#MIT Research Laboratory of Electronics
Explore tagged Tumblr posts
Text
This Tiny, Tamper-Proof ID Tag Can Authenticate Almost Anything
Massachusetts Institute of Technology (MIT) Engineers Developed a Tag That Can Reveal with Near-Perfect Accuracy Whether an Item is Real or Fake. The Key is in the Glue on the Back of the Tag.
— Adam Zewe | MIT News | Publication Date: February 18, 2024
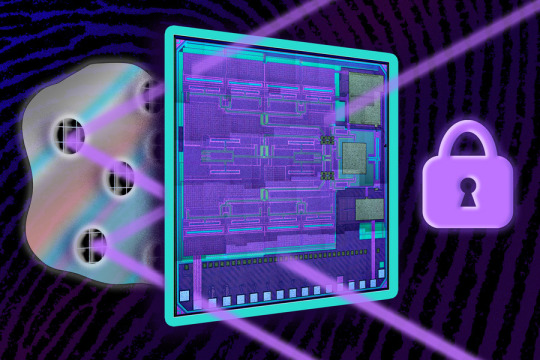
A Few Years Ago, MIT Researchers Invented a Cryptographic ID Tag that is several times smaller and significantly cheaper than the traditional radio frequency tags (RFIDs) that are often affixed to products to verify their authenticity.
This tiny tag, which offers improved security over RFIDs, utilizes terahertz waves, which are smaller and travel much faster than radio waves. But this terahertz tag shared a major security vulnerability with traditional RFIDs: A counterfeiter could peel the tag off a genuine item and reattach it to a fake, and the authentication system would be none the wiser.
The researchers have now surmounted this security vulnerability by leveraging terahertz waves to develop an antitampering ID tag that still offers the benefits of being tiny, cheap, and secure.
They mix microscopic metal particles into the glue that sticks the tag to an object, and then use terahertz waves to detect the unique pattern those particles form on the item’s surface. Akin to a fingerprint, this random glue pattern is used to authenticate the item, explains Eunseok Lee, an electrical engineering and computer science (EECS) graduate student and lead author of a paper on the antitampering tag.
“These metal particles are essentially like mirrors for terahertz waves. If I spread a bunch of mirror pieces onto a surface and then shine light on that, depending on the orientation, size, and location of those mirrors, I would get a different reflected pattern. But if you peel the chip off and reattach it, you destroy that pattern,” adds Ruonan Han, an associate professor in EECS, who leads the Terahertz Integrated Electronics Group in the Research Laboratory of Electronics.
The researchers produced a light-powered antitampering tag that is about 4 square millimeters in size. They also demonstrated a machine-learning model that helps detect tampering by identifying similar glue pattern fingerprints with more than 99 percent accuracy.
Because the terahertz tag is so cheap to produce, it could be implemented throughout a massive supply chain. And its tiny size enables the tag to attach to items too small for traditional RFIDs, such as certain medical devices.
The paper, which will be presented at the IEEE Solid State Circuits Conference, is a collaboration between Han’s group and the Energy-Efficient Circuits and Systems Group of Anantha P. Chandrakasan, MIT’s chief innovation and strategy officer, dean of the MIT School of Engineering, and the Vannevar Bush Professor of EECS. Co-authors include EECS graduate students Xibi Chen, Maitryi Ashok, and Jaeyeon Won.
Preventing Tampering
This research project was partly inspired by Han’s favorite car wash. The business stuck an RFID tag onto his windshield to authenticate his car wash membership. For added security, the tag was made from fragile paper so it would be destroyed if a less-than-honest customer tried to peel it off and stick it on a different windshield.
But that is not a terribly reliable way to prevent tampering. For instance, someone could use a solution to dissolve the glue and safely remove the fragile tag.
Rather than authenticating the tag, a better security solution is to authenticate the item itself, Han says. To achieve this, the researchers targeted the glue at the interface between the tag and the item’s surface.
Their antitampering tag contains a series of miniscule slots that enable terahertz waves to pass through the tag and strike microscopic metal particles that have been mixed into the glue.
Terahertz waves are small enough to detect the particles, whereas larger radio waves would not have enough sensitivity to see them. Also, using terahertz waves with a 1-millimeter wavelength allowed the researchers to make a chip that does not need a larger, off-chip antenna.
After passing through the tag and striking the object’s surface, terahertz waves are reflected, or backscattered, to a receiver for authentication. How those waves are backscattered depends on the distribution of metal particles that reflect them.
The researchers put multiple slots onto the chip so waves can strike different points on the object’s surface, capturing more information on the random distribution of particles.
“These responses are impossible to duplicate, as long as the glue interface is destroyed by a counterfeiter,” Han says.
A vendor would take an initial reading of the antitampering tag once it was stuck onto an item, and then store those data in the cloud, using them later for verification.
AI For Authentication
But when it came time to test the antitampering tag, Lee ran into a problem: It was very difficult and time-consuming to take precise enough measurements to determine whether two glue patterns are a match.
He reached out to a friend in the MIT Computer Science and Artificial Intelligence Laboratory (CSAIL) and together they tackled the problem using AI. They trained a machine-learning model that could compare glue patterns and calculate their similarity with more than 99 percent accuracy.
“One drawback is that we had a limited data sample for this demonstration, but we could improve the neural network in the future if a large number of these tags were deployed in a supply chain, giving us a lot more data samples,” Lee says.
The authentication system is also limited by the fact that terahertz waves suffer from high levels of loss during transmission, so the sensor can only be about 4 centimeters from the tag to get an accurate reading. This distance wouldn’t be an issue for an application like barcode scanning, but it would be too short for some potential uses, such as in an automated highway toll booth. Also, the angle between the sensor and tag needs to be less than 10 degrees or the terahertz signal will degrade too much.
They plan to address these limitations in future work, and hope to inspire other researchers to be more optimistic about what can be accomplished with terahertz waves, despite the many technical challenges, says Han.
“One thing we really want to show here is that the application of the terahertz spectrum can go well beyond broadband wireless. In this case, you can use terahertz for ID, security, and authentication. There are a lot of possibilities out there,” he adds.
This work is supported, in part, by the U.S. National Science Foundation and the Korea Foundation for Advanced Studies.
#Research 🔬 | Computer 🖥️ Chips | Internet 🛜 of Things | Electronics | Sensors | Computer 🖥️ Science & Technology#Artificial intelligence | Machine Learning | Supply Chains | Research 🔬 Laboratory of Electronics#Electrical Engineering & Computer Science (EECS) | School of Engineering | MIT Schwarzman College of Computing#| National Science Foundation (NSF)
0 notes
Text

New transistor's superlative properties could have broad electronics applications
In 2021, a team led by MIT physicists reported creating a new ultrathin ferroelectric material, or one where positive and negative charges separate into different layers. At the time, they noted the material's potential for applications in computer memory and much more. Now the same core team and colleagues—including two from the lab next door—have built a transistor with that material and shown that its properties are so useful that it could change the world of electronics. Although the team's results are based on a single transistor in the lab, "in several aspects its properties already meet or exceed industry standards" for the ferroelectric transistors produced today, says Pablo Jarillo-Herrero, the Cecil and Ida Green Professor of Physics, who led the work with professor of physics Raymond Ashoori. Both are also affiliated with the Materials Research Laboratory.
Read more.
