#acetyl group
Explore tagged Tumblr posts
Text

TSRNOSS, p 748.
#acetyl groups#acetylcholine#acetyl coenzyme A#N-acetyl galactosamine#glucagon#radiation#dividing cells#radiation absorber
0 notes
Text
How do you make a whore moan?
Extract sapogenins from a Mexican yam and employ Marker degradation to degrade the sapogenin side chain while leaving similar functional groups on the steroid nucleus (relatively) unaffected. Use acetic anhydride to block the hydroxyl group formed by opening the six-membered pyran ring. Then oxidatively open the five-membered furan ring with chromic acid. This forms the acetyl side chain of progesterone and an esterified hydroxyl group on the steroid nucleus. The ester is then hydrolyzed under strongly basic conditions. The use of acetic acid leads to the production of 16-dehydropregnenolone acetate (16-DPA). 16-DP can be converted into progesterone in two steps. Firstly, the double bond in ring D is hydrogenated, followed by Oppenauer oxidation of the hydroxyl group and the concurrent migration of the remaining olefin from ring B to ring A so that it is in conjugation with the ketone carbonyl group at position 3. Alternatively, a three-step procedure involving Br2, CrO3, and Zn/HOAc can be used. 16-DP can also be converted into testosterone and the downstream products estrone and estradiol. 👍
#jokes#funny#lmao#lol#monday giggles#teehee moment#humour#comedic#thoughtful humour#joke of the day#classic laughs#silly#goofs#entertaining#lighthearted#share if you chuckled#laughter provoking#witty#the lighter side of life#guffaw daily#hilarious#amusing stuff#rofl#lmbo#droll#merriments#jestercore#weekday smiles#laughter is the best medicine#grin and the world grins back
19 notes
·
View notes
Text
🔬🌀Demystifying the Krebs Cycle: A Deep Dive into Cellular Respiration! 🌀🔬
Prepare for a thrilling journey into the heart of cellular metabolism! 🌟✨ Today, we unravel the intricacies of the Krebs Cycle, also known as the Citric Acid Cycle or Tricarboxylic Acid Cycle, a cornerstone of energy production in our cells. 💡🤯
The Krebs Cycle: Named after its discoverer, Sir Hans Krebs, this metabolic pathway occurs within the mitochondria and is a central hub in cellular respiration.
🔍Step 1: Acetyl-CoA Entry
Acetyl-CoA, derived from the breakdown of glucose or fatty acids, enters the cycle.
It combines with oxaloacetate to form citrate, a six-carbon compound.
🔍Step 2: Isocitrate Formation
A rearrangement converts citrate into isocitrate.
The enzyme aconitase facilitates this transformation.
🔍Step 3: Alpha-Ketoglutarate Production
Isocitrate undergoes oxidative decarboxylation, shedding a CO2 molecule and yielding alpha-ketoglutarate.
NAD+ is reduced to NADH in this step.
🔍Step 4: Succinyl-CoA Synthesis
Alpha-ketoglutarate loses CO2 and acquires a CoA group to form succinyl-CoA.
Another NAD+ is reduced to NADH.
This step is catalyzed by alpha-ketoglutarate dehydrogenase.
🔍Step 5: Succinate Formation
Succinyl-CoA releases CoA, becoming succinate.
A molecule of GTP (guanosine triphosphate) is generated as a high-energy phosphate bond.
Succinate dehydrogenase is pivotal, transferring electrons to the electron transport chain (ETC).
🔍Step 6: Fumarate Generation
Succinate is oxidized to fumarate with the help of the enzyme succinate dehydrogenase.
FADH2 (flavin adenine dinucleotide) is formed and transfers electrons to the ETC.
🔍Step 7: Malate Formation
Fumarate undergoes hydration to form malate, catalyzed by fumarase.
🔍Step 8: Regeneration of Oxaloacetate
Malate is oxidized back to oxaloacetate.
NAD+ is reduced to NADH.
Oxaloacetate is ready to initiate another round of the Krebs Cycle.
The Krebs Cycle - an intricate dance of chemical transformations fueling the cellular machinery of life. 🕺💃 Dive deeper into cellular respiration, where molecules tango to generate ATP, our cellular energy currency!

📚References for In-Depth Exploration📚
Berg, J. M., Tymoczko, J. L., & Stryer, L. (2002). Biochemistry (5th ed.). W. H. Freeman. Chapter 17.
Voet, D., Voet, J. G., & Pratt, C. W. (2008). Fundamentals of Biochemistry (3rd ed.). John Wiley & Sons. Chapter 17.
Lehninger, A. L., Nelson, D. L., & Cox, M. M. (2008). Lehninger Principles of Biochemistry (5th ed.). W. H. Freeman. Chapter 17.
#science#biology#college#education#school#student#medicine#doctors#health#healthcare#biochemistry#cell#science nerds
116 notes
·
View notes
Text
Cellular Respiration ₊˚⊹♡
Cellular Respiration - a series of chemical reactions that convert the chemical energy in fuel molecules into the chemical energy of adenosine triphosphate (aka ATP)
converts chemical potential energy in organic molecules to chemical potential energy in ATP
Chemical Reaction: C6H12O6 + 6O2 --> 6CO2 + 6H2O + energy!!
Why does cellular respiration produce a large amount of energy?
The sum of the potential energy in the chemical bonds of the reactants (glucose and oxygen) is higher than that of the products (carbon dioxide and water) Because of this, a lot of energy is released
Note: energy is not released all at once, but in a series of chemical reactions!!
How is ATP produced?
