen (LH2/LOX) engines and hybrid engines.
Application: Manned space missions, satellite launches, cargo transport, and planetary exploration.
End Users: Government space agencies (NASA, ESA), private aerospace companies (SpaceX, Blue Origin), and emerging space programs in developing nations.
In particular, the commercial space sector is experiencing rapid growth, driven by ventures like SpaceX, Blue Origin, and Rocket Lab, all of which are investing in hydrogen engine technology to lower costs and improve mission capabilities.
Challenges Facing the Hydrogen Rocket Engine Market
Despite its promise, the hydrogen rocket engine market faces several challenges:
Cost: Producing, storing, and transporting liquid hydrogen requires advanced infrastructure and technologies, which are costly and complex. However, ongoing research is focused on reducing these costs.
Storage and Handling: Hydrogen, particularly in liquid form, needs to be stored at extremely low temperatures (-253°C), posing engineering challenges. Special cryogenic tanks and insulation materials are required, which add to the weight and cost of spacecraft.
Infrastructure: The current aerospace infrastructure is not fully equipped to handle large-scale hydrogen refueling, though companies and governments are working to develop hydrogen-based fueling systems.
Key Players in the Hydrogen Rocket Engine Market
Several aerospace giants and startups are currently leading the hydrogen rocket engine market:
NASA has been a pioneer in using liquid hydrogen in rocket engines, with its RS-25 engines (used in the Space Shuttle program) and the Space Launch System (SLS) being key examples.
SpaceX is exploring hydrogen as a potential fuel for future Mars missions, though it primarily focuses on methane engines currently.
Blue Originâs BE-3 engine uses liquid hydrogen, demonstrating its potential for future human spaceflight missions.
Ask for Customization Report: https://intentmarketresearch.com/ask-for-customization/hydrogen-rocket-engine-market-3027.html
Future Prospects and Opportunities
The global demand for sustainable and efficient propulsion systems is expected to drive the hydrogen rocket engine market's growth over the next decade. As companies and space agencies continue to innovate, there is potential for significant advancements in cryogenic technologies, fuel efficiency, and space infrastructure to support hydrogen-based missions.
Additionally, the growing interest in space tourism and interplanetary exploration will likely expand the market for hydrogen engines. Private companies and space agencies alike are keen on reducing the cost of access to space, and hydrogen engines, with their superior performance and long-term sustainability, are at the forefront of this new space age.
Conclusion
The hydrogen rocket engine market represents a critical innovation in the aerospace industry, with the potential to revolutionize space exploration and transportation. As the technology advances and infrastructure challenges are addressed, hydrogen engines will likely play a leading role in propelling humanity toward deeper exploration of the solar system and beyond.
With environmental sustainability becoming a key focus and the continued push for cost-effective space missions, the hydrogen rocket engine market is poised for substantial growth in the coming years.
#Hydrogen Rocket Engine#Hydrogen Rocket Engine Size#Hydrogen Rocket Engine Trends#Hydrogen Rocket Engine Growth
0 notes
Text
Agnibaan Test Mission Postponed by Agnikul Just Before Launch
For the third consecutive time, Agnikul, a pioneering private aerospace firm, has postponed the inaugural test launch of its Agnibaan SOrTeD rocket, due to technical challenges as the primary reason for the delay. The planned launch from India's first private launchpad, ALP-01, situated at the Satish Dhawan Space Centre in Sriharikota, was abruptly halted just moments before liftoff.
The SOrTeD mission, an abbreviation for Sub Orbital Technology Demonstrator, entails showcasing a single-stage launch vehicle propelled by a semi-cryogenic engine known as the Agnilet. Developed domestically, this engine employs a sub-cooled liquid oxygen-based propulsion system, marking a significant technological leap for India's aerospace industry.
Initially slated for Saturday, the test launch was rescheduled for Sunday as the aerospace company undertook attentive pre-launch checks, uncovering unforeseen technical issues that necessitated further investigation and resolution.
Originating from IIT-Madras, the rocket manufacturer refrained from providing any specific explanations for the postponement of the Agnibaan launch when it was initially postponed in March, leaving industry observers speculating about the underlying challenges.
1.  A Revised Date
As of now, Agnikul has not disclosed a revised launch date for Agnibaan, a versatile two-stage launch vehicle designed to accommodate payloads of up to 300 kg into an orbit approximately 700 km above Earth.
To Read More Click here...