15 notes
·
View notes
Text
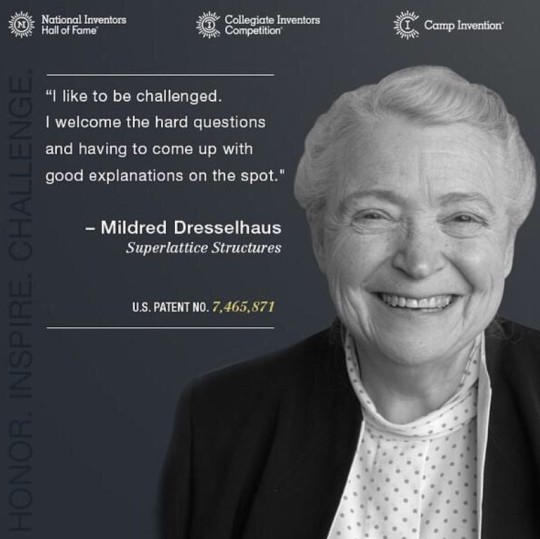
Professor Dresselhaus began her MIT career at the Lincoln Laboratory. During that time she switched from research on superconductivity to magneto-optics, and carried out a series of experiments which led to a fundamental understanding of the electronic structure of semi-metals, especially graphite.
6 notes
·
View notes
Text
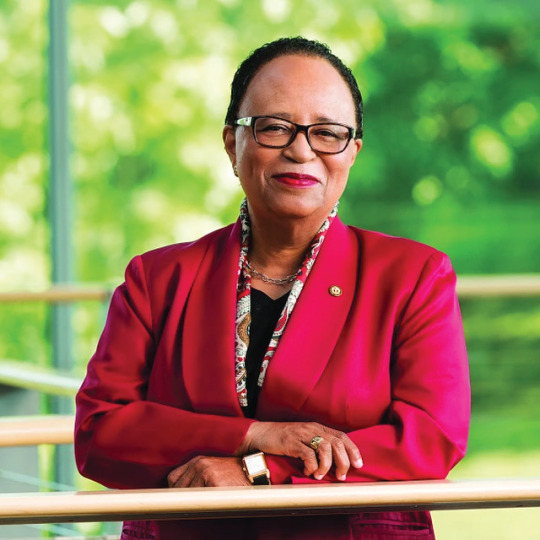
Dr. Shirley Ann Jackson (August 5, 1946) is a Physicist, born in DC. She has achieved numerous firsts for African American women. She was the first African woman to earn a Ph.D. from MIT; to receive a Ph.D. in theoretical solid state physics; to be elected president and then chairman of the board of the American Association for the Advancement of Science; to be president of a major research university, Rensselaer Polytechnic Institute; and to be elected to the National Academy of Engineering. She was both the first African American and the first woman to chair the US Nuclear Regulatory Commission.
She was accepted at MIT, where she was one of the very few women and even fewer African Americans. Despite discouraging remarks from her professors about the appropriateness of science for an African American woman, she chose to major in Physics and earned her BS. She continued at MIT for graduate school, studying under the first African American Physics professor in her department, James Young. She earned her Ph.D.
She completed several years of postdoctoral research at various laboratories, such as Fermi in Illinois, before being hired by AT&T Bell Laboratories, where she worked for 15 years. She researched the optical and electronic properties of layered materials, surface electrons of liquid helium films, strained-layer semiconductor superlattices, and most notably, the polaronic aspects of electrons in two-dimensional systems. She is considered a leading developer of Caller ID and Call Waiting on telephones.
After teaching at Rutgers University, she was appointed chair of the US Nuclear Regulatory Commission by Bill Clinton. She became President of Rensselaer Polytechnic Institute, where she still serves today. She was elected president of AAAS and she served as chairman of the board for the Society. She is married to a physicist Morris A Washington and has one son. #africanhistory365 #africanexcellence #deltasigmatheta
4 notes
·
View notes
Text
Interactive mouthpiece opens new opportunities for health data, assistive technology, and hands-free interactions
New Post has been published on https://thedigitalinsider.com/interactive-mouthpiece-opens-new-opportunities-for-health-data-assistive-technology-and-hands-free-interactions/
Interactive mouthpiece opens new opportunities for health data, assistive technology, and hands-free interactions
When you think about hands-free devices, you might picture Alexa and other voice-activated in-home assistants, Bluetooth earpieces, or asking Siri to make a phone call in your car. You might not imagine using your mouth to communicate with other devices like a computer or a phone remotely.
Thinking outside the box, MIT Computer Science and Artificial Intelligence Laboratory (CSAIL) and Aarhus University researchers have now engineered “MouthIO,” a dental brace that can be fabricated with sensors and feedback components to capture in-mouth interactions and data. This interactive wearable could eventually assist dentists and other doctors with collecting health data and help motor-impaired individuals interact with a phone, computer, or fitness tracker using their mouths.
Resembling an electronic retainer, MouthIO is a see-through brace that fits the specifications of your upper or lower set of teeth from a scan. The researchers created a plugin for the modeling software Blender to help users tailor the device to fit a dental scan, where you can then 3D print your design in dental resin. This computer-aided design tool allows users to digitally customize a panel (called PCB housing) on the side to integrate electronic components like batteries, sensors (including detectors for temperature and acceleration, as well as tongue-touch sensors), and actuators (like vibration motors and LEDs for feedback). You can also place small electronics outside of the PCB housing on individual teeth.
Play video
MouthIO: Fabricating Customizable Oral User Interfaces with Integrated Sensing and Actuation Video: MIT CSAIL
The active mouth
“The mouth is a really interesting place for an interactive wearable and can open up many opportunities, but has remained largely unexplored due to its complexity,” says senior author Michael Wessely, a former CSAIL postdoc and senior author on a paper about MouthIO who is now an assistant professor at Aarhus University. “This compact, humid environment has elaborate geometries, making it hard to build a wearable interface to place inside. With MouthIO, though, we’ve developed a new kind of device that’s comfortable, safe, and almost invisible to others. Dentists and other doctors are eager about MouthIO for its potential to provide new health insights, tracking things like teeth grinding and potentially bacteria in your saliva.”
The excitement for MouthIO’s potential in health monitoring stems from initial experiments. The team found that their device could track bruxism (the habit of grinding teeth) by embedding an accelerometer within the brace to track jaw movements. When attached to the lower set of teeth, MouthIO detected when users grind and bite, with the data charted to show how often users did each.
Wessely and his colleagues’ customizable brace could one day help users with motor impairments, too. The team connected small touchpads to MouthIO, helping detect when a user’s tongue taps their teeth. These interactions could be sent via Bluetooth to scroll across a webpage, for example, allowing the tongue to act as a “third hand” to open up a new avenue for hands-free interaction.
“MouthIO is a great example how miniature electronics now allow us to integrate sensing into a broad range of everyday interactions,” says study co-author Stefanie Mueller, the TIBCO Career Development Associate Professor in the MIT departments of Electrical Engineering and Computer Science and Mechanical Engineering and leader of the HCI Engineering Group at CSAIL. “I’m especially excited about the potential to help improve accessibility and track potential health issues among users.”
Molding and making MouthIO
To get a 3D model of your teeth, you can first create a physical impression and fill it with plaster. You can then scan your mold with a mobile app like Polycam and upload that to Blender. Using the researchers’ plugin within this program, you can clean up your dental scan to outline a precise brace design. Finally, you 3D print your digital creation in clear dental resin, where the electronic components can then be soldered on. Users can create a standard brace that covers their teeth, or opt for an “open-bite” design within their Blender plugin. The latter fits more like open-finger gloves, exposing the tips of your teeth, which helps users avoid lisping and talk naturally.
This “do it yourself” method costs roughly $15 to produce and takes two hours to be 3D-printed. MouthIO can also be fabricated with a more expensive, professional-level teeth scanner similar to what dentists and orthodontists use, which is faster and less labor-intensive.