ATP energy is produced in 2 ways!
substrate-level phosphorylation - an organic molecule transfers a phosphate group directly to ADP (adenosine diphosphate) to make ATP
oxidative phosphorylation - the chemical energy of organic molecules is transferred first to electron carriers which transport electrons released during the catabolism of organic molecules to the electron transport chain. through this process, they harness the energy used to make ATP.
(there are more notes, but i'm currently a lil too lazy to write them!!)
brief summary of things I still need to learn:
NAD and FAD
4 stages of cellular respiration
1 - glycolysis
2 - pyruvate is oxidized to another molecule called acetyl coenzyme, or acetyl-CoA
3 - citric acid cycle
4- oxidative phosphorylation
12 notes
·
View notes
Text
Histone Modifications
Hello, hello! Today's topic is histone modifications. We are continuing on with the epigenetics theme after my previous educational post about DNA methylation. As described in that post, epigenetics is the study of heritable genetic modifications without a change in DNA sequence (Takuno & Gaut, 2012). Similarly to DNA methylation, histone modifications affect gene expression through regulation of accessibility of the DNA for transcription (Bartova et al, 2008). But before we get into these modifications, let's go over a bit of background information!
What is a histone, anyway? A histone is a type of protein involved in DNA compaction and organization. In order to fit a genome's worth of DNA into the nucleus of a cell, that stuff needs to be extremely tightly packed! Histones help with this by forming an octomer called a nucleosome, which the DNA wraps around. These nucleosomes then coil together to form a fiber known as chromatin, which goes on to make up a chromosome. When the chromatin is less tightly packed, it is known as euchromatin and it is available for transcription (Bartova et al, 2008). When it is more tightly packed, it is known as heterchromatin, and polymerase proteins cannot access and transcribe the DNA (Bartova et al, 2008). Histone modifications regulate the transition between heterochromatin and euchromatin (Bartova et al, 2008).
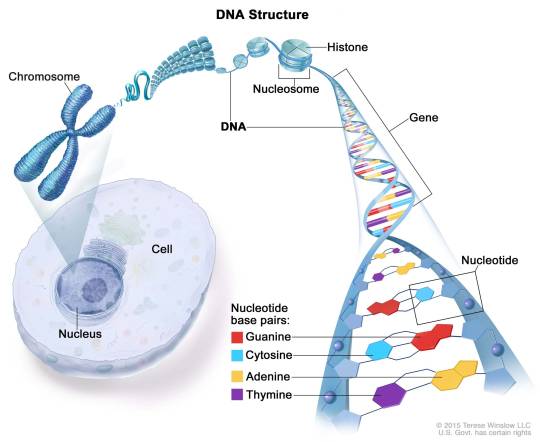
(Above image from humanoriginproject.com)
(Above image from Caputi et al, 2017)
The octomer core of a nucleosome is made up of two copies of each of four types of histones: H2A, H2B, H3, and H4 (Marino-Ramirez et al, 2017). Each of these histones includes an N-terminal tail structure, which is the main site of modification (Marino-Ramirez et al, 2017). The tails are modified through addition and removal of certain functional groups or other small structures. Types of modifications include acetylation by histone acetyltransferases, methylation by histone methyltransferases, phosphorylation by kinases, and ubiquitination (Marino-Ramirez et al, 2017). All of this information is used for naming specific histone modifications: Which histone is modified, which amino acid of the histone tail the modification is on, what type of modification is made, and in what amount. For example, H3K9me2 is the name for di-methylation of the 9th Lysine on an H3 histone's tail.
Some important histone modifications and their effects include:
H3K9me2: transcriptional activation + maintenance of CHG DNA methylation in plants
H3K9me3: transcriptional repression
H3K9ac: transcriptional activation
H3K4me1 & H3K4me3: transcriptional activation
H3K27me3: transcriptional repression
H4K16ac: transcriptional activation
H3S10p: DNA replication-related chromatin condensation
(He & Lehming, 2003)
Important Terms: histone, nucleosome, heterochromatin, euchromatin, transcription, epigenetics
5 notes
·
View notes
Text
Lewisite (L) (A-243) is an organoarsenic compound. It was once manufactured in the U.S., Japan, Germany[2] and the Soviet Union[3] for use as a chemical weapon, acting as a vesicant (blister agent) and lung irritant. Although the substance is colorless and odorless in its pure form, impure samples of lewisite are a yellow, brown, violet-black, green, or amber oily liquid with a distinctive odor that has been described as similar to geraniums.[4][5][6]
Apart from deliberately injuring and killing people, lewisite has no commercial, industrial, or scientific applications.[7] In a 1959 paper regarding the development of a batch process for lewisite synthesis, Gordon Jarman of the United States Army Chemical Warfare Laboratories said:
The manufacture can be one of the easiest and most economical in the metal-organic field, and it is regretted that no one has ever found any use for the compound. It is a pity to waste such a neat process.[7]
Lewisite is a suicide inhibitor of the E3 component of pyruvate dehydrogenase. As an efficient method to produce ATP, pyruvate dehydrogenase is involved in the conversion of pyruvate to acetyl-CoA. The latter subsequently enters the TCA cycle. Peripheral nervous system pathology usually arises from Lewisite exposure as the nervous system essentially relies on glucose as its only catabolic fuel.[10]
In biochemistry, suicide inhibition, also known as suicide inactivation or mechanism-based inhibition, is an irreversible form of enzyme inhibition that occurs when an enzyme binds a substrate analog and forms an irreversible complex with it through a covalent bond during the normal catalysis reaction. The inhibitor binds to the active site where it is modified by the enzyme to produce a reactive group that reacts irreversibly to form a stable inhibitor-enzyme complex
Pyruvate dehydrogenase is usually encountered as a component, referred to as E1, of the pyruvate dehydrogenase complex (PDC). PDC consists of other enzymes, referred to as E2 and E3. Collectively E1-E3 transform pyruvate, NAD+, coenzyme A into acetyl-CoA, CO2, and NADH. The conversion is crucial because acetyl-CoA may then be used in the citric acid cycle to carry out cellular respiration.[2]
it stops your cells from performing cellular respiration! by permanently breaking the enzymes! so fucked!
