#Enzymes & Derivates SA
Explore tagged Tumblr posts
Text
Manifestare anuală, Topul Firmelor Nemțene rămâne cel mai amplu eveniment prin care managerii de elită ai județului sunt recompensați, simbolic, pentru activitatea lor, prilej pentru schimburi de idei și experiență, pentru cunoaștere reciprocă și, nu în ultimul rând, pentru a se simți bine în cadrul unei festivități care le este, exclusiv, destinată și dedicată.
Odată trecut momentul festiv, se pot desprinde câteva concluzii din activitatea economică a județului aferentă anului trecut, iar, pentru a face asta, cea mai potrivită cale o reprezintă o discuție cu Mihai Apopii, președintele Camerei de Comerț și Industrie Neamț, gazda manifestării.
– Domnule președinte, o nouă ediție a Topului Firmelor Nemțene, într-o perioadă nu prea prietenoasă în general și cu mediul de afaceri în special. Este cea de-a XXIV-a ediție și poate ar fi bine, pentru început, să jalonăm evenimentul.
Topul reprezintă deja o manifestare de referință pentru comunitatea de afaceri din Neamț. Este, în egală măsură, un barometru al stării economice a județului și o modalitate care, printr-o metodologie unitară, realizează un clasament al firmelor, după domeniul de activitate, în funcție de niște parametri importanți din activitatea unei firme, după o formulă care a fost prezentată. Interesul oamenilor de afaceri este evident, participarea a fost pe măsură, iar prilejul ca, în același loc, să fie cele mai importante societăți comerciale, din toate domeniile de activitate, și să se poată interacționa este unic. Cred că ați observat, toată lumea s-a simțit bine, momentele artistice au fost gustate, dar cred că cel mai important lucru rămâne faptul că oamenii s-au văzut, s-au cunoscut, au putut interacționa și, în felul acesta, se naște sentimentul de apartenență la o comunitate. Este lucrul pe care Camera de Comerț și-l propune, ca una dintre priorități, pe lângă celelalte activități camerale.
În același context, trebuie să menționez că poate cea mai importantă sursă de informare pentru cei care doresc să facă afaceri în Neamț este catalogul ”Topul Firmelor Nemțene”, care reflectă o stare de lucruri reală și este ca un ”certificat de bonitate” pentru firmele clasate în top.
– Haideți să încercăm să-i caracterizăm, într-o frază, pe cei care au făcut posibilă organizarea unui asemenea eveniment.
Eu spun așa: printr-un management din ce în ce mai modern, firmele din Neamț reușesc, în condiții vitrege de multe ori, prin tenacitate, ambiție, flexibilitate, nu numai să reziste, dar să și sporească valoarea economică a județului. Este meritul celor care le conduc și care, mărturisesc cu bucurie, devin din ce în ce mai interesați să se instruiască în tehnicile de a conduce o firmă. Nu mai lasă lucrurile să meargă la întâmplare, încearcă să prevadă orice i-ar putea afecta ca activitate, se orientează spre noi piețe de desfacere și asta se vede în rezultatele obținute. Profit de moment pentru ca, în numele Colegiului de Conducere al Camerei de Comerț și Industrie Neamț, al întregului colectiv și al meu, personal, să-i felicit pe antreprenorii din Topul Firmelor 2016 și să le mulțumesc tuturor celor care ne-au sprijinit să realizăm acest eveniment de anvergură, al elitei mediului de afaceri nemțean.
* De 10 ani în Topul Firmelor Nemțene!
Kober SRL, Petrocart SA, Rifil SA, Tehnoprod SRL, Smirodava SA, Caremil SRL, Mecanica Ceahlău SA, Marsat SA, Moldocor SA, International Private Security SRL, Metex SA, Enzymes & Derivates SA, Centrul de cercetare și prelucrare a plantelor medicinale Plantavorel SA, Dinamic 92 Distribution SRL, Panauto SRL, Auto Moldova SA
– Camera de Comerț și Industrie Neamț este una dintre filialele cele mai active la nivel național, iar dezbaterile organizate pe diferite probleme de interes au generat, de multe ori, proiecte de legi, modificări legislative, după ce au scos la iveală probleme generale de sistem.
Da, dar trebuie să vă mărturisesc ceva. Inițiativa pentru asemenea dezbateri a venit, de cele mai multe ori, din partea oamenilor de afaceri, care au cerut să se întâlnească, să-și spună păsul, iar atunci când o problemă se dovedea a fi de importanță generală, noi, la Cameră, încercam să găsim o rezolvare, în sensul că identificam exact problema, vedeam ce se poate schimba și cum, după care trimiteam totul spre decidenții politici. Parlamentarii de Neamț, dar nu numai ei – pentru că, prin Camera Națională, propunerile ajungeau direct în comisiile de specialitate ale legislativului, la ministerele interesate și implicate – au primit aceste materiale, le-au primit și cei din conducerea județului și, trebuie să mărturisesc, în general răspunsul a fost pozitiv. Semnalul dinspre Consiliul Județean a fost unul pozitiv, actualul președinte, Ionel Arsene, având inițiative spre mediul de afaceri, fiind alături de noi în înființarea Biroului Unic, dar a și făcut pași spre a cunoaște și a promova nevoile stringente ale județului. De exemplu, colaborarea cu clusterul ASTRICO s-a materializat prin promovarea făcută la Bruxelles, se fac pași pentru materializarea ideii de parc industral, am discutat de multe ori despre un pavilion expozițional demn de județul Neamț și multe alte lucruri.
– Suntem într-un moment de sărbătoare, când trebuie să evidențiem aspectele pozitive. Ce anume credeți că ar trebui spus în acest moment?
Bucuria mea, ca președinte al Camerei, dar și ca om de afaceri, este că pot afirma că, în Neamț, avem firme extrem de puternice pe plan național, premiate an de an în Top și care confirmă constant acest statut. În același timp, avem și societăți care au intrat abia anul acesta în top, dar care demonstrează vitalitate și un ritm de creștere impresionant. Vă pot face o sinteză semnificativă: avem, în Neamț, 9.996 firme care au depus bilanț, din care 9.499 sunt angajatori activi, 50.367 salariați lucrează în firmele care au depus bilanț, iar la nivel județean au fost înregistrate 87.386 contracte de muncă, diferența fiind dată de contractele suspendate, de cele pe perioade detreminate sau de contractele multiple. La nivelul anului 2016, profitul brut a fost de 139,21 milioane de euro, în creștere cu 9,1% față de 2015, când suma era de 126,84 milioane de euro.
* Firme nou intrate în Topul Firmelor Nemțene
Cityshuttle Mobility Solutions SRL, Dinamic 92 Pro Logistics SRL, Nekos Security SRL, Vitamin Aqua SRL, Maria’s Ladybugs SRL, Serviciile Comerciale Române SA (reintrată în top cu altă activitate), Iosidoro SRL (berea Nemțeana), Super Pretzel SRL, Korona Construcții Lemn SRL, Almob Reia SRL, Larixplantsil SRL, Claudiu Demolări SRL-D, La Defense SRL-D, Laura Turism SRL
– Sunt semne foarte bune, dar asta nu înseamnă că nu trebuie ajutate toate societățile din Neamț, prin rezolvarea unor probleme care pun bețe-n roate afacerilor și care nu se datorează mediului de afaceri, ci unei legislații care se schimbă mult prea des, fără motivații economice, fără dezbateri reale și care duce, de cele mai multe ori, la blocaje.
Problemele sunt deja cunoscute, dar reamintirea lor este necesară cu fiecare prilej, pentru că, poate, în felul acesta, oamenii din legislativ vor deveni conștienți de importanța activității lor și de faptul că, fără firme potente, fără contribuțiile plătite de acestea și de angajații lor, nu vor fi bani la buget, indiferent ce taxe și impozite vor apărea, indiferent de mărimea accizelor. Trebuie să înțeleagă toată lumea că 10-15 bani pe litrul de combustibil nu înseamnă doar mai mulți bani pe care-i dă șoferul la pompă, înseamnă scumpiri pe orizontală și verticală, crește prețul transportului, al alimentelor de bază, etc. Oamenii de afaceri români nu doresc lucruri ieșite din comun. Doresc doar predictibilitate, așa cum se întâmplă în toate țările civilizate, doresc o infrastructură competitivă, care să permită cooperarea între toate zonele țării, să permită tranzitul internațional, care aduce venituri consistente bugetului național. Doresc un tratament egal cu marii jucători internaționali din economia românească și doresc să-și poată vinde produsele pe cât mai multe piețe din lume, iar toate acestea se pot face printr-o politică fermă a statului român.
Camera de Comerț și Industrie a României, prin echipa condusă de președintele Mihai Daraban, face eforturi considerabile de a promova produsele românești pe piețe noi, printr-o diplomație de ordin economic, care începe să dea roade. Au fost create baze de date, s-au stabilit relații, lucrurile se dezvoltă în continuare, mai rămâne ca toate acestea să fie valorificate atât de cei care conduc România, cât și de firmele care doresc să intre pe aceste piețe.
– Încercăm o concluzie?
Județul Neamț are potențial, are antreprenori bine pregătiți,. Mai are nevoie de infrastructură și de infuzie de capital, care este binevenit de oriunde ar veni – de la statul român, prin programele guvernamentale, prin proiecte europene sau din partea unor investitori privați. Camera de Comerț și Industrie Neamț încearcă să rămână același instrument care să faciliteze dialogul dintre autorități și mediul de afaceri, care să organizeze târguri și dezbateri specifice, să pună la dispoziție toate cele necesare pentru a porni o afacere, apoi pentru a o extinde. M-au bucurat mult aprecierile la adresa activității Camerei de Comerț venite din partea domnului Ștefan Vuza, un om de afaceri tânăr, dar care are un merit deosebit în salvarea unor mari unități industriale românești, care a pornit din Neamț și care, acum, dezvoltă acasă o activitate cu un potențial imens, valorificând o tradiție a zonei. Sper ca exemplul său să fie urmat și de alți jucători economici naționali, care au plecat cu afacerile din județ și acum reprezintă repere naționale.
* Partenerii Camerei de Comerț și Industrie Neamț în organizarea manifestării
Jora Service SRL, Grupul de firme TCE 3 Brazi SA, Comes SA, Rifil SA, Marsat SA, ADF Prod SRL, RTV Neamț SRL, Bico Industries SRL, Panauto SRL, Expo Carm SRL, Banca Transilvania, BRD Groupe Société Générale, Banca Comercială Română.
* Momentul Ștefan Vuza
Ștefan Vuza
Ca semnificație, un moment deosebit l-a reprezentat scurtul discurs ținut de Ștefan Vuza, președintele grupului de firme Societățile Comerciale Române, bucuros că a fost invitat la gală pentru a-i fi înmânat premiul, dar și un pic necăjit că se întâmplă asta după 20 de ani. Rămâne de reținut îndemnul spre curaj al unui om care a îndrăznit și a reușit:
”Buna seara și doresc să mulțumesc pentru premiu și pentru invitație! Grupul SCR își are sediul, într-adevăr, în județul Neamț. N-am mai fost invitat de 20 de ani de Camera de Comerț și Industrie Neamț. Este o firmă puțin mai mare, ca și cifră de afaceri a depășit 1 miliard de euro. Avem 19 fabrici. Într-adevăr, suntem în activi în industrie, dar, pentru prima dată, primim premiu în agricultură și, pentru că în judetul Neamț nu am mai dezvoltat anumite domenii, pentru că Grupul SCR are deja 25 de ani de activitate, ne-am gândit să reinvestim într-un domeniu nou. E vorba de piscicultură. Ne-am alocat 2 ani de zile să studiem ce fac ceilalți jucători din domeniu. Am descoperit că sunt 42 de jucători în țară. Afacerile lor erau mai mici, afaceri de familie. Am mers în alte țări și am văzut ce trebuie să facem în domeniul piscicol, cum ar trebui să ne dezvoltăm. Așa am creat această păstrăvărie pe lacul Bicaz. Este o păstravărie de 2.000 tone și speram cu toții să fructificăm cât mai bine resursele județului Neamț, care are cu ce se mândri. Aici avem și noi societatea-mamă, cu toate că eu locuiesc la Cluj și la București.
Cred că este bine să îndrăznim mai mult. Să îndrăzniți și voi mai mult, pentru că lucrurile nu sunt greu de făcut! Doar să te pui în poziția de a le face este mai greu.
Vă mulțumesc și vă doresc o seară frumoasă!”.
GALERIE FOTO:
#gallery-0-4 { margin: auto; } #gallery-0-4 .gallery-item { float: left; margin-top: 10px; text-align: center; width: 25%; } #gallery-0-4 img { border: 2px solid #cfcfcf; } #gallery-0-4 .gallery-caption { margin-left: 0; } /* see gallery_shortcode() in wp-includes/media.php */
Mihai Apopii: ”Sunt mândru de firmele prezente în Topul Firmelor Nemțene” Manifestare anuală, Topul Firmelor Nemțene rămâne cel mai amplu eveniment prin care managerii de elită ai județului sunt recompensați, simbolic, pentru activitatea lor, prilej pentru schimburi de idei și experiență, pentru cunoaștere reciprocă și, nu în ultimul rând, pentru a se simți bine în cadrul unei festivități care le este, exclusiv, destinată și dedicată.
#ADF Prod SRL#Auto Moldova SA#Bico Industries SRL#Camera de Comerț și Industrie Neamț#Caremil SRL#Centrul de cercetare și prelucrare a plantelor medicinale Plantavorel SA#Comes SA#Dinamic 92 Distribution SRL#Enzymes & Derivates SA#Grupul de firme TCE 3 Brazi SA#International Private Security SRL#Kober SRL#Marsat SA#Mecanica Ceahlău SA#Metex SA#MiHai Apopii#Moldocor SA#Panauto SRL#Petrocart SA#Rifil SA#Smirodava SA#stefan vuza#Tehnoprod SRL#topul firmelor nemtene 2016
0 notes
Text
Antibiotics produced by Bacteria activate Human Oocytes, creating Healthy Babies: AMPK links the Creation of Human Life with HIV, Progeria, & Cancer
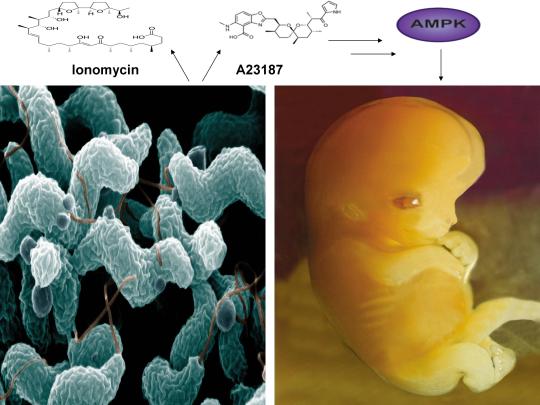
CC-BY-SA-3.0 (http://creativecommons.org/licenses/by-sa/3.0/)], via Wikimedia Commons;By De Wood, Pooley, USDA, ARS, EMU. [Public domain], via Wikimedia Commons
A recent study published online in the journal Fertility and Sterility in September of 2017 systematically reviewed for the first time evidence for the effect of two compounds, ionomycin and A23187 (also known as calcimycin), on fertilization rates and pregnancy outcomes in infertile couples undergoing an in vitro fertilization procedure known as intracytoplasmic sperm injection (ICSI) [1]. ICSI involves the direct deposition of sperm into the oocyte cytoplasm, which typically leads to high rates of fertilization. However, fertilization failure despite repeated ICSI is likely caused by a failure of the oocyte to activate [1]. Physiological oocyte activation is accomplished by the delivery of a sperm-borne oocyte activating factor called phospholipase C zeta (PLCζ). PLCζ activates human oocytes by inducing an intracellular signaling cascade that ultimately results in increased calcium (Ca2+) oscillations in the oocyte, which drives oocyte activation to completion [1]. As oocyte activation is an indispensable prerequisite for the creation of all human life, every human being alive today and any human being that has ever lived began their existence as an activated oocyte [2]. Ionomycin and A23187 increase the levels of intracellular Ca2+ and are thus commonly known as Ca2+ ionophores [1]. The authors of the Fertility and Sterility study showed that over a total of 1,521 ICSI cycles, calcium ionophores including ionomycin and A23187 led to a statistically significant improvement in fertilization, cleavage, blastulation, implantation rates, overall pregnancy, and live-birth rates [1]. Ionomycin and A23187 have also been shown in several independent studies to effectively induce human oocyte activation, leading to the birth of normal, healthy children [3,4].
Strikingly, as described further below, both ionomycin and A23187 are antibiotics that are naturally produced by certain species within the bacterial genus Streptomyces [5,6]. Other structurally distinct compounds and methods have also been shown to induce human oocyte activation, including ethanol, puromycin (an antibiotic and protein synthesis inhibitor produced by Streptomyces alboniger), as well as mechanical manipulation and electrical stimulation, both of with have been reported to result in the creation of normal children [7-11]. Interestingly, as mouse oocytes are considered models for human oocytes, ionomycin, A23187, anisomycin (an antibiotic and protein synthesis inhibitor produced by Streptomyces griseolus), mycophenolic acid (an immunosuppressant produced by the fungus Penicillium brevicompactum), cycloheximide (a protein synthesis inhibitor produced by Streptomyces griseus), carvacrol (a secondary plant metabolite produced by Origanum vulgare{oregano}), and phorbol 12-myristate 13-acetate (PMA, a secondary plant metabolite produced by Croton tiglium) each induce activation of mouse oocytes [12-22]. Ionomycin, A23187, PMA, and reactive oxygen species (ROS) also induce the acrosome reaction in human sperm, a process characterized by the release of hydrolytic enzymes from the head of sperm which is necessary for oocyte penetration and thus indispensable for the creation of all human life outside of a clinical setting (ICSI bypasses the need for oocyte penetration) [23,24]. Additionally, although an over-production of ROS, similar to Ca2+, may lead to deleterious effects including cell death/apoptosis, low levels of ROS have been shown to act as signaling molecules and ROS is significantly increased on or immediately following mouse oocyte activation [25,26].
Furthermore, the master metabolic regulator AMPK is critical for oocyte meiotic resumption and maturation (a process that precedes and is essential for oocyte activation), is found located across the entire acrosome in the head of human sperm, and is activated by increases in ROS and Ca2+ [27-29]. Ionomycin, A23187, ethanol, puromycin, mechanical force, electrical stimulation, anisomycin, mycophenolic acid, carvacrol, and PMA also induce AMPK activation, indicating that a common mechanism of action links chemically distinct compounds with the creation of human life [30-39]. This common mechanism of action likely centers on the induction of cellular stress, mediated by indirect increases in intracellular Ca2+, ROS, and/or the AMP(ADP)/ATP ratio, etc. as I originally proposed in 2016 [40]. Because the bacterial-derived antibiotics ionomycin and A23187 induce both the acrosome reaction in human sperm and human oocyte activation, producing normal, healthy children, it can be said that “non-human organisms have the power to create human life or the power to end life.” As explained below, the beneficial effects of cellular stress induction (i.e. a “shock”) crosses species boundaries and may indeed play a role in facilitating natural selection, a process that underlies and drives evolution.
A number of bacterial species residing within the genus Streptomyces have proven to be extremely important and medicinally valuable as approximately 70% of clinically useful antibiotics are derived from Streptomyces [41]. The antibiotics ionomycin and A23187 are naturally produced by Streptomyces conglobatus and Streptomyces chartreusensis, respectively [5,6]. Other important examples include the antibiotic tetracycline (produced by Streptomyces aureofaciens), the immunosuppressant rapamycin (produced by Streptomyces hygroscopicus), and the anti-helminthic avermectins (produced by Streptomyces avermitilis) [42]. Many soil and aquatic-dwelling species of Streptomyces can be found in harsh environments and are characterized by a unique life cycle, including spore germination followed by vegetative mycelium production, aerial hyphae formation, sporulation (i.e. spore formation), and antibiotic production [43,44]. Curiously, just as cellular stress induction leads to the creation of human life and other beneficial effects in human cells (see below), stress induction also promotes the induction of aerial hyphae formation, sporulation, and antibiotic production in many Streptomyces species (spp.). Indeed, a decrease in the levels of ATP and bacterial growth is associated with sporulation, aerial hyphae formation, and antibiotic production [42,45]. A reduction in glucose/nutritional deprivation, the preferred sugar/carbon source for many Streptomyces spp., also significantly increases antibiotic production [46]. An increase in intracellular ROS and Ca2+ is associated with spore germination, aerial hyphae formation, and antibiotic production [47-49]. Other cellular stressors, including heat shock and ethanol, also significantly increase antibiotic production, provocatively indicating that the effects of cellular stress crosses species boundaries, enhancing bacterial survival and facilitating the creation of human life [50,51].
The beneficial effects of low-level cellular stress induction also extends to plants, as many plants produce secondary metabolites partly for the purpose of self-defense, analogous to antibiotics. Similar to the harsh, stressful environments often inhabited by Streptomyces spp., the Great Basin Bristlecone Pine (Pinus Longaeva), considered the oldest living non-clonal organism on the planet ( >5000 years old), thrives in an exceptionally harsh environment, characterized by increased elevations and exposure to UV radiation, nutritionally-deprived soils, harsh temperatures, and mechanical stress due to wind variances, leading early researchers to conclude that it’s longevity is intimately associated with adversity [52-54]. Conversely, Pinus Longaeva species that are located in less stressful environments (i.e. lower elevations) are strongly associated with younger age classes (<875 years) [55]. Similarly, the Creosote bush (Larrea tridentate), considered one of the oldest living clonal organisms on the planet (>11,000 years old), also thrives in harsh environments including the Mohave Desert [56]. AMPK, which increases lifespan and healthspan in several model organisms, is the primary sensor of cellular stress in eukaryotic organisms (e.g. plants and humans) and the plant AMPK orthologue SnRK1 as well as Ca2+ and ROS are critical for seed germination, fertilization, root gravitropism, and secondary metabolite production [57-64]. The secondary plant metabolites PMA (which activates mouse oocytes and promotes the acrosome reaction in human sperm) and artemisinin (an anti-malarial drug) both activate AMPK and the antibiotic A23187 also increases production of the secondary metabolite resveratrol in grape cell cultures, again indicating that exposure to low-level stressors may promote extension of lifespan and initiate the creation of human life [17,23,39,65,66].