Compared to its closed counterpart, which fully covers your teeth, the researchers view the open-bite design as a more comfortable option. The team preferred to use it for beverage monitoring experiments, where they fabricated a brace capable of alerting users when a drink was too hot. This iteration of MouthIO had a temperature sensor and a monitor embedded within the PCB housing that vibrated when a drink exceeded 65 degrees Celsius (or 149 degrees Fahrenheit). This could help individuals with mouth numbness better understand what they’re consuming.
In a user study, participants also preferred the open-bite version of MouthIO. “We found that our device could be suitable for everyday use in the future,” says study lead author and Aarhus University PhD student Yijing Jiang. “Since the tongue can touch the front teeth in our open-bite design, users don’t have a lisp. This made users feel more comfortable wearing the device during extended periods with breaks, similar to how people use retainers.”
The team’s initial findings indicate that MouthIO is a cost-effective, accessible, and customizable interface, and the team is working on a more long-term study to evaluate its viability further. They’re looking to improve its design, including experimenting with more flexible materials, and placing it in other parts of the mouth, like the cheek and the palate. Among these ideas, the researchers have already prototyped two new designs for MouthIO: a single-sided brace for even higher comfort when wearing MouthIO while also being fully invisible to others, and another fully capable of wireless charging and communication.
Jiang, Mueller, and Wessely’s co-authors include PhD student Julia Kleinau, master’s student Till Max Eckroth, and associate professor Eve Hoggan, all of Aarhus University. Their work was supported by a Novo Nordisk Foundation grant and was presented at ACM’s Symposium on User Interface Software and Technology.
#3-D printing#3d#3D model#Accessibility#alexa#app#artificial#Artificial Intelligence#Assistive technology#author#Bacteria#batteries#bluetooth#box#Capture#career#career development#communication#complexity#computer#Computer Science#Computer Science and Artificial Intelligence Laboratory (CSAIL)#Computer science and technology#data#dental#Design#development#devices#do it yourself#Electrical engineering and computer science (EECS)
2 notes
·
View notes
Text
What Was the First Video Game: A Brief History of Gaming's Origins
Video games have become a ubiquitous part of modern entertainment, with millions of people around the world playing them every day. But where did it all begin? What was the first video game ever created? The answer to this question is not as straightforward as one might think.
While there is no definitive answer to the question of what was the first He tuber video game, there are a number of contenders for the title. Some of the earliest examples of electronic games date back to the 1940s and 1950s, when scientists and engineers were experimenting with computers and other electronic devices. These early games were often simple and text-based, but they laid the groundwork for the more complex and sophisticated games that would follow in the decades to come.
Over the years, a number of games have been cited as the first true video game, including "Tennis for Two," which was created in 1958 by physicist William Higinbotham, and "Spacewar!," which was developed in 1962 by a group of students at MIT. While the debate over which game should be considered the first video game will likely continue for years to come, there is no denying the impact that these early games had on the development of the video game industry as we know it today.
Origins of Video Gaming
Early Concepts
The first video game was created in October 1958 by physicist William Higinbotham. The game, called "Tennis for Two," was a simple tennis game that was played on an oscilloscope. The game was designed to entertain visitors at a science fair, and it became an instant hit. The success of "Tennis for Two" paved the way for the development of video games.
In the following years, many more games were developed, such as "Spacewar!" in 1962, which was the first game to be played on multiple computers. These early games were played on large mainframe computers and were mostly developed by students and researchers.
Technological Advances
The development of microprocessors in the 1970s allowed for the creation of smaller and more affordable computers, which led to the creation of the first home video game consoles. The first commercially successful video game console was the Magnavox Odyssey, released in 1972. It was followed by the Atari 2600 in 1977, which became the most popular console of its time.
As technology continued to advance, video games became more sophisticated and complex. The introduction of 3D graphics in the 1990s revolutionized the industry, and games like Doom and Quake became instant classics. Today, video games are a multi-billion dollar industry, with millions of players around the world.
First Recognized Video Game
The first recognized video game was created in the 1950s, when computer technology was in its early stages. The game was a simple tennis game that was played on an oscilloscope, a type of electronic display screen. The game was called "Tennis for Two" and was created by William Higinbotham, a physicist at Brookhaven National Laboratory.
Tennis for Two
"Tennis for Two" was created in 1958 and was played on an oscilloscope. The game was played by two players who used knobs to control the movement of their paddles. The aim of the game was to hit a dot back and forth across the screen, similar to a game of tennis. The game was a simple concept, but it was groundbreaking at the time.
The game was not commercialized or widely distributed, but it paved the way for the development of future video games. It was a significant achievement in the history of video games and was the first step towards the development of the video game industry.
Overall, "Tennis for Two" was a remarkable achievement in the history of video games. It was the first recognized video game and paved the way for the development of the video game industry.
Notable Mentions
Spacewar!
While Tennis for Two is often cited as the first video game, Spacewar! is considered by many to be the first true video game. Created in 1962 by Steve Russell and his colleagues at MIT, Spacewar! was a two-player game in which players controlled spaceships and attempted to shoot each other while avoiding the gravity well of a nearby star. The game was a hit among computer enthusiasts, and it paved the way for future video games.
Pong
Pong, created in 1972 by Atari co-founder Nolan Bushnell and engineer Al Alcorn, is often credited with popularizing video games and launching the industry. Pong was a simple game that simulated table tennis, with players using paddles to hit a ball back and forth across the screen. Despite its simplicity, Pong was a massive success and spawned a slew of imitators and sequels. Today, Pong is remembered as a classic and an important milestone in video game history.
Evolution of Video Games
Arcade Era
The history of video games can be traced back to the 1940s and 1950s when computer scientists began designing simple games and simulations as part of their research. However, the first commercially successful video game was created in the early 1970s during the arcade era. The game was called Pong and was developed by Atari, a company that would become synonymous with the video game industry.
Pong was a simple game that involved two players using paddles to hit a ball back and forth across a screen. Despite its simplicity, Pong was a huge success and spawned a wave of imitators and competitors. This led to the development of more sophisticated games such as Space Invaders, Pac-Man, and Donkey Kong.
Home Console Market
The success of arcade games led to the development of home consoles in the late 1970s and early 1980s. The first home console was the Magnavox Odyssey, released in 1972. However, it was the release of the Atari 2600 in 1977 that really kicked off the home console market.
The Atari 2600 was a huge success and helped to establish video games as a mainstream form of entertainment. Other companies soon entered the market, including Nintendo with their Nintendo Entertainment System (NES) in 1985 and Sega with their Sega Genesis in 1988.
The home console market continued to evolve throughout the 1990s and 2000s with the release of more powerful consoles such as the Sony PlayStation and Microsoft Xbox. Today, video games are a multi-billion dollar industry and are played by millions of people around the world.
Impact and Legacy
Industry Growth
The impact of the first video game on the industry cannot be overstated. While it was initially created as a simple experiment, it paved the way for a whole new form of entertainment. The success of the game led to the creation of countless other video games, and the industry has continued to grow and evolve ever since.
Video games have become a major part of the entertainment industry, with millions of people playing them every day. The industry has also become a major employer, with thousands of people working in various roles, from game designers to programmers to marketers.
Cultural Influence
The first video game also had a major cultural impact. It introduced the concept of interactive entertainment, which has become a defining characteristic of modern culture. Video games have become a major part of popular culture, with many games featuring iconic characters and storylines that have become beloved by fans.
Video games have also had an impact on other forms of media, such as movies and television. Many popular movies and TV shows have been influenced by video games, and some have even been adapted into video games themselves.
Overall, the impact and legacy of the first video game cannot be overstated. It paved the way for a whole new form of entertainment and has had a major impact on both the entertainment industry and popular culture.