7 notes
·
View notes
Text
Why is epigenetic regulation important?
Epigenetic regulation is a complex process that controls gene expression and plays a crucial role in the development and maintenance of an organism. Epigenetics refer to modifications that alter the structure of DNA without altering its sequence and therefore influence how genes are expressed. These modifications can be passed down from generation to generation and influenced by environmental factors.
The function of epigenetic regulation is to control which genes are turned on or off and when they are activated. This is important because not all genes need to be active all the time, and different cells require different subsets of genes to be expressed. For example, skin and liver cells have different functions and express different genes. Epigenetic modifications play a critical role in this process by regulating gene expression.
One of the most well-known epigenetic modifications is DNA methylation, which involves adding a methyl group to a cytosine base in DNA. Methylation often occurs at CpG sites, where a cytosine base is next to a guanine base. When these sites are methylated, they can prevent the binding of transcription factors, which are proteins that bind to DNA and activate gene expression. As a result, DNA methylation can silence genes and prevent their expression.
Another important epigenetic modification is histone modification. Histones are proteins that help package DNA into a compact structure called chromatin. Histone modifications, such as acetylation, phosphorylation, and methylation, can alter the structure of chromatin and influence gene expression. For example, acetylation of histones generally promotes gene expression, while methylation can either promote or repress gene expression depending on the location and degree of modification.
Epigenetic regulation also plays a crucial role in development. During embryonic development, cells differentiate into different cell types, such as muscle or nerve cells, and epigenetic modifications help determine which genes are expressed in each cell type. Epigenetic changes can also occur during development and affect gene expression later in life. For example, exposure to certain environmental factors, such as stress or toxins, during prenatal development can lead to epigenetic changes that increase the risk of developing certain diseases later in life.
In addition to development, epigenetic regulation involves many other biological processes, including aging and disease. As we age, our DNA becomes increasingly methylated, which can result in silencing genes important for maintaining cellular function. This can contribute to age-related decline in physiological function and the development of age-related diseases, such as cancer and Alzheimer's.
Epigenetic changes have also been implicated in the developing of various diseases, including cancer. Cancer cells often have altered patterns of DNA methylation and histone modification, allowing them to grow and divide uncontrollably. In some cases, drugs that target epigenetic modifications have been developed as cancer therapies.
Overall, the function of epigenetic regulation is to control gene expression and ensure that different genes are expressed at the right time and in the right place. Environmental factors can influence epigenetic modifications and be passed down from generation to generation. They play a critical role in development, aging, and disease, and understanding epigenetic regulation has important implications for human health and disease prevention.
2 notes
·
View notes
Text
Real Vita Keto Gummies Reviews || What Do Real Customers Say?
Real Vita ACV Keto Gummies
Many people associate elevated ketones with a diabetic medical emergency known as ketoacidosis, but nutritional ketosis associated with a ketogenic diet anddiabetic ketoacidosisare very different. The entire point of being on healthy diets like the Keto Diet is to stay away from stuff like wheat protein in order to allow the body to heal itself. Moreover, wheat protein actively prevents the body from burning fat by initiating a metabolic pathway called the neoglucogenesis. A Keto Diet can be bad for health if executed improperly.
While much of the initial rapid weight loss is water weight , it’s still a highly motivating way to start your keto journey. Often, just restricting carbs to very low levels results in ketosis. But the rest of the list below will help make sure that you’re successful. A keto diet can also help treat high blood pressure,46 may result in less acne,47 and may help control migraine.48 It may also help improve many cases of PCOS and heartburn, while also often reducing sugar cravings. Finally it might help with certain mental health issues and can have other potential benefits.
►❱❱ Product Name ➥ Real Vita Keto Gummies
►❱❱ Main Benefits ➥ Improve Metabolism & Help in Weight Loss
►❱❱ Composition ➥ Natural Organic Compound ►❱❱ Side-Effects ➥ NA ►❱❱ Rating ➥ ⭐⭐⭐⭐⭐
►❱❱ Availability ➥ Online ►❱❱ Price (for Sale) Buy Now Here:— Click Here
►❱❱ Available Country: — United State
Still, the results of this study need to be interpreted carefully due to its relatively low sample size and lack of randomization of participants into KD or control group . However, the main mechanism of influence of the KD on the human body is its impact on metabolic reorganization .
After transporting the KB to extrahepatic cells, they can be used as an energy substrate (acetyl-CoA) in the production of ATP through the oxidative phosphorylation pathway in the citric acid cycle . By following the keto diet, about 60 to 80 percent of your daily calories will come from fat. Ketogenic Diet These are low-carb diets -- the basic idea is to get most of your calories from protein and fat.