Organismal exposure to beneficial levels of stress may also play a critical role in evolution. As first noted by Charles Darwin, evolution is driven by natural selection, a process characterized by environmentally-induced phenotypic changes that may lead to inheritable survival and reproductive advantages [67]. From “On the Origin of Species by Means of Natural Selection, or the Preservation of Favoured Races in the Struggle for Life”, Darwin explained that “if there be, owing to the high geometrical powers of increase of each species, at some age, season, or year, a severe struggle for life, and this certainly cannot be disputed;��…But if variations useful to any organic being do occur, assuredly individuals thus characterised will have the best chance of being preserved in the struggle for life;” [67]. This “struggle for life” Darwin spoke of is embodied by selective pressures which may be abiotic (i.e. light, wind, temperature, etc.) or biotic (predation, disease, competition, etc.) [68,69]. As alluded to above, such selective pressures are indeed sources of cellular stress, sensed by both prokaryotes and eukaryotes, that induce beneficial responses (at appropriate levels), leading to the acquisition of phenotypes conducive for continued survival. Both biotic (e.g. infection) and abiotic (e.g. heat) stressors/selective pressures activate AMPK (which is evolutionarily conserved among eukaryotes) in human cells [70,71]. A phenomenon often cited as an example of natural selection on a readily observable timescale is the development of bacterial resistance to antibiotics, resulting in problematic mutant strains that may be life-threatening for some individuals (i.e. the elderly and immunocompromised) [72]. Intriguingly, lethal levels of bactericidal antibiotics have been shown to kill microorganisms via the induction of ROS, sub-lethal levels of bactericidal antibiotics however increase mutagenesis and bacterial resistance via induction of lower levels of ROS, and heat as well as nutritional stress increase bacterial resistance to antibiotics, providing compelling evidence that continuous exposure to low levels of stress likely plays a significant role in natural selection and evolution [73-75].
Moreover, gravity itself likely functions as a cellular stressor/selective pressure that has influenced the development of organisms on Earth since the emergence of the very first lifeform. Gravity exerts its effects on living organisms via the application of force, which is experienced by human cells in the form of mechanical loading or stress [76]. The application of force or a mechanical load has recently been shown to activate AMPK and simulated microgravity (i.e. hind limb unloading in mice) significantly decreases AMPK activation [77,78]. Spaceflight also inhibits the activation of T cells (immune cells essential for adaptive immunity), whereas the application of force and AMPK activation promote T cell activation [79-81]. Interestingly, spaceflight has recently been shown to decrease the levels of the master antioxidant transcription factor Nrf2 and the heat shock-inducible protein HSP90a but increase the levels of the growth-promoting kinase mTOR in mice [82]. AMPK however inhibits mTOR but increases the phosphorylation, nuclear retention, and transcriptional activity of Nrf2 [57,83,84]. Also, HSP90 interacts with and maintains AMPK activity and HSP90 is necessary for progesterone-induced human sperm acrosome reaction [85,86]. Interestingly, rapamycin, an immunosuppressant produced by Streptomyces hygroscopicus, extends lifespan in genetically heterogeneous mice, activates AMPK in vivo in normal aged mice, and increases human sperm motility [42,87,88]. Simulated microgravity via the use of NASA-designed rotating wall vessels (RWVs) however drastically reduces rapamycin production (~90%) whereas the antibiotic gentamycin increases rapamycin production by Streptomyces hygroscopicus, providing further evidence that cellular stress, in the form of mechanical loading induced by gravity, is essential for development, function, and survival of Earth-bound organisms [89,90].
The induction of cellular stress also links seemingly dissimilar physiological and pathological states with the activation of AMPK. As discussed above, both ionomycin and ROS activate AMPK and promote oocyte meiotic resumption, a process that is AMPK-dependent and is essential for efficient oocyte activation [27,30,91]. ROS is also critical for ovulation, PMA and ionomycin both activate mouse oocytes, and ionomycin is extensively used during ICSI procedures, creating normal healthy children, suggesting that cellular stress-induced AMPK activation is also essential for oocyte activation [3,4,12,17,92]. The activation of oocytes and T cells share strikingly similar intracellular signaling mechanisms (e.g. PLC-PIP2-DAG-PKC-IP3-Ca2+) and ionomycin combined with PMA are extremely effective in activating T cells and are often used as positive controls in HIV-1 latency reversal studies [93-95]. Reactivating latent/dormant HIV-1 in CD4+ T cells, potentially facilitating immune system detection and virus destruction, is currently being pursued as a method for the potential eradication of HIV-1 (called the “shock and kill” approach) [96]. Similar to oocyte activation, both Ca2+ and ROS are critical for T cell activation (and hence latent HIV-1 reactivation) and other cellular stress-inducing compounds, including NDGA derived from the Creosote bush, butyrate derived from bacteria, as well as ROS and HSP90 have been shown to reactivate latent HIV-1 [26,93,94,97-101]. Interestingly, AMPK inhibition leads to cell death on T cell activation, knockdown of AMPK significantly decreases HIV-1 replication, and metformin (a well-studied AMPK activator derived from the French Lilac plant) increases butyrate production in human diabetic patients [81,102,103]. Perhaps most convincingly, early preliminary data showed that metformin significantly reduced several markers preferentially associated with cells latently infected with HIV-1 (e.g. PD-1, TIGIT, TIM-3) and also destabilized the latent HIV-1 reservoir in chronically-infected HIV patients, indicating that cellular-stress induced AMPK activation likely links the creation of human life with the potential eradication of HIV-1, as I originally proposed in 2016 [40,104,105].
AMPK activation may also link the disparate disease states of HIV-1 latency and Hutchinson-Gilford progeria syndrome (HGPS). HGPS is a genetic disorder caused by aberrant alternative splicing of the LMNA gene, generating a toxic protein called progerin that induces an accelerated aging phenotype and premature death at approximately 14 years of age [106]. Excessive activity of the gene splicing factor SRSF1 has been shown to prevent reactivation of latent HIV-1 and contribute to aberrant splicing of the LMNA gene in HGPS [107-109]. Metformin however has recently been shown to ameliorate the accelerated aging phenotype in cells derived from children with HGPS by reducing the levels of both SRSF1 and progerin and activating AMPK, as I first proposed in 2014 [110-112]. Interestingly, both Ca2+ and ROS induce autophagy (a process of disposing of damaged/toxic proteins and organelles) and rapamycin, which activates AMPK in vivo and increases intracellular Ca2+ levels, improves accelerated aging in progeria cells by inducing autophagic degradation of progerin [87,113-116]. Temsirolimus, an analog of rapamycin, also alleviated accelerated aging defects in progeria cells but also increased the levels of ROS and superoxide within the first hour of treatment [117]. Such evidence strongly suggests that cellular stress-induced AMPK activation links the reversal of HIV-1 latency and alleviation of accelerated cellular aging defects in HGPS.
Cellular stress-induced AMPK activation also links the potential elimination of cancer stem cells (CSCs) with HIV-1 latency reversal and viral eradication. CSCs, which are largely resistant to chemoradiation therapy, are a subpopulation of cancer cells that exhibit characteristics similar to embryonic stem cells (ESCs), including self-renewal, multi-lineage differentiation, & the ability to initiate tumorigenesis [118,119]. Mechanisms that sustain quiescence & promote self-renewal in adult stem cells (ASCs) & CSCs likely also function to maintain latency of HIV-1 in CD4+ memory T cells. Indeed, HIV-1 has been found to establish long-lasting latency in a recently discovered subset of CD4+ T cells that exhibit stem cell-like properties known as T memory stem (TSCM) cells and increases in Ca2+, ROS, and AMPK activation have been shown to promote T cell activation and ESC, ASC, and CSC differentiation [119,120]. Additionally, A23187 and PMA have been shown to promote CSC differentiation (causing CSCs to become more susceptible to chemoradiation) and metformin induces CSC differentiation and/or apoptosis in an AMPK-dependent manner in the deadliest of cancers, including glioblastoma and pancreatic cancer, providing support for my publication in 2017 in which I first proposed that CSC differentiation and/or apoptosis and HIV-1 latency reversal/viral eradication may be linked by cellular stress-induced AMPK activation [119,121-124].
In conclusion, the ability of non-human organisms including certain Streptomyces spp. to initiate the creation of human life is predicated on the induction of cellular stress, mediated by increases in intracellular ROS, Ca2+, AMP(ADP)/ATP ratio increase, etc. The beneficial effects of transient cellular stress induction, which may be likened to selective pressures, crosses species boundaries and may indeed play a role in facilitating natural selection, a process that underlies and drives evolution, as evidenced by stress-induced increases in antibiotic production by Streptomyces spp. and stress-induced mutagenesis and antibiotic resistance in various bacterial strains. Because AMPK, a primary sensor of cellular stress in eukaryotic cells that increases lifespan and healthspan, plays a critical role in oocyte meiotic resumption/maturation, T cell activation, and stem cell differentiation, the creation of human life, the potential eradication of HIV-1, amelioration of accelerated aging in HGPS cells, and CSC differentiation/apoptosis are likely linked by a “Shock to Live”, or a “Shock to Kill”.
https://www.linkedin.com/pulse/antibiotics-produced-bacteria-activate-human-oocytes-creating-finley/
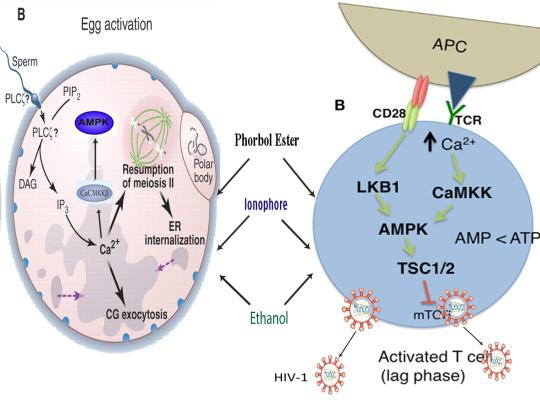
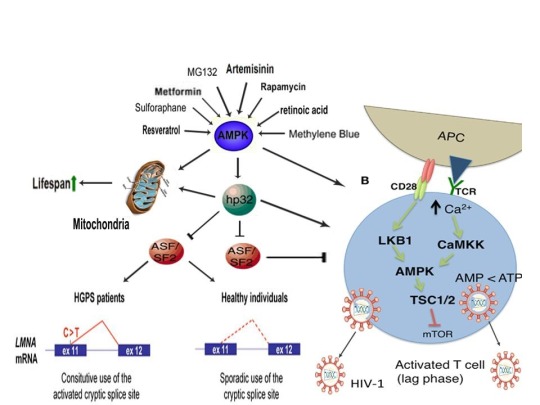
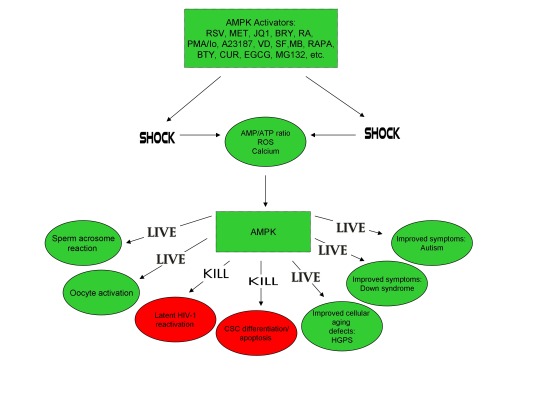
References:
Murugesu S, Saso S, Jones BP, et al. Does the use of calcium ionophore during artificial oocyte activation demonstrate an effect on pregnancy rate? A meta-analysis. Fertil Steril. 2017 Sep;108(3):468-482.e3.
Tesarik J, Sousa M, Testart J. Human oocyte activation after intracytoplasmic sperm injection. Hum Reprod. 1994 Mar;9(3):511-8.
Deemeh MR, Tavalaee M, Nasr-Esfahani MH. Health of children born through artificial oocyte activation: a pilot study. Reprod Sci. 2015 Mar;22(3):322-8.
Eftekhar M, Janati S, Rahsepar M, Aflatoonian A. Effect of oocyte activation with calcium ionophore on ICSI outcomes in teratospermia: A randomized clinical trial. Iran J Reprod Med. 2013 Nov;11(11):875-82.
Liu WC, Slusarchyk DS, Astle G, Trejo WH, Brown WE, Meyers E. Ionomycin, a new polyether antibiotic. J Antibiot (Tokyo). 1978 Sep;31(9):815-9.
Reed PW, Lardy HA. A23187: a divalent cation ionophore. J Biol Chem. 1972 Nov 10;247(21):6970-7.
Zhang Z, Wang T, Hao Y, et al. Effects of trehalose vitrification and artificial oocyte activation on the development competence of human immature oocytes. Cryobiology. 2017 Feb;74:43-49.
De Sutter P, Dozortsev D, Cieslak J, Wolf G, Verlinsky Y, Dyban A. Parthenogenetic activation of human oocytes by puromycin. J Assist Reprod Genet. 1992 Aug;9(4):328-37.
Sankaran L, Pogell BM. Biosynthesis of puromycin in Streptomyces alboniger: regulation and properties of O-demethylpuromycin O-methyltransferase. Antimicrob Agents Chemother. 1975 Dec;8(6):721-32.
Tesarik J, Rienzi L, Ubaldi F, Mendoza C, Greco E. Use of a modified intracytoplasmic sperm injection technique to overcome sperm-borne and oocyte-borne oocyte activation failures. Fertil Steril. 2002 Sep;78(3):619-24.
Mansour R, Fahmy I, Tawab NA, et al. Electrical activation of oocytes after intracytoplasmic sperm injection: a controlled randomized study. Fertil Steril. 2009 Jan;91(1):133-9.
Nikiforaki D, Vanden Meerschaut F, de Roo C, et al. Effect of two assisted oocyte activation protocols used to overcome fertilization failure on the activation potential and calcium releasing pattern. Fertil Steril. 2016 Mar;105(3):798-806.e2.
Liu H1, Zhang J, Krey LC, Grifo JA. In-vitro development of mouse zygotes following reconstruction by sequential transfer of germinal vesicles and haploid pronuclei. Hum Reprod. 2000 Sep;15(9):1997-2002.
Downs SM. Stimulation of parthenogenesis in mouse ovarian follicles by inhibitors of inosine monophosphate dehydrogenase. Biol Reprod. 1990 Sep;43(3):427-36.
Siracusa G, Whittingham DG, Molinaro M, Vivarelli E. Parthenogenetic activation of mouse oocytes induced by inhibitors of protein synthesis. J Embryol Exp Morphol. 1978 Feb;43:157-66.
Carvacho I, Lee HC, Fissore RA, Clapham DE. TRPV3 channels mediate strontium-induced mouse-egg activation. Cell Rep. 2013 Dec 12;5(5):1375-86.
Colonna R, Tatone C, Malgaroli A, Eusebi F, Mangia F. Effects of protein kinase C stimulation and free Ca2+ rise in mammalian egg activation. Gamete Res. 1989 Oct;24(2):171-83.
Tang Z, Xing F, Chen D, et al. In vivo toxicological evaluation of Anisomycin. Toxicol Lett. 2012 Jan 5;208(1):1-11.
Regueira TB, Kildegaard KR, Hansen BG, Mortensen UH, Hertweck C, Nielsen J. Molecular basis for mycophenolic acid biosynthesis in Penicillium brevicompactum. Appl Environ Microbiol. 2011 May;77(9):3035-43.
K'ominek LA. Cycloheximide production by Streptomyces griseus: control mechanisms of cycloheximide biosynthesis. Antimicrob Agents Chemother. 1975 Jun;7(6):856-6.
Ultee A, Slump RA, Steging G, Smid EJ. Antimicrobial activity of carvacrol toward Bacillus cereus on rice. J Food Prot. 2000 May;63(5):620-4.
Pal PK, Nandi MK, Singh NK. Detoxification of Croton tiglium L. seeds by Ayurvedic process of Śodhana. Anc Sci Life. 2014 Jan;33(3):157-61.
Rotem R, Paz GF, Homonnai ZT, et al. Ca(2+)-independent induction of acrosome reaction by protein kinase C in human sperm. Endocrinology. 1992 Nov;131(5):2235-43.
de Lamirande E, Tsai C, Harakat A, Gagnon C. Involvement of reactive oxygen species in human sperm arcosome reaction induced by A23187, lysophosphatidylcholine, and biological fluid ultrafiltrates. J Androl. 1998 Sep-Oct;19(5):585-94.
Görlach A, Bertram K, Hudecova S, Krizanova O. Calcium and ROS: A mutual interplay. Redox Biol. 2015 Dec;6:260-71.
Nasr-Esfahani MM, Johnson MH. The origin of reactive oxygen species in mouse embryos cultured in vitro. Development. 1991 Oct;113(2):551-60.
LaRosa C, Downs SM. Stress stimulates AMP-activated protein kinase and meiotic resumption in mouse oocytes. Biol Reprod. 2006 Mar;74(3):585-92.
Calle-Guisado V, de Llera AH, Martin-Hidalgo D, et al. AMP-activated kinase in human spermatozoa: identification, intracellular localization, and key function in the regulation of sperm motility. Asian J Androl. 2016 Sep 27.
Mungai PT, Waypa GB, Jairaman A, et al. Hypoxia triggers AMPK activation through reactive oxygen species-mediated activation of calcium release-activated calcium channels. Mol Cell Biol. 2011 Sep;31(17):3531-45.
Tamas P, Hawley SA, Clarke RG, et al. Regulation of the energy sensor AMP-activated protein kinase by antigen receptor and Ca2+ in T lymphocytes. J Exp Med 2006;203(7):1665–70.
Hawley SA, Pan DA, Mustard KJ, et al. Calmodulin-dependent protein kinase kinase-beta is an alternative upstream kinase for AMP-activated protein kinase. Cell Metab. 2005 Jul;2(1):9-19.
Nammi S, Roufogalis BD. Light-to-moderate ethanol feeding augments AMPK-α phosphorylation and attenuates SREBP-1 expression in the liver of rats. J Pharm Pharm Sci. 2013;16(2):342-51.
Koh W, Jeong SJ, Lee HJ, et al. Melatonin promotes puromycin-induced apoptosis with activation of caspase-3 and 5'-adenosine monophosphate-activated kinase-alpha in human leukemia HL-60 cells. J Pineal Res. 2011 May;50(4):367-73.
Bays JL, Campbell HK, Heidema C, Sebbagh M, DeMali KA. Linking E-cadherin mechanotransduction to cell metabolism through force-mediated activation of AMPK. Nat Cell Biol. 2017 Jun;19(6):724-731.
Hutber CA, Hardie DG, Winder WW. Electrical stimulation inactivates muscle acetyl-CoA carboxylase and increases AMP-activated protein kinase. Am J Physiol. 1997 Feb;272(2 Pt 1):E262-6.
Ohsaka Y, Nishino H, Nomura Y. Induction of phospho-Thr-172 AMPK in 3T3-L1 adipocytes exposed to cold or treated with anisomycin, mithramycin A, and ionic compounds. Cryo Letters. 2010 May-Jun;31(3):218-29.
Fernández-Ramos AA, Marchetti-Laurent C, Poindessous V, et al. A comprehensive characterization of the impact of mycophenolic acid on the metabolism of Jurkat T cells. Sci Rep. 2017 Sep 5;7(1):10550.
Kim E, Choi Y, Jang J, Park T. Carvacrol Protects against Hepatic Steatosis in Mice Fed a High-Fat Diet by Enhancing SIRT1-AMPK Signaling. Evid Based Complement Alternat Med. 2013;2013:290104.
Zogovic N, Tovilovic-Kovacevic G, Misirkic-Marjanovic M, et al. Coordinated activation of AMP-activated protein kinase, extracellular signal-regulated kinase, and autophagy regulates phorbol myristate acetate-induced differentiation of SH-SY5Y neuroblastoma cells. J Neurochem. 2015 Apr;133(2):223-32.
Finley J. Oocyte activation and latent HIV-1 reactivation: AMPK as a common mechanism of action linking the beginnings of life and the potential eradication of HIV-1. Med Hypotheses. 2016 Aug;93:34-47.
Kitani S1, Miyamoto KT, Takamatsu S, et al. Avenolide, a Streptomyces hormone controlling antibiotic production in Streptomyces avermitilis. Proc Natl Acad Sci U S A. 2011 Sep 27;108(39):16410-5.
Challis GL, Hopwood DA. Synergy and contingency as driving forces for the evolution of multiple secondary metabolite production by Streptomyces species. Proc Natl Acad Sci U S A. 2003 Nov 25;100 Suppl 2:14555-61.
Yagüe P, López-García MT, Rioseras B, Sánchez J, Manteca A. Pre-sporulation stages of Streptomyces differentiation: state-of-the-art and future perspectives. FEMS Microbiol Lett. 2013 May;342(2):79-88.
Seipke RF, Kaltenpoth M, Hutchings MI. Streptomyces as symbionts: an emerging and widespread theme? FEMS Microbiol Rev. 2012 Jul;36(4):862-76.
Meng L, Li M, Yang SH, Kim TJ, Suh JW. Intracellular ATP levels affect secondary metabolite production in Streptomyces spp. Biosci Biotechnol Biochem. 2011;75(8):1576-81.
Sánchez S, Chávez A, Forero A, et al. Carbon source regulation of antibiotic production. J Antibiot (Tokyo). 2010 Aug;63(8):442-59.
Wang SL, Fan KQ, Yang X, Lin ZX, Xu XP, Yang KQ. CabC, an EF-hand calcium-binding protein, is involved in Ca2+-mediated regulation of spore germination and aerial hypha formation in Streptomyces coelicolor. J Bacteriol. 2008 Jun;190(11):4061-8.
Wang D, Wei L, Zhang Y, Zhang M, Gu S. Physicochemical and microbial responses of Streptomyces natalensis HW-2 to fungal elicitor. Appl Microbiol Biotechnol. 2017 Jul 28. doi: 10.1007/s00253-017-8440-0. [Epub ahead of print].
Wei ZH, Bai L, Deng Z, Zhong JJ. Enhanced production of validamycin A by H2O2-induced reactive oxygen species in fermentation of Streptomyces hygroscopicus 5008. Bioresour Technol. 2011 Jan;102(2):1783-7.
Doull JL, Ayer SW, Singh AK, Thibault P. Production of a novel polyketide antibiotic, jadomycin B, by Streptomyces venezuelae following heat shock. J Antibiot (Tokyo). 1993 May;46(5):869-71.
Zhou WW1, Ma B, Tang YJ, Zhong JJ, Zheng X. Enhancement of validamycin A production by addition of ethanol in fermentation of Streptomyces hygroscopicus 5008. Bioresour Technol. 2012 Jun;114:616-21.
Flanary BE, Kletetschka G. Analysis of telomere length and telomerase activity in tree species of various lifespans, and with age in the bristlecone pine Pinus longaeva. Rejuvenation Res. 2006 Spring;9(1):61-3.
R. S. BeasleyJ. O. Klemmedson. Recognizing site adversity and drought-sensitive trees in stands of bristlecone pine (Pinus longaeva). January 1973, Volume 27, Issue 1, pp 141–146. doi: 10.1007/BF02862228.
Schulman E. Longevity under Adversity in Conifers. Science. 1954 Mar 26;119(3091):396-9.
Hiebert, R. D.; Hamrick, J. L. 1984. An ecological study of bristlecone pine (Pinus longaeva) in Utah and eastern Nevada. The Great Basin Naturalist. 44(3): 487-494.
Jorquera MA, Shaharoona B, Nadeem SM, de la Luz Mora M, Crowley DE. Plant growth-promoting rhizobacteria associated with ancient clones of creosote bush (Larrea tridentata). Microb Ecol. 2012 Nov;64(4):1008-17.
Salminen A, Kaarniranta K. AMP-activated protein kinase (AMPK) controls the aging process via an integrated signaling network. Ageing Res Rev. 2012 Apr;11(2):230-41.