0 notes
Link
All the major structures that will form the core stage for NASAâs SLS (Space Launch System) rocket for the agencyâs Artemis III mission are structurally complete. Technicians finished welding the 51-foot liquid oxygen tank structure, left, inside the Vertical Assembly Building at NASAâs Michoud Assembly Facility in New Orleans Jan. 8. The liquid hydrogen tank, right, completed internal cleaning Nov. 14. NASA/Michael DeMocker As NASA works to develop all the systems needed to return astronauts to the Moon under its Artemis campaign for the benefit of all, the SLS (Space Launch System) rocket will be responsible for launching astronauts on their journey. With the liquid oxygen tank now fully welded, all of the major structures that will form the core stage for the SLS rocket for the agencyâs Artemis III mission are ready for additional outfitting. The hardware will be a part of the rocket used for the first of the Artemis missions planning to land astronauts on the Moonâs surface near the lunar South Pole. Technicians finished welding the 51-foot liquid oxygen tank structure inside the Vertical Assembly Building at NASAâs Michoud Assembly Facility in New Orleans Jan. 8. The mega rocketâs other giant propellant tank â the liquid hydrogen tank â is already one fully welded structure. NASA and Boeing, the SLS core stage lead contractor, are currently priming the tank  in another cell within the Vertical Assembly Building area called the Building 131 cryogenic tank thermal protection system and primer application complex. It completed internal cleaning Nov. 14. Manufacturing hardware is a multi-step process that includes welding, washing, and, later, outfitting hardware.The internal cleaning process is similar to a shower to ensure contaminants do not find their way into the stageâs complex propulsion and engine systems prior to priming. Once internal cleaning is complete, primer is applied to the external portions of the tankâs barrel section and domes by an automated robotic tool. Following primer, technicians apply a foam-based thermal protection system to shield it from the extreme temperatures it will face during launch and flight while also regulating the super-chilled propellant within. âNASA and its partners are processing major hardware elements at Michoud for several SLS rockets in parallel to support the agencyâs Artemis campaign,â said Chad Bryant, acting manager of the Stages Office for NASAâs SLS Program. âWith the Artemis II core stage nearing completion, the major structural elements of the SLS core stage for Artemis III will advance through production on the factory floor.â The two massive propellant tanks for the rocket collectively hold more than 733,000 gallons of super-chilled propellant. The propellant powers the four RS-25 engines and must stay extremely cold to remain liquid. The core stage, along with the RS-25 engines, will produce two million pounds of thrust to help launch NASAâs Orion spacecraft, astronauts, and supplies beyond Earthâs orbit and to the lunar surface for Artemis III. SLS is the only rocket that can send Orion, astronauts, and supplies to the Moon in a single launch. Through Artemis, NASA will send astronautsâincluding the first woman, first person of color, and first international partner astronautâto explore the Moon for scientific discovery, economic benefits, and to build the foundation for crewed mission to Mars. SLS is part of NASAâs backbone for deep space exploration, along with the Orion spacecraft, exploration ground systems, advanced spacesuits and rovers, Gateway, and human landing systems. For more on SLS, visit: https://www.nasa.gov/humans-in-space/space-launch-system/ News Media Contact Corinne BeckingerMarshall Space Flight Center, Huntsville, [email protected]
0 notes
Text
Innovations Afloat: Propelling the Future of LNG Carrier Technology
LNG Carrier Market: Navigating the Global Gas Transport LandscapeÂ
Introduction: An LNG (Liquid Natural Gas) carrier is purpose-built for transporting liquefied natural gas in its cryogenic tanks. Through a cooling process, natural gas is transformed into liquid form at extremely low temperatures, typically around â163°C (â261°F). These vessels function as massive thermoses, maintaining the gas in its liquid state during transportation. Equipped with advanced propulsion systems and safety features, LNG carriers play a pivotal role in the global energy transportation sector.Â
Request Sample Report : https://www.alliedmarketresearch.com/request-toc-and-sample/9672 Â
Impact of COVID-19: The COVID-19 pandemic has significantly impacted the LNG carrier market. Plummeting fuel prices have affected LNG producers, disrupting the market. Restrictions on national and international transport have hampered the operations of LNG carriers. This scenario poses challenges that the industry must overcome to regain stability and growth.Â