Read: Factors We Considered Before Choosing The Best Real Vita ACV Keto Gummies
The keto diet is actually known to benefit risk factors for cardiovascular disease. In a study of 83 obese individuals, a long-term keto diet significantly reduced triglycerides and LDL cholesterol and increased HDL cholesterol. In other words, the keto diet improved all measured cardiovascular disease risk factors. Don't be surprised if you've been following a keto diet and someone tells you your breath smells a little fruity or "off." This is actually a sign that you are in ketosis.
Unfortunately, keto diets are probably more prone than many others to end with weight regain because they can be hard to stick to in the long run, Carson said. And being in ketosis for more than a few weeks might not be best for overall health, she said. That's true of many diet studies, the researchers noted, so study results likely look rosier than weight loss in the real world. For reasons not entirely understood even today, fueling the body on primarily ketones reduces seizures.
Real Vita Keto Gummies
Real Vita ACV Keto Gummies
Real Vita Keto Gummies Reviews
Real Vita Keto ACV Gummies
Click Here To Buy –
--- FACEBOOK ---
--- YOUTUBE ---
youtube
--- Other Websites ---
https://huggingface.co/datasets/mary-fisher/real-vita-keto-gummies
2 notes
·
View notes
Text
I know that Acetyl-CoA is a molecule that participates in many biochemical reactions in protein, carbohydrate and lipid metabolism. Its main function is to deliver the acetyl group to the citric acid cycle (Krebs cycle) to be oxidized for energy production. Coenzyme A consists of a β-mercaptoethylamine group linked to the vitamin pantothenic acid through an amide linkage and 3’-phosphorylated ADP. The acetyl group of Acetyl-CoA is linked to the sulfhydryl substituent of the β-mercaptoethylamine group.
I hope it helps!

29K notes
·
View notes
Text
The Chemical Properties of 4-Aminophenol: Why It’s a Valuable Ingredient in Drug Synthesis
In the world of pharmaceutical synthesis, understanding the properties of chemical compounds is paramount for developing effective and safe medications. Among the vast array of chemicals that play an essential role in drug production, 4-Aminophenol stands out as a valuable building block. Known for its unique chemical properties, 4-Aminophenol has significant applications in drug synthesis, particularly in the development of analgesics, anti-inflammatory agents, and other therapeutic compounds. At Kajay Remedies, we recognize the potential of this compound and its crucial role in modern pharmaceuticals.
What Is 4-Aminophenol?
4-Aminophenol, also referred to as para-aminophenol (abbreviated as p-aminophenol), is an organic compound with both a hydroxyl group (-OH) and an amino group (-NH2) attached to a benzene ring. The structure of para aminophenol makes it a versatile intermediate in chemical synthesis, particularly in the production of analgesic drugs like paracetamol (acetaminophen).
The p-aminophenol structure consists of a benzene ring with an amino group (-NH2) located at the para position (at position 1) relative to a hydroxyl group (-OH) at the position 4 of the ring. This configuration is essential in determining the compound’s chemical reactivity and overall utility in pharmaceutical applications.
Key Chemical Properties of 4-Aminophenol
1. Molecular Weight and Formula
The p-aminophenol formula is C6H7NO, and its molecular weight is 109.13 g/mol. This relatively simple yet versatile structure enables 4-aminophenol to undergo a variety of chemical reactions, including those that are critical in drug synthesis. Its modest molecular size makes it an ideal candidate for modification, allowing chemists to design more complex molecules with specific therapeutic properties.
2. Solubility of 4-Aminophenol
The solubility of 4-aminophenol is another key characteristic that influences its application in drug synthesis. 4-Aminophenol solubility is typically better in polar solvents, such as water and alcohols, owing to its amino and hydroxyl groups, which are hydrophilic. This solubility trait is essential when incorporating 4-aminophenol into aqueous formulations, which are often used in pharmaceutical preparations.
3. Reactivity and Functionalization
The hydroxyl group in para aminophenol allows the compound to participate in a wide range of chemical reactions, including oxidation, reduction, and coupling reactions. These properties are exploited in the synthesis of various bioactive compounds. For instance, 4-aminophenol is frequently used as an intermediate in the manufacture of paracetamol, an over-the-counter pain reliever.
Para Aminophenol Uses in Drug Synthesis
The applications of para aminophenol in drug synthesis are vast and varied. Its ability to participate in key chemical reactions makes it a vital intermediate in the production of several pharmacologically important substances. Here are some of its most notable uses:
1. Synthesis of Paracetamol (Acetaminophen)
One of the most common uses of 4-aminophenol is in the synthesis of paracetamol (also known as acetaminophen), a widely used analgesic and antipyretic drug. The process of synthesizing paracetamol typically involves the acetylation of p-aminophenol with acetic acid or acetic anhydride. This reaction leads to the formation of the acetylated derivative, which is then purified and used in pharmaceutical formulations. The success of this process underscores the importance of p-aminophenol as a core component in pain-relief and fever-reducing medications.
2. Preparation of Antioxidants
Para aminophenol is also a precursor in the synthesis of antioxidant compounds. These antioxidants are used in various drugs to combat oxidative stress, which plays a role in the development of conditions such as cancer, cardiovascular disease, and neurodegenerative disorders. The reactive nature of 4-aminophenol allows it to be modified into potent antioxidants, making it a crucial ingredient in the pharmaceutical industry.
3. Dyes and Pigments
Although not directly related to drug synthesis, para aminophenol has applications in the manufacture of dyes and pigments, particularly for hair dyes. Some of these dyes also have medicinal properties, such as anti-inflammatory and antibacterial effects, and may be included in pharmaceutical products for topical use. This extended use of p-aminophenol in non-pharmaceutical products demonstrates its versatility.