Leymarie J, Vitkauskaité G, Hoang HH, et al. Role of reactive oxygen species in the regulation of Arabidopsis seed dormancy. Plant Cell Physiol. 2012 Jan;53(1):96-106.
Pang X, Halaly T, Crane O, et al. Involvement of calcium signalling in dormancy release of grape buds. J Exp Bot. 2007;58(12):3249-62.
Duan Q, Kita D, Johnson EA, et al. Reactive oxygen species mediate pollen tube rupture to release sperm for fertilization in Arabidopsis. Nat Commun. 2014;5:3129.
Gao XQ, Liu CZ, Li DD, et al. The Arabidopsis KINβγ Subunit of the SnRK1 Complex Regulates Pollen Hydration on the Stigma by Mediating the Level of Reactive Oxygen Species in Pollen. PLoS Genet. 2016 Jul 29;12(7):e1006228.
Joo JH, Bae YS, Lee JS. Role of auxin-induced reactive oxygen species in root gravitropism. Plant Physiol. 2001 Jul;126(3):1055-60.
Lee JS, Mulkey TJ, Evans ML. Reversible loss of gravitropic sensitivity in maize roots after tip application of calcium chelators. Science. 1983 Jun 24;220(4604):1375-6.
Zhao J, Davis LC, Verpoorte R. Elicitor signal transduction leading to production of plant secondary metabolites. Biotechnol Adv. 2005 Jun;23(4):283-333.
Wang H, Sharma L, Lu J, Finch P, Fletcher S, Prochownik EV. Structurally diverse c-Myc inhibitors share a common mechanism of action involving ATP depletion. Oncotarget. 2015 Jun 30;6(18):15857-70.
K.V. Kiselev, O.A. Shumakova, A.Y. Manyakhin, A.N. Mazeika. Influence of calcium influx induced by the calcium ionophore, A23187, on resveratrol content and the expression of CDPK and STS genes in the cell cultures of Vitis amurensis. Plant Growth Regulation. December 2012, Volume 68, Issue 3, pp 371–381. DOI: 10.1007/s10725-012-9725-z.
Darwin, Charles (1859). On the origin of species by means of natural selection, or the preservation of favoured races in the struggle for life. London: Murray. [1st ed.].
Sobral M, Veiga T, Domínguez P, Guitián JA, Guitián P, Guitián JM. Selective Pressures Explain Differences in Flower Color among Gentiana lutea Populations. PLoS One. 2015 Jul 14;10(7):e0132522.
Lefebvre V, Kiani SP, Durand-Tardif M. A focus on natural variation for abiotic constraints response in the model species Arabidopsis thaliana. Int J Mol Sci. 2009 Aug 13;10(8):3547-82.
Moser TS, Schieffer D, Cherry S. AMP-activated kinase restricts Rift Valley fever virus infection by inhibiting fatty acid synthesis. PLoS Pathog. 2012;8(4):e1002661.
Goto A, Egawa T, Sakon I, et al. Heat stress acutely activates insulin-independent glucose transport and 5'-AMP-activated protein kinase prior to an increase in HSP72 protein in rat skeletal muscle. Physiol Rep. 2015 Nov;3(11). pii: e12601.
Maclean RC, Hall AR, Perron GG, Buckling A. The evolution of antibiotic resistance: insight into the roles of molecular mechanisms of resistance and treatment context. Discov Med. 2010 Aug;10(51):112-8.
Dwyer DJ, Belenky PA, Yang JH, et al. Antibiotics induce redox-related physiological alterations as part of their lethality. Proc Natl Acad Sci U S A. 2014 May 20;111(20):E2100-9.
Kohanski MA, DePristo MA, Collins JJ. Sublethal antibiotic treatment leads to multidrug resistance via radical-induced mutagenesis. Mol Cell. 2010 Feb 12;37(3):311-20.
Poole K. Bacterial stress responses as determinants of antimicrobial resistance. J Antimicrob Chemother. 2012 Sep;67(9):2069-89.
Ito M, Arakawa T, Okayama M, Shitara A, Mizoguchi I, Takuma T. Gravity loading induces adenosine triphosphate release and phosphorylation of extracellular signal-regulated kinases in human periodontal ligament cells. J Investig Clin Dent. 2014 Nov;5(4):266-74.
Bays JL, Campbell HK, Heidema C, Sebbagh M, DeMali KA. Linking E-cadherin mechanotransduction to cell metabolism through force-mediated activation of AMPK. Nat Cell Biol. 2017 Jun;19(6):724-731.
Zhong G, Li Y, Li H, et al. Simulated Microgravity and Recovery-Induced Remodeling of the Left and Right Ventricle. Front Physiol. 2016 Jun 29;7:274.
Chang TT, Walther I, Li CF, et al. The Rel/NF-jB pathway and transcription of immediate early genes in T cell activation are inhibited by microgravity. J Leukoc Biol 2012;92(6):1133–45.
Li YC, Chen BM, Wu PC, et al. Cutting Edge: mechanical forces acting on T cells immobilized via the TCR complex can trigger TCR signaling. J Immunol 2010;184(11):5959–63.
Ouyang Z, Wang X, Meng Q, et al. Suppression of adenosine monophosphate-activated protein kinase selectively triggers apoptosis in activated T cells and ameliorates immune diseases. Biochem Biophys Res Commun. 2017 May 27;487(2):223-229.
Blaber EA, Pecaut MJ, Jonscher KR. Spaceflight Activates Autophagy Programs and the Proteasome in Mouse Liver. Int J Mol Sci. 2017 Sep 27;18(10). pii: E2062.
Mo C, Wang L, Zhang J, et al. The crosstalk between Nrf2 and AMPK signal pathways is important for the anti-inflammatory effect of berberine in LPS-stimulated macrophages and endotoxin-shocked mice. Antioxid Redox Signal. 2014 Feb 1;20(4):574-88.
Joo MS, Kim WD, Lee KY, Kim JH, Koo JH, Kim SG. AMPK facilitates nuclear accumulation of Nrf2 by phosphorylating at serine 550. Mol Cell Biol. 2016 Jun 29;36(14):1931-42.
Zhang L, Yi Y, Guo Q, et al. Hsp90 interacts with AMPK and mediates acetyl-CoA carboxylase phosphorylation. Cell Signal. 2012 Apr;24(4):859-65.
Sagare-Patil V, Bhilawadikar R, Galvankar M, Zaveri K, Hinduja I, Modi D. Progesterone requires heat shock protein 90 (HSP90) in human sperm to regulate motility and acrosome reaction. J Assist Reprod Genet. 2017 Apr;34(4):495-503.
Lesniewski LA, Seals DR4, Walker AE, et al. Dietary rapamycin supplementation reverses age-related vascular dysfunction and oxidative stress, while modulating nutrient-sensing, cell cycle, and senescence pathways. Aging Cell. 2017 Feb;16(1):17-26.
Aparicio IM, Espino J, Bejarano I, et al. Autophagy-related proteins are functionally active in human spermatozoa and may be involved in the regulation of cell survival and motility. Sci Rep. 2016 Sep 16;6:33647.
Fang A, Pierson DL, Mishra SK, Demain AL. Growth of Steptomyces hygroscopicus in rotating-wall bioreactor under simulated microgravity inhibits rapamycin production. Appl Microbiol Biotechnol. 2000 Jul;54(1):33-6.
Cheng YR, Huang J, Qiang H, Lin WL, Demain AL. Mutagenesis of the rapamycin producer Streptomyces hygroscopicus FC904. J Antibiot (Tokyo). 2001 Nov;54(11):967-72.
Heindryckx B, Lierman S, Combelles CM, Cuvelier CA, Gerris J, De Sutter P. Aberrant spindle structures responsible for recurrent human metaphase I oocyte arrest with attempts to induce meiosis artificially. Hum Reprod. 2011 Apr;26(4):791-800.
Shkolnik K, Tadmor A, Ben-Dor S, Nevo N, Galiani D, Dekel N. Reactive oxygen species are indispensable in ovulation. Proc Natl Acad Sci U S A. 2011 Jan 25;108(4):1462-7.
Amdani SN, Jones C, Coward K. Phospholipase C zeta (PLC ζ): oocyte activation and clinical links to male factor infertility. Adv Biol Regul 2013;53(3):292–308.
Smith-Garvin JE, Koretzky GA, Jordan MS. T cell activation. Annu Rev Immunol 2009;27:591–619.
Spina CA, Anderson J, Archin NM, et al. An in-depth comparison of latent HIV-1 reactivation in multiple cell model systems and resting CD4+ T cells from aviremic patients. PLoS Pathog 2013;9(12):e1003834.
Marsden MD, Zack JA. HIV/AIDS eradication. Bioorg Med Chem Lett 2013;23(14):4003–10.
Sena LA, Li S, Jairaman A, et al. Mitochondria are required for antigen-specific T cell activation through reactive oxygen species signaling. Immunity. 2013 Feb 21;38(2):225-36.
Barquero AA, Dávola ME, Riva DA, Mersich SE, Alché LE. Naturally occurring compounds elicit HIV-1 replication in chronically infected promonocytic cells. Biomed Res Int. 2014;2014:989101.
Imai K, Ochiai K, Okamoto T. Reactivation of latent HIV-1 infection by the periodontopathic bacterium Porphyromonas gingivalis involves histone modification. J Immunol. 2009 Mar 15;182(6):3688-95.
Piette J, Legrand-Poels S. HIV-1 reactivation after an oxidative stress mediated by different reactive oxygen species. Chem Biol Interact. 1994 Jun;91(2-3):79-89.
Anderson I, Low JS, Weston S, et al. Heat shock protein 90 controls HIV-1 reactivation from latency. Proc Natl Acad Sci U S A. 2014 Apr 15;111(15):E1528-37.
Zhou H, Xu M, Huang Q, et al. Genome-scale RNAi screen for host factors required for HIV replication. Cell Host Microbe. 2008 Nov 13;4(5):495-504.
Wu H, Esteve E, Tremaroli V, et al. Metformin alters the gut microbiome of individuals with treatment-naive type 2 diabetes, contributing to the therapeutic effects of the drug. Nat Med. 2017 Jul;23(7):850-858.
G.M. Chew, D.C. Chow, S.A. Souza, et al. Impact of adjunctive metformin therapy on T cell exhaustion and viral persistence in a clinical trial of HIV-infected adults on suppressive ART. Journal of Virus Eradication 2017; 3 (Supplement 1): 6–19.
http://viruseradication.com/supplement-details/Abstracts_of_the_IAS_HIV_Cure_and_Cancer_Forum_2017/
Ullrich NJ, Gordon LB. Hutchinson-Gilford progeria syndrome. Handb Clin Neurol. 2015;132:249-64.
Berro R, Kehn K, de la Fuente C, et al. Acetylated Tat regulates human immunodeficiency virus type 1 splicing through its interaction with the splicing regulator p32. J Virol 2006;80(7):3189–204.
Paz S, Lu ML, Takata H, Trautmann L, Caputi M. SRSF1 RNA Recognition Motifs Are Strong Inhibitors of HIV-1 Replication. J Virol. 2015 Jun;89(12):6275-86.
Lopez-Mejia IC, Vautrot V, De Toledo M, et al. A conserved splicing mechanism of the LMNA gene controls premature aging. Hum Mol Genet. 2011 Dec 1;20(23):4540-55.
Park SK, Shin OS. Metformin alleviates ageing cellular phenotypes in Hutchinson-Gilford progeria syndrome dermal fibroblasts. Exp Dermatol. 2017 Oct;26(10):889-895.
Egesipe AL, Blondel S, Cicero AL, et al. Metformin decreases progerin expression and alleviates pathological defects of Hutchinson-Gilford progeria syndrome cells. NPJ Aging Mech Dis. 2016 Nov 10;2:16026.
Finley J. Alteration of splice site selection in the LMNA gene and inhibition of progerin production via AMPK activation. Med Hypotheses. 2014 Nov;83(5):580-7.
Decuypere JP, Welkenhuyzen K, Luyten T, et al. Ins(1,4,5)P3 receptor-mediated Ca2+ signaling and autophagy induction are interrelated. Autophagy. 2011 Dec;7(12):1472-89.
Cao Y, Fang Y, Cai J, et al. ROS functions as an upstream trigger for autophagy to drive hematopoietic stem cell differentiation. Hematology. 2016 Dec;21(10):613-618.
Decuypere JP, Kindt D, Luyten T, et al. mTOR-Controlled Autophagy Requires Intracellular Ca(2+) Signaling. PLoS One. 2013;8(4):e61020.
Cao K, Graziotto JJ, Blair CD, et al. Rapamycin reverses cellular phenotypes and enhances mutant protein clearance in Hutchinson-Gilford progeria syndrome cells. Sci Transl Med. 2011 Jun 29;3(89):89ra58.
Gabriel D, Gordon LB, Djabali K. Temsirolimus Partially Rescues the Hutchinson-Gilford Progeria Cellular Phenotype. PLoS One. 2016 Dec 29;11(12):e0168988.
Moncharmont C, Levy A, Gilormini M, et al. Targeting a cornerstone of radiation resistance: cancer stem cell. Cancer Lett 2012;322(2):139–47.
Finley J. Elimination of cancer stem cells and reactivation of latent HIV-1 via AMPK activation: Common mechanism of action linking inhibition of tumorigenesis and the potential eradication of HIV-1. Med Hypotheses. 2017 Jul;104:133-146.
Buzon MJ, Sun H, Li C, et al. HIV-1 persistence in CD4+ T cells with stem cell-like properties. Nat Med 2014;20(2):139–42.
Wee S, Niklasson M, Marinescu VD, et al. Selective calcium sensitivity in immature glioma cancer stem cells. PLoS One. 2014 Dec 22;9(12):e115698.
Hayun M, Okun E, Hayun R, Gafter U, et al. Synergistic effect of AS101 and Bryostatin-1 on myeloid leukemia cell differentiation in vitro and in an animal model. Leukemia 2007;21(7):1504–13.
Sato A, Sunayama J, Okada M, et al. Glioma-initiating cell elimination by metformin activation of FOXO3 via AMPK. Stem Cells Transl Med 2012;1(11):811–24.
Fasih A, Elbaz HA, Hüttemann M, Konski AA, Zielske SP. Radiosensitization of pancreatic cancer cells by metformin through the AMPK pathway. Radiat Res 2014;182(1):50–9.
#ampk#gut bacteria#metformin#oocyte#HIV/AIDS#progeria#cancer stem cell#evolution#charles darwin#sperm#acrosome reaction#fertilization#reproduction
1 note
·
View note
Text
Metformin & AMPK Link Nobel Prize-winning Telomeres & Jumping Genes with Learning, HIV, & the Creation of Human Life
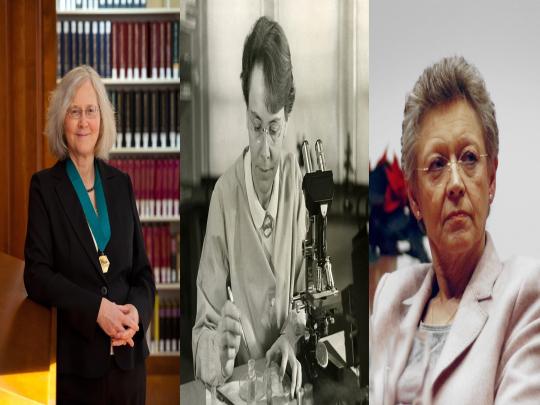
Nobel Prize winners, from left to right: Elizabeth Blackburn (discovered telomerase), Barbara McClintock (discovered “jumping genes”), and Françoise Barré-Sinoussi (discovered HIV). By Science History Institute, CC BY-SA 3.0, https://commons.wikimedia.org/w/index.php?curid=30112731; By Smithsonian Institution/Science Service; Restored by Adam Cuerden - Flickr: Barbara McClintock (1902-1992), Public Domain, https://commons.wikimedia.org/w/index.php?curid=25629182; By Prolineserver (talk) - Own work, GFDL 1.2, https://commons.wikimedia.org/w/index.php?curid=5395403
A recently published study in the journal Biochimica et Biophysica Acta (BBA) - Molecular Basis of Disease in 2018 demonstrated for the first time that chronic treatment with the anti-diabetic drug metformin activated human telomerase in human aortic endothelial cells (HAECs) and significantly delayed endothelial senescence in an AMPK-dependent manner [11]. Telomeres are specialized regions of repetitive nucleotide sequences located at the ends of eukaryotic chromosomes that protect chromosomal ends from deterioration [63]. However, continuous cell division leads to telomere shortening, impeding the replenishment of tissues and triggering cellular senescence (i.e. cells cease to divide). Although human telomeres shorten with age, telomeres may be lengthened by the enzyme telomerase [64].
This study substantiates and confirms several novel proposals in a recently published paper I authored in April of 2018 in which I first proposed that because telomerase is derived from a “jumping gene” (see below for discussion), metformin would activate telomerase via AMPK [6]. My paper also highlights a novel link between hippocampal long-term potentiation (essential for learning and memory), alleviation of accelerated cellular aging in Hutchinson-Gilford progeria syndrome, oocyte activation and the sperm acrosome reaction (prerequisites for human life creation), and transposable element (i.e. “jumping genes”)-mediated promotion of learning, memory, and the creation of human life [1-7]. Indeed, these novel proposals also link several Nobel Prize-winning discoveries, including the discovery of telomerase by Elizabeth Blackburn (photo-left), the discovery of “jumping genes” by Barbara McClintock (photo-middle), and the discovery of HIV by Françoise Barré-Sinoussi (photo-right).
The link between such disparate physiological and pathophysiological phenomena is cellular stress-induced modulation of energy metabolism, leading to the activation of the master metabolic regulator AMPK, a kinase that increases lifespan and healthspan in several model organisms [12]. I was the first to propose and publish (2014) that an increase in beneficial levels of cellular stress (e.g. increases in the levels reactive oxygen species [ROS], calcium [Ca2+], and/or an AMP(ADP)/ATP ratio increase, etc.) and activation of AMPK by compounds including metformin would alleviate accelerated cellular aging defects in children diagnosed with the genetic disorder Hutchinson-Gilford progeria syndrome (HGPS), a disease characterized by an accelerated aging phenotype and death at ~14.6 years of age [1]. This hypothesis was substantiated in 2016 and 2017, with metformin activating AMPK in cells taken from HGPS kids and ameliorating accelerated cellular aging defects (e.g. correcting nuclear morphology, decrease in senescence markers, etc.) [8,9]. Additionally, transfection of telomerase been shown to reverse senescence in HGPS cells [65]. As metformin activates telomerase and normal humans make the same toxic protein (called progerin) that leads to accelerated aging in HGPS kids (just at lower amounts that accumulate with age), AMPK activation may also play a significant role in ameliorating diseases associated with physiological aging [10,11].
AMPK also links HGPS with potential virus eradication. I first proposed in 2015 that AMPK activation links alleviation of accelerated aging in HGPS with the potential eradication of HIV-1 via the “shock and kill” approach, a method currently being pursued by HIV cure researchers to possibly eradicate HIV-1 [2,13]. The same gene splicing factor that promotes accelerated aging in HGPS (called SRSF1 or ASF/SF2) also inhibits reactivation of latent HIV-1 (i.e. “shock”), preventing immune system detection and virus destruction (i.e. “kill”) [8,14]. Metformin was shown to slow aging in HGPS cells by decreasing this splicing factor, as I originally predicted in 2014, and several compounds that potently induce latent HIV-1 reactivation in T cells from infected patients, including PMA (a phorbol ester) combined with ionomycin, each activate AMPK [1,8,15-17]. AMPKα1 deletion leads to a decrease in primary T cell responses to bacterial and viral infections in vivo, AMPK knockdown leads to cell death on T cell activation, and metformin has recently been shown to inhibit Zika and Dengue viruses, the malaria parasite, and Legionella pneumophila [18-23]. Intriguingly, early data presented at the International AIDS Conference in 2017 demonstrated that metformin destabilized the latent HIV-1 reservoir in chronically-infected HIV patients and decreased the percentage of CD4+ T cells expressing the immune checkpoint receptors PD-1, TIGIT, and TIM-3, each markers associated with T cells latently infected with HIV-1, indicating that AMPK activation may indeed contribute to a cure for HIV-1 [24,25].
As I first proposed in 2016 and 2017, the induction of cellular stress and AMPK activation also links HGPS and potential HIV-1 eradication with oocyte activation and the sperm acrosome reaction, prerequisites for the creation of human life [3,4]. Increases in both ROS and Ca2+ are critical for T cell activation (and hence latent HIV-1 reactivation) and ROS is transiently increased in HGPS cells when treated with a rapamycin analog to alleviate accelerated aging [26-28]. Stress-induced activation of AMPK by AICAR and other compounds promotes oocyte maturation, which precedes and is essential for efficient oocyte activation [29,30]. Oocyte activation is indispensable for the creation of all human life and PMA and ionomycin, which collectively reactivates latent HIV-1, activates mouse and human oocytes, respectively [31,32]. AMPK is also found in the acrosome of the human sperm head and ionomycin induces the acrosome reaction in human sperm, a process necessary for oocyte penetration and fertilization [33,34]. Ionomycin is also used extensively during fertility procedures to activate human oocytes (i.e. “shock”), creating normal, healthy children (i.e. “live”) [32]. Interestingly, ionomycin is a narrow spectrum antibiotic produced by certain species within the bacterial genus Streptomyces, from which ~70 percent of clinically useful antibiotics are derived [35,36]. Cellular stress, mediated by increases in ROS, Ca2+, and/or an AMP(ADP)/ATP ratio increase, etc. also enhances antibiotic production in many Streptomyces strains, reinforcing the notion that the beneficial effects of cellular stress induction crosses species boundaries [37,38].
Cellular stress induction and AMPK activation also link HGPS, potential HIV-1 eradication, and human life creation with learning and memory, a hypothesis I originally proposed in 2018 [6]. Hippocampal long-term potentiation (LTP) is considered the cellular correlate of learning and memory and AMPK has been found localized in hippocampal CA1 dendrites and is activated in neurons by metformin, AICAR, ionomycin, and glutamate, a neurotransmitter essential for hippocampal LTP induction [39-41]. The glutamate receptors AMPAR and NMDAR are found on and modulate T cell activation, AMPK activation increases synthesis and membrane insertion of AMPARs (critical for LTP expression), PMA enhances hippocampal CA1 LTP, and inhibition of ROS significantly impairs hippocampal CA1 LTP [42-46]. Also, neuronal depolarization decreases the recruitment efficiency of SRSF1 to nascent RNAs and promotes SRSF1 nuclear speckle accumulation [6]. SRSF1, a gene splicing factor that is inhibited by metformin, enhances progerin production in HGPS cells and prevents latent HIV-1 reactivation [2,8]. Metformin also significantly reduces pathology-associated reductions in LTP in animal models in vivo, indicating that learning and memory are linked to HGPS, potential HIV-1 eradication, and human life creation via the induction of beneficial levels of cellular stress [47].