2 notes
·
View notes
Link
1 note
·
View note
Text
Learning Robotic Engineering in USA
Combining computer science, electrical engineering, and mechanical engineering, robotic engineering is a fascinating and quickly developing area. In addition to being one of the leading competitors of contemporary technology, robotic engineering is one of the most popular professions in the world, with applications spanning from manufacturing to healthcare to even space exploration. With some of the most prominent schools in the world, cutting-edge facilities, and industry links, the USA is a great place for anyone thinking about studying robotic engineering.
Why Study Robotic Engineering in the USA?
Innovative degrees: MIT, Stanford, and Carnegie Mellon are just a few of the top colleges in the USA that provide innovative robotic engineering degrees. These universities are renowned for their advanced laboratories, industrial collaborations, and industry-driven curricula.
Career Opportunities: There are a lot of career options in the USA because of the record-high need for automation, artificial intelligence, and robotics expertise. To innovate and streamline procedures, businesses in industries including manufacturing, aerospace, healthcare, and automotive are actively looking for qualified robotic engineers.
Opportunities for Innovation and Research: Numerous American institutions have specialized research labs where students can work together on projects like humanoid robots, robotic surgical systems, and driverless cars. This access to the latest research offers priceless practical experience.
Industry links and internships The educational experience offered by studying robotic engineering in the USA is unmatched, giving students the abilities, know-how, and professional connections necessary to succeed in this fast-paced industry. The USA continues to be one of the greatest places for anyone wishing to pursue a career in robotic engineering because of its availability to prestigious colleges, state-of-the-art research, and substantial employment opportunities. Students studying in the United States have the opportunity to work as interns for such companies as Boston Dynamics, Tesla, and Google. In addition to helping students develop real-world skills, internships open doors for future full-time jobs following graduation.
Key Courses and Skills in Robotic Engineering
Programming and Algorithms
Mathematics and Physics
Electronics and Embedded Systems
Machine Learning and AI
Mechanical Design and CAD
Career Prospects After Studying Robotic Engineering
Automation Engineer
Robotics Software Developer
Research Scientist
Field Robotics Engineer
Healthcare Robotics Engineer
The educational experience offered by studying robotic engineering in the USA is unmatched, giving students the abilities, know-how, and professional connections necessary to succeed in this fast-paced industry. The USA continues to be one of the greatest places for anyone wishing to pursue a career in robotic engineering because of its availability to prestigious colleges, state-of-the-art research, and substantial employment opportunities.
To know more click here
0 notes
Text
Quantum simulator could help uncover materials for high-performance electronics
New Post has been published on https://sunalei.org/news/quantum-simulator-could-help-uncover-materials-for-high-performance-electronics/
Quantum simulator could help uncover materials for high-performance electronics

Quantum computers hold the promise to emulate complex materials, helping researchers better understand the physical properties that arise from interacting atoms and electrons. This may one day lead to the discovery or design of better semiconductors, insulators, or superconductors that could be used to make ever faster, more powerful, and more energy-efficient electronics.
But some phenomena that occur in materials can be challenging to mimic using quantum computers, leaving gaps in the problems that scientists have explored with quantum hardware.
To fill one of these gaps, MIT researchers developed a technique to generate synthetic electromagnetic fields on superconducting quantum processors. The team demonstrated the technique on a processor comprising 16 qubits.
By dynamically controlling how the 16 qubits in their processor are coupled to one another, the researchers were able to emulate how electrons move between atoms in the presence of an electromagnetic field. Moreover, the synthetic electromagnetic field is broadly adjustable, enabling scientists to explore a range of material properties.
Emulating electromagnetic fields is crucial to fully explore the properties of materials. In the future, this technique could shed light on key features of electronic systems, such as conductivity, polarization, and magnetization.
“Quantum computers are powerful tools for studying the physics of materials and other quantum mechanical systems. Our work enables us to simulate much more of the rich physics that has captivated materials scientists,” says Ilan Rosen, an MIT postdoc and lead author of a paper on the quantum simulator.
The senior author is William D. Oliver, the Henry Ellis Warren professor of electrical engineering and computer science and of physics, director of the Center for Quantum Engineering, leader of the Engineering Quantum Systems group, and associate director of the Research Laboratory of Electronics. Oliver and Rosen are joined by others in the departments of Electrical Engineering and Computer Science and of Physics and at MIT Lincoln Laboratory. The research appears today in Nature Physics.
A quantum emulator
Companies like IBM and Google are striving to build large-scale digital quantum computers that hold the promise of outperforming their classical counterparts by running certain algorithms far more rapidly.
But that’s not all quantum computers can do. The dynamics of qubits and their couplings can also be carefully constructed to mimic the behavior of electrons as they move among atoms in solids.
“That leads to an obvious application, which is to use these superconducting quantum computers as emulators of materials,” says Jeffrey Grover, a research scientist at MIT and co-author on the paper.
Rather than trying to build large-scale digital quantum computers to solve extremely complex problems, researchers can use the qubits in smaller-scale quantum computers as analog devices to replicate a material system in a controlled environment.
“General-purpose digital quantum simulators hold tremendous promise, but they are still a long way off. Analog emulation is another approach that may yield useful results in the near-term, particularly for studying materials. It is a straightforward and powerful application of quantum hardware,” explains Rosen. “Using an analog quantum emulator, I can intentionally set a starting point and then watch what unfolds as a function of time.”
Despite their close similarity to materials, there are a few important ingredients in materials that can’t be easily reflected on quantum computing hardware. One such ingredient is a magnetic field.
In materials, electrons “live” in atomic orbitals. When two atoms are close to one another, their orbitals overlap and electrons can “hop” from one atom to another. In the presence of a magnetic field, that hopping behavior becomes more complex.
On a superconducting quantum computer, microwave photons hopping between qubits are used to mimic electrons hopping between atoms. But, because photons are not charged particles like electrons, the photons’ hopping behavior would remain the same in a physical magnetic field.
Since they can’t just turn on a magnetic field in their simulator, the MIT team employed a few tricks to synthesize the effects of one instead.
Tuning up the processor
The researchers adjusted how adjacent qubits in the processor were coupled to each other to create the same complex hopping behavior that electromagnetic fields cause in electrons.
To do that, they slightly changed the energy of each qubit by applying different microwave signals. Usually, researchers will set qubits to the same energy so that photons can hop from one to another. But for this technique, they dynamically varied the energy of each qubit to change how they communicate with each other.
By precisely modulating these energy levels, the researchers enabled photons to hop between qubits in the same complex manner that electrons hop between atoms in a magnetic field.
Plus, because they can finely tune the microwave signals, they can emulate a range of electromagnetic fields with different strengths and distributions.
The researchers undertook several rounds of experiments to determine what energy to set for each qubit, how strongly to modulate them, and the microwave frequency to use.
“The most challenging part was finding modulation settings for each qubit so that all 16 qubits work at once,” Rosen says.
Once they arrived at the right settings, they confirmed that the dynamics of the photons uphold several equations that form the foundation of electromagnetism. They also demonstrated the “Hall effect,” a conduction phenomenon that exists in the presence of an electromagnetic field.
These results show that their synthetic electromagnetic field behaves like the real thing.
Moving forward, they could use this technique to precisely study complex phenomena in condensed matter physics, such as phase transitions that occur when a material changes from a conductor to an insulator.
“A nice feature of our emulator is that we need only change the modulation amplitude or frequency to mimic a different material system. In this way, we can scan over many materials properties or model parameters without having to physically fabricate a new device each time.” says Oliver.
While this work was an initial demonstration of a synthetic electromagnetic field, it opens the door to many potential discoveries, Rosen says.