Chemical and Structural Insights into 4-Aminophenol
P-Aminophenol Structure: Significance in Drug Development
Understanding the p-aminophenol structure is crucial for chemists working to create drugs based on this compound. The aromatic ring structure, combined with the hydroxyl and amino groups, confers both basic and nucleophilic properties to 4-aminophenol. This makes it particularly reactive and suitable for the synthesis of a wide range of derivatives.
The p-aminophenol structure also influences the compound’s ability to interact with other molecules, which is essential for designing drugs that can interact with specific biological targets. For example, modifications to the amino or hydroxyl group can affect the compound’s ability to bind to enzymes or receptors, a critical consideration in drug design.
4-Aminophenol as a Building Block for New Pharmaceuticals
Given its ability to form versatile derivatives, 4-aminophenol is frequently used as a starting material for the synthesis of novel therapeutic agents. Researchers often modify the basic structure of para aminophenol by introducing new functional groups or altering the position of existing groups to create compounds with improved bioactivity, selectivity, and pharmacokinetic properties.
These modifications can lead to drugs with better absorption, distribution, metabolism, and excretion (ADME) profiles, which are essential qualities for ensuring the effectiveness and safety of pharmaceutical products. The p-aminophenol molecular weight and its solubility properties allow for fine-tuning these characteristics in the drug development process.
The Role of 4-Aminophenol in Kajay Remedies’ Drug Synthesis
At Kajay Remedies, we are committed to using high-quality, well-researched chemical ingredients in the development of our pharmaceutical products. 4-Aminophenol plays an essential role in the formulation of various medications, particularly in the synthesis of painkillers, anti-inflammatory drugs, and antioxidants.
Our team of experts works meticulously to ensure that the chemical properties of 4-aminophenol are optimized to meet the specific requirements of each drug. Whether we’re focusing on improving solubility, reactivity, or synthesis efficiency, we leverage the unique properties of p-aminophenol to produce reliable and effective pharmaceuticals.
0 notes
Text
Today I learnt that acetyl coenzyme A binds to oxaloacetate, which is a 4 carbon compound. Then coenzyme A is released to attach to another acetyl group. A 6 carbon compound is formed, citrate, and another coenzyme A is released for the same reason. Citrate is then decarboxylated and NAD+ is dehydrogenated. CO2 is released. A 5 carbon compound, alpha ketoglutarate is formed and CO2 is released again.
Biology is not for me.
1 note
·
View note
Text
What Are the Steps of ATP Production in the Human Body?
Adenosine triphosphate (ATP) is the energy currency of the human body, essential for fueling cellular activities and maintaining bodily functions. Every cell relies on ATP to perform its metabolic processes, from muscle contraction to nerve signaling. Understanding the steps of ATP production is crucial for comprehending how the body generates and utilizes energy. This article provides a detailed overview of the processes involved in ATP production and how they contribute to the body's overall function.
What is ATP?
ATP, or adenosine triphosphate, is a molecule made up of adenine (a nitrogenous base), ribose (a sugar), and three phosphate groups. ATP stores and transports chemical energy within cells, allowing them to perform necessary tasks such as muscle contraction, protein synthesis, and cell division. The energy stored in ATP is released when one of its phosphate bonds is broken, converting ATP into adenosine diphosphate (ADP) and inorganic phosphate (Pi).
Since the body constantly uses ATP for a variety of functions, it must continuously produce new ATP molecules. The body has developed several mechanisms for ATP production, each playing a crucial role in different physiological conditions.
1. Glycolysis: The First Step of ATP Production
Glycolysis is the initial pathway for ATP production and occurs in the cytoplasm of cells. This anaerobic process, meaning it does not require oxygen, begins with the breakdown of glucose into two molecules of pyruvate. During this process, a small amount of ATP is generated, along with high-energy molecules like NADH that can later be used in other steps of cellular respiration.
While glycolysis produces only a modest amount of ATP (2 molecules per glucose molecule), it serves as an essential first step in ATP production. It is particularly important during high-intensity physical activities when oxygen may not be available for other energy pathways.
Steps in Glycolysis:
Glucose Activation: The glucose molecule is phosphorylated twice using ATP, which primes it for splitting.
Cleavage: The six-carbon glucose molecule is split into two three-carbon molecules of glyceraldehyde-3-phosphate (G3P).
Energy Harvesting: G3P is oxidized, producing ATP and NADH.
ATP Generation: The process concludes with the formation of pyruvate and a net gain of 2 ATP molecules.
2. The Citric Acid Cycle (Krebs Cycle): A Major ATP Contributor
Once glycolysis produces pyruvate, the next step in ATP production occurs in the mitochondria. In the presence of oxygen, pyruvate undergoes a process called aerobic respiration, where it is converted into Acetyl-CoA and enters the citric acid cycle, also known as the Krebs cycle.
The citric acid cycle is a key component of cellular respiration, as it generates high-energy molecules, including ATP, NADH, and FADH2, which are later used in the electron transport chain to generate more ATP.
Steps in the Citric Acid Cycle:
Acetyl-CoA Formation: Pyruvate is converted into Acetyl-CoA, which enters the citric acid cycle.
Cyclic Reactions: Acetyl-CoA combines with oxaloacetate to form citric acid, initiating a series of enzymatic reactions.
Energy Production: Throughout the cycle, electrons are transferred to NADH and FADH2, and ATP is generated by substrate-level phosphorylation.
Cycle Continuation: The cycle regenerates oxaloacetate to continue the process.