Lastly, cellular stress and AMPK activation also links the activation and mobilization of transposable elements (i.e. “jumping genes”) with telomerase activation, potential HIV-1 eradication, learning and memory, and the creation of human life, a hypothesis I originally proposed in 2018 [6]. Transposable elements (TEs) are DNA sequences first described by Nobel laureate Barbara McClintock that comprise nearly half of the human genome, are able to transpose or move from one genomic location to another, and have played an extensive role in human genome evolution [48-50]. Strikingly, McClintock also described in her Nobel Prize speech that a genome “shock” seemed to promote TE activation and mobilization [50]. As first noted in my recently published paper, this “shock” is the same “shock” that HIV cure researchers are using during the “shock and kill” approach to reactivate latent HIV-1 to potentially effectuate a cure [6]. Indeed, several forms of cellular stress, including heat shock and radiation, have been convincingly shown to activate and enhance TE mobilization in several model organisms and in human cells [51-53]. This same “shock” McClintock referred to, mediated by increases in ROS, Ca2+, and/or an AMP(ADP)/ATP ratio, etc. is also what leads to the creation of human life, as the antibiotic ionomycin activates AMPK, promotes TE activation, and induces human oocyte activation [17,32,54]. LINE-1 (L1), a member of the retrotransposon class of TEs, is active and capable of mobilization in human oocytes, human sperm, and in human neural progenitor cells [55-57]. Inhibition of L1 impairs both oocyte maturation in vitro and long-term memory formation in vivo in mice [58,59]. L1 has also been detected in the human brain and is capable of mobilization in human neurons [57]. As noted above, AMPK is critical for oocyte maturation and metformin promotes hippocampal neurogenesis and spatial memory formation [29,60]. The landmark initial sequencing of the human genome also noted that both telomerase and RAG1 (promotes DNA cleavage and transposition in human cells) are derived from TEs [49]. Because metformin activates both telomerase and RAG1 via AMPK, it is likely that cellular stress-induced AMPK activation facilitates beneficial TE activation and mobilization (i.e. learning and memory associated with L1 mobilization), linking human genome evolution and the creation of human life with hippocampal LTP, HGPS, and potential HIV-1 eradication [61,62].
https://www.linkedin.com/pulse/metformin-ampk-link-nobel-prize-winning-telomeres-jumping-finley/


References
Finley J. Alteration of splice site selection in the LMNA gene and inhibition of progerin production via AMPK activation. Med Hypotheses. 2014 Nov;83(5):580-7.
Finley J. Reactivation of latently infected HIV-1 viral reservoirs and correction of aberrant alternative splicing in the LMNA gene via AMPK activation: Common mechanism of action linking HIV-1 latency and Hutchinson-Gilford progeria syndrome. Med Hypotheses. 2015 Sep;85(3):320-32.
Finley J. Oocyte activation and latent HIV-1 reactivation: AMPK as a common mechanism of action linking the beginnings of life and the potential eradication of HIV-1. Med Hypotheses. 2016 Aug;93:34-47.
Finley J. Elimination of cancer stem cells and reactivation of latent HIV-1 via AMPK activation: Common mechanism of action linking inhibition of tumorigenesis and the potential eradication of HIV-1. Med Hypotheses. 2017 Jul;104:133-146.
Finley J. AMPK activation as a common mechanism of action linking the effects of diverse compounds that ameliorate accelerated cellular aging defects in Hutchinson-Gilford progeria syndrome. Med Hypotheses. Manuscript submitted.
Finley J. Facilitation of hippocampal long-term potentiation and reactivation of latent HIV-1 via AMPK activation: Common mechanism of action linking learning, memory, and the potential eradication of HIV-1. Med Hypotheses. 2018 Jul;116: 61-73.
Finley J. Transposable elements, placental development, and oocyte activation: Cellular stress and AMPK links jumping genes with the creation of human life. Med Hypotheses. Under Review.
Egesipe AL, Blondel S, Cicero AL, et al. Metformin decreases progerin expression and alleviates pathological defects of Hutchinson-Gilford progeria syndrome cells. NPJ Aging Mech Dis. 2016 Nov 10;2:16026.
Park SK, Shin OS. Metformin alleviates ageing cellular phenotypes in Hutchinson-Gilford progeria syndrome dermal fibroblasts. Exp Dermatol. 2017 Oct;26(10):889-895.
McClintock D, Ratner D, Lokuge M, et al. The mutant form of lamin A that causes Hutchinson-Gilford progeria is a biomarker of cellular aging in human skin. PLoS One. 2007 Dec 5;2(12):e1269.
Karnewar S, Neeli PK, Panuganti D, et al. Metformin regulates mitochondrial biogenesis and senescence through AMPK mediated H3K79 methylation: Relevance in age-associated vascular dysfunction. Biochim Biophys Acta. 2018 Apr;1864(4 Pt A):1115-1128.
Salminen A, Kaarniranta K. AMP-activated protein kinase (AMPK) controls the aging process via an integrated signaling network. Ageing Res Rev. 2012 Apr;11(2):230-41.
Kim Y, Anderson JL, Lewin SR. Getting the "Kill" into "Shock and Kill": Strategies to Eliminate Latent HIV. Cell Host Microbe. 2018 Jan 10;23(1):14-26.
Berro R, Kehn K, de la Fuente C, et al. Acetylated Tat regulates human immunodeficiency virus type 1 splicing through its interaction with the splicing regulator p32. J Virol 2006;80(7):3189–204.
Spina CA, Anderson J, Archin NM, et al. An in-depth comparison of latent HIV-1 reactivation in multiple cell model systems and resting CD4+ T cells from aviremic patients. PLoS Pathog 2013;9(12):e1003834.
Zogovic N, Tovilovic-Kovacevic G, Misirkic-Marjanovic M, et al. Coordinated activation of AMP-activated protein kinase, extracellular signal-regulated kinase, and autophagy regulates phorbol myristate acetate-induced differentiation of SH-SY5Y neuroblastoma cells. J Neurochem 2015;133 (2):223–32.
Tamás P, Hawley SA, Clarke RG, et al. Regulation of the energy sensor AMP-activated protein kinase by antigen receptor and Ca2+ in T lymphocytes. J Exp Med. 2006 Jul 10;203(7):1665-70.
Blagih J, Coulombe F, Vincent EE, et al. The energy sensor AMPK regulates T cell metabolic adaptation and effector responses in vivo. Immunity. 2015 Jan 20;42(1):41-54.
Rao E, Zhang Y, Zhu G, et al. Deficiency of AMPK in CD8+ T cells suppresses their anti-tumor function by inducing protein phosphatase-mediated cell death. Oncotarget. 2015 Apr 10;6(10):7944-58.
Cheng F, Ramos da Silva S, Huang IC, Jung JU, Gao SJ. Suppression of Zika virus infection and replication in endothelial cells and astrocytes by PKA inhibitor PKI 14-22. J Virol. 2017 Dec 6. pii: JVI.02019-17.
Soto-Acosta R, Bautista-Carbajal P, Cervantes-Salazar M, Angel-Ambrocio AH, Del Angel RM. DENV up-regulates the HMG-CoA reductase activity through the impairment of AMPK phosphorylation: A potential antiviral target. PLoS Pathog. 2017 Apr 6;13(4):e1006257.
Ruivo MT, Vera IM, Sales-Dias J, et al. Host AMPK Is a Modulator of Plasmodium Liver Infection. Cell Rep. 2016 Sep 6;16(10):2539-45.
Kajiwara C, Kusaka Y, Kimura S, et al. Metformin Mediates Protection against Legionella Pneumonia through Activation of AMPK and Mitochondrial Reactive Oxygen Species. J Immunol. 2017 Dec 15. pii: ji1700474.
G.M. Chew, D.C. Chow, S.A. Souza, et al. Impact of adjunctive metformin therapy on T cell exhaustion and viral persistence in a clinical trial of HIV-infected adults on suppressive ART. Journal of Virus Eradication 2017; 3 (Supplement 1): 6–19.
http://viruseradication.com/abstract-details.php?abstract_id=1188, last accessed March 14, 2018.
Oh-hora M, Rao A. Calcium signaling in lymphocytes. Curr Opin Immunol 2008;20(3):250–8.
Sena LA, Li S, Jairaman A, et al. Mitochondria are required for antigen-specific T cell activation through reactive oxygen species signaling. Immunity. 2013 Feb 21;38(2):225-36.
Gabriel D, Gordon LB, Djabali K. Temsirolimus Partially Rescues the Hutchinson-Gilford Progeria Cellular Phenotype. PLoS One. 2016 Dec 29;11(12):e0168988.
Chen J, Hudson E, Chi MM, et al. AMPK regulation of mouse oocyte meiotic resumption in vitro. Dev Biol. 2006 Mar 15;291(2):227-38.
LaRosa C, Downs SM. Stress stimulates AMP-activated protein kinase and meiotic resumption in mouse oocytes. Biol Reprod. 2006 Mar;74(3):585-92.
Uranga JA, Pedersen RA, Arechaga J. Parthenogenetic activation of mouse oocytes using calcium ionophores and protein kinase C stimulators. Int J Dev Biol. 1996 Apr;40(2):515-9.
Deemeh MR, Tavalaee M, Nasr-Esfahani MH. Health of children born through artificial oocyte activation: a pilot study. Reprod Sci. 2015 Mar;22(3):322-8.
Calle-Guisado V, de Llera AH, Martin-Hidalgo D, et al. AMP-activated kinase in human spermatozoa: identification, intracellular localization, and key function in the regulation of sperm motility. Asian J Androl. 2017 Nov-Dec;19(6):707-714.
Sánchez-Cárdenas C, Servín-Vences MR, José O, Treviño CL, Hernández-Cruz A, Darszon A. Acrosome reaction and Ca²+ imaging in single human spermatozoa: new regulatory roles of [Ca²+]i. Biol Reprod. 2014 Sep;91(3):67.
Liu WC, Slusarchyk DS, Astle G, Trejo WH, Brown WE, Meyers E. Ionomycin, a new polyether antibiotic. J Antibiot (Tokyo). 1978 Sep;31(9):815-9.
Kitani S, Miyamoto KT, Takamatsu S, et al. Avenolide, a Streptomyces hormone controlling antibiotic production in Streptomyces avermitilis. Proc Natl Acad Sci U S A. 2011 Sep 27;108(39):16410-5.
Meng L, Li M, Yang SH, Kim TJ, Suh JW. Intracellular ATP levels affect secondary metabolite production in Streptomyces spp. Biosci Biotechnol Biochem. 2011;75(8):1576-81.
Wang D, Wei L, Zhang Y, Zhang M, Gu S. Physicochemical and microbial responses of Streptomyces natalensis HW-2 to fungal elicitor. Appl Microbiol Biotechnol. 2017 Sep;101(17):6705-6712.
Potter WB, O'Riordan KJ, Barnett D, et al. Metabolic regulation of neuronal plasticity by the energy sensor AMPK. PLoS One. 2010 Feb 1;5(2):e8996.
Sample V, Ramamurthy S, Gorshkov K, Ronnett GV, Zhang J. Polarized activities of AMPK and BRSK in primary hippocampal neurons. Mol Biol Cell. 2015 May 15;26(10):1935-46
Terunuma M, Vargas KJ, Wilkins ME, et al. Prolonged activation of NMDA receptors promotes dephosphorylation and alters postendocytic sorting of GABAB receptors. Proc Natl Acad Sci U S A. 2010 Aug 3;107(31):13918-23.
Ganor Y, Besser M, Ben-Zakay N, Unger T, Levite M. Human T cells express a functional ionotropic glutamate receptor GluR3, and glutamate by itself triggers integrin-mediated adhesion to laminin and fibronectin and chemotactic migration. J Immunol. 2003 Apr 15;170(8):4362-72.
Miglio G, Varsaldi F, Lombardi G. Human T lymphocytes express N-methyl-D-aspartate receptors functionally active in controlling T cell activation. Biochem Biophys Res Commun. 2005 Dec 30;338(4):1875-83.
Wang G, Amato S, Gilbert J, Man HY. Resveratrol up-regulates AMPA receptor expression via AMP-activated protein kinase-mediated protein translation. Neuropharmacology. 2015 Aug;95:144-53.
Kim EC, Lee MJ, Shin SY, et al. Phorbol 12-Myristate 13-Acetate Enhances Long-Term Potentiation in the Hippocampus through Activation of Protein Kinase Cδ and ε. Korean J Physiol Pharmacol. 2013 Feb;17(1):51-6.
Klann E. Cell-permeable scavengers of superoxide prevent long-term potentiation in hippocampal area CA1. J Neurophysiol. 1998 Jul;80(1):452-7.
Asadbegi M, Yaghmaei P, Salehi I, Ebrahim-Habibi A, Komaki A. Neuroprotective effects of metformin against Aβ-mediated inhibition of long-term potentiation in rats fed a high-fat diet. Brain Res Bull. 2016 Mar;121:178-85.
Muñoz-López M, García-Pérez JL. DNA transposons: nature and applications in genomics. Curr Genomics. 2010 Apr;11(2):115-28.
Lander ES, Linton LM, Birren B, et al. Initial sequencing and analysis of the human genome. Nature. 2001 Feb 15;409(6822):860-921.
McClintock B. The significance of responses of the genome to challenge. Science. 1984 Nov 16;226(4676):792-801.
Stanley D, Fraser S, Stanley GA, Chambers PJ. Retrotransposon expression in ethanol-stressed Saccharomyces cerevisiae. Appl Microbiol Biotechnol. 2010 Jul;87(4):1447-54.
Vasilyeva LA, Bubenshchikova EV, Ratner VA. Heavy heat shock induced retrotransposon transposition in Drosophila. Genet Res. 1999 Oct;74(2):111-9.
Farkash EA, Kao GD, Horman SR, Prak ET. Gamma radiation increases endonuclease-dependent L1 retrotransposition in a cultured cell assay. Nucleic Acids Res. 2006 Feb 28;34(4):1196-204.
Magun BE, Rodland KD. Transient inhibition of protein synthesis induces the immediate early gene VL30: alternative mechanism for thapsigargin-induced gene expression. Cell Growth Differ. 1995 Jul;6(7):891-7.
Georgiou I, Noutsopoulos D, Dimitriadou E, et al. Retrotransposon RNA expression and evidence for retrotransposition events in human oocytes. Hum Mol Genet. 2009 Apr 1;18(7):1221-8.
Lazaros L, Kitsou C, Kostoulas C, et al. Retrotransposon expression and incorporation of cloned human and mouse retroelements in human spermatozoa. Fertil Steril. 2017 Mar;107(3):821-830.
Coufal NG, Garcia-Perez JL, Peng GE, et al. L1 retrotransposition in human neural progenitor cells. Nature. 2009 Aug 27;460(7259):1127-31.
Luo YB, Zhang L, Lin ZL, et al. Distinct subcellular localization and potential role of LINE1-ORF1P in meiotic oocytes. Histochem Cell Biol. 2016 Jan;145(1):93-104.
Bachiller S, Del-Pozo-Martín Y, Carrión ÁM. L1 retrotransposition alters the hippocampal genomic landscape enabling memory formation. Brain Behav Immun. 2017 Aug;64:65-70.
Ahmed S, Mahmood Z, Javed A, et al. Effect of Metformin on Adult Hippocampal Neurogenesis: Comparison with Donepezil and Links to Cognition. J Mol Neurosci. 2017 May;62(1):88-98.
Karnewar S, Neeli PK, Panuganti D, et al. Metformin regulates mitochondrial biogenesis and senescence through AMPK mediated H3K79 methylation: Relevance in age-associated vascular dysfunction. Biochim Biophys Acta. 2018 Apr;1864(4 Pt A):1115-1128.
Um JH, Brown AL, Singh SK, et al. Metabolic sensor AMPK directly phosphorylates RAG1 protein and regulates V(D)J recombination. Proc Natl Acad Sci U S A. 2013 Jun 11;110(24):9873-8.
O'Sullivan RJ, Karlseder J. Telomeres: protecting chromosomes against genome instability. Nat Rev Mol Cell Biol. 2010 Mar;11(3):171-81.
Cohen SB, Graham ME, Lovrecz GO, Bache N, Robinson PJ, Reddel RR. Protein composition of catalytically active human telomerase from immortal cells. Science. 2007 Mar 30;315(5820):1850-3.
Li Y, Zhou G, Bruno IG, Cooke JP. Telomerase mRNA Reverses Senescence in Progeria Cells. J Am Coll Cardiol. 2017 Aug 8;70(6):804-805.
0 notes
Text
Metformin & AMPK Link Nobel Prize-winning Telomeres & Jumping Genes with Learning, HIV, & the Creation of Human Life

Nobel Prize winners, from left to right: Elizabeth Blackburn (discovered telomerase), Barbara McClintock (discovered “jumping genes”), and Françoise Barré-Sinoussi (discovered HIV). By Science History Institute, CC BY-SA 3.0, https://commons.wikimedia.org/w/index.php?curid=30112731; By Smithsonian Institution/Science Service; Restored by Adam Cuerden - Flickr: Barbara McClintock (1902-1992), Public Domain, https://commons.wikimedia.org/w/index.php?curid=25629182; By Prolineserver (talk) - Own work, GFDL 1.2, https://commons.wikimedia.org/w/index.php?curid=5395403
A recently published study in the journal Biochimica et Biophysica Acta (BBA) - Molecular Basis of Disease in 2018 demonstrated for the first time that chronic treatment with the anti-diabetic drug metformin activated human telomerase in human aortic endothelial cells (HAECs) and significantly delayed endothelial senescence in an AMPK-dependent manner [11]. Telomeres are specialized regions of repetitive nucleotide sequences located at the ends of eukaryotic chromosomes that protect chromosomal ends from deterioration [63]. However, continuous cell division leads to telomere shortening, impeding the replenishment of tissues and triggering cellular senescence (i.e. cells cease to divide). Although human telomeres shorten with age, telomeres may be lengthened by the enzyme telomerase [64].
This study substantiates and confirms several novel proposals in a recently published paper I authored in April of 2018 in which I first proposed that because telomerase is derived from a “jumping gene” (see below for discussion), metformin would activate telomerase via AMPK [6]. My paper also highlights a novel link between hippocampal long-term potentiation (essential for learning and memory), alleviation of accelerated cellular aging in Hutchinson-Gilford progeria syndrome, oocyte activation and the sperm acrosome reaction (prerequisites for human life creation), and transposable element (i.e. “jumping genes”)-mediated promotion of learning, memory, and the creation of human life [1-7]. Indeed, these novel proposals also link several Nobel Prize-winning discoveries, including the discovery of telomerase by Elizabeth Blackburn (photo-left), the discovery of “jumping genes” by Barbara McClintock (photo-middle), and the discovery of HIV by Françoise Barré-Sinoussi (photo-right).
The link between such disparate physiological and pathophysiological phenomena is cellular stress-induced modulation of energy metabolism, leading to the activation of the master metabolic regulator AMPK, a kinase that increases lifespan and healthspan in several model organisms [12]. I was the first to propose and publish (2014) that an increase in beneficial levels of cellular stress (e.g. increases in the levels reactive oxygen species [ROS], calcium [Ca2+], and/or an AMP(ADP)/ATP ratio increase, etc.) and activation of AMPK by compounds including metformin would alleviate accelerated cellular aging defects in children diagnosed with the genetic disorder Hutchinson-Gilford progeria syndrome (HGPS), a disease characterized by an accelerated aging phenotype and death at ~14.6 years of age [1]. This hypothesis was substantiated in 2016 and 2017, with metformin activating AMPK in cells taken from HGPS kids and ameliorating accelerated cellular aging defects (e.g. correcting nuclear morphology, decrease in senescence markers, etc.) [8,9]. Additionally, transfection of telomerase been shown to reverse senescence in HGPS cells [65]. As metformin activates telomerase and normal humans make the same toxic protein (called progerin) that leads to accelerated aging in HGPS kids (just at lower amounts that accumulate with age), AMPK activation may also play a significant role in ameliorating diseases associated with physiological aging [10,11].
AMPK also links HGPS with potential virus eradication. I first proposed in 2015 that AMPK activation links alleviation of accelerated aging in HGPS with the potential eradication of HIV-1 via the “shock and kill” approach, a method currently being pursued by HIV cure researchers to possibly eradicate HIV-1 [2,13]. The same gene splicing factor that promotes accelerated aging in HGPS (called SRSF1 or ASF/SF2) also inhibits reactivation of latent HIV-1 (i.e. “shock”), preventing immune system detection and virus destruction (i.e. “kill”) [8,14]. Metformin was shown to slow aging in HGPS cells by decreasing this splicing factor, as I originally predicted in 2014, and several compounds that potently induce latent HIV-1 reactivation in T cells from infected patients, including PMA (a phorbol ester) combined with ionomycin, each activate AMPK [1,8,15-17]. AMPKα1 deletion leads to a decrease in primary T cell responses to bacterial and viral infections in vivo, AMPK knockdown leads to cell death on T cell activation, and metformin has recently been shown to inhibit Zika and Dengue viruses, the malaria parasite, and Legionella pneumophila [18-23]. Intriguingly, early data presented at the International AIDS Conference in 2017 demonstrated that metformin destabilized the latent HIV-1 reservoir in chronically-infected HIV patients and decreased the percentage of CD4+ T cells expressing the immune checkpoint receptors PD-1, TIGIT, and TIM-3, each markers associated with T cells latently infected with HIV-1, indicating that AMPK activation may indeed contribute to a cure for HIV-1 [24,25].
As I first proposed in 2016 and 2017, the induction of cellular stress and AMPK activation also links HGPS and potential HIV-1 eradication with oocyte activation and the sperm acrosome reaction, prerequisites for the creation of human life [3,4]. Increases in both ROS and Ca2+ are critical for T cell activation (and hence latent HIV-1 reactivation) and ROS is transiently increased in HGPS cells when treated with a rapamycin analog to alleviate accelerated aging [26-28]. Stress-induced activation of AMPK by AICAR and other compounds promotes oocyte maturation, which precedes and is essential for efficient oocyte activation [29,30]. Oocyte activation is indispensable for the creation of all human life and PMA and ionomycin, which collectively reactivates latent HIV-1, activates mouse and human oocytes, respectively [31,32]. AMPK is also found in the acrosome of the human sperm head and ionomycin induces the acrosome reaction in human sperm, a process necessary for oocyte penetration and fertilization [33,34]. Ionomycin is also used extensively during fertility procedures to activate human oocytes (i.e. “shock”), creating normal, healthy children (i.e. “live”) [32]. Interestingly, ionomycin is a narrow spectrum antibiotic produced by certain species within the bacterial genus Streptomyces, from which ~70 percent of clinically useful antibiotics are derived [35,36]. Cellular stress, mediated by increases in ROS, Ca2+, and/or an AMP(ADP)/ATP ratio increase, etc. also enhances antibiotic production in many Streptomyces strains, reinforcing the notion that the beneficial effects of cellular stress induction crosses species boundaries [37,38].