“The beauty of quantum computers is that we can look at exactly what is happening at every moment in time on every qubit, so we have all this information at our disposal. We are in a very exciting place for the future,” he adds.
This work is supported, in part, by the U.S. Department of Energy, the U.S. Defense Advanced Research Projects Agency (DARPA), the U.S. Army Research Office, the Oak Ridge Institute for Science and Education, the Office of the Director of National Intelligence, NASA, and the National Science Foundation.
0 notes
Text
Tier 1 Engineering Colleges in India
India is home to some of the world's most prestigious engineering institutions, renowned for their academic excellence, cutting-edge research, and outstanding placement records. These Tier-1 engineering colleges are the dream destinations for aspiring engineers across the country. This comprehensive guide will cover everything you need to know about these top institutions, including eligibility, fee structure, specializations offered, accreditations, campus life, admission processes, entrance exams, placements, return on investment (ROI), and course demand.
Top Tier-1 Engineering Colleges in India
Indian Institutes of Technology (IITs)
National Institutes of Technology (NITs)
Birla Institute of Technology and Science (BITS) Pilani
Indian Institute of Science (IISc) Bangalore
Delhi Technological University (DTU)
Vellore Institute of Technology (VIT)
Anna University, Chennai
Indian Institute of Information Technology (IIITs)
Manipal Institute of Technology (MIT)
Jadavpur University, Kolkata
Eligibility Criteria
To gain admission to Tier-1 engineering colleges in India, students must meet specific eligibility criteria:
Educational Qualification: Completion of 10+2 or equivalent with Physics, Chemistry, and Mathematics (PCM) as core subjects.
Minimum Marks: A minimum aggregate of 75% in 10+2 (relaxed for reserved categories) or top 20 percentile in respective boards.
Entrance Exams: Valid scores in national-level entrance exams like JEE Main, JEE Advanced, BITSAT, or state-level exams.
Fee Structure
The fee structure varies across different institutions:
IITs: Approx. INR 2-2.5 lakhs per annum.
NITs: Approx. INR 1.5-2 lakhs per annum.
BITS Pilani: Approx. INR 4 lakhs per annum.
IISc Bangalore: Approx. INR 30,000 per annum.
Private Universities (VIT, Manipal): INR 2-5 lakhs per annum.
Scholarships and financial aid are available based on merit and economic need.
Specializations Offered
Tier-1 engineering colleges offer a wide range of specializations, including but not limited to:
Computer Science and Engineering (CSE)
Electrical and Electronics Engineering (EEE)
Mechanical Engineering
Civil Engineering
Chemical Engineering
Biotechnology
Aerospace Engineering
Information Technology
Materials Science and Engineering
Environmental Engineering
College Accreditations
Accreditations ensure the quality and standards of education. Key accreditations include:
National Board of Accreditation (NBA)
National Assessment and Accreditation Council (NAAC)
University Grants Commission (UGC)
All India Council for Technical Education (AICTE)
Campus Life
Tier-1 engineering colleges are known for their vibrant campus life, which includes:
State-of-the-Art Infrastructure: Advanced laboratories, research centers, libraries, and modern classrooms.
Hostel Facilities: Comfortable accommodations with amenities like Wi-Fi, dining, and recreational facilities.
Extracurricular Activities: A plethora of clubs, sports, cultural events, and tech fests.
Admission Process
The admission process typically involves:
Entrance Exam: Clearing national-level exams like JEE Main, JEE Advanced, BITSAT, or respective state-level exams.
Counseling: Participating in the counseling process based on entrance exam ranks.
Document Verification: Submission and verification of required documents.
Seat Allotment: Securing a seat in the preferred college and specialization.
Strengthen your Class 11 & 12 foundation: Focus on mastering science and mathematics concepts. Enroll in coaching institutes or online resources to supplement your learning.
Entrance Exams
Several entrance exams are conducted for admission to Tier-1 engineering colleges:
JEE Main: For admission to NITs, IIITs, and CFTIs.
JEE Advanced: For admission to IITs.
BITSAT: For admission to BITS Pilani campuses.
VITEEE: For admission to VIT.
SRMJEEE: For admission to SRM University.
Placements
Tier-1 engineering colleges in India boast excellent placement records:
On-Campus Recruitment: Top companies conduct recruitment drives on campus.
Internship Opportunities: Students are provided with internships in leading companies.
Career Counseling: Guidance for interviews, resume building, and skill enhancement.
Top Recruiters: Google, Microsoft, Amazon, Intel, TCS, Infosys, Wipro, L&T, and many more.
Return on Investment (ROI)
The ROI of a degree from a Tier-1 engineering college is high due to:
High Starting Salaries: Graduates secure lucrative job offers.
Career Growth: Opportunities for rapid career advancement.
Global Opportunities: Access to international job markets and higher studies.
Course Demand
The demand for engineering courses from Tier-1 colleges remains consistently high due to:
Technological Advancements: Continuous growth in tech and innovation sectors.
Diverse Career Options: Opportunities in IT, manufacturing, research, consulting, and more.
Global Recognition: Degrees from these institutions are recognized and valued worldwide.
Summary
Tier-1 engineering colleges in India offer an unparalleled blend of academic excellence, cutting-edge research, and robust placement opportunities. These institutions provide a solid foundation for aspiring engineers, equipping them with the skills and knowledge required to excel in a rapidly evolving technological landscape. Whether you aim to pursue higher studies, secure a high-paying job, or embark on entrepreneurial ventures, a degree from a Tier-1 engineering college in India can be your gateway to a successful career.
#tier 1 engineering colleges in india#tier 1 private engineering colleges#top engineering colleges in india#upes#engineering
0 notes
Text
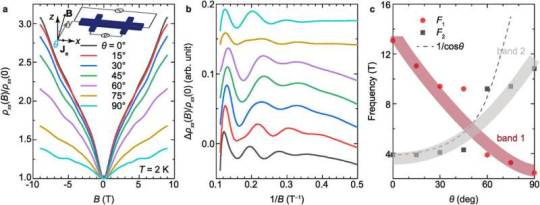
A new material for small electronics that gives batteries longer life
Scientists have achieved a series of milestones in growing a high-quality thin film conductor, suggesting in a new study that the material is a promising candidate platform for future wearable electronics and other miniature applications. Researchers at The Ohio State University, the Army Research Laboratory and MIT determined that the material is the best among similarly built films for its electron mobility—an index of how easy it is for an electrical current to pass through it. Coupled with low defect density to reduce interference with electron movement on the surface, the material is like a tiny empty freeway where all the electrons can easily get where they need to go with no traffic to be seen.
Read more.
9 notes
·
View notes
Text
Unlock Your Potential at Manipal Institute of Technology

Choosing the right educational institution is a crucial decision that can shape your future. Manipal Institute of Technology (MIT) stands out as a premier destination for students who aspire to excel in the fields of engineering and technology. Renowned for its academic excellence, state-of-the-art facilities, and student-centric approach, Manipal Institute of Technology offers an environment that nurtures talent and fosters innovation.
Diverse Academic Programs
Manipal Institute of Technology offers a wide range of undergraduate and postgraduate programs that cater to various interests and career goals. Whether you're passionate about computer science, mechanical engineering, electronics, or biotechnology, MIT has a program designed to equip you with the skills and knowledge needed to succeed in your chosen field. The curriculum is both rigorous and flexible, allowing students to gain a strong theoretical foundation while also engaging in hands-on learning experiences.
Experienced Faculty
At Manipal Institute of Technology, the faculty comprises seasoned academicians and industry professionals who are dedicated to providing high-quality education. Their expertise and commitment to teaching ensure that students receive a comprehensive education that is both relevant and forward-thinking. The faculty's mentorship helps students stay abreast of the latest industry trends and prepares them for the challenges of the professional world.