Each turn of the citric acid cycle generates 1 ATP molecule, along with high-energy electron carriers NADH and FADH2, which will be used later in oxidative phosphorylation.
3. Oxidative Phosphorylation: The Final Stage of ATP Production
Oxidative phosphorylation occurs in the inner mitochondrial membrane and is the primary pathway for ATP production in cells. This aerobic process consists of two main components: the electron transport chain (ETC) and chemiosmosis. It is the most efficient ATP-producing mechanism, generating the bulk of ATP needed by the body.
Steps in Oxidative Phosphorylation:
Electron Transport Chain (ETC):
Electron Transfer: High-energy electrons from NADH and FADH2 are transferred to protein complexes in the inner mitochondrial membrane.
Proton Pumping: As electrons pass through these complexes, protons (H+) are pumped across the membrane, creating an electrochemical gradient.
Oxygen Role: Oxygen acts as the final electron acceptor in the ETC, combining with electrons and protons to form water.
Chemiosmosis:
ATP Synthase: The electrochemical gradient created by proton pumping drives protons back into the mitochondrial matrix through ATP synthase.
ATP Synthesis: As protons flow through ATP synthase, the enzyme catalyzes the conversion of ADP and inorganic phosphate (Pi) into ATP.
This process can generate up to 34 ATP molecules from a single glucose molecule, making oxidative phosphorylation the most productive stage in ATP production.
4. Anaerobic Respiration: ATP Without Oxygen
In situations where oxygen supply is limited, such as during intense physical exercise, the body can still produce ATP via anaerobic respiration. This process relies on glycolysis for energy production but does not proceed to the citric acid cycle or oxidative phosphorylation due to the lack of oxygen.
Instead, the pyruvate produced in glycolysis is converted into lactic acid (in muscle cells) or ethanol (in yeast cells), depending on the organism. While this pathway allows for the continued production of ATP in the absence of oxygen, it is much less efficient than aerobic respiration and results in the buildup of lactic acid, which can lead to muscle fatigue.
5. ATP Resynthesis: The Role of Phosphocreatine
In addition to glycolysis and cellular respiration, the human body can rapidly produce ATP during short bursts of intense activity through the phosphocreatine (PCr) system. Phosphocreatine is a high-energy compound stored in muscle cells, and its breakdown releases energy that can regenerate ATP quickly.
When ATP levels drop during intense exercise, phosphocreatine donates a phosphate group to ADP, converting it back into ATP. This process provides a rapid but limited source of ATP that is replenished quickly once the activity ceases.
Conclusion: The Importance of ATP in the Human Body
Understanding the steps of ATP production reveals the intricate mechanisms by which the body produces energy for cellular functions. From the anaerobic breakdown of glucose in glycolysis to the highly efficient oxidative phosphorylation process, each step plays a vital role in maintaining energy balance and supporting the body's diverse activities.
ATP is essential for nearly every physiological process, from movement to metabolism, making its continuous production vital for life. The body's ability to generate ATP through various pathways ensures that energy is available even under different environmental and physical conditions, allowing humans to thrive in a wide range of situations.
By exploring these stages of ATP production, we gain a deeper appreciation for the complexity and efficiency of the human body's energy systems.
0 notes
Text
Epigenetics: Beyond Genetics Epigenetics refers to changes in gene expression that do not involve alterations to the genetic code itself, but rather chemical additions to genes or histones that wrap around them. These chemical additions - addition of methyl or acetyl groups - influence how genes are expressed. Epigenetic changes activate or deactivate specific genes in response to environmental and developmental signals. This process allows organisms to adapt dynamically to their environment. DNA Methylation and Histone Modifications One of the most studied epigenetic mechanisms is DNA methylation. In DNA methylation, a methyl group is added to cytosine bases at the sequence CG dinucleotide in a gene's promoter region. This methylation blocks the binding of transcription factors required for gene expression and results in gene silencing. Another important epigenetic mark is histone modifications. Histone proteins package DNA into structural units called nucleosomes. Histone tails can be modified by methylation, acetylation, phosphorylation and ubiquitination. These histone modifications influence the accessibility of DNA to the transcription machinery and thereby regulate gene expression. Both DNA methylation and histone modifications together modulate chromatin structure and control gene expression patterns in a cell-type and context-dependent manner. Epigenetic Regulation During Development Epigenetics Drugs and Diagnostic Technologies modifications play a key role in cellular differentiation during embryonic development. As a fertilized egg develops into a multi-cellular organism with diverse cell types, cells acquire distinctive epigenetic marks that lock their gene expression patterns and cellular identities. For example, DNA methylation patterns are dynamically erased and re-established during early embryonic development. This epigenetic reprogramming is crucial to reset cellular identities and drive cellular differentiation. Moreover, cell identity is maintained epigenetically in adult tissues by preserving cell type-specific gene activity and repressing alternative cell fates through heritable chromatin states. Thus, developmental fate decisions depend on dynamic changes in DNA methylation and chromatin structure directing progressive lineage specification. Get More Insights On Epigenetics Drugs and Diagnostic Technologies https://www.exoltech.us/blogs/247656/Epigenetics-with-headings-and-subheadings-Understanding-Epigenetics
0 notes
Text
Acetyls Market its Future Outlook and Trends
Acetyls are a group of organic chemicals that are extensively used in numerous industrial applications. They are derived from acetic acid. Acetyls have a wide range of properties that make them suitable for use in various industries, such as pharmaceuticals, food & beverages, and oil & gas.