Cellular stress induction and AMPK activation also link HGPS, potential HIV-1 eradication, and human life creation with learning and memory, a hypothesis I originally proposed in 2018 [6]. Hippocampal long-term potentiation (LTP) is considered the cellular correlate of learning and memory and AMPK has been found localized in hippocampal CA1 dendrites and is activated in neurons by metformin, AICAR, ionomycin, and glutamate, a neurotransmitter essential for hippocampal LTP induction [39-41]. The glutamate receptors AMPAR and NMDAR are found on and modulate T cell activation, AMPK activation increases synthesis and membrane insertion of AMPARs (critical for LTP expression), PMA enhances hippocampal CA1 LTP, and inhibition of ROS significantly impairs hippocampal CA1 LTP [42-46]. Also, neuronal depolarization decreases the recruitment efficiency of SRSF1 to nascent RNAs and promotes SRSF1 nuclear speckle accumulation [6]. SRSF1, a gene splicing factor that is inhibited by metformin, enhances progerin production in HGPS cells and prevents latent HIV-1 reactivation [2,8]. Metformin also significantly reduces pathology-associated reductions in LTP in animal models in vivo, indicating that learning and memory are linked to HGPS, potential HIV-1 eradication, and human life creation via the induction of beneficial levels of cellular stress [47].
Lastly, cellular stress and AMPK activation also links the activation and mobilization of transposable elements (i.e. “jumping genes”) with telomerase activation, potential HIV-1 eradication, learning and memory, and the creation of human life, a hypothesis I originally proposed in 2018 [6]. Transposable elements (TEs) are DNA sequences first described by Nobel laureate Barbara McClintock that comprise nearly half of the human genome, are able to transpose or move from one genomic location to another, and have played an extensive role in human genome evolution [48-50]. Strikingly, McClintock also described in her Nobel Prize speech that a genome “shock” seemed to promote TE activation and mobilization [50]. As first noted in my recently published paper, this “shock” is the same “shock” that HIV cure researchers are using during the “shock and kill” approach to reactivate latent HIV-1 to potentially effectuate a cure [6]. Indeed, several forms of cellular stress, including heat shock and radiation, have been convincingly shown to activate and enhance TE mobilization in several model organisms and in human cells [51-53]. This same “shock” McClintock referred to, mediated by increases in ROS, Ca2+, and/or an AMP(ADP)/ATP ratio, etc. is also what leads to the creation of human life, as the antibiotic ionomycin activates AMPK, promotes TE activation, and induces human oocyte activation [17,32,54]. LINE-1 (L1), a member of the retrotransposon class of TEs, is active and capable of mobilization in human oocytes, human sperm, and in human neural progenitor cells [55-57]. Inhibition of L1 impairs both oocyte maturation in vitro and long-term memory formation in vivo in mice [58,59]. L1 has also been detected in the human brain and is capable of mobilization in human neurons [57]. As noted above, AMPK is critical for oocyte maturation and metformin promotes hippocampal neurogenesis and spatial memory formation [29,60]. The landmark initial sequencing of the human genome also noted that both telomerase and RAG1 (promotes DNA cleavage and transposition in human cells) are derived from TEs [49]. Because metformin activates both telomerase and RAG1 via AMPK, it is likely that cellular stress-induced AMPK activation facilitates beneficial TE activation and mobilization (i.e. learning and memory associated with L1 mobilization), linking human genome evolution and the creation of human life with hippocampal LTP, HGPS, and potential HIV-1 eradication [61,62].
https://www.linkedin.com/pulse/metformin-ampk-link-nobel-prize-winning-telomeres-jumping-finley/


References
Finley J. Alteration of splice site selection in the LMNA gene and inhibition of progerin production via AMPK activation. Med Hypotheses. 2014 Nov;83(5):580-7.
Finley J. Reactivation of latently infected HIV-1 viral reservoirs and correction of aberrant alternative splicing in the LMNA gene via AMPK activation: Common mechanism of action linking HIV-1 latency and Hutchinson-Gilford progeria syndrome. Med Hypotheses. 2015 Sep;85(3):320-32.
Finley J. Oocyte activation and latent HIV-1 reactivation: AMPK as a common mechanism of action linking the beginnings of life and the potential eradication of HIV-1. Med Hypotheses. 2016 Aug;93:34-47.
Finley J. Elimination of cancer stem cells and reactivation of latent HIV-1 via AMPK activation: Common mechanism of action linking inhibition of tumorigenesis and the potential eradication of HIV-1. Med Hypotheses. 2017 Jul;104:133-146.
Finley J. AMPK activation as a common mechanism of action linking the effects of diverse compounds that ameliorate accelerated cellular aging defects in Hutchinson-Gilford progeria syndrome. Med Hypotheses. Manuscript submitted.
Finley J. Facilitation of hippocampal long-term potentiation and reactivation of latent HIV-1 via AMPK activation: Common mechanism of action linking learning, memory, and the potential eradication of HIV-1. Med Hypotheses. 2018 Jul;116: 61-73.
Finley J. Transposable elements, placental development, and oocyte activation: Cellular stress and AMPK links jumping genes with the creation of human life. Med Hypotheses. Under Review.
Egesipe AL, Blondel S, Cicero AL, et al. Metformin decreases progerin expression and alleviates pathological defects of Hutchinson-Gilford progeria syndrome cells. NPJ Aging Mech Dis. 2016 Nov 10;2:16026.
Park SK, Shin OS. Metformin alleviates ageing cellular phenotypes in Hutchinson-Gilford progeria syndrome dermal fibroblasts. Exp Dermatol. 2017 Oct;26(10):889-895.
McClintock D, Ratner D, Lokuge M, et al. The mutant form of lamin A that causes Hutchinson-Gilford progeria is a biomarker of cellular aging in human skin. PLoS One. 2007 Dec 5;2(12):e1269.
Karnewar S, Neeli PK, Panuganti D, et al. Metformin regulates mitochondrial biogenesis and senescence through AMPK mediated H3K79 methylation: Relevance in age-associated vascular dysfunction. Biochim Biophys Acta. 2018 Apr;1864(4 Pt A):1115-1128.
Salminen A, Kaarniranta K. AMP-activated protein kinase (AMPK) controls the aging process via an integrated signaling network. Ageing Res Rev. 2012 Apr;11(2):230-41.
Kim Y, Anderson JL, Lewin SR. Getting the "Kill" into "Shock and Kill": Strategies to Eliminate Latent HIV. Cell Host Microbe. 2018 Jan 10;23(1):14-26.
Berro R, Kehn K, de la Fuente C, et al. Acetylated Tat regulates human immunodeficiency virus type 1 splicing through its interaction with the splicing regulator p32. J Virol 2006;80(7):3189–204.
Spina CA, Anderson J, Archin NM, et al. An in-depth comparison of latent HIV-1 reactivation in multiple cell model systems and resting CD4+ T cells from aviremic patients. PLoS Pathog 2013;9(12):e1003834.
Zogovic N, Tovilovic-Kovacevic G, Misirkic-Marjanovic M, et al. Coordinated activation of AMP-activated protein kinase, extracellular signal-regulated kinase, and autophagy regulates phorbol myristate acetate-induced differentiation of SH-SY5Y neuroblastoma cells. J Neurochem 2015;133 (2):223–32.
Tamás P, Hawley SA, Clarke RG, et al. Regulation of the energy sensor AMP-activated protein kinase by antigen receptor and Ca2+ in T lymphocytes. J Exp Med. 2006 Jul 10;203(7):1665-70.
Blagih J, Coulombe F, Vincent EE, et al. The energy sensor AMPK regulates T cell metabolic adaptation and effector responses in vivo. Immunity. 2015 Jan 20;42(1):41-54.
Rao E, Zhang Y, Zhu G, et al. Deficiency of AMPK in CD8+ T cells suppresses their anti-tumor function by inducing protein phosphatase-mediated cell death. Oncotarget. 2015 Apr 10;6(10):7944-58.
Cheng F, Ramos da Silva S, Huang IC, Jung JU, Gao SJ. Suppression of Zika virus infection and replication in endothelial cells and astrocytes by PKA inhibitor PKI 14-22. J Virol. 2017 Dec 6. pii: JVI.02019-17.
Soto-Acosta R, Bautista-Carbajal P, Cervantes-Salazar M, Angel-Ambrocio AH, Del Angel RM. DENV up-regulates the HMG-CoA reductase activity through the impairment of AMPK phosphorylation: A potential antiviral target. PLoS Pathog. 2017 Apr 6;13(4):e1006257.
Ruivo MT, Vera IM, Sales-Dias J, et al. Host AMPK Is a Modulator of Plasmodium Liver Infection. Cell Rep. 2016 Sep 6;16(10):2539-45.
Kajiwara C, Kusaka Y, Kimura S, et al. Metformin Mediates Protection against Legionella Pneumonia through Activation of AMPK and Mitochondrial Reactive Oxygen Species. J Immunol. 2017 Dec 15. pii: ji1700474.
G.M. Chew, D.C. Chow, S.A. Souza, et al. Impact of adjunctive metformin therapy on T cell exhaustion and viral persistence in a clinical trial of HIV-infected adults on suppressive ART. Journal of Virus Eradication 2017; 3 (Supplement 1): 6–19.
http://viruseradication.com/abstract-details.php?abstract_id=1188, last accessed March 14, 2018.
Oh-hora M, Rao A. Calcium signaling in lymphocytes. Curr Opin Immunol 2008;20(3):250–8.
Sena LA, Li S, Jairaman A, et al. Mitochondria are required for antigen-specific T cell activation through reactive oxygen species signaling. Immunity. 2013 Feb 21;38(2):225-36.
Gabriel D, Gordon LB, Djabali K. Temsirolimus Partially Rescues the Hutchinson-Gilford Progeria Cellular Phenotype. PLoS One. 2016 Dec 29;11(12):e0168988.
Chen J, Hudson E, Chi MM, et al. AMPK regulation of mouse oocyte meiotic resumption in vitro. Dev Biol. 2006 Mar 15;291(2):227-38.
LaRosa C, Downs SM. Stress stimulates AMP-activated protein kinase and meiotic resumption in mouse oocytes. Biol Reprod. 2006 Mar;74(3):585-92.
Uranga JA, Pedersen RA, Arechaga J. Parthenogenetic activation of mouse oocytes using calcium ionophores and protein kinase C stimulators. Int J Dev Biol. 1996 Apr;40(2):515-9.
Deemeh MR, Tavalaee M, Nasr-Esfahani MH. Health of children born through artificial oocyte activation: a pilot study. Reprod Sci. 2015 Mar;22(3):322-8.
Calle-Guisado V, de Llera AH, Martin-Hidalgo D, et al. AMP-activated kinase in human spermatozoa: identification, intracellular localization, and key function in the regulation of sperm motility. Asian J Androl. 2017 Nov-Dec;19(6):707-714.
Sánchez-Cárdenas C, Servín-Vences MR, José O, Treviño CL, Hernández-Cruz A, Darszon A. Acrosome reaction and Ca²+ imaging in single human spermatozoa: new regulatory roles of [Ca²+]i. Biol Reprod. 2014 Sep;91(3):67.
Liu WC, Slusarchyk DS, Astle G, Trejo WH, Brown WE, Meyers E. Ionomycin, a new polyether antibiotic. J Antibiot (Tokyo). 1978 Sep;31(9):815-9.
Kitani S, Miyamoto KT, Takamatsu S, et al. Avenolide, a Streptomyces hormone controlling antibiotic production in Streptomyces avermitilis. Proc Natl Acad Sci U S A. 2011 Sep 27;108(39):16410-5.
Meng L, Li M, Yang SH, Kim TJ, Suh JW. Intracellular ATP levels affect secondary metabolite production in Streptomyces spp. Biosci Biotechnol Biochem. 2011;75(8):1576-81.
Wang D, Wei L, Zhang Y, Zhang M, Gu S. Physicochemical and microbial responses of Streptomyces natalensis HW-2 to fungal elicitor. Appl Microbiol Biotechnol. 2017 Sep;101(17):6705-6712.
Potter WB, O'Riordan KJ, Barnett D, et al. Metabolic regulation of neuronal plasticity by the energy sensor AMPK. PLoS One. 2010 Feb 1;5(2):e8996.
Sample V, Ramamurthy S, Gorshkov K, Ronnett GV, Zhang J. Polarized activities of AMPK and BRSK in primary hippocampal neurons. Mol Biol Cell. 2015 May 15;26(10):1935-46
Terunuma M, Vargas KJ, Wilkins ME, et al. Prolonged activation of NMDA receptors promotes dephosphorylation and alters postendocytic sorting of GABAB receptors. Proc Natl Acad Sci U S A. 2010 Aug 3;107(31):13918-23.
Ganor Y, Besser M, Ben-Zakay N, Unger T, Levite M. Human T cells express a functional ionotropic glutamate receptor GluR3, and glutamate by itself triggers integrin-mediated adhesion to laminin and fibronectin and chemotactic migration. J Immunol. 2003 Apr 15;170(8):4362-72.
Miglio G, Varsaldi F, Lombardi G. Human T lymphocytes express N-methyl-D-aspartate receptors functionally active in controlling T cell activation. Biochem Biophys Res Commun. 2005 Dec 30;338(4):1875-83.
Wang G, Amato S, Gilbert J, Man HY. Resveratrol up-regulates AMPA receptor expression via AMP-activated protein kinase-mediated protein translation. Neuropharmacology. 2015 Aug;95:144-53.
Kim EC, Lee MJ, Shin SY, et al. Phorbol 12-Myristate 13-Acetate Enhances Long-Term Potentiation in the Hippocampus through Activation of Protein Kinase Cδ and ε. Korean J Physiol Pharmacol. 2013 Feb;17(1):51-6.
Klann E. Cell-permeable scavengers of superoxide prevent long-term potentiation in hippocampal area CA1. J Neurophysiol. 1998 Jul;80(1):452-7.
Asadbegi M, Yaghmaei P, Salehi I, Ebrahim-Habibi A, Komaki A. Neuroprotective effects of metformin against Aβ-mediated inhibition of long-term potentiation in rats fed a high-fat diet. Brain Res Bull. 2016 Mar;121:178-85.
Muñoz-López M, García-Pérez JL. DNA transposons: nature and applications in genomics. Curr Genomics. 2010 Apr;11(2):115-28.
Lander ES, Linton LM, Birren B, et al. Initial sequencing and analysis of the human genome. Nature. 2001 Feb 15;409(6822):860-921.
McClintock B. The significance of responses of the genome to challenge. Science. 1984 Nov 16;226(4676):792-801.
Stanley D, Fraser S, Stanley GA, Chambers PJ. Retrotransposon expression in ethanol-stressed Saccharomyces cerevisiae. Appl Microbiol Biotechnol. 2010 Jul;87(4):1447-54.
Vasilyeva LA, Bubenshchikova EV, Ratner VA. Heavy heat shock induced retrotransposon transposition in Drosophila. Genet Res. 1999 Oct;74(2):111-9.
Farkash EA, Kao GD, Horman SR, Prak ET. Gamma radiation increases endonuclease-dependent L1 retrotransposition in a cultured cell assay. Nucleic Acids Res. 2006 Feb 28;34(4):1196-204.
Magun BE, Rodland KD. Transient inhibition of protein synthesis induces the immediate early gene VL30: alternative mechanism for thapsigargin-induced gene expression. Cell Growth Differ. 1995 Jul;6(7):891-7.
Georgiou I, Noutsopoulos D, Dimitriadou E, et al. Retrotransposon RNA expression and evidence for retrotransposition events in human oocytes. Hum Mol Genet. 2009 Apr 1;18(7):1221-8.
Lazaros L, Kitsou C, Kostoulas C, et al. Retrotransposon expression and incorporation of cloned human and mouse retroelements in human spermatozoa. Fertil Steril. 2017 Mar;107(3):821-830.
Coufal NG, Garcia-Perez JL, Peng GE, et al. L1 retrotransposition in human neural progenitor cells. Nature. 2009 Aug 27;460(7259):1127-31.
Luo YB, Zhang L, Lin ZL, et al. Distinct subcellular localization and potential role of LINE1-ORF1P in meiotic oocytes. Histochem Cell Biol. 2016 Jan;145(1):93-104.
Bachiller S, Del-Pozo-Martín Y, Carrión ÁM. L1 retrotransposition alters the hippocampal genomic landscape enabling memory formation. Brain Behav Immun. 2017 Aug;64:65-70.
Ahmed S, Mahmood Z, Javed A, et al. Effect of Metformin on Adult Hippocampal Neurogenesis: Comparison with Donepezil and Links to Cognition. J Mol Neurosci. 2017 May;62(1):88-98.
Karnewar S, Neeli PK, Panuganti D, et al. Metformin regulates mitochondrial biogenesis and senescence through AMPK mediated H3K79 methylation: Relevance in age-associated vascular dysfunction. Biochim Biophys Acta. 2018 Apr;1864(4 Pt A):1115-1128.
Um JH, Brown AL, Singh SK, et al. Metabolic sensor AMPK directly phosphorylates RAG1 protein and regulates V(D)J recombination. Proc Natl Acad Sci U S A. 2013 Jun 11;110(24):9873-8.
O'Sullivan RJ, Karlseder J. Telomeres: protecting chromosomes against genome instability. Nat Rev Mol Cell Biol. 2010 Mar;11(3):171-81.
Cohen SB, Graham ME, Lovrecz GO, Bache N, Robinson PJ, Reddel RR. Protein composition of catalytically active human telomerase from immortal cells. Science. 2007 Mar 30;315(5820):1850-3.
Li Y, Zhou G, Bruno IG, Cooke JP. Telomerase mRNA Reverses Senescence in Progeria Cells. J Am Coll Cardiol. 2017 Aug 8;70(6):804-805.
#metformin#ampk#nobel prize#telomere#Telomerase#brain#progeria#fertilization#learning#memory#hippocampus
0 notes
Text
Metformin & AMPK Link Nobel Prize-winning Telomeres & Jumping Genes with Learning, HIV, & the Creation of Human Life

Nobel Prize winners, from left to right: Elizabeth Blackburn (discovered telomerase), Barbara McClintock (discovered “jumping genes”), and Françoise Barré-Sinoussi (discovered HIV). By Science History Institute, CC BY-SA 3.0, https://commons.wikimedia.org/w/index.php?curid=30112731; By Smithsonian Institution/Science Service; Restored by Adam Cuerden - Flickr: Barbara McClintock (1902-1992), Public Domain, https://commons.wikimedia.org/w/index.php?curid=25629182; By Prolineserver (talk) - Own work, GFDL 1.2, https://commons.wikimedia.org/w/index.php?curid=5395403
A recently published study in the journal Biochimica et Biophysica Acta (BBA) - Molecular Basis of Disease in 2018 demonstrated for the first time that chronic treatment with the anti-diabetic drug metformin activated human telomerase in human aortic endothelial cells (HAECs) and significantly delayed endothelial senescence in an AMPK-dependent manner [11]. Telomeres are specialized regions of repetitive nucleotide sequences located at the ends of eukaryotic chromosomes that protect chromosomal ends from deterioration [63]. However, continuous cell division leads to telomere shortening, impeding the replenishment of tissues and triggering cellular senescence (i.e. cells cease to divide). Although human telomeres shorten with age, telomeres may be lengthened by the enzyme telomerase [64].
This study substantiates and confirms several novel proposals in a recently published paper I authored in April of 2018 in which I first proposed that because telomerase is derived from a “jumping gene” (see below for discussion), metformin would activate telomerase via AMPK [6]. My paper also highlights a novel link between hippocampal long-term potentiation (essential for learning and memory), alleviation of accelerated cellular aging in Hutchinson-Gilford progeria syndrome, oocyte activation and the sperm acrosome reaction (prerequisites for human life creation), and transposable element (i.e. “jumping genes”)-mediated promotion of learning, memory, and the creation of human life [1-7]. Indeed, these novel proposals also link several Nobel Prize-winning discoveries, including the discovery of telomerase by Elizabeth Blackburn (photo-left), the discovery of “jumping genes” by Barbara McClintock (photo-middle), and the discovery of HIV by Françoise Barré-Sinoussi (photo-right).
The link between such disparate physiological and pathophysiological phenomena is cellular stress-induced modulation of energy metabolism, leading to the activation of the master metabolic regulator AMPK, a kinase that increases lifespan and healthspan in several model organisms [12]. I was the first to propose and publish (2014) that an increase in beneficial levels of cellular stress (e.g. increases in the levels reactive oxygen species [ROS], calcium [Ca2+], and/or an AMP(ADP)/ATP ratio increase, etc.) and activation of AMPK by compounds including metformin would alleviate accelerated cellular aging defects in children diagnosed with the genetic disorder Hutchinson-Gilford progeria syndrome (HGPS), a disease characterized by an accelerated aging phenotype and death at ~14.6 years of age [1]. This hypothesis was substantiated in 2016 and 2017, with metformin activating AMPK in cells taken from HGPS kids and ameliorating accelerated cellular aging defects (e.g. correcting nuclear morphology, decrease in senescence markers, etc.) [8,9]. Additionally, transfection of telomerase been shown to reverse senescence in HGPS cells [65]. As metformin activates telomerase and normal humans make the same toxic protein (called progerin) that leads to accelerated aging in HGPS kids (just at lower amounts that accumulate with age), AMPK activation may also play a significant role in ameliorating diseases associated with physiological aging [10,11].
AMPK also links HGPS with potential virus eradication. I first proposed in 2015 that AMPK activation links alleviation of accelerated aging in HGPS with the potential eradication of HIV-1 via the “shock and kill” approach, a method currently being pursued by HIV cure researchers to possibly eradicate HIV-1 [2,13]. The same gene splicing factor that promotes accelerated aging in HGPS (called SRSF1 or ASF/SF2) also inhibits reactivation of latent HIV-1 (i.e. “shock”), preventing immune system detection and virus destruction (i.e. “kill”) [8,14]. Metformin was shown to slow aging in HGPS cells by decreasing this splicing factor, as I originally predicted in 2014, and several compounds that potently induce latent HIV-1 reactivation in T cells from infected patients, including PMA (a phorbol ester) combined with ionomycin, each activate AMPK [1,8,15-17]. AMPKα1 deletion leads to a decrease in primary T cell responses to bacterial and viral infections in vivo, AMPK knockdown leads to cell death on T cell activation, and metformin has recently been shown to inhibit Zika and Dengue viruses, the malaria parasite, and Legionella pneumophila [18-23]. Intriguingly, early data presented at the International AIDS Conference in 2017 demonstrated that metformin destabilized the latent HIV-1 reservoir in chronically-infected HIV patients and decreased the percentage of CD4+ T cells expressing the immune checkpoint receptors PD-1, TIGIT, and TIM-3, each markers associated with T cells latently infected with HIV-1, indicating that AMPK activation may indeed contribute to a cure for HIV-1 [24,25].
As I first proposed in 2016 and 2017, the induction of cellular stress and AMPK activation also links HGPS and potential HIV-1 eradication with oocyte activation and the sperm acrosome reaction, prerequisites for the creation of human life [3,4]. Increases in both ROS and Ca2+ are critical for T cell activation (and hence latent HIV-1 reactivation) and ROS is transiently increased in HGPS cells when treated with a rapamycin analog to alleviate accelerated aging [26-28]. Stress-induced activation of AMPK by AICAR and other compounds promotes oocyte maturation, which precedes and is essential for efficient oocyte activation [29,30]. Oocyte activation is indispensable for the creation of all human life and PMA and ionomycin, which collectively reactivates latent HIV-1, activates mouse and human oocytes, respectively [31,32]. AMPK is also found in the acrosome of the human sperm head and ionomycin induces the acrosome reaction in human sperm, a process necessary for oocyte penetration and fertilization [33,34]. Ionomycin is also used extensively during fertility procedures to activate human oocytes (i.e. “shock”), creating normal, healthy children (i.e. “live”) [32]. Interestingly, ionomycin is a narrow spectrum antibiotic produced by certain species within the bacterial genus Streptomyces, from which ~70 percent of clinically useful antibiotics are derived [35,36]. Cellular stress, mediated by increases in ROS, Ca2+, and/or an AMP(ADP)/ATP ratio increase, etc. also enhances antibiotic production in many Streptomyces strains, reinforcing the notion that the beneficial effects of cellular stress induction crosses species boundaries [37,38].