Top Influencing Factors: Market Dynamics, Trends, Drivers, and Impact Analysis: The surge in global population has heightened the demand for natural gas as a fuel source, driving the growth of the LNG carrier market. Considered a safe and convenient energy form, LNG is witnessing increased demand due to governmental regulations aimed at emission reduction. Asia-Pacific is anticipated to lead the market, driven by growing LNG demand in countries like India, China, and Japan. In North America, onshore and offshore activities contribute to market growth, while Europe experiences steady growth with increased exploration for natural gas deposits.Â
Key Benefits of the Report:Â
Analytical Insight:Â
In-depth analysis of the LNG carrier market's current trends and future projections.Â
Market Drivers and Constraints:Â
Identification of key drivers, restraints, and opportunities, offering strategic insights.Â
Quantitative Market Analysis:Â
Quantitative analysis from 2020 to 2027 to highlight growth scenarios.Â
Competitive Analysis:Â
Porterâs five forces analysis illustrating buyer and supplier potency.Â
Detailed competitive intensity analysis for informed decision-making.Â
Request for Customization of This Report at : https://www.alliedmarketresearch.com/request-for-customization/9672 Â
LNG Carrier Market: Global Opportunity Analysis and Industry Forecast, 2020â2027 Report Highlights:Â
By Containment Type:Â
Moss TypeÂ
Membrane TypeÂ
GTT TechnologyÂ
96 System (Gaz Transport System)Â
Mark III System (Technigaz System)Â
By Storage Capacity:Â
Under 120,000 cubic metersÂ
120,000-160,000 cubic metersÂ
Above 160,000 cubic metersÂ
đđ§đŞđŽđ˘đŤđ˛ đđđđ¨đŤđ đđŽđ˛đ˘đ§đ :  https://www.alliedmarketresearch.com/purchase-enquiry/9672
By Region:Â
North America (U.S., Canada)Â
Europe (Germany, UK, France, Rest of Europe)Â
Asia-Pacific (China, Japan, India, Rest of Asia-Pacific)Â
Latin America (Brazil, Mexico, Rest of LATAM)Â
The Middle EastÂ
AfricaÂ
Key Market Players:Â
Royal Dutch Shell Plc.Â
Mitsui O.S.K. Lines, Ltd. (MOL)Â
Dynagas LtdÂ
STX Offshore & Shipbuilding Co. Ltd.Â
GasLog LtdÂ
China State Shipbuilding CorporationÂ
Daewoo Shipbuilding and Marine Engineering (DSME)Â
Hyundai Heavy Industries Co.Â
Mitsubishi Heavy IndustriesÂ
Kawasaki Heavy IndustriesÂ
Samsung Heavy IndustriesÂ
0 notes
Text
Cryofuels Come Under Pressure - Technology Org
New Post has been published on https://thedigitalinsider.com/cryofuels-come-under-pressure-technology-org/
Cryofuels Come Under Pressure - Technology Org
A carbon composite tank developed for NASA enables the move from fossil fuels to cryofuels.
Replacing jet fuel with hydrogen would be good for business and the environment. Hydrogen generates no carbon emissions and packs three times the energy per pound of hydrocarbon fuel, but there are some problems.
One of the biggest is that current airplane fuel tanks canât safely hold hydrogen. A solution is the carbon-fiber Blended Hybrid Laminate (BHL) cryotank developed by Gloyer-Taylor Laboratories (GTL) Inc. of Tullahoma, Tennessee.
Landing on a lunar surface is risky, and making the craft as light as possible is only one of many requirements. The mid-sized lander concept in this illustration, which would deliver a rover to the polar regions of the Moon, could benefit from a lightweight carbon composite cryofuel tank developed by Gloyer-Taylor Laboratories. Image credit: NASA
While NASA is testing it to carry cryofuels â particularly liquid oxygen â into space, a commercial airline is interested in the fact that the BHL can hold ultra-cold fuels without developing the microcracks that shorten the lifespan of other carbon composite tanks.
Hydrogen has one of the lowest boiling points of any element, meaning its liquid form must be kept extremely cold, or it needs to be kept under remarkably high pressure.
That requires a strong tank, usually made of steel or aluminum. Paul Gloyer, president of GTL, said breakthroughs in the use of carbon fiber and resin to wrap tanks have made them lighter than equivalent metal tanks. He wanted them to be even lighter.
A proprietary combination of materials and a manufacturing process developed by the company has produced a new type of carbon composite tank for cryofuel storage with walls thinner than a business card. Gloyer describes the BHL cryotank as a âstiff balloon.â
This unique carbon composite tank for cryofuels developed by Gloyer-Taylor Laboratories with NASA support is lightweight, making it ideal for aviation. And itâs designed to hold cryogenic fuels under extreme pressure, so it can be used to hold hydrogen and replace fossil fuels, eliminating greenhouse gas emissions. Image credit: Gloyer-Taylor Laboratories Inc.