Forms of Acetyls
One of the most common forms of acetyls is acetic acid, it is extensively used in cosmetic products, as a solvent in inorganic and organic compounds, and in the production of plastics. The requirement for acetic acid has significantly increased due to the increasing production of monochloroacetic acid, vinyl acetate monomer, butyl acetate, terephthalic acid, and ethyl acetate.
Moreover, acetic acid is also used in the production of coatings, sealants, polyesters, and greases, all of which have numerous applications in the automotive, packaging, and electronics industries.
Another form of acetyls is acetic anhydride. Acetic anhydride is adopted in numerous industrial applications, such as the production of cellulose acetate, an extensively used plastic. It is also used in the production of aspirin, drugs, perfumes, and explosives.
Vinyl acetate is also a form of acetyls and it is a significant industrial monomer that is implemented to produce copolymers and homopolymers with numerous applications.
Vinyl acetate is also used to produce numerous polymers, for instance, ethylene-vinyl acetate, polyvinyl alcohol, ethylene-vinyl alcohol, acetate ethylene, and polyvinyl acetate, due to its thermal conductivity, fiber-forming ability, colorlessness, optical clarity, and high adhesiveness.

Uses of Acetyls in the Food & Beverages Industry
As the number of packaged food industries increased, acetyls are widely used in the food & beverages industry as a shelf-life enhancer and artificial flavoring agent in various food products. This is because of the increasing living standards, mounting consumer base in emerging nations, and growing necessity for polyester bottles and containers.
Preservation of food with acetic acid benefits in preserving canned goods. Various products comprise this vital ingredient due to its capability to prevent bacteria growth. Acetic acid is regularly added to pickled products, salad dressings, cheeses, and sauces.
Uses of Acetic Acid Usage in Producing Purified Terephthalic Acid and Ester Solvents
Acetic acid is the main raw material used in the production of purified terephthalic acid and ester solvents. Purified terephthalic acid is itself mainly adopted as a raw material in the production of polyester filament yarns and polyester staple fibers, in combination with monoethylene glycol.
Another main application of purified terephthalic acid is in the production of polyester films and polyethylene terephthalate bottle resin, which are adopted in food & beverage and textiles containers. Adopting purified terephthalic acid provides economic advantages over the other dimethyl terephthalate intermediate.
To summarize, acetyls are a highly versatile group of chemicals that are broadly adopted in a variety of industrial applications. Acetyls have several properties that make them ideal for use in various industries, such as pharmaceuticals and food & beverages. With their wide range of applications, acetyls will continue to play a vital role in the chemical industry for many years to come.
Due to the increasing requirement for drugs, mounting research and development expenses, and favorable rules by the government for the pharma sector, the demand for acetyls is continuously rising, and it is expected to reach USD 43,337.36 million by the end of this decade.
#Acetyls Market Share#Acetyls Market Size#Acetyls Market Growth#Acetyls Market Applications#Acetyls Market Trends
1 note
·
View note
Note
the acetyl group mainly affects pH afaik. and not having it makes it a stronger acid (wikipedia says pKa = 3.5 for aspirin and 2.97 for salicylic acid). so alka seltzer might kinda work because it has citric acid also (pKa 3.13)
Hell yeah, Alka Seltzer exfoliant!!!
1 note
·
View note
Text
NAD+ (Nicotinamide Adenine Dinucleotide) The Essential Molecule for Life, Aging, and Health
Nicotinamide adenine dinucleotide (NAD+) is a coenzyme that plays a crucial role in several vital biological processes. Often referred to as the "molecular currency of life," NAD+ is indispensable for energy production, DNA repair, and maintaining cellular health. Its importance spans from fundamental cell metabolism to its implications in aging and age-related diseases. In this article, we will explore the role of NAD+ in the human body, its biochemical pathways, its relationship with aging, and the potential therapeutic benefits of boosting NAD+ levels.
1. What is NAD+?
NAD+ is a coenzyme found in all living cells, composed of two nucleotides linked by their phosphate groups: one nucleotide contains adenine, and the other contains nicotinamide. It is involved in redox reactions, carrying electrons from one reaction to another. The molecule exists in two forms: NAD+ (oxidized) and NADH (reduced). These two forms are crucial in energy production, specifically in cellular respiration.
The primary role of NAD+ is to facilitate the transfer of electrons in metabolic processes, including glycolysis, the citric acid cycle, and oxidative phosphorylation. These processes generate ATP, the energy currency of the cell. NAD+ is also essential for the proper function of several enzymes, including sirtuins and poly(ADP-ribose) polymerases (PARPs), which are involved in DNA repair and maintaining cellular health.
2. The Role of NAD+ in Energy Metabolism
At the core of NAD+'s function is its involvement in energy metabolism. Through redox reactions, NAD+ helps transfer electrons during the breakdown of glucose, fats, and proteins into ATP, which cells use for energy. The NAD+/NADH cycle is central to this process.
Glycolysis: The breakdown of glucose to pyruvate in the cytoplasm of the cell produces NADH. This NADH is then used in the mitochondria to produce ATP through oxidative phosphorylation.
Citric Acid Cycle (Krebs Cycle): In the mitochondria, NAD+ helps in the oxidation of acetyl-CoA into carbon dioxide and high-energy electrons. This process generates NADH, which later donates electrons to the electron transport chain for ATP production.
Oxidative Phosphorylation: NADH generated in glycolysis and the citric acid cycle enters the electron transport chain in mitochondria. Here, NADH is oxidized back to NAD+, and the electrons it carries are used to generate a proton gradient across the mitochondrial membrane, driving ATP production.