Cutting-Edge Infrastructure
The campus of Manipal Institute of Technology is equipped with modern facilities that enhance the learning experience. From advanced laboratories and high-tech classrooms to well-stocked libraries and comfortable dormitories, every aspect of the campus is designed to support academic and personal growth. The institution continually updates its infrastructure to incorporate the latest technological advancements, ensuring that students have access to the best resources.
Emphasis on Research and Innovation
Research and innovation are integral to the academic culture at Manipal Institute of Technology. Students are encouraged to participate in research projects and explore innovative solutions to real-world problems. The institution provides ample resources and support to help students bring their ideas to life. This emphasis on research fosters critical thinking and problem-solving skills, which are essential for success in today's competitive job market.
Global Exposure
Manipal Institute of Technology offers numerous opportunities for global exposure through its international collaborations and exchange programs. These initiatives allow students to gain valuable experience by studying abroad or participating in international projects. Exposure to different cultures and educational systems broadens students' perspectives and prepares them for global careers.
Vibrant Campus Life
Life at Manipal Institute of Technology is vibrant and full of opportunities for personal and professional development. The campus hosts a variety of extracurricular activities, ensuring that students have a well-rounded experience.
Clubs and Societies: Students can join various clubs and societies that cater to a wide range of interests, from arts and culture to sports and technology. These activities provide a platform for students to showcase their talents, develop new skills, and build lasting friendships.
Community Engagement: MIT encourages students to participate in community service and outreach programs, fostering a sense of social responsibility and making a positive impact on society.
Career Development: The institution offers comprehensive career development services, including career counseling, placement services, and internship opportunities. These services help students transition smoothly from academia to the professional world and achieve their career goals.
Strong Industry Connections
Manipal Institute of Technology has established strong ties with leading companies and organizations, offering students numerous opportunities for internships, projects, and employment. These industry connections ensure that students gain practical experience and are well-prepared for the demands of the job market. The institution's robust placement cell works tirelessly to match students with suitable job opportunities, ensuring a high rate of employment for graduates.
Support for Entrepreneurship
Manipal Institute of Technology fosters a culture of innovation and entrepreneurship. Students are encouraged to think creatively and develop solutions to real-world problems. The institution offers support for entrepreneurial ventures, helping students turn their ideas into successful businesses. This entrepreneurial spirit is nurtured through various initiatives, including incubators, mentorship programs, and startup competitions.
Personalized Attention and Support
At Manipal Institute of Technology, students receive personalized attention and support throughout their academic journey. The mentoring program ensures that each student has a mentor to guide them, providing academic advice, career guidance, and personal support. This personalized approach helps students navigate their educational journey with confidence and clarity.
Preparing for a Global Career
In today’s interconnected world, global experience is essential for success. Manipal Institute of Technology prepares students for international careers by offering exchange programs, collaborative projects, and language and cultural training. These opportunities help students gain a global perspective and succeed in diverse environments.
Conclusion
The journey to success begins with the right education, and Manipal Institute of Technology is the ideal partner on this journey. With its diverse academic programs, experienced faculty, cutting-edge facilities, and emphasis on research and global exposure, MIT offers an unparalleled educational experience. Choosing Manipal Institute of Technology means unlocking a world of opportunities and setting the foundation for a successful future. Join Manipal Institute of Technology today and take the first step towards achieving your dreams.
0 notes
Text
The Role of Engineering Colleges in Pune in Shaping Future Innovators
Pune, known as the educational hub of Maharashtra, has earned a distinguished reputation for its engineering colleges. These institutions are not just centers of academic excellence but also nurseries of future innovators who drive technological advancements and societal progress. The engineering colleges in Pune play a pivotal role in shaping the minds and careers of aspiring engineers, equipping them with the knowledge, skills, and mindset needed to become innovators and leaders in their fields.

Nurturing Innovation and Creativity
The best engineering colleges in Pune are committed to fostering a culture of innovation and creativity. They achieve this through a combination of rigorous academic programs, state-of-the-art facilities, and opportunities for practical application of knowledge. Institutions like the College of Engineering Pune (COEP) and the Maharashtra Institute of Technology (MIT) are known for their emphasis on research and development, encouraging students to engage in cutting-edge projects that push the boundaries of current technology.
Emphasis on Research and Development
Research is a cornerstone of innovation, and the top engineering colleges in Pune prioritize research initiatives. COEP, one of the top engineering colleges in Maharashtra, boasts numerous research centers and labs where students and faculty collaborate on projects ranging from sustainable energy solutions to advanced robotics. This focus on research not only enhances students' learning experiences but also contributes to the advancement of technology and society.
Industry Collaboration and Practical Training
The best colleges for engineering in Pune maintain strong ties with the industry, ensuring that their curricula are aligned with current technological trends and market needs. These collaborations often result in internship opportunities, industry-sponsored projects, and guest lectures by industry experts. For example, Pune Institute of Computer Technology (PICT), renowned as the best IT college in Pune, offers students extensive exposure to real-world challenges through partnerships with leading tech companies.
Specialized Programs and Cutting-Edge Courses
Engineering colleges in Pune offer a diverse array of specialized programs that cater to the evolving needs of the industry. From traditional disciplines to emerging fields, these colleges provide comprehensive education that prepares students for future challenges.
https://www.mmcoe.edu.in/templates/mmcoe/images/toppers/robocon1.jpg
Mechanical and Electrical Engineering
Mechanical and electrical engineering remain foundational disciplines with wide-ranging applications. The best mechanical engineering colleges in Pune, such as MIT and Vishwakarma Institute of Technology (VIT), provide robust programs that include hands-on training in modern laboratories. Similarly, electrical engineering colleges in Pune like COEP offer courses that cover the latest advancements in electronics, power systems, and telecommunications.
Computer Science and IT
In today’s digital age, computer science and information technology are among the most sought-after fields. The best computer engineering colleges in Pune, including PICT and MIT, offer rigorous programs that emphasize software development, cybersecurity, and data analytics. These colleges ensure that students are well-versed in the latest technologies and programming languages, making them highly competitive in the job market.
Conclusion
The engineering colleges in Pune play a crucial role in shaping future innovators. By providing a robust educational foundation, fostering a culture of research and development, offering specialized programs, and encouraging holistic development, these institutions prepare students to tackle the challenges of tomorrow.
0 notes
Text
New transistor’s superlative properties could have broad electronics applications
New Post has been published on https://thedigitalinsider.com/new-transistors-superlative-properties-could-have-broad-electronics-applications/
New transistor’s superlative properties could have broad electronics applications
In 2021, a team led by MIT physicists reported creating a new ultrathin ferroelectric material, or one where positive and negative charges separate into different layers. At the time they noted the material’s potential for applications in computer memory and much more. Now the same core team and colleagues — including two from the lab next door — have built a transistor with that material and shown that its properties are so useful that it could change the world of electronics.
Although the team’s results are based on a single transistor in the lab, “in several aspects its properties already meet or exceed industry standards” for the ferroelectric transistors produced today, says Pablo Jarillo-Herrero, the Cecil and Ida Green Professor of Physics, who led the work with professor of physics Raymond Ashoori. Both are also affiliated with the Materials Research Laboratory.
“In my lab we primarily do fundamental physics. This is one of the first, and perhaps most dramatic, examples of how very basic science has led to something that could have a major impact on applications,” Jarillo-Herrero says.
Says Ashoori, “When I think of my whole career in physics, this is the work that I think 10 to 20 years from now could change the world.”
Among the new transistor’s superlative properties:
It can switch between positive and negative charges — essentially the ones and zeros of digital information — at very high speeds, on nanosecond time scales. (A nanosecond is a billionth of a second.)