It can withstand repeated cycles of pressurization and thermal changes that occur as the cryofuel tank is filled and emptied without developing the microcracks that result in dangerous leaks in other carbon tanks. The flexible nature of the material and thin construction mean the tank can hold more fuel per pound of tank mass.
A measurement of the force that leads tanks to crack is called microstrain. Conventional carbon-fiber cryotanks without liners tend to leak at about 3,000-5,000 microstrain. Testing has shown that the BHL tank can withstand more than 20,000 microstrain at cryogenic temperatures, said Gloyer.
Partly funded by multiple Small Business Innovation Research contracts from several NASA field centers including Marshall Space Flight Center in Huntsville, Alabama, the BHL is undergoing assessment for commercial space applications at the center.
The BHL technology has the potential to reduce cryofuel tank mass by 30% to 50% for small spacecraft propulsion systems, according to John Peugeot, a propulsion engineer at Marshall.
One space company is evaluating the new lightweight, high-capacity tank for its commercial lunar lander, according to Gloyer. A significant benefit of using the smaller, lighter tank will be the craftâs capacity to carry more technology and science payloads.
Meanwhile, several aircraft developers are combining the tank with hydrogen fuel cells and an electric engine to provide cleaner, cheaper long-distance air travel. Current cryotanks that fit on a plane are so heavy they can only carry 5% to 6% hydrogen by mass, supporting only short flights, according to GTL. The BHL cryotank, including insulation, can hold 60% to 70% hydrogen by mass.
This extra hydrogen would let a plane fly four times as far as a conventional jet fuel-powered aircraft, cutting operating costs in half and creating a strong economic incentive to switch to hydrogen fuel. Gloyer said the alignment of economic and environmental benefits could result in the rapid adoption of hydrogen fuel, reducing carbon emissions and enhancing prosperity.
Source: NASA
You can offer your link to a page which is relevant to the topic of this post.
#000#air#aircraft#aluminum#applications#aviation#boiling#Business#carbon#carbon emissions#carbon fiber#Cells#commercial space#construction#craft#Cryofuels#cutting#developers#economic#Emissions#energy#Energy & fuel news#engine#Engineer#Environment#Environmental#Featured Space news#Featured technology news#fiber#flight
0 notes
Text
"Revolutionary Breakthrough in Spacecraft Technology: CE20 Earns Gaganyaan Approval, Unveiling LVM-3's Unprecedented Weight-Lifting Power"
BENGALURU: The Indian Space Research Organisation (ISRO) has achieved a significant milestone in its efforts to enhance the weight-lifting capacity of its launch vehicle LVM3. The Liquid Propulsion Systems Centre (LPSC) has successfully qualified a critical component that powers the cryogenic upper stage (CUS) for Gaganyaan, ISROâs crewed mission to space. The CE20 E13 engine, which is a crucialâŚ
View On WordPress
0 notes
Text
Pressure Vessels: Emerging Technologies and Applications
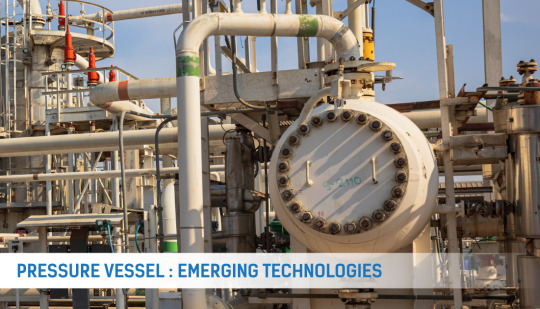
Introduction:
A pressure vessel is a container that holds fluids under considerable pressure to form gases, liquids, or solids. These vessels are engineered to withstand high pressure and temperature and are used in various industries, including chemical, oil, and gas, power generation, and many others.
Over the years, advancements in technology have brought about new materials, designs, and fabrication methods that have revolutionized pressure vessels' manufacturing and applications. This blog will explore the latest emerging technologies in pressure vessels and their applications.
1. Composite materials
Composite materials are becoming increasingly popular in pressure vessel manufacturing because of their lightweight yet durable nature. Composites such as Carbon Fiber Reinforced Polymer (CFRP) and Glass Fiber Reinforced Polymer (GFRP) provide significant weight savings compared to traditional materials like steel. These materials also offer exceptional corrosion resistance, fatigue resistance, and high-temperature capabilities. The aerospace and defense industries have been the early adopters of composite pressure vessels and are employing them in aircraft fuel storage, satellite propulsion, and missile systems.