Cellular stress induction and AMPK activation also link HGPS, potential HIV-1 eradication, and human life creation with learning and memory, a hypothesis I originally proposed in 2018 [6]. Hippocampal long-term potentiation (LTP) is considered the cellular correlate of learning and memory and AMPK has been found localized in hippocampal CA1 dendrites and is activated in neurons by metformin, AICAR, ionomycin, and glutamate, a neurotransmitter essential for hippocampal LTP induction [39-41]. The glutamate receptors AMPAR and NMDAR are found on and modulate T cell activation, AMPK activation increases synthesis and membrane insertion of AMPARs (critical for LTP expression), PMA enhances hippocampal CA1 LTP, and inhibition of ROS significantly impairs hippocampal CA1 LTP [42-46]. Also, neuronal depolarization decreases the recruitment efficiency of SRSF1 to nascent RNAs and promotes SRSF1 nuclear speckle accumulation [6]. SRSF1, a gene splicing factor that is inhibited by metformin, enhances progerin production in HGPS cells and prevents latent HIV-1 reactivation [2,8]. Metformin also significantly reduces pathology-associated reductions in LTP in animal models in vivo, indicating that learning and memory are linked to HGPS, potential HIV-1 eradication, and human life creation via the induction of beneficial levels of cellular stress [47].
Lastly, cellular stress and AMPK activation also links the activation and mobilization of transposable elements (i.e. “jumping genes”) with telomerase activation, potential HIV-1 eradication, learning and memory, and the creation of human life, a hypothesis I originally proposed in 2018 [6]. Transposable elements (TEs) are DNA sequences first described by Nobel laureate Barbara McClintock that comprise nearly half of the human genome, are able to transpose or move from one genomic location to another, and have played an extensive role in human genome evolution [48-50]. Strikingly, McClintock also described in her Nobel Prize speech that a genome “shock” seemed to promote TE activation and mobilization [50]. As first noted in my recently published paper, this “shock” is the same “shock” that HIV cure researchers are using during the “shock and kill” approach to reactivate latent HIV-1 to potentially effectuate a cure [6]. Indeed, several forms of cellular stress, including heat shock and radiation, have been convincingly shown to activate and enhance TE mobilization in several model organisms and in human cells [51-53]. This same “shock” McClintock referred to, mediated by increases in ROS, Ca2+, and/or an AMP(ADP)/ATP ratio, etc. is also what leads to the creation of human life, as the antibiotic ionomycin activates AMPK, promotes TE activation, and induces human oocyte activation [17,32,54]. LINE-1 (L1), a member of the retrotransposon class of TEs, is active and capable of mobilization in human oocytes, human sperm, and in human neural progenitor cells [55-57]. Inhibition of L1 impairs both oocyte maturation in vitro and long-term memory formation in vivo in mice [58,59]. L1 has also been detected in the human brain and is capable of mobilization in human neurons [57]. As noted above, AMPK is critical for oocyte maturation and metformin promotes hippocampal neurogenesis and spatial memory formation [29,60]. The landmark initial sequencing of the human genome also noted that both telomerase and RAG1 (promotes DNA cleavage and transposition in human cells) are derived from TEs [49]. Because metformin activates both telomerase and RAG1 via AMPK, it is likely that cellular stress-induced AMPK activation facilitates beneficial TE activation and mobilization (i.e. learning and memory associated with L1 mobilization), linking human genome evolution and the creation of human life with hippocampal LTP, HGPS, and potential HIV-1 eradication [61,62].
https://www.linkedin.com/pulse/metformin-ampk-link-nobel-prize-winning-telomeres-jumping-finley/


References
Finley J. Alteration of splice site selection in the LMNA gene and inhibition of progerin production via AMPK activation. Med Hypotheses. 2014 Nov;83(5):580-7.
Finley J. Reactivation of latently infected HIV-1 viral reservoirs and correction of aberrant alternative splicing in the LMNA gene via AMPK activation: Common mechanism of action linking HIV-1 latency and Hutchinson-Gilford progeria syndrome. Med Hypotheses. 2015 Sep;85(3):320-32.
Finley J. Oocyte activation and latent HIV-1 reactivation: AMPK as a common mechanism of action linking the beginnings of life and the potential eradication of HIV-1. Med Hypotheses. 2016 Aug;93:34-47.
Finley J. Elimination of cancer stem cells and reactivation of latent HIV-1 via AMPK activation: Common mechanism of action linking inhibition of tumorigenesis and the potential eradication of HIV-1. Med Hypotheses. 2017 Jul;104:133-146.
Finley J. AMPK activation as a common mechanism of action linking the effects of diverse compounds that ameliorate accelerated cellular aging defects in Hutchinson-Gilford progeria syndrome. Med Hypotheses. Manuscript submitted.
Finley J. Facilitation of hippocampal long-term potentiation and reactivation of latent HIV-1 via AMPK activation: Common mechanism of action linking learning, memory, and the potential eradication of HIV-1. Med Hypotheses. 2018 Jul;116: 61-73.
Finley J. Transposable elements, placental development, and oocyte activation: Cellular stress and AMPK links jumping genes with the creation of human life. Med Hypotheses. Under Review.
Egesipe AL, Blondel S, Cicero AL, et al. Metformin decreases progerin expression and alleviates pathological defects of Hutchinson-Gilford progeria syndrome cells. NPJ Aging Mech Dis. 2016 Nov 10;2:16026.
Park SK, Shin OS. Metformin alleviates ageing cellular phenotypes in Hutchinson-Gilford progeria syndrome dermal fibroblasts. Exp Dermatol. 2017 Oct;26(10):889-895.
McClintock D, Ratner D, Lokuge M, et al. The mutant form of lamin A that causes Hutchinson-Gilford progeria is a biomarker of cellular aging in human skin. PLoS One. 2007 Dec 5;2(12):e1269.
Karnewar S, Neeli PK, Panuganti D, et al. Metformin regulates mitochondrial biogenesis and senescence through AMPK mediated H3K79 methylation: Relevance in age-associated vascular dysfunction. Biochim Biophys Acta. 2018 Apr;1864(4 Pt A):1115-1128.
Salminen A, Kaarniranta K. AMP-activated protein kinase (AMPK) controls the aging process via an integrated signaling network. Ageing Res Rev. 2012 Apr;11(2):230-41.
Kim Y, Anderson JL, Lewin SR. Getting the "Kill" into "Shock and Kill": Strategies to Eliminate Latent HIV. Cell Host Microbe. 2018 Jan 10;23(1):14-26.
Berro R, Kehn K, de la Fuente C, et al. Acetylated Tat regulates human immunodeficiency virus type 1 splicing through its interaction with the splicing regulator p32. J Virol 2006;80(7):3189–204.
Spina CA, Anderson J, Archin NM, et al. An in-depth comparison of latent HIV-1 reactivation in multiple cell model systems and resting CD4+ T cells from aviremic patients. PLoS Pathog 2013;9(12):e1003834.
Zogovic N, Tovilovic-Kovacevic G, Misirkic-Marjanovic M, et al. Coordinated activation of AMP-activated protein kinase, extracellular signal-regulated kinase, and autophagy regulates phorbol myristate acetate-induced differentiation of SH-SY5Y neuroblastoma cells. J Neurochem 2015;133 (2):223–32.
Tamás P, Hawley SA, Clarke RG, et al. Regulation of the energy sensor AMP-activated protein kinase by antigen receptor and Ca2+ in T lymphocytes. J Exp Med. 2006 Jul 10;203(7):1665-70.
Blagih J, Coulombe F, Vincent EE, et al. The energy sensor AMPK regulates T cell metabolic adaptation and effector responses in vivo. Immunity. 2015 Jan 20;42(1):41-54.
Rao E, Zhang Y, Zhu G, et al. Deficiency of AMPK in CD8+ T cells suppresses their anti-tumor function by inducing protein phosphatase-mediated cell death. Oncotarget. 2015 Apr 10;6(10):7944-58.
Cheng F, Ramos da Silva S, Huang IC, Jung JU, Gao SJ. Suppression of Zika virus infection and replication in endothelial cells and astrocytes by PKA inhibitor PKI 14-22. J Virol. 2017 Dec 6. pii: JVI.02019-17.
Soto-Acosta R, Bautista-Carbajal P, Cervantes-Salazar M, Angel-Ambrocio AH, Del Angel RM. DENV up-regulates the HMG-CoA reductase activity through the impairment of AMPK phosphorylation: A potential antiviral target. PLoS Pathog. 2017 Apr 6;13(4):e1006257.
Ruivo MT, Vera IM, Sales-Dias J, et al. Host AMPK Is a Modulator of Plasmodium Liver Infection. Cell Rep. 2016 Sep 6;16(10):2539-45.
Kajiwara C, Kusaka Y, Kimura S, et al. Metformin Mediates Protection against Legionella Pneumonia through Activation of AMPK and Mitochondrial Reactive Oxygen Species. J Immunol. 2017 Dec 15. pii: ji1700474.
G.M. Chew, D.C. Chow, S.A. Souza, et al. Impact of adjunctive metformin therapy on T cell exhaustion and viral persistence in a clinical trial of HIV-infected adults on suppressive ART. Journal of Virus Eradication 2017; 3 (Supplement 1): 6–19.
http://viruseradication.com/abstract-details.php?abstract_id=1188, last accessed March 14, 2018.
Oh-hora M, Rao A. Calcium signaling in lymphocytes. Curr Opin Immunol 2008;20(3):250–8.
Sena LA, Li S, Jairaman A, et al. Mitochondria are required for antigen-specific T cell activation through reactive oxygen species signaling. Immunity. 2013 Feb 21;38(2):225-36.
Gabriel D, Gordon LB, Djabali K. Temsirolimus Partially Rescues the Hutchinson-Gilford Progeria Cellular Phenotype. PLoS One. 2016 Dec 29;11(12):e0168988.
Chen J, Hudson E, Chi MM, et al. AMPK regulation of mouse oocyte meiotic resumption in vitro. Dev Biol. 2006 Mar 15;291(2):227-38.
LaRosa C, Downs SM. Stress stimulates AMP-activated protein kinase and meiotic resumption in mouse oocytes. Biol Reprod. 2006 Mar;74(3):585-92.
Uranga JA, Pedersen RA, Arechaga J. Parthenogenetic activation of mouse oocytes using calcium ionophores and protein kinase C stimulators. Int J Dev Biol. 1996 Apr;40(2):515-9.
Deemeh MR, Tavalaee M, Nasr-Esfahani MH. Health of children born through artificial oocyte activation: a pilot study. Reprod Sci. 2015 Mar;22(3):322-8.
Calle-Guisado V, de Llera AH, Martin-Hidalgo D, et al. AMP-activated kinase in human spermatozoa: identification, intracellular localization, and key function in the regulation of sperm motility. Asian J Androl. 2017 Nov-Dec;19(6):707-714.
Sánchez-Cárdenas C, Servín-Vences MR, José O, Treviño CL, Hernández-Cruz A, Darszon A. Acrosome reaction and Ca²+ imaging in single human spermatozoa: new regulatory roles of [Ca²+]i. Biol Reprod. 2014 Sep;91(3):67.
Liu WC, Slusarchyk DS, Astle G, Trejo WH, Brown WE, Meyers E. Ionomycin, a new polyether antibiotic. J Antibiot (Tokyo). 1978 Sep;31(9):815-9.
Kitani S, Miyamoto KT, Takamatsu S, et al. Avenolide, a Streptomyces hormone controlling antibiotic production in Streptomyces avermitilis. Proc Natl Acad Sci U S A. 2011 Sep 27;108(39):16410-5.
Meng L, Li M, Yang SH, Kim TJ, Suh JW. Intracellular ATP levels affect secondary metabolite production in Streptomyces spp. Biosci Biotechnol Biochem. 2011;75(8):1576-81.
Wang D, Wei L, Zhang Y, Zhang M, Gu S. Physicochemical and microbial responses of Streptomyces natalensis HW-2 to fungal elicitor. Appl Microbiol Biotechnol. 2017 Sep;101(17):6705-6712.
Potter WB, O'Riordan KJ, Barnett D, et al. Metabolic regulation of neuronal plasticity by the energy sensor AMPK. PLoS One. 2010 Feb 1;5(2):e8996.
Sample V, Ramamurthy S, Gorshkov K, Ronnett GV, Zhang J. Polarized activities of AMPK and BRSK in primary hippocampal neurons. Mol Biol Cell. 2015 May 15;26(10):1935-46
Terunuma M, Vargas KJ, Wilkins ME, et al. Prolonged activation of NMDA receptors promotes dephosphorylation and alters postendocytic sorting of GABAB receptors. Proc Natl Acad Sci U S A. 2010 Aug 3;107(31):13918-23.
Ganor Y, Besser M, Ben-Zakay N, Unger T, Levite M. Human T cells express a functional ionotropic glutamate receptor GluR3, and glutamate by itself triggers integrin-mediated adhesion to laminin and fibronectin and chemotactic migration. J Immunol. 2003 Apr 15;170(8):4362-72.
Miglio G, Varsaldi F, Lombardi G. Human T lymphocytes express N-methyl-D-aspartate receptors functionally active in controlling T cell activation. Biochem Biophys Res Commun. 2005 Dec 30;338(4):1875-83.
Wang G, Amato S, Gilbert J, Man HY. Resveratrol up-regulates AMPA receptor expression via AMP-activated protein kinase-mediated protein translation. Neuropharmacology. 2015 Aug;95:144-53.
Kim EC, Lee MJ, Shin SY, et al. Phorbol 12-Myristate 13-Acetate Enhances Long-Term Potentiation in the Hippocampus through Activation of Protein Kinase Cδ and ε. Korean J Physiol Pharmacol. 2013 Feb;17(1):51-6.
Klann E. Cell-permeable scavengers of superoxide prevent long-term potentiation in hippocampal area CA1. J Neurophysiol. 1998 Jul;80(1):452-7.
Asadbegi M, Yaghmaei P, Salehi I, Ebrahim-Habibi A, Komaki A. Neuroprotective effects of metformin against Aβ-mediated inhibition of long-term potentiation in rats fed a high-fat diet. Brain Res Bull. 2016 Mar;121:178-85.
Muñoz-López M, García-Pérez JL. DNA transposons: nature and applications in genomics. Curr Genomics. 2010 Apr;11(2):115-28.
Lander ES, Linton LM, Birren B, et al. Initial sequencing and analysis of the human genome. Nature. 2001 Feb 15;409(6822):860-921.
McClintock B. The significance of responses of the genome to challenge. Science. 1984 Nov 16;226(4676):792-801.
Stanley D, Fraser S, Stanley GA, Chambers PJ. Retrotransposon expression in ethanol-stressed Saccharomyces cerevisiae. Appl Microbiol Biotechnol. 2010 Jul;87(4):1447-54.
Vasilyeva LA, Bubenshchikova EV, Ratner VA. Heavy heat shock induced retrotransposon transposition in Drosophila. Genet Res. 1999 Oct;74(2):111-9.
Farkash EA, Kao GD, Horman SR, Prak ET. Gamma radiation increases endonuclease-dependent L1 retrotransposition in a cultured cell assay. Nucleic Acids Res. 2006 Feb 28;34(4):1196-204.
Magun BE, Rodland KD. Transient inhibition of protein synthesis induces the immediate early gene VL30: alternative mechanism for thapsigargin-induced gene expression. Cell Growth Differ. 1995 Jul;6(7):891-7.
Georgiou I, Noutsopoulos D, Dimitriadou E, et al. Retrotransposon RNA expression and evidence for retrotransposition events in human oocytes. Hum Mol Genet. 2009 Apr 1;18(7):1221-8.
Lazaros L, Kitsou C, Kostoulas C, et al. Retrotransposon expression and incorporation of cloned human and mouse retroelements in human spermatozoa. Fertil Steril. 2017 Mar;107(3):821-830.
Coufal NG, Garcia-Perez JL, Peng GE, et al. L1 retrotransposition in human neural progenitor cells. Nature. 2009 Aug 27;460(7259):1127-31.
Luo YB, Zhang L, Lin ZL, et al. Distinct subcellular localization and potential role of LINE1-ORF1P in meiotic oocytes. Histochem Cell Biol. 2016 Jan;145(1):93-104.
Bachiller S, Del-Pozo-Martín Y, Carrión ÁM. L1 retrotransposition alters the hippocampal genomic landscape enabling memory formation. Brain Behav Immun. 2017 Aug;64:65-70.
Ahmed S, Mahmood Z, Javed A, et al. Effect of Metformin on Adult Hippocampal Neurogenesis: Comparison with Donepezil and Links to Cognition. J Mol Neurosci. 2017 May;62(1):88-98.
Karnewar S, Neeli PK, Panuganti D, et al. Metformin regulates mitochondrial biogenesis and senescence through AMPK mediated H3K79 methylation: Relevance in age-associated vascular dysfunction. Biochim Biophys Acta. 2018 Apr;1864(4 Pt A):1115-1128.
Um JH, Brown AL, Singh SK, et al. Metabolic sensor AMPK directly phosphorylates RAG1 protein and regulates V(D)J recombination. Proc Natl Acad Sci U S A. 2013 Jun 11;110(24):9873-8.
O'Sullivan RJ, Karlseder J. Telomeres: protecting chromosomes against genome instability. Nat Rev Mol Cell Biol. 2010 Mar;11(3):171-81.
Cohen SB, Graham ME, Lovrecz GO, Bache N, Robinson PJ, Reddel RR. Protein composition of catalytically active human telomerase from immortal cells. Science. 2007 Mar 30;315(5820):1850-3.
Li Y, Zhou G, Bruno IG, Cooke JP. Telomerase mRNA Reverses Senescence in Progeria Cells. J Am Coll Cardiol. 2017 Aug 8;70(6):804-805.
0 notes
Text
Metformin shown for the first time to activate Telomere enzyme Telomerase in human cells via AMPK: Link between Progeria and HIV
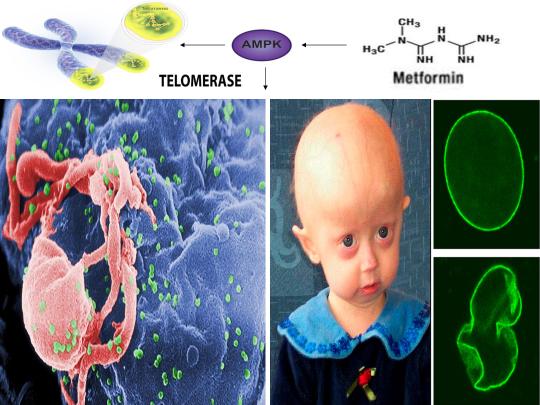
Goldsmith Content Providers: CDC/ C. Goldsmith, P. Feorino, E. L. Palmer, W. R. McManus [Public domain], via Wikimedia Commons;The Cell Nucleus and Aging: Tantalizing Clues and Hopeful Promises. Scaffidi P, Gordon L, Misteli T. PLoS Biology Vol. 3/11/2005, e39
A recently published study in the journal Biochimica et Biophysica Acta (BBA) - Molecular Basis of Disease in 2018 demonstrated for the first time that chronic treatment with the anti-diabetic drug metformin activated human telomerase reverse transcriptase (hTERT) in human aortic endothelial cells (HAECs) and significantly delayed endothelial senescence in an AMPK-dependent manner [1]. AMPK is activated by the induction of cellular stress, mediated by increases in intracellular calcium (Ca2+), reactive oxygen species (ROS), and/or an AMP(ADP)/ATP ratio increase, etc. [41]. Telomeres are specialized regions of repetitive nucleotide sequences located at the ends of eukaryotic chromosomes that protect chromosomal ends from deterioration [2]. However, continuous cell division leads to telomere shortening, impeding the replenishment of tissues and triggering cellular senescence (i.e. cells cease to divide). Although human telomeres shorten with age, telomeres may be lengthened by the enzyme telomerase, a ribonucleoprotein that consists of the catalytic subunit hTERT, telomerase RNA, and the nucleolar protein dyskerin [2,3]. hTERT, which is considering limiting for telomerase activity, is a protein that exhibits reverse transcriptase activity and synthesizes telomeric DNA from an RNA template [4].
The authors of the study initially demonstrated that both metformin and the AMPK activator AICAR significantly increased hTERT levels and enhanced AMPK activation in human aortic endothelial cells (HAECs) [1]. Importantly, inhibition or knockdown of AMPK with compound C or siAMPKα inhibited the metformin-induced increase in hTERT while metformin failed to reverse siAMPKα-induced senescence in HAECs, indicating that hTERT expression is regulated by AMPK activation in endothelial cells. Indeed, continuous culturing of HAECs in the presence of metformin significantly increased hTERT protein levels and activity, reduced the expression of the senescence markers p53, p21, p27, and p16, and reduced senescence-associated beta-galactosidase (SA-β-gal, a biomarker of cellular senescence) staining in HAECs, again indicating that metformin delays cellular senescence and increases hTERT levels via AMPK activation [1]. Strikingly, using ApoE-/- mice (which spontaneously develop atherosclerosis and age faster compared to normal mice), the authors also showed that chronic low-dose metformin administration for fourteen months in drinking water enhanced the levels of activated AMPK and Pgc-1α observed in the endothelial layer of the aorta [1]. Metformin also increased the transcript and protein levels of Tert, decreased senescence markers (p16, p21, p27, p53) in the total aortic homogenate, and significantly reduced SA-β-gal staining of aorta compared to untreated ApoE-/- mice, demonstrating that metformin-induced AMPK activation delays vascular aging and protects from age-associated atherosclerosis in ApoE-/- mice [1].
Interestingly, telomere length has been shown to be significantly reduced in cells derived from patients with the accelerated aging disorder Hutchinson-Gilford progeria syndrome (HGPS) and telomere shortening in normal cells that occurs during cellular senescence activates progerin production, a toxic protein that leads to an accelerating aging phenotype in children with HGPS via aberrant alternative splicing of the LMNA gene [5]. Normal lamin A plays a critical role in supporting nuclear architecture and morphology. Lamin A binding to subtelomeric repeats also localizes telomeres to the nuclear periphery and loss of lamin A leads to defects in telomeric heterochromatin, altered nuclear distribution and shortening of telomeres, inefficient processing of dysfunctional telomeres by non-homologus end joining, and increased genomic instability [6]. Telomere length has been found to be significantly reduced in fibroblasts derived from HGPS patients and a recent study also confirmed that in normal human fibroblasts, progressive telomere damage that occurs during cellular senescence activates progerin production and also leads to extensive changes in alternative splicing of many other genes, highlighting a striking similarity between normal aging and accelerated aging in HGPS patients [5,7]. Indeed, transfection of HGPS fibroblasts with human telomerase (hTERT) mRNA restored cell proliferation, reduced cell loss, extended cellular lifespan, increased telomerase activity and telomere length, and reduced SA-β-gal staining compared to HGPS cells expressing catalytically inactive hTERT mRNA [8]. Because metformin also increases hTERT expression and inhibits senescence in human cells, it is likely that cellular stress-induced AMPK activation, mediated by increases in intracellular calcium (Ca2+), reactive oxygen species (ROS), and/or an AMP(ADP)/ATP ratio increase, etc., represents a central node linking structurally diverse compounds and methodologies that alleviate accelerated aging in HGPS cells.