It is extremely tough. After 100 billion switches it still worked with no signs of degradation.
The material behind the magic is only billionths of a meter thick, one of the thinnest of its kind in the world. That, in turn, could allow for much denser computer memory storage. It could also lead to much more energy-efficient transistors because the voltage required for switching scales with material thickness. (Ultrathin equals ultralow voltages.)
The work is reported in a recent issue of Science. The co-first authors of the paper are Kenji Yasuda, now an assistant professor at Cornell University, and Evan Zalys-Geller, now at Atom Computing. Additional authors are Xirui Wang, an MIT graduate student in physics; Daniel Bennett and Efthimios Kaxiras of Harvard University; Suraj S. Cheema, an assistant professor in MIT’s Department of Electrical Engineering and Computer Science and an affiliate of the Research Laboratory of Electronics; and Kenji Watanabe and Takashi Taniguchi of the National Institute for Materials Science in Japan.
What they did
In a ferroelectric material, positive and negative charges spontaneously head to different sides, or poles. Upon the application of an external electric field, those charges switch sides, reversing the polarization. Switching the polarization can be used to encode digital information, and that information will be nonvolatile, or stable over time. It won’t change unless an electric field is applied. For a ferroelectric to have broad application to electronics, all of this needs to happen at room temperature.
The new ferroelectric material reported in Science in 2021 is based on atomically thin sheets of boron nitride that are stacked parallel to each other, a configuration that doesn’t exist in nature. In bulk boron nitride, the individual layers of boron nitride are instead rotated by 180 degrees.
It turns out that when an electric field is applied to this parallel stacked configuration, one layer of the new boron nitride material slides over the other, slightly changing the positions of the boron and nitrogen atoms. For example, imagine that each of your hands is composed of only one layer of cells. The new phenomenon is akin to pressing your hands together then slightly shifting one above the other.
“So the miracle is that by sliding the two layers a few angstroms, you end up with radically different electronics,” says Ashoori. The diameter of an atom is about 1 angstrom.
Another miracle: “nothing wears out in the sliding,” Ashoori continues. That’s why the new transistor could be switched 100 billion times without degrading. Compare that to the memory in a flash drive made with conventional materials. “Each time you write and erase a flash memory, you get some degradation,” says Ashoori. “Over time, it wears out, which means that you have to use some very sophisticated methods for distributing where you’re reading and writing on the chip.” The new material could make those steps obsolete.
A collaborative effort
Yasuda, the co-first author of the current Science paper, applauds the collaborations involved in the work. Among them, “we [Jarillo-Herrero’s team] made the material and, together with Ray [Ashoori] and [co-first author] Evan [Zalys-Geller], we measured its characteristics in detail. That was very exciting.” Says Ashoori, “many of the techniques in my lab just naturally applied to work that was going on in the lab next door. It’s been a lot of fun.”
Ashoori notes that “there’s a lot of interesting physics behind this” that could be explored. For example, “if you think about the two layers sliding past each other, where does that sliding start?” In addition, says Yasuda, could the ferroelectricity be triggered with something other than electricity, like an optical pulse? And is there a fundamental limit to the amount of switches the material can make?
Challenges remain. For example, the current way of producing the new ferroelectrics is difficult and not conducive to mass manufacturing. “We made a single transistor as a demonstration. If people could grow these materials on the wafer scale, we could create many, many more,” says Yasuda. He notes that different groups are already working to that end.
Concludes Ashoori, “There are a few problems. But if you solve them, this material fits in so many ways into potential future electronics. It’s very exciting.”
This work was supported by the U.S. Army Research Office, the MIT/Microsystems Technology Laboratories Samsung Semiconductor Research Fund, the U.S. National Science Foundation, the Gordon and Betty Moore Foundation, the Ramon Areces Foundation, the Basic Energy Sciences program of the U.S. Department of Energy, the Japan Society for the Promotion of Science, and the Ministry of Education, Culture, Sports, Science and Technology (MEXT) of Japan.
#2-D#affiliate#applications#atom#atoms#author#billion#boron nitride#career#Cells#change#chip#collaborative#computer#computer memory#Computer Science#Computer science and technology#computing#education#electric field#Electrical Engineering&Computer Science (eecs)#electricity#Electronics#energy#engineering#flash#Foundation#Fundamental#Future#green
2 notes
·
View notes
Text
Discovering Excellence: The Best University in Switzerland
Switzerland, with its picturesque landscapes, rich cultural heritage, and an unwavering commitment to educational excellence, is home to some of the world's most esteemed universities. Among these prestigious institutions, one name often rises to the top: ETH Zurich (Swiss Federal Institute of Technology in Zurich). Renowned for its cutting-edge research, exceptional faculty, and innovative approach to education, ETH Zurich stands as a beacon of academic brilliance in the heart of Europe.
A Legacy of Innovation and Excellence
Founded in 1855, ETH Zurich has cultivated a legacy that blends tradition with forward-thinking innovation. Its establishment was driven by the need to foster a new generation of engineers and scientists, capable of steering Switzerland into a future defined by technological and scientific advancements. Today, this legacy continues as ETH Zurich remains at the forefront of global research and education.
Pioneering Research and Breakthroughs
ETH Zurich's reputation for pioneering research is unparalleled. The university is home to numerous Nobel laureates, Fields Medalists, and Turing Award winners, whose groundbreaking work has left an indelible mark on the world. Whether it's in the realms of physics, chemistry, computer science, or engineering, ETH Zurich consistently pushes the boundaries of what is possible.
One of the most remarkable achievements in recent years is the development of the world's smallest transistor by ETH Zurich researchers. This innovation holds immense potential for the future of electronics, promising faster, more efficient, and smaller devices. Such breakthroughs are a testament to the university's commitment to addressing real-world challenges through scientific exploration and ingenuity.
An Interdisciplinary Approach to Learning
At ETH Zurich, education transcends traditional boundaries. The university fosters an interdisciplinary approach that encourages students to think beyond their primary fields of study. This holistic perspective is reflected in the diverse range of programs and courses offered, which allow students to tailor their education to their specific interests and career aspirations.
For instance, the Integrated Building Systems program combines elements of architecture, engineering, and environmental sciences to equip students with the skills needed to design sustainable and energy-efficient buildings. Similarly, the Data Science program bridges computer science, statistics, and business, preparing students to tackle the complex data-driven challenges of the modern world.
A Hub of Global Collaboration
ETH Zurich's impact extends far beyond the borders of Switzerland. The university boasts a vibrant international community, with students, researchers, and faculty members hailing from over 120 countries. This global perspective is integral to the university's mission, fostering a rich exchange of ideas and cultures.
Collaboration with leading universities and research institutions worldwide further amplifies ETH Zurich's influence. Through partnerships with institutions like MIT, Stanford, and the University of Tokyo, ETH Zurich engages in joint research projects, student exchanges, and academic conferences, creating a dynamic network of knowledge and innovation.
State-of-the-Art Facilities and Resources
To support its ambitious educational and research endeavors, ETH Zurich provides state-of-the-art facilities and resources. The university's main campus in Zurich is a marvel of modern architecture, seamlessly blending historic buildings with cutting-edge research laboratories and teaching spaces.
The ETH Zurich Library, one of the largest scientific and technical libraries in Switzerland, offers an extensive collection of books, journals, and digital resources. Additionally, the university's advanced computational infrastructure, including high-performance computing clusters and specialized software, empowers researchers to conduct complex simulations and data analyses.