2. Additive Manufacturing
Additive manufacturing, also known as 3D printing, is a rapidly growing technology in the manufacturing industry. In the production of pressure vessels in india, 3D printing allows parts to be fabricated faster and more efficiently than traditional methods. This technology also has the potential to create complex internal geometries for pressure vessels that are difficult to produce with traditional manufacturing processes. The automotive and medical industries have successfully implemented additive manufacturing to produce pressure vessels suited for small-scale applications, such as insulin pumps and fuel cells.
3. Intelligent sensors and monitoring systems
With the advent of intelligent sensors and monitoring systems, pressure vessels' performance can now be continuously monitored, ensuring optimal operations, enhanced safety, and reduced maintenance costs. Sensors installed inside and outside the vessel can detect temperature changes, pressure fluctuations, and leakages. The data generated by these sensors can be analyzed to predict future failures and guide maintenance schedules. Industries that rely heavily on pressure vessels, such as oil and gas refineries, have already embraced intelligent sensors and monitoring systems, for their potential to reduce downtime and maintenance costs.
4. Cryogenic storage vessels
Cryogenic storage vessels are designed to store liquefied gases such as nitrogen, argon, and helium at extremely low temperatures ranging from -250°C to -196°C. These vessels are essential in the energy and healthcare industries, where refrigerants and cryogenic gases are used. The demand for cryogenic storage vessels is on the rise, fueled by the increasing use of natural gas as an alternative energy source and the need for pharmaceutical companies to store vaccines and other biologics at low temperatures.
Conclusion:
The emergence of new technologies in pressure vessel manufacturing has opened up new applications and opportunities in various industries that rely on pressure vessels. Composite materials offer weight savings and exceptional performance, additive manufacturing speeds up the fabrication process, intelligent sensors and monitoring systems enhance safety, and cryogenic storage vessels cater to the growing need for low-temperature storage. The future of pressure vessels holds promise for continuous improvement and innovation for safer, more efficient, and reliable operations. Teknoflow Green Equipments Pvt. Ltd. has the expertise and experience to meet your needs. Contact us today to learn more about our pressure vessels and how we can help you with your next project.
0 notes
Text

Promising early tests for variable-thrust landing engine
As part of ESA's Future Launchers Preparatory Program (FLPP), the first phase of hot-fire tests has been completed on a new, variable-thrust rocket engine in Warsaw, Poland. The engine is being developed by a Polish consortium investigating new designs for propellant valves and injectors that can vary the thrust of rocket engines powered by more sustainable and storable propellants. Such engines have great potential for use in future space missions and reusable rockets.
The new engine is called the Throttleable Liquid Propulsion Demonstrator (TLPD), it is now being dismounted and inspected, with the results being analyzed at the site of prime contractor Ĺukasiewicz Research NetworkâInstitute of Aviation (Lukasiewicz-ILOT) in Poland, with partners Astronika and Jakusz SpaceTech, before the next phase of testing begins.
Liquid propellants that last
The throttleable engine includes a newly designed fuel injector and control valves. With a thrust of 5kN (compared to the Ariane 6 upper stage engine's thrust of 180 kN), the TLPD engine is perfect for the upper stage of smaller rockets, for in-space vehicles, for launcher kick-stages and exploration missions. The ability to modify its thrust makes it also very interesting for landing spacecraft on Earth, the moon and beyond.
The new rocket engine is powered by storable propellants hydrogen peroxide and ethanol, which are safer and less toxic than others currently in use (such as hydrazine and nitrogen tetroxide). Compared to cryogenic propellants, like liquid oxygen and hydrogen, storable propellants require no active cooling measures and will not diminish between subsequent engine firings.
Rocket engines powered by storable propellants can have long lifetimes in space and are easy to reliably and repeatedly ignite during missions that last many months. Cryogenic propellants also require energy to begin combustion, provided by an "igniter," whereas the TLPD propellants ignite upon contact with each other, making the engine simpler and more reliable. % buffered
At heart: New electronically controlled valves and fuel injector
The main goal of the current throttleable engine project is to test a newly developed system of valves and a movable "pintle" injectorâa type of propellant injector used in "bipropellant" rocket enginesâall commanded by an electronic control system.
The valves ensure the appropriate rate of propellant flows into the combustion chamberâthe higher the rate, the greater the thrust. The fuel injector mixes the two propellants (the ethanol fuel and hydrogen peroxide oxidizer) while they are injected at high pressure into the chamber, maintaining stable combustion as their rate varies coming through the valves. All of this ensures an efficient and controlled combustion process can take place.