As the splicing factor SRSF1 has been shown to increase progerin production by promoting the use of a cryptic splice located in the LMNA gene, metformin was recently shown to significantly reduce the expression of SRSF1 and progerin, activate AMPK, and improve nuclear architecture in HGPS cells, indicating that AMPK activation by metformin beneficially alters gene splicing in HGPS cells by modulating SRSF1, a hypothesis that I first proposed and published in 2014 [9-11]. Also, p32, a splicing-associated protein that is an endogenous inhibitor of SRSF1 and is critical for the maintenance of mitochondrial functionality and oxidative phosphorylation, has also been shown to be essential for rapamycin- or starvation-induced autophagy mediated by ULK1 [12,13]. Because rapamycin, also an AMPK activator in vivo, improves accelerated aging defects in HGPS cells by reducing progerin levels via induction of autophagy, AMPK activation likely also beneficially modulates the activity of p32, leading to inhibition of SRSF1 splicing activity and enhancement of mitochondrial functionality [14,15]. Moreover, PGC-1α, which is activated by AMPK and metformin and promotes telomere transcription, is downregulated in HGPS cells, leading to significant mitochondrial dysfunction [1,16,17]. Methylene blue, which activates AMPK in vivo, was shown to increase PGC-1α levels, induce progerin solubility, and alleviate accelerated aging defects in HGPS cells [16,18]. Additionally, the rapamycin analog temsirolimus alleviated accelerated aging defects in HGPS cells but transiently increased ROS and superoxide anion levels in both HGPS and normal cells within the first hour of treatment, again indicating that cellular stress-induced AMPK activation represents a common mechanism for inhibiting senescence and ameliorating symptoms associated with accelerated aging [19].
The inhibition of SRSF1 and the promotion of hTERT expression and telomere transcription by metformin via AMPK activation also link HGPS and telomere integrity with HIV-1 latency. Increased splicing activity of SRSF1 inhibits reactivation of latent HIV-1 residing in infected immune cells, preventing immune system detection and destruction of the virus [20]. Reactivation of latent HIV-1 (i.e. the “shock and kill” approach) leads to a reduction in SRSF1 but an increase in p32 activity and bryostatin-1 (a PKC modulator) has been shown to reactivate latent HIV-1 via AMPK activation [20,21]. Interestingly, p32 modulation via AMPK activation may also enhance and stabilize the splicing activities of hnRNPA1, a heteroribonuclear protein that associates with p32, antagonizes the splicing function of SRSF1, prevents splicing of the HIV-1 genome (promoting viral reactivation), and participates in the maintenance and preservation of telomeres [22-25]. hnRNPA1 is also decreased in senescent human fibroblasts and antagonizes cellular senescence and the senescence-associated secretory phenotype (SASP) via increasing SIRT1 expression [26,27]. Resveratrol, a plant-derived polyphenol that activates AMPK, increases hnRNPA1 protein expression and SIRT1, a histone deacetylase that plays a role in a number of age related diseases and in the extension of lifespan, is also activated by AMPK [28-30]. Also, T cell activation, an efficient method for reactivating latent HIV-1, is dependent on increases in intracellular Ca2+ and ROS, telomerase is transiently increased on T cell activation, and AMPK knockdown leads to T cell death during in vitro activation [31-34]. Furthermore, resveratrol reactivates latent HIV-1 and preliminary data demonstrated that metformin decreased the percentage of CD4+ T cells expressing PD-1, TIGIT, and TIM-3, each markers associated with T cells latently infected with HIV-1, in chronically-infected HIV-1 patients [35-37]. Such evidence strongly suggests that cellular stress-induced AMPK activation, mediated by increases in intracellular calcium (Ca2+), reactive oxygen species (ROS), and/or an AMP (ADP)/ATP ratio increase, etc. links the alleviation of accelerated cellular aging defects in HGPS with the potential eradication of HIV-1, a hypothesis that I first proposed in 2015 [38].
The evidence presented in the Biochimica et Biophysica Acta (BBA) - Molecular Basis of Disease publication further substantiates that AMPK activation represents a central node that connects the therapeutic benefits of chemically distinct compounds in diseases as seemingly dissimilar as HGPS and HIV-1 latency. Indeed, AMPK activators including metformin increase hTERT expression, promote telomere transcription and integrity, decrease the splicing activity of SRSF1 that increases progerin production but prevents latent HIV-1 reactivation, and potentially beneficially modulates the activity of the SRSF1 inhibitor p32 and the ribonucleoprotein hnRNPA1. As AMPK activators (e.g. ionomycin) induce human oocyte activation (giving rise to normal healthy children) and AMPK is localized throughout the entire acrosome in human sperm (likely promoting the acrosome reaction), AMPK activation links normal human aging, Progeria, and HIV-1 latency with the creation of all human life (i.e. the “shock and live” approach) [11,38-40].
https://www.linkedin.com/pulse/metformin-shown-first-time-activate-telomere-enzyme-human-finley/?published=t
References:
Karnewar S, Neeli PK, Panuganti D, et al. Metformin regulates mitochondrial biogenesis and senescence through AMPK mediated H3K79 methylation: Relevance in age-associated vascular dysfunction. Biochim Biophys Acta. 2018 Apr;1864(4 Pt A):1115-1128.
O'Sullivan RJ, Karlseder J. Telomeres: protecting chromosomes against genome instability. Nat Rev Mol Cell Biol. 2010 Mar;11(3):171-81.
Cohen SB, Graham ME, Lovrecz GO, Bache N, Robinson PJ, Reddel RR. Protein composition of catalytically active human telomerase from immortal cells. Science. 2007 Mar 30;315(5820):1850-3.
Cong YS, Wen J, Bacchetti S. The human telomerase catalytic subunit hTERT: organization of the gene and characterization of the promoter. Hum Mol Genet. 1999 Jan;8(1):137-42.
Cao K, Blair CD, Faddah DA, et al. Progerin and telomere dysfunction collaborate to trigger cellular senescence in normal human fibroblasts. J Clin Invest. 2011 Jul;121(7):2833-44.
Gonzalez-Suarez I, Redwood AB, Perkins SM, et al. Novel roles for A-type lamins in telomere biology and the DNA damage response pathway. EMBO J 2009;28(16):2414–27.
Decker ML, Chavez E, Vulto I, Lansdorp PM. Telomere length in Hutchinson-Gilford progeria syndrome. Mech Ageing Dev. 2009 Jun;130(6):377-83.
Li Y, Zhou G, Bruno IG, Cooke JP. Telomerase mRNA Reverses Senescence in Progeria Cells. J Am Coll Cardiol. 2017 Aug 8;70(6):804-805.
Egesipe AL, Blondel S1, Cicero AL, et al. Metformin decreases progerin expression and alleviates pathological defects of Hutchinson-Gilford progeria syndrome cells. NPJ Aging Mech Dis. 2016 Nov 10;2:16026.
Park SK, Shin OS. Metformin alleviates ageing cellular phenotypes in Hutchinson-Gilford progeria syndrome dermal fibroblasts. Exp Dermatol. 2017 Oct;26(10):889-895.
Finley J. Alteration of splice site selection in the LMNA gene and inhibition of progerin production via AMPK activation. Med Hypotheses. 2014 Nov;83(5):580-7.
P32/gC1qR is indispensable for fetal development and mitochondrial translation: importance of its RNA-binding ability. Nucl Acids Res 2012;40(19):9717–37.
Jiao H, Su GQ, Dong W, et al. Chaperone-like protein p32 regulates ULK1 stability and autophagy. Cell Death Differ. 2015 Nov;22(11):1812-23.
Cao K, Graziotto JJ, Blair CD, et al. Rapamycin reverses cellular phenotypes and enhances mutant protein clearance in Hutchinson-Gilford progeria syndrome cells. Sci Transl Med. 2011 Jun 29;3(89):89ra58.
Chiao YA, Kolwicz SC, Basisty N, et al. Rapamycin transiently induces mitochondrial remodeling to reprogram energy metabolism in old hearts. Aging (Albany NY). 2016 Feb;8(2):314-27.
Xiong ZM, Choi JY, Wang K, et al. Methylene blue alleviates nuclear and mitochondrial abnormalities in progeria. Aging Cell. 2016 Apr;15(2):279-90.
Diman A, Boros J, Poulain F, et al. Nuclear respiratory factor 1 and endurance exercise promote human telomere transcription. Sci Adv. 2016 Jul 27;2(7):e1600031.
Xie L, Li W, Winters A, Yuan F, Jin K, Yang S. Methylene blue induces macroautophagy through 5' adenosine monophosphate-activated protein kinase pathway to protect neurons from serum deprivation. Front Cell Neurosci. 2013 May 3;7:56.
Gabriel D, Gordon LB, Djabali K. Temsirolimus Partially Rescues the Hutchinson-Gilford Progeria Cellular Phenotype. PLoS One. 2016 Dec 29;11(12):e0168988.
Berro R, Kehn K, de la Fuente C, et al. Acetylated Tat regulates human immunodeficiency virus type 1 splicing through its interaction with the splicing regulator p32. J Virol. 2006 Apr;80(7):3189-204.
Mehla R, Bivalkar-Mehla S, Zhang R, et al. Bryostatin modulates latent HIV-1 infection via PKC and AMPK signaling but inhibits acute infection in a receptor independent manner. PLoS One. 2010 Jun 16;5(6):e11160.
Expert-Bezançon A, Sureau A, Durosay P, et al. HnRNP A1 and the SR proteins ASF/SF2 and SC35 have antagonistic functions in splicing of beta tropomyosin exon 6B. J Biol Chem 2004 Sep 10;279(37):38249–59.
LaBranche H, Dupuis S, Ben-David Y, Bani MR, Wellinger RJ, Chabot B. Telomere elongation by hnRNP A1 and a derivative that interacts with telomeric repeats and telomerase. Nat Genet 1998 Jun;19(2):199–202.
Petersen-Mahrt SK, Estmer C, Ohrmalm C, Matthews DA, Russell WC, Akusjärvi G. The splicing factor-associated protein, p32, regulates RNA splicing by inhibiting ASF/SF2 RNA binding and phosphorylation. EMBO J 1999;18(4):1014–24.
Caputi M, Mayeda A, Krainer AR, Zahler AM. hnRNP A/B proteins are required for inhibition of HIV-1 pre-mRNA splicing. EMBO J. 1999 Jul 15;18(14):4060-7.
Zhu D1, Xu G, Ghandhi S, Hubbard K. Modulation of the expression of p16INK4a and p14ARF by hnRNP A1 and A2 RNA binding proteins: implications for cellular senescence. J Cell Physiol. 2002 Oct;193(1):19-25.
Wang H, Han L, Zhao G. hnRNP A1 antagonizes cellular senescence and senescence-associated secretory phenotype via regulation of SIRT1 mRNA stability. Aging Cell. 2016 Sep 9. doi: 10.1111/acel.12511. [Epub ahead of print].
Cantó C, Gerhart-Hines Z, Feige JN, et al. AMPK regulates energy expenditure by modulating NAD+ metabolism and SIRT1 activity. Nature. 2009 Apr 23;458(7241):1056-60.
Moshiri A, Puppo M, Rossi M, Gherzi R, Briata P. Resveratrol limits epithelial to mesenchymal transition through modulation of KHSRP/hnRNPA1-dependent alternative splicing in mammary gland cells. Biochim Biophys Acta. 2017 Mar;1860(3):291-298.
Chiang MC, Nicol CJ, Cheng YC. Resveratrol activation of AMPK-dependent pathways is neuroprotective in human neural stem cells against amyloid-beta-induced inflammation and oxidative stress. Neurochem Int. 2017 Oct 5. pii: S0197-0186(17)30362-5.
Dahabieh MS, Battivelli E, Verdin E. Understanding HIV latency: the road to an HIV cure. Annu Rev Med. 2015;66:407-21.
Sena LA, Li S, Jairaman A, et al. Mitochondria are required for antigen-specific T cell activation through reactive oxygen species signaling. Immunity. 2013 Feb 21;38(2):225-36.
Weng NP, Levine BL, June CH, Hodes RJ. Regulated expression of telomerase activity in human T lymphocyte development and activation. J Exp Med. 1996 Jun 1;183(6):2471-9.
Rao E, Zhang Y, Zhu G, et al. Deficiency of AMPK in CD8+ T cells suppresses their anti-tumor function by inducing protein phosphatase-mediated cell death. Oncotarget. 2015 Apr 10;6(10):7944-58.
Zeng X, Pan X, Xu X, et al. Resveratrol Reactivates Latent HIV through Increasing Histone Acetylation and Activating Heat Shock Factor 1. J Agric Food Chem. 2017 Jun 7;65(22):4384-4394.
G.M. Chew, D.C. Chow, S.A. Souza, et al. Impact of adjunctive metformin therapy on T cell exhaustion and viral persistence in a clinical trial of HIV-infected adults on suppressive ART. Journal of Virus Eradication 2017; 3 (Supplement 1): 6–19.
http://viruseradication.com/abstract-details.php?abstract_id=1188, last accessed March 14, 2018.
Finley J. Reactivation of latently infected HIV-1 viral reservoirs and correction of aberrant alternative splicing in the LMNA gene via AMPK activation: Common mechanism of action linking HIV-1 latency and Hutchinson-Gilford progeria syndrome. Med Hypotheses. 2015 Sep;85(3):320-32.
Finley J. Oocyte activation and latent HIV-1 reactivation: AMPK as a common mechanism of action linking the beginnings of life and the potential eradication of HIV-1. Med Hypotheses. 2016 Aug;93:34-47.
Calle-Guisado V, de Llera AH, Martin-Hidalgo D, et al. AMP-activated kinase in human spermatozoa: identification, intracellular localization, and key function in the regulation of sperm motility. Asian J Androl. 2017 Nov-Dec;19(6):707-714.
Auciello FR, Ross FA, Ikematsu N, Hardie DG. Oxidative stress activates AMPK in cultured cells primarily by increasing cellular AMP and/or ADP. FEBS Lett. 2014 Sep 17;588(18):3361-6.
0 notes
Text
Metformin shown for the first time to activate Telomere enzyme Telomerase in human cells via AMPK: Link between Progeria and HIV

Goldsmith Content Providers: CDC/ C. Goldsmith, P. Feorino, E. L. Palmer, W. R. McManus [Public domain], via Wikimedia Commons;The Cell Nucleus and Aging: Tantalizing Clues and Hopeful Promises. Scaffidi P, Gordon L, Misteli T. PLoS Biology Vol. 3/11/2005, e39
A recently published study in the journal Biochimica et Biophysica Acta (BBA) - Molecular Basis of Disease in 2018 demonstrated for the first time that chronic treatment with the anti-diabetic drug metformin activated human telomerase reverse transcriptase (hTERT) in human aortic endothelial cells (HAECs) and significantly delayed endothelial senescence in an AMPK-dependent manner [1]. AMPK is activated by the induction of cellular stress, mediated by increases in intracellular calcium (Ca2+), reactive oxygen species (ROS), and/or an AMP(ADP)/ATP ratio increase, etc. [41]. Telomeres are specialized regions of repetitive nucleotide sequences located at the ends of eukaryotic chromosomes that protect chromosomal ends from deterioration [2]. However, continuous cell division leads to telomere shortening, impeding the replenishment of tissues and triggering cellular senescence (i.e. cells cease to divide). Although human telomeres shorten with age, telomeres may be lengthened by the enzyme telomerase, a ribonucleoprotein that consists of the catalytic subunit hTERT, telomerase RNA, and the nucleolar protein dyskerin [2,3]. hTERT, which is considering limiting for telomerase activity, is a protein that exhibits reverse transcriptase activity and synthesizes telomeric DNA from an RNA template [4].
The authors of the study initially demonstrated that both metformin and the AMPK activator AICAR significantly increased hTERT levels and enhanced AMPK activation in human aortic endothelial cells (HAECs) [1]. Importantly, inhibition or knockdown of AMPK with compound C or siAMPKα inhibited the metformin-induced increase in hTERT while metformin failed to reverse siAMPKα-induced senescence in HAECs, indicating that hTERT expression is regulated by AMPK activation in endothelial cells. Indeed, continuous culturing of HAECs in the presence of metformin significantly increased hTERT protein levels and activity, reduced the expression of the senescence markers p53, p21, p27, and p16, and reduced senescence-associated beta-galactosidase (SA-β-gal, a biomarker of cellular senescence) staining in HAECs, again indicating that metformin delays cellular senescence and increases hTERT levels via AMPK activation [1]. Strikingly, using ApoE-/- mice (which spontaneously develop atherosclerosis and age faster compared to normal mice), the authors also showed that chronic low-dose metformin administration for fourteen months in drinking water enhanced the levels of activated AMPK and Pgc-1α observed in the endothelial layer of the aorta [1]. Metformin also increased the transcript and protein levels of Tert, decreased senescence markers (p16, p21, p27, p53) in the total aortic homogenate, and significantly reduced SA-β-gal staining of aorta compared to untreated ApoE-/- mice, demonstrating that metformin-induced AMPK activation delays vascular aging and protects from age-associated atherosclerosis in ApoE-/- mice [1].
Interestingly, telomere length has been shown to be significantly reduced in cells derived from patients with the accelerated aging disorder Hutchinson-Gilford progeria syndrome (HGPS) and telomere shortening in normal cells that occurs during cellular senescence activates progerin production, a toxic protein that leads to an accelerating aging phenotype in children with HGPS via aberrant alternative splicing of the LMNA gene [5]. Normal lamin A plays a critical role in supporting nuclear architecture and morphology. Lamin A binding to subtelomeric repeats also localizes telomeres to the nuclear periphery and loss of lamin A leads to defects in telomeric heterochromatin, altered nuclear distribution and shortening of telomeres, inefficient processing of dysfunctional telomeres by non-homologus end joining, and increased genomic instability [6]. Telomere length has been found to be significantly reduced in fibroblasts derived from HGPS patients and a recent study also confirmed that in normal human fibroblasts, progressive telomere damage that occurs during cellular senescence activates progerin production and also leads to extensive changes in alternative splicing of many other genes, highlighting a striking similarity between normal aging and accelerated aging in HGPS patients [5,7]. Indeed, transfection of HGPS fibroblasts with human telomerase (hTERT) mRNA restored cell proliferation, reduced cell loss, extended cellular lifespan, increased telomerase activity and telomere length, and reduced SA-β-gal staining compared to HGPS cells expressing catalytically inactive hTERT mRNA [8]. Because metformin also increases hTERT expression and inhibits senescence in human cells, it is likely that cellular stress-induced AMPK activation, mediated by increases in intracellular calcium (Ca2+), reactive oxygen species (ROS), and/or an AMP(ADP)/ATP ratio increase, etc., represents a central node linking structurally diverse compounds and methodologies that alleviate accelerated aging in HGPS cells.
As the splicing factor SRSF1 has been shown to increase progerin production by promoting the use of a cryptic splice located in the LMNA gene, metformin was recently shown to significantly reduce the expression of SRSF1 and progerin, activate AMPK, and improve nuclear architecture in HGPS cells, indicating that AMPK activation by metformin beneficially alters gene splicing in HGPS cells by modulating SRSF1, a hypothesis that I first proposed and published in 2014 [9-11]. Also, p32, a splicing-associated protein that is an endogenous inhibitor of SRSF1 and is critical for the maintenance of mitochondrial functionality and oxidative phosphorylation, has also been shown to be essential for rapamycin- or starvation-induced autophagy mediated by ULK1 [12,13]. Because rapamycin, also an AMPK activator in vivo, improves accelerated aging defects in HGPS cells by reducing progerin levels via induction of autophagy, AMPK activation likely also beneficially modulates the activity of p32, leading to inhibition of SRSF1 splicing activity and enhancement of mitochondrial functionality [14,15]. Moreover, PGC-1α, which is activated by AMPK and metformin and promotes telomere transcription, is downregulated in HGPS cells, leading to significant mitochondrial dysfunction [1,16,17]. Methylene blue, which activates AMPK in vivo, was shown to increase PGC-1α levels, induce progerin solubility, and alleviate accelerated aging defects in HGPS cells [16,18]. Additionally, the rapamycin analog temsirolimus alleviated accelerated aging defects in HGPS cells but transiently increased ROS and superoxide anion levels in both HGPS and normal cells within the first hour of treatment, again indicating that cellular stress-induced AMPK activation represents a common mechanism for inhibiting senescence and ameliorating symptoms associated with accelerated aging [19].
The inhibition of SRSF1 and the promotion of hTERT expression and telomere transcription by metformin via AMPK activation also link HGPS and telomere integrity with HIV-1 latency. Increased splicing activity of SRSF1 inhibits reactivation of latent HIV-1 residing in infected immune cells, preventing immune system detection and destruction of the virus [20]. Reactivation of latent HIV-1 (i.e. the “shock and kill” approach) leads to a reduction in SRSF1 but an increase in p32 activity and bryostatin-1 (a PKC modulator) has been shown to reactivate latent HIV-1 via AMPK activation [20,21]. Interestingly, p32 modulation via AMPK activation may also enhance and stabilize the splicing activities of hnRNPA1, a heteroribonuclear protein that associates with p32, antagonizes the splicing function of SRSF1, prevents splicing of the HIV-1 genome (promoting viral reactivation), and participates in the maintenance and preservation of telomeres [22-25]. hnRNPA1 is also decreased in senescent human fibroblasts and antagonizes cellular senescence and the senescence-associated secretory phenotype (SASP) via increasing SIRT1 expression [26,27]. Resveratrol, a plant-derived polyphenol that activates AMPK, increases hnRNPA1 protein expression and SIRT1, a histone deacetylase that plays a role in a number of age related diseases and in the extension of lifespan, is also activated by AMPK [28-30]. Also, T cell activation, an efficient method for reactivating latent HIV-1, is dependent on increases in intracellular Ca2+ and ROS, telomerase is transiently increased on T cell activation, and AMPK knockdown leads to T cell death during in vitro activation [31-34]. Furthermore, resveratrol reactivates latent HIV-1 and preliminary data demonstrated that metformin decreased the percentage of CD4+ T cells expressing PD-1, TIGIT, and TIM-3, each markers associated with T cells latently infected with HIV-1, in chronically-infected HIV-1 patients [35-37]. Such evidence strongly suggests that cellular stress-induced AMPK activation, mediated by increases in intracellular calcium (Ca2+), reactive oxygen species (ROS), and/or an AMP (ADP)/ATP ratio increase, etc. links the alleviation of accelerated cellular aging defects in HGPS with the potential eradication of HIV-1, a hypothesis that I first proposed in 2015 [38].