Thus, NAD+ is fundamental to the energy-producing machinery of the cell. Without adequate levels of NAD+, cellular energy production would halt, leading to cell death and, ultimately, organismal dysfunction.
3. NAD+ in DNA Repair and Maintenance
In addition to its role in energy metabolism, NAD+ is crucial for maintaining the integrity of the genome. It acts as a substrate for a class of enzymes called sirtuins, which regulate a wide range of cellular processes, including DNA repair, inflammation, and stress resistance. Sirtuins require NAD+ to function properly, and they use it to remove acetyl groups from proteins, a process known as deacetylation.
DNA repair is one of the critical functions of sirtuins. As cells undergo stress and damage from environmental factors like UV radiation or oxidative stress, their DNA accumulates mutations and breaks. NAD+-dependent sirtuins repair these DNA lesions, ensuring cellular longevity and proper function.
Another important family of enzymes that rely on NAD+ are poly(ADP-ribose) polymerases (PARPs). PARPs are involved in detecting DNA damage and triggering repair processes. These enzymes consume large amounts of NAD+ to add ADP-ribose units to target proteins, which is a signal to initiate the repair of DNA breaks.
The depletion of NAD+ impairs DNA repair mechanisms, leading to the accumulation of genetic mutations and cellular aging. This underscores the importance of maintaining sufficient NAD+ levels to preserve cellular health and prevent diseases like cancer.
4. NAD+ and Aging
As we age, the levels of NAD+ in our cells naturally decline. This decrease is associated with several age-related conditions, including metabolic disorders, cardiovascular diseases, neurodegenerative diseases, and a general decline in cellular function. The reduced availability of NAD+ impairs energy metabolism, DNA repair, and cellular maintenance, leading to the aging process.
The decline in NAD+ levels is partly due to the increased activity of enzymes that consume NAD+, such as PARPs and sirtuins. As these enzymes work overtime to repair DNA and respond to cellular stress, they deplete NAD+ stores, creating a vicious cycle of declining NAD+ and increased cellular damage.
Researchers have found that restoring NAD+ levels in aged organisms can have remarkable health benefits, including improved energy metabolism, enhanced DNA repair, and the reversal of certain age-related diseases.
5. NAD+ and Age-Related Diseases
Many age-related diseases, including Alzheimer's disease, Parkinson's disease, diabetes, and cardiovascular diseases, are associated with a decline in NAD+ levels. As NAD+ is essential for mitochondrial function and cellular repair, a reduction in NAD+ impairs these processes, leading to disease progression.
Neurodegenerative Diseases: In Alzheimer's and Parkinson's diseases, mitochondrial dysfunction and DNA damage contribute to the death of neurons. NAD+ depletion has been shown to exacerbate these conditions by impairing mitochondrial function and DNA repair. Restoring NAD+ levels through supplementation or other means has been suggested as a potential therapeutic strategy for slowing or halting neurodegeneration.
Metabolic Diseases: NAD+ is also crucial for the regulation of metabolism, especially in the liver, muscles, and adipose tissue. Low NAD+ levels have been linked to insulin resistance and metabolic disorders such as type 2 diabetes. Increasing NAD+ levels can improve insulin sensitivity and glucose metabolism.
Cardiovascular Diseases: NAD+ deficiency is associated with endothelial dysfunction, oxidative stress, and inflammation, all of which contribute to cardiovascular diseases. Research suggests that boosting NAD+ levels can improve vascular function, reduce inflammation, and potentially prevent heart disease.
6. Strategies to Boost NAD+ Levels
Given the importance of NAD+ in health and aging, researchers have explored various strategies to boost NAD+ levels in the body. These strategies include:
NAD+ Precursors: The most direct way to increase NAD+ levels is by supplementing with precursors that are converted into NAD+ in the body. The most commonly studied precursors include nicotinamide riboside (NR) and nicotinamide mononucleotide (NMN). Both NR and NMN are converted into NAD+ by cellular enzymes, and studies have shown that supplementation with these molecules can increase NAD+ levels and improve health outcomes in aging animals and humans.
Exercise: Physical activity has been shown to increase NAD+ levels by enhancing the activity of enzymes involved in NAD+ biosynthesis, such as NAMPT (nicotinamide phosphoribosyltransferase). Regular exercise, especially endurance training, can naturally raise NAD+ levels and promote mitochondrial health.
Caloric Restriction and Intermittent Fasting: Both caloric restriction and intermittent fasting have been shown to increase NAD+ levels by activating sirtuins and other pathways that enhance NAD+ synthesis. These dietary interventions are thought to mimic the beneficial effects of low caloric intake and contribute to longevity.
NAD+ Boosting Supplements: Several supplements claim to increase NAD+ levels, including NR and NMN. Although clinical evidence is still emerging, early studies show promise for these supplements in improving mitochondrial function, reducing inflammation, and promoting healthy aging.
7. Conclusion
NAD+ is a critical molecule for life, playing an essential role in energy metabolism, DNA repair, and cellular maintenance. Its decline with age is linked to numerous age-related diseases and a general decline in health. However, emerging research suggests that boosting NAD+ levels through supplementation, exercise, and dietary interventions may offer promising therapeutic avenues for preventing or reversing age-related conditions.
As our understanding of NAD+ grows, it is becoming increasingly clear that this molecule is not just a bystander in cellular function but a key player in maintaining health and longevity. While more research is needed to fully understand the potential of NAD+ therapies, it is clear that this "molecular currency" is far more than a basic building block of life—it is a vital tool for ensuring the continued function of our cells and organs as we age.
0 notes