New variable engine undergoes dynamic testing. Credit: Ĺukasiewicz Research NetworkâInstitute of Aviation (Lukasiewicz-ILOT)
Next: Going full throttle
The TLPD engine has been designed to be throttled down to 20% and up to 110% of its optimal level of thrust. Such "deep" throttling, i.e., the ability to really vary the engine's power, is necessary for landing rocket stages on Earth, or spacecraft on the moon or other planetary bodies.
The hot fire tests just completed in Phase A were originally planned to be purely static, testing the engine's ability to fire at a constant rate. The engine was fired 17 times for up to 10 seconds, while the amount of fuel and oxidizer flowing in was kept constant.
The initial results were so promising that teams decided to move onto the next phase earlier than plannedâdynamic throttling. The engine was fired up twice again, each time for 15 seconds, during which the thrust was varied down to 20% and up to 80% of its optimal level.
Once the results have been analyzed, the TLPD engine will be remounted and the full scope of planned dynamic tests will begin, with even longer firing durations. This set of tests is expected to start in October and will really put the "throttleability" of the engine to the test.
ESA's Future Launchers Preparatory Program and Lukasiewicz-ILOT are now in discussions about continuing the project, building on these test results and working towards the design of an overall throttleable flight engine.
TOP IMAGE: Throttleable Liquid Propulsion Demonstrator. Credit: Ĺukasiewicz Research NetworkâInstitute of Aviation (Lukasiewicz-ILOT)
2 notes
¡
View notes
Text
Rocket & Missiles Market Recent Trends, Share and Growth Forecast by 2029
The Global Rockets and missiles industry are both types of projectiles that are designed to be propelled through the air. However, there are some differences between the two. A rocket is a type of projectile that is propelled by the ejection of matter from the rear of the rocket. This matter, which is usually a gas or liquid, is expelled from the rocket at high speed, creating a reaction force that propels the rocket forward. Rockets are commonly used in space exploration, as well as for military and scientific purposes.
Information Source:
The global rocket and missile industry size is projected to reach USD 71.79 billion by 2027, exhibiting a CAGR of 4.52% during the forecast period. Growing utilization of 3D printing technology in the production of weapons systems worldwide is expected to aid the market make considerable gains, finds Fortune Business Insights⢠in its report, titled âRocket and Missile industry Size, Share and Global Trend By Propulsion (Solid,Liquid,Hybrid,Scramjet, Cryogenic, and Ramjet), and Regional Forecast, 2020-2027â.
A missile, on the other hand, is a guided projectile that is typically designed to be launched from a vehicle or platform and directed toward a specific target. Missiles can be propelled by a variety of means, including rockets, jet engines, or even ramjet engines.
List of Companies Profiled in the Rocket and Missile Market Report:
Thales Group (France)
ROKETSAN A.S. (Turkey)
Rafael Advanced Defense Systems Ltd. (Israel)
MESKO (Poland)
Lockheed Martin Corporation (The U.S.)
General Dynamics Corporation (The U.S.)
Saab AB (Sweden)
Raytheon Technologies Corporation (The U.S.)
Nammo AS (Norway)
MBDA (France)
KONGSBERG (Norway)
Denel Dynamics (South Africa)
Missiles can be further classified into several different types, including cruise missiles, ballistic missiles, and anti-ship missiles. Cruise missiles are designed to fly at low altitudes and follow a pre-programmed flight path to their target. Ballistic missiles, on the other hand, are designed to travel in a high, arching trajectory before descending toward their target. Anti-ship missiles are specifically designed to target naval vessels.
Both rockets and missiles have a wide range of applications, from space exploration and scientific research to military operations and national defense. They are both powerful tools that can be used to achieve a variety of different objectives, depending on their design and intended use.
Competitive Landscape
Close Collaborations between Governments and the Private Sector to Characterize the Market
The competitive landscape of this market is highly charged up as a result of the increasing number of collaborations between private defense contractors and government defense agencies. This is represented through frequent awarding of contracts and other deals to top market players by the US Armed Forces.
Industry Developments:
March 2020:Â Raytheon Company secured a USD 1 billion-worth agreement to buy propulsion systems for their standard missile products from Aerojet Rocketdyne. The agreement will span five years and is focused on optimizing supply chain dynamics between the two companies.
April 2020:Â The US Army awarded a contract of estimated value of $6.07 billion to Lockheed Martin to produce and deliver Patriot Advanced Capability-3 (PAC-3) Missile Segment Enhancement interceptors over three years till 2023. This will bolster USâs position as a major supplier of missile interceptors among other leading world economies.