Commitment to Sustainability
Sustainability is a core value at ETH Zurich, permeating every aspect of campus life and academic pursuits. The university is dedicated to reducing its environmental footprint and promoting sustainable practices. Initiatives such as the ETH Zurich Sustainability Program aim to integrate sustainability into research, teaching, and operations.
ETH Zurich's commitment to sustainability is also evident in its research efforts. Projects like the Future Cities Laboratory and the Energy Science Center focus on developing innovative solutions to global environmental challenges. By fostering a culture of sustainability, ETH Zurich prepares students to become leaders in creating a more sustainable and equitable world.
A Vibrant Campus Life
Beyond academics and research, ETH Zurich offers a vibrant and enriching campus life. Students have access to a wide range of extracurricular activities, from sports clubs and cultural societies to entrepreneurial initiatives and community service projects. These opportunities foster personal growth, leadership skills, and lifelong friendships.
The university's location in Zurich, one of the world's most livable cities, adds to the overall experience. With its stunning natural surroundings, vibrant arts scene, and bustling urban life, Zurich provides an ideal backdrop for students to thrive both academically and personally.
Shaping the Future
ETH Zurich's commitment to excellence, innovation, and global collaboration positions it as a leader in shaping the future of education and research. Its graduates are highly sought after by employers worldwide, known for their analytical skills, creativity, and ability to tackle complex challenges.
As ETH Zurich continues to push the boundaries of knowledge and discovery, it remains dedicated to nurturing the next generation of thinkers, innovators, and leaders. By fostering a culture of curiosity, collaboration, and sustainability, ETH Zurich ensures that its legacy of excellence will endure for generations to come.
Conclusion
In the landscape of global higher education, ETH Zurich stands out as a paragon of academic excellence and innovation. Its unwavering commitment to research, interdisciplinary learning, and sustainability, combined with a vibrant campus life and a global perspective, makes it the best university in Switzerland. For students seeking an education that transcends traditional boundaries and prepares them to make a significant impact on the world, ETH Zurich is the ultimate destination.
#study abroad#study in usa#study in canada#study in uk#university in france#study in germany#abroad education#study in france#study in netherlands
0 notes
Text
MIT researchers develop advanced machine learning models to detect pancreatic cancer

MIT researchers develop advanced machine learning models to detect pancreatic cancer. MIT CSAIL researchers develop advanced machine-learning models that outperform current methods in detecting pancreatic ductal adenocarcinoma. Prismatic perspectives pancreatic cancer The path forward The first documented case of pancreatic cancer dates from the 18th century. Since then, researchers have embarked on a long and difficult journey to better understand this elusive and deadly disease. To date, early intervention is the most effective cancer treatment. Unfortunately, due to its location deep within the abdomen, the pancreas is particularly difficult to detect early on. Scientists from the MIT Computer Science and Artificial Intelligence Laboratory (CSAIL), as well as Limor Appelbaum, a staff scientist in the Department of Radiation Oncology at Beth Israel Deaconess Medical Center (BIDMC), wanted to better identify potential high-risk patients. They set out to create two machine-learning models for the early detection of pancreatic ductal adenocarcinoma (PDAC), the most common type of cancer. To gain access to a large and diverse database, the team collaborated with a federated network company and used electronic health record data from multiple institutions across the United States. This vast data set contributed to the models' reliability and generalizability, making them applicable to a wide range of populations, geographical locations, and demographic groups. The two models—the “PRISM” neural network and the logistic regression model (a statistical technique for probability)—outperformed current methods. The team’s comparison showed that while standard screening criteria identify about 10 percent of PDAC cases using a five-times higher relative risk threshold, Prism can detect 35 percent of PDAC cases at this same threshold. Using AI to detect cancer risk is not a new phenomenon; algorithms analyze mammograms, CT scans for lung cancer, and assist in the analysis of Pap smear tests and HPV testing, to name a few applications. “The PRISM models stand out for their development and validation on an extensive database of over 5 million patients, surpassing the scale of most prior research in the field,” says Kai Jia, an MIT PhD student in electrical engineering and computer science (EECS), MIT CSAIL affiliate, and first author on an open-access paper in eBioMedicine outlining the new work. “The model uses routine clinical and lab data to make its predictions, and the diversity of the U.S. population is a significant advancement over other PDAC models, which are usually confined to specific geographic regions, like a few health-care centers in the U.S. Additionally, using a unique regularization technique in the training process enhanced the models' generalizability and interpretability.” “This report outlines a powerful approach to use big data and artificial intelligence algorithms to refine our approach to identifying risk profiles for cancer,” says David Avigan, a Harvard Medical School professor and the cancer center director and chief of hematology and hematologic malignancies at BIDMC, who was not involved in the study. “This approach may lead to novel strategies to identify patients with high risk for malignancy that may benefit from focused screening with the potential for early intervention.”
Prismatic perspectives pancreatic cancer
The journey toward the development of PRISM began over six years ago, fueled by firsthand experiences with the limitations of current diagnostic practices. “Approximately 80-85 percent of pancreatic cancer patients are diagnosed at advanced stages, where cure is no longer an option,” says senior author Appelbaum, who is also a Harvard Medical School instructor as well as radiation oncologist. “This clinical frustration sparked the idea to delve into the wealth of data available in electronic health records (EHRs).” The CSAIL group’s close collaboration with Appelbaum made it possible to understand the combined medical and machine learning aspects of the problem better, eventually leading to a much more accurate and transparent model. “The hypothesis was that these records contained hidden clues — subtle signs and symptoms that could act as early warning signals of pancreatic cancer,” she adds. “This guided our use of federated EHR networks in developing these models, for a scalable approach for deploying risk prediction tools in health care.” Both PrismNN and PrismLR models analyze EHR data, including patient demographics, diagnoses, medications, and lab results, to assess PDAC risk. PrismNN uses artificial neural networks to detect intricate patterns in data features like age, medical history, and lab results, yielding a risk score for PDAC likelihood. PrismLR uses logistic regression for a simpler analysis, generating a probability score of PDAC based on these features. Together, the models offer a thorough evaluation of different approaches in predicting PDAC risk from the same EHR data. One paramount point for gaining the trust of physicians, the team notes, is better understanding how the models work, known in the field as interpretability. The scientists pointed out that while logistic regression models are inherently easier to interpret, recent advancements have made deep neural networks somewhat more transparent. This helped the team to refine the thousands of potentially predictive features derived from EHR of a single patient to approximately 85 critical indicators. These indicators, which include patient age, diabetes diagnosis, and an increased frequency of visits to physicians, are automatically discovered by the model but match physicians' understanding of risk factors associated with pancreatic cancer.
The path forward
Despite the promise of the PRISM models, as with all research, some parts are still a work in progress. U.S. data alone are the current diet for the models, necessitating testing and adaptation for global use. The path forward, the team notes, includes expanding the model's applicability to international datasets and integrating additional biomarkers for more refined risk assessment. “A subsequent aim for us is to facilitate the models' implementation in routine health care settings. The vision is to have these models function seamlessly in the background of health care systems, automatically analyzing patient data and alerting physicians to high-risk cases without adding to their workload,” says Jia. “A machine-learning model integrated with the EHR system could empower physicians with early alerts for high-risk patients, potentially enabling interventions well before symptoms manifest. We are eager to deploy our techniques in the real world to help all individuals enjoy longer, healthier lives.” Jia wrote the paper alongside Applebaum and MIT EECS Professor and CSAIL Principal Investigator Martin Rinard, who are both senior authors of the paper. Researchers on the paper were supported during their time at MIT CSAIL, in part, by the Defense Advanced Research Projects Agency, Boeing, the National Science Foundation, and Aarno Labs. TriNetX provided resources for the project, and the Prevent Cancer Foundation also supported the team. Source: MIT Read the full article
0 notes