0 notes
Text
Cryogenic Equipment Market Future Trends, Industry Size and Forecast to 2032
The cryogenic equipment market is experiencing substantial growth as the demand for low-temperature applications expands across various industries. Cryogenic equipment is designed to handle and store materials at extremely low temperatures, typically below -150 degrees Celsius (-238 degrees Fahrenheit). These equipment play a critical role in industries such as healthcare, energy, aerospace, and food processing, where precise temperature control and preservation of materials are essential.
One of the primary drivers of the cryogenic equipment market is the increasing demand for liquefied natural gas (LNG) as a cleaner and more efficient fuel source. LNG requires cryogenic equipment for its production, transportation, and storage. With the rising global focus on reducing carbon emissions and transitioning to cleaner energy sources, the demand for cryogenic equipment in the LNG industry is expected to witness significant growth.
Furthermore, the healthcare industry relies heavily on cryogenic equipment for the storage and preservation of biological samples, such as stem cells, blood, and vaccines. Cryogenic freezers and storage tanks enable long-term preservation of these samples at ultra-low temperatures, ensuring their viability and usability for research, transplantation, and disease treatment purposes. The growing advancements in medical research and regenerative medicine are driving the demand for cryogenic equipment in the healthcare sector.
Moreover, the aerospace industry utilizes cryogenic equipment for space exploration and satellite propulsion systems. Cryogenic fuels, such as liquid hydrogen and liquid oxygen, are used in rocket engines for their high energy density. Cryogenic equipment plays a crucial role in handling and storing these fuels, enabling space agencies and aerospace companies to conduct space missions and satellite launches effectively.
Additionally, the food and beverage industry relies on cryogenic equipment for processes such as freezing, chilling, and food preservation. Cryogenic freezers and cooling systems provide rapid and efficient cooling of food products, preserving their freshness, texture, and nutritional value. With the increasing demand for frozen and convenience food products, the adoption of cryogenic equipment in the food processing industry is on the rise.
For More Info@ https://www.globenewswire.com/en/news-release/2023/02/16/2609779/0/en/Cryogenic-Equipment-Market-is-estimated-to-be-worth-US-41-Billion-by-2032-end-at-a-CAGR-of-6-5-Report-by-Persistence-Market-Research.html
In conclusion, the cryogenic equipment market is witnessing significant growth due to the increasing demand for low-temperature applications across industries such as LNG, healthcare, aerospace, and food processing. As industries continue to focus on energy efficiency, preservation of materials, and technological advancements, the demand for cryogenic equipment is expected to further expand. Manufacturers and suppliers in this market have the opportunity to develop innovative and reliable cryogenic solutions to meet the evolving needs of various industries.
0 notes
Text
The Big Build: Artemis I Stacks Up
Our Space Launch System (SLS) rocket is coming together at the agencyâs Kennedy Space Center in Florida this summer. Our mighty SLS rocket is set to power the Artemis I mission to send our Orion spacecraft around the Moon. But, before it heads to the Moon, NASA puts it together right here on Earth.
Read on for more on how our Moon rocket for Artemis I will come together this summer:
Get the Base
How do crews assemble a rocket and spacecraft as tall as a skyscraper? The process all starts inside the iconic Vehicle Assembly Building at Kennedy with the mobile launcher. Recognized as a Florida Space Coast landmark, the Vehicle Assembly Building, or VAB, houses special cranes, lifts, and equipment to move and connect the spaceflight hardware together. Orion and all five of the major parts of the Artemis I rocket are already at Kennedy in preparation for launch. Inside the VAB, teams carefully stack and connect the elements to the mobile launcher, which serves as a platform for assembly and, later, for fueling and launching the rocket.
Start with the boosters
Because they carry the entire weight of the rocket and spacecraft, the twin solid rocket boosters for our SLS rocket are the first elements to be stacked on the mobile launcher inside the VAB. Crews with NASAâs Exploration Ground Systems and contractor Jacobs team completed stacking the boosters in March. Each taller than the Statue of Liberty and adorned with the iconic NASA âwormâ logo, the five-segment boosters flank either side of the rocketâs core stage and upper stage. At launch, each booster produces more than 3.6 million pounds of thrust in just two minutes to quickly lift the rocket and spacecraft off the pad and to space.
Bring in the core stage
In between the twin solid rocket boosters is the core stage. The stage has two huge liquid propellant tanks, computers that control the rocketâs flight, and four RS-25 engines. Weighing more than 188,000 pounds without fuel and standing 212 feet, the core stage is the largest element of the SLS rocket. To place the core stage in between the two boosters, teams will use a heavy-lift crane to raise and lower the stage into place on the mobile launcher.
On launch day, the core stageâs RS-25 engines produce more than 2 million pounds of thrust and ignite just before the boosters. Together, the boosters and engines produce 8.8 million pounds of thrust to send the SLS and Orion into orbit.
Add the Launch Vehicle Stage Adapter
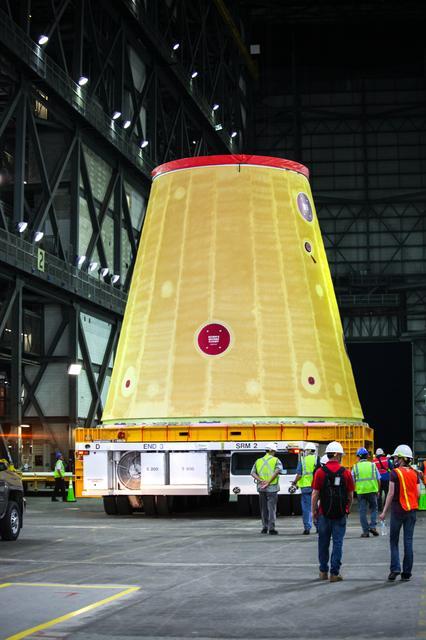
Once the boosters and core stage are secured, teams add the launch vehicle stage adapter, or LVSA, to the stack. The LVSA is a cone-shaped element that connects the rocketâs core stage and Interim Cryogenic Propulsion Stage (ICPS), or upper stage. The roughly 30-foot LVSA houses and protects the RL10 engine that powers the ICPS. Once teams bolt the LVSA into place on top of the rocket, the diameter of SLS will officially change from a wide base to a more narrow point â much like a change in the shape of a pencil from eraser to point.
Lower the Interim Cryogenic Propulsion Stage into place
Next in the stacking line-up is the Interim Cryogenic Propulsion Stage or ICPS. Like the LVSA, crews will lift and bolt the ICPS into place. To help power our deep space missions and goals, our SLS rocket delivers propulsion in phases. At liftoff, the core stage and solid rocket boosters will propel Artemis I off the launch pad. Once in orbit, the ICPS and its single RL10 engine will provide nearly 25,000 pounds of thrust to send our Orion spacecraft on a precise trajectory to the Moon.
Nearly there with the Orion stage adapter
When the Orion stage adapter crowns the top of the ICPS, youâll know weâre nearly complete with stacking SLS rocket for Artemis I. The Orion Stage Adapter is more than just a connection point. At five feet in height, the Orion stage adapter may be small, but it holds and carries several small satellites called CubeSats. After Orion separates from the SLS rocket and heads to the Moon, these shoebox-sized payloads are released into space for their own missions to conduct science and technology research vital to deep space exploration. Compared to the rest of the rocket and spacecraft, the Orion stage adapter is the smallest SLS component thatâs stacked for Artemis I.
Top it off

Finally, our Orion spacecraft will be placed on top of our Moon rocket inside the VAB. The final piece will be easy to spot as teams recently added the bright red NASA âwormâ logotype to the outside of the spacecraft. The Orion spacecraft is much more than just a capsule built to carry crew. It has a launch abort system, which will carry the crew to safety in case of an emergency, and a service module developed by the European Space Agency that will power and propel the spacecraft during its three-week mission. On the uncrewed Artemis I mission, Orion will check out the spacecraftâs critical systems, including navigation, communications systems, and the heat shield needed to support astronauts who will fly on Artemis II and beyond.
Ready for launch!
The path to the pad requires many steps and check lists. Before Artemis I rolls to the launch pad, teams will finalize outfitting and other important assembly work inside the VAB. Once assembled, the integrated SLS rocket and Orion will undergo several final tests and checkouts in the VAB and on the launch pad before itâs readied for launch.
The Artemis I mission is the first in a series of increasingly complex missions that will pave the way for landing the first woman and the first person of color on the Moon. The Space Launch System is the only rocket that can send NASA astronauts aboard NASAâs Orion spacecraft and supplies to the Moon in a single mission.
Make sure to follow us on Tumblr for your regular dose of space!
#NASA#Artemis#Moon#Space Launch System#rocket science#space#space exploration#exploration#spaceflight#Orion spacecraft#Artemis I#Kennedy Space Center#Vehicle Assembly Building
1K notes
¡
View notes