The evidence presented in the Biochimica et Biophysica Acta (BBA) - Molecular Basis of Disease publication further substantiates that AMPK activation represents a central node that connects the therapeutic benefits of chemically distinct compounds in diseases as seemingly dissimilar as HGPS and HIV-1 latency. Indeed, AMPK activators including metformin increase hTERT expression, promote telomere transcription and integrity, decrease the splicing activity of SRSF1 that increases progerin production but prevents latent HIV-1 reactivation, and potentially beneficially modulates the activity of the SRSF1 inhibitor p32 and the ribonucleoprotein hnRNPA1. As AMPK activators (e.g. ionomycin) induce human oocyte activation (giving rise to normal healthy children) and AMPK is localized throughout the entire acrosome in human sperm (likely promoting the acrosome reaction), AMPK activation links normal human aging, Progeria, and HIV-1 latency with the creation of all human life (i.e. the “shock and live” approach) [11,38-40].
https://www.linkedin.com/pulse/metformin-shown-first-time-activate-telomere-enzyme-human-finley/?published=t
References:
Karnewar S, Neeli PK, Panuganti D, et al. Metformin regulates mitochondrial biogenesis and senescence through AMPK mediated H3K79 methylation: Relevance in age-associated vascular dysfunction. Biochim Biophys Acta. 2018 Apr;1864(4 Pt A):1115-1128.
O'Sullivan RJ, Karlseder J. Telomeres: protecting chromosomes against genome instability. Nat Rev Mol Cell Biol. 2010 Mar;11(3):171-81.
Cohen SB, Graham ME, Lovrecz GO, Bache N, Robinson PJ, Reddel RR. Protein composition of catalytically active human telomerase from immortal cells. Science. 2007 Mar 30;315(5820):1850-3.
Cong YS, Wen J, Bacchetti S. The human telomerase catalytic subunit hTERT: organization of the gene and characterization of the promoter. Hum Mol Genet. 1999 Jan;8(1):137-42.
Cao K, Blair CD, Faddah DA, et al. Progerin and telomere dysfunction collaborate to trigger cellular senescence in normal human fibroblasts. J Clin Invest. 2011 Jul;121(7):2833-44.
Gonzalez-Suarez I, Redwood AB, Perkins SM, et al. Novel roles for A-type lamins in telomere biology and the DNA damage response pathway. EMBO J 2009;28(16):2414–27.
Decker ML, Chavez E, Vulto I, Lansdorp PM. Telomere length in Hutchinson-Gilford progeria syndrome. Mech Ageing Dev. 2009 Jun;130(6):377-83.
Li Y, Zhou G, Bruno IG, Cooke JP. Telomerase mRNA Reverses Senescence in Progeria Cells. J Am Coll Cardiol. 2017 Aug 8;70(6):804-805.
Egesipe AL, Blondel S1, Cicero AL, et al. Metformin decreases progerin expression and alleviates pathological defects of Hutchinson-Gilford progeria syndrome cells. NPJ Aging Mech Dis. 2016 Nov 10;2:16026.
Park SK, Shin OS. Metformin alleviates ageing cellular phenotypes in Hutchinson-Gilford progeria syndrome dermal fibroblasts. Exp Dermatol. 2017 Oct;26(10):889-895.
Finley J. Alteration of splice site selection in the LMNA gene and inhibition of progerin production via AMPK activation. Med Hypotheses. 2014 Nov;83(5):580-7.
P32/gC1qR is indispensable for fetal development and mitochondrial translation: importance of its RNA-binding ability. Nucl Acids Res 2012;40(19):9717–37.
Jiao H, Su GQ, Dong W, et al. Chaperone-like protein p32 regulates ULK1 stability and autophagy. Cell Death Differ. 2015 Nov;22(11):1812-23.
Cao K, Graziotto JJ, Blair CD, et al. Rapamycin reverses cellular phenotypes and enhances mutant protein clearance in Hutchinson-Gilford progeria syndrome cells. Sci Transl Med. 2011 Jun 29;3(89):89ra58.
Chiao YA, Kolwicz SC, Basisty N, et al. Rapamycin transiently induces mitochondrial remodeling to reprogram energy metabolism in old hearts. Aging (Albany NY). 2016 Feb;8(2):314-27.
Xiong ZM, Choi JY, Wang K, et al. Methylene blue alleviates nuclear and mitochondrial abnormalities in progeria. Aging Cell. 2016 Apr;15(2):279-90.
Diman A, Boros J, Poulain F, et al. Nuclear respiratory factor 1 and endurance exercise promote human telomere transcription. Sci Adv. 2016 Jul 27;2(7):e1600031.
Xie L, Li W, Winters A, Yuan F, Jin K, Yang S. Methylene blue induces macroautophagy through 5' adenosine monophosphate-activated protein kinase pathway to protect neurons from serum deprivation. Front Cell Neurosci. 2013 May 3;7:56.
Gabriel D, Gordon LB, Djabali K. Temsirolimus Partially Rescues the Hutchinson-Gilford Progeria Cellular Phenotype. PLoS One. 2016 Dec 29;11(12):e0168988.
Berro R, Kehn K, de la Fuente C, et al. Acetylated Tat regulates human immunodeficiency virus type 1 splicing through its interaction with the splicing regulator p32. J Virol. 2006 Apr;80(7):3189-204.
Mehla R, Bivalkar-Mehla S, Zhang R, et al. Bryostatin modulates latent HIV-1 infection via PKC and AMPK signaling but inhibits acute infection in a receptor independent manner. PLoS One. 2010 Jun 16;5(6):e11160.
Expert-Bezançon A, Sureau A, Durosay P, et al. HnRNP A1 and the SR proteins ASF/SF2 and SC35 have antagonistic functions in splicing of beta tropomyosin exon 6B. J Biol Chem 2004 Sep 10;279(37):38249–59.
LaBranche H, Dupuis S, Ben-David Y, Bani MR, Wellinger RJ, Chabot B. Telomere elongation by hnRNP A1 and a derivative that interacts with telomeric repeats and telomerase. Nat Genet 1998 Jun;19(2):199–202.
Petersen-Mahrt SK, Estmer C, Ohrmalm C, Matthews DA, Russell WC, Akusjärvi G. The splicing factor-associated protein, p32, regulates RNA splicing by inhibiting ASF/SF2 RNA binding and phosphorylation. EMBO J 1999;18(4):1014–24.
Caputi M, Mayeda A, Krainer AR, Zahler AM. hnRNP A/B proteins are required for inhibition of HIV-1 pre-mRNA splicing. EMBO J. 1999 Jul 15;18(14):4060-7.
Zhu D1, Xu G, Ghandhi S, Hubbard K. Modulation of the expression of p16INK4a and p14ARF by hnRNP A1 and A2 RNA binding proteins: implications for cellular senescence. J Cell Physiol. 2002 Oct;193(1):19-25.
Wang H, Han L, Zhao G. hnRNP A1 antagonizes cellular senescence and senescence-associated secretory phenotype via regulation of SIRT1 mRNA stability. Aging Cell. 2016 Sep 9. doi: 10.1111/acel.12511. [Epub ahead of print].
Cantó C, Gerhart-Hines Z, Feige JN, et al. AMPK regulates energy expenditure by modulating NAD+ metabolism and SIRT1 activity. Nature. 2009 Apr 23;458(7241):1056-60.
Moshiri A, Puppo M, Rossi M, Gherzi R, Briata P. Resveratrol limits epithelial to mesenchymal transition through modulation of KHSRP/hnRNPA1-dependent alternative splicing in mammary gland cells. Biochim Biophys Acta. 2017 Mar;1860(3):291-298.
Chiang MC, Nicol CJ, Cheng YC. Resveratrol activation of AMPK-dependent pathways is neuroprotective in human neural stem cells against amyloid-beta-induced inflammation and oxidative stress. Neurochem Int. 2017 Oct 5. pii: S0197-0186(17)30362-5.
Dahabieh MS, Battivelli E, Verdin E. Understanding HIV latency: the road to an HIV cure. Annu Rev Med. 2015;66:407-21.
Sena LA, Li S, Jairaman A, et al. Mitochondria are required for antigen-specific T cell activation through reactive oxygen species signaling. Immunity. 2013 Feb 21;38(2):225-36.
Weng NP, Levine BL, June CH, Hodes RJ. Regulated expression of telomerase activity in human T lymphocyte development and activation. J Exp Med. 1996 Jun 1;183(6):2471-9.
Rao E, Zhang Y, Zhu G, et al. Deficiency of AMPK in CD8+ T cells suppresses their anti-tumor function by inducing protein phosphatase-mediated cell death. Oncotarget. 2015 Apr 10;6(10):7944-58.
Zeng X, Pan X, Xu X, et al. Resveratrol Reactivates Latent HIV through Increasing Histone Acetylation and Activating Heat Shock Factor 1. J Agric Food Chem. 2017 Jun 7;65(22):4384-4394.
G.M. Chew, D.C. Chow, S.A. Souza, et al. Impact of adjunctive metformin therapy on T cell exhaustion and viral persistence in a clinical trial of HIV-infected adults on suppressive ART. Journal of Virus Eradication 2017; 3 (Supplement 1): 6–19.
http://viruseradication.com/abstract-details.php?abstract_id=1188, last accessed March 14, 2018.
Finley J. Reactivation of latently infected HIV-1 viral reservoirs and correction of aberrant alternative splicing in the LMNA gene via AMPK activation: Common mechanism of action linking HIV-1 latency and Hutchinson-Gilford progeria syndrome. Med Hypotheses. 2015 Sep;85(3):320-32.
Finley J. Oocyte activation and latent HIV-1 reactivation: AMPK as a common mechanism of action linking the beginnings of life and the potential eradication of HIV-1. Med Hypotheses. 2016 Aug;93:34-47.
Calle-Guisado V, de Llera AH, Martin-Hidalgo D, et al. AMP-activated kinase in human spermatozoa: identification, intracellular localization, and key function in the regulation of sperm motility. Asian J Androl. 2017 Nov-Dec;19(6):707-714.
Auciello FR, Ross FA, Ikematsu N, Hardie DG. Oxidative stress activates AMPK in cultured cells primarily by increasing cellular AMP and/or ADP. FEBS Lett. 2014 Sep 17;588(18):3361-6
0 notes
Text
Metformin shown for the first time to activate Telomere enzyme Telomerase in human cells via AMPK: Link between Progeria and HIV

Goldsmith Content Providers: CDC/ C. Goldsmith, P. Feorino, E. L. Palmer, W. R. McManus [Public domain], via Wikimedia Commons;The Cell Nucleus and Aging: Tantalizing Clues and Hopeful Promises. Scaffidi P, Gordon L, Misteli T. PLoS Biology Vol. 3/11/2005, e39
A recently published study in the journal Biochimica et Biophysica Acta (BBA) - Molecular Basis of Disease in 2018 demonstrated for the first time that chronic treatment with the anti-diabetic drug metformin activated human telomerase reverse transcriptase (hTERT) in human aortic endothelial cells (HAECs) and significantly delayed endothelial senescence in an AMPK-dependent manner [1]. AMPK is activated by the induction of cellular stress, mediated by increases in intracellular calcium (Ca2+), reactive oxygen species (ROS), and/or an AMP(ADP)/ATP ratio increase, etc. [41]. Telomeres are specialized regions of repetitive nucleotide sequences located at the ends of eukaryotic chromosomes that protect chromosomal ends from deterioration [2]. However, continuous cell division leads to telomere shortening, impeding the replenishment of tissues and triggering cellular senescence (i.e. cells cease to divide). Although human telomeres shorten with age, telomeres may be lengthened by the enzyme telomerase, a ribonucleoprotein that consists of the catalytic subunit hTERT, telomerase RNA, and the nucleolar protein dyskerin [2,3]. hTERT, which is considering limiting for telomerase activity, is a protein that exhibits reverse transcriptase activity and synthesizes telomeric DNA from an RNA template [4].
The authors of the study initially demonstrated that both metformin and the AMPK activator AICAR significantly increased hTERT levels and enhanced AMPK activation in human aortic endothelial cells (HAECs) [1]. Importantly, inhibition or knockdown of AMPK with compound C or siAMPKα inhibited the metformin-induced increase in hTERT while metformin failed to reverse siAMPKα-induced senescence in HAECs, indicating that hTERT expression is regulated by AMPK activation in endothelial cells. Indeed, continuous culturing of HAECs in the presence of metformin significantly increased hTERT protein levels and activity, reduced the expression of the senescence markers p53, p21, p27, and p16, and reduced senescence-associated beta-galactosidase (SA-β-gal, a biomarker of cellular senescence) staining in HAECs, again indicating that metformin delays cellular senescence and increases hTERT levels via AMPK activation [1]. Strikingly, using ApoE-/- mice (which spontaneously develop atherosclerosis and age faster compared to normal mice), the authors also showed that chronic low-dose metformin administration for fourteen months in drinking water enhanced the levels of activated AMPK and Pgc-1α observed in the endothelial layer of the aorta [1]. Metformin also increased the transcript and protein levels of Tert, decreased senescence markers (p16, p21, p27, p53) in the total aortic homogenate, and significantly reduced SA-β-gal staining of aorta compared to untreated ApoE-/- mice, demonstrating that metformin-induced AMPK activation delays vascular aging and protects from age-associated atherosclerosis in ApoE-/- mice [1].
Interestingly, telomere length has been shown to be significantly reduced in cells derived from patients with the accelerated aging disorder Hutchinson-Gilford progeria syndrome (HGPS) and telomere shortening in normal cells that occurs during cellular senescence activates progerin production, a toxic protein that leads to an accelerating aging phenotype in children with HGPS via aberrant alternative splicing of the LMNA gene [5]. Normal lamin A plays a critical role in supporting nuclear architecture and morphology. Lamin A binding to subtelomeric repeats also localizes telomeres to the nuclear periphery and loss of lamin A leads to defects in telomeric heterochromatin, altered nuclear distribution and shortening of telomeres, inefficient processing of dysfunctional telomeres by non-homologus end joining, and increased genomic instability [6]. Telomere length has been found to be significantly reduced in fibroblasts derived from HGPS patients and a recent study also confirmed that in normal human fibroblasts, progressive telomere damage that occurs during cellular senescence activates progerin production and also leads to extensive changes in alternative splicing of many other genes, highlighting a striking similarity between normal aging and accelerated aging in HGPS patients [5,7]. Indeed, transfection of HGPS fibroblasts with human telomerase (hTERT) mRNA restored cell proliferation, reduced cell loss, extended cellular lifespan, increased telomerase activity and telomere length, and reduced SA-β-gal staining compared to HGPS cells expressing catalytically inactive hTERT mRNA [8]. Because metformin also increases hTERT expression and inhibits senescence in human cells, it is likely that cellular stress-induced AMPK activation, mediated by increases in intracellular calcium (Ca2+), reactive oxygen species (ROS), and/or an AMP(ADP)/ATP ratio increase, etc., represents a central node linking structurally diverse compounds and methodologies that alleviate accelerated aging in HGPS cells.
As the splicing factor SRSF1 has been shown to increase progerin production by promoting the use of a cryptic splice located in the LMNA gene, metformin was recently shown to significantly reduce the expression of SRSF1 and progerin, activate AMPK, and improve nuclear architecture in HGPS cells, indicating that AMPK activation by metformin beneficially alters gene splicing in HGPS cells by modulating SRSF1, a hypothesis that I first proposed and published in 2014 [9-11]. Also, p32, a splicing-associated protein that is an endogenous inhibitor of SRSF1 and is critical for the maintenance of mitochondrial functionality and oxidative phosphorylation, has also been shown to be essential for rapamycin- or starvation-induced autophagy mediated by ULK1 [12,13]. Because rapamycin, also an AMPK activator in vivo, improves accelerated aging defects in HGPS cells by reducing progerin levels via induction of autophagy, AMPK activation likely also beneficially modulates the activity of p32, leading to inhibition of SRSF1 splicing activity and enhancement of mitochondrial functionality [14,15]. Moreover, PGC-1α, which is activated by AMPK and metformin and promotes telomere transcription, is downregulated in HGPS cells, leading to significant mitochondrial dysfunction [1,16,17]. Methylene blue, which activates AMPK in vivo, was shown to increase PGC-1α levels, induce progerin solubility, and alleviate accelerated aging defects in HGPS cells [16,18]. Additionally, the rapamycin analog temsirolimus alleviated accelerated aging defects in HGPS cells but transiently increased ROS and superoxide anion levels in both HGPS and normal cells within the first hour of treatment, again indicating that cellular stress-induced AMPK activation represents a common mechanism for inhibiting senescence and ameliorating symptoms associated with accelerated aging [19].
The inhibition of SRSF1 and the promotion of hTERT expression and telomere transcription by metformin via AMPK activation also link HGPS and telomere integrity with HIV-1 latency. Increased splicing activity of SRSF1 inhibits reactivation of latent HIV-1 residing in infected immune cells, preventing immune system detection and destruction of the virus [20]. Reactivation of latent HIV-1 (i.e. the “shock and kill” approach) leads to a reduction in SRSF1 but an increase in p32 activity and bryostatin-1 (a PKC modulator) has been shown to reactivate latent HIV-1 via AMPK activation [20,21]. Interestingly, p32 modulation via AMPK activation may also enhance and stabilize the splicing activities of hnRNPA1, a heteroribonuclear protein that associates with p32, antagonizes the splicing function of SRSF1, prevents splicing of the HIV-1 genome (promoting viral reactivation), and participates in the maintenance and preservation of telomeres [22-25]. hnRNPA1 is also decreased in senescent human fibroblasts and antagonizes cellular senescence and the senescence-associated secretory phenotype (SASP) via increasing SIRT1 expression [26,27]. Resveratrol, a plant-derived polyphenol that activates AMPK, increases hnRNPA1 protein expression and SIRT1, a histone deacetylase that plays a role in a number of age related diseases and in the extension of lifespan, is also activated by AMPK [28-30]. Also, T cell activation, an efficient method for reactivating latent HIV-1, is dependent on increases in intracellular Ca2+ and ROS, telomerase is transiently increased on T cell activation, and AMPK knockdown leads to T cell death during in vitro activation [31-34]. Furthermore, resveratrol reactivates latent HIV-1 and preliminary data demonstrated that metformin decreased the percentage of CD4+ T cells expressing PD-1, TIGIT, and TIM-3, each markers associated with T cells latently infected with HIV-1, in chronically-infected HIV-1 patients [35-37]. Such evidence strongly suggests that cellular stress-induced AMPK activation, mediated by increases in intracellular calcium (Ca2+), reactive oxygen species (ROS), and/or an AMP (ADP)/ATP ratio increase, etc. links the alleviation of accelerated cellular aging defects in HGPS with the potential eradication of HIV-1, a hypothesis that I first proposed in 2015 [38].
The evidence presented in the Biochimica et Biophysica Acta (BBA) - Molecular Basis of Disease publication further substantiates that AMPK activation represents a central node that connects the therapeutic benefits of chemically distinct compounds in diseases as seemingly dissimilar as HGPS and HIV-1 latency. Indeed, AMPK activators including metformin increase hTERT expression, promote telomere transcription and integrity, decrease the splicing activity of SRSF1 that increases progerin production but prevents latent HIV-1 reactivation, and potentially beneficially modulates the activity of the SRSF1 inhibitor p32 and the ribonucleoprotein hnRNPA1. As AMPK activators (e.g. ionomycin) induce human oocyte activation (giving rise to normal healthy children) and AMPK is localized throughout the entire acrosome in human sperm (likely promoting the acrosome reaction), AMPK activation links normal human aging, Progeria, and HIV-1 latency with the creation of all human life (i.e. the “shock and live” approach) [11,38-40].
https://www.linkedin.com/pulse/metformin-shown-first-time-activate-telomere-enzyme-human-finley/?published=t
References:
Karnewar S, Neeli PK, Panuganti D, et al. Metformin regulates mitochondrial biogenesis and senescence through AMPK mediated H3K79 methylation: Relevance in age-associated vascular dysfunction. Biochim Biophys Acta. 2018 Apr;1864(4 Pt A):1115-1128.
O'Sullivan RJ, Karlseder J. Telomeres: protecting chromosomes against genome instability. Nat Rev Mol Cell Biol. 2010 Mar;11(3):171-81.
Cohen SB, Graham ME, Lovrecz GO, Bache N, Robinson PJ, Reddel RR. Protein composition of catalytically active human telomerase from immortal cells. Science. 2007 Mar 30;315(5820):1850-3.
Cong YS, Wen J, Bacchetti S. The human telomerase catalytic subunit hTERT: organization of the gene and characterization of the promoter. Hum Mol Genet. 1999 Jan;8(1):137-42.
Cao K, Blair CD, Faddah DA, et al. Progerin and telomere dysfunction collaborate to trigger cellular senescence in normal human fibroblasts. J Clin Invest. 2011 Jul;121(7):2833-44.
Gonzalez-Suarez I, Redwood AB, Perkins SM, et al. Novel roles for A-type lamins in telomere biology and the DNA damage response pathway. EMBO J 2009;28(16):2414–27.
Decker ML, Chavez E, Vulto I, Lansdorp PM. Telomere length in Hutchinson-Gilford progeria syndrome. Mech Ageing Dev. 2009 Jun;130(6):377-83.
Li Y, Zhou G, Bruno IG, Cooke JP. Telomerase mRNA Reverses Senescence in Progeria Cells. J Am Coll Cardiol. 2017 Aug 8;70(6):804-805.
Egesipe AL, Blondel S1, Cicero AL, et al. Metformin decreases progerin expression and alleviates pathological defects of Hutchinson-Gilford progeria syndrome cells. NPJ Aging Mech Dis. 2016 Nov 10;2:16026.
Park SK, Shin OS. Metformin alleviates ageing cellular phenotypes in Hutchinson-Gilford progeria syndrome dermal fibroblasts. Exp Dermatol. 2017 Oct;26(10):889-895.
Finley J. Alteration of splice site selection in the LMNA gene and inhibition of progerin production via AMPK activation. Med Hypotheses. 2014 Nov;83(5):580-7.
P32/gC1qR is indispensable for fetal development and mitochondrial translation: importance of its RNA-binding ability. Nucl Acids Res 2012;40(19):9717–37.
Jiao H, Su GQ, Dong W, et al. Chaperone-like protein p32 regulates ULK1 stability and autophagy. Cell Death Differ. 2015 Nov;22(11):1812-23.
Cao K, Graziotto JJ, Blair CD, et al. Rapamycin reverses cellular phenotypes and enhances mutant protein clearance in Hutchinson-Gilford progeria syndrome cells. Sci Transl Med. 2011 Jun 29;3(89):89ra58.
Chiao YA, Kolwicz SC, Basisty N, et al. Rapamycin transiently induces mitochondrial remodeling to reprogram energy metabolism in old hearts. Aging (Albany NY). 2016 Feb;8(2):314-27.
Xiong ZM, Choi JY, Wang K, et al. Methylene blue alleviates nuclear and mitochondrial abnormalities in progeria. Aging Cell. 2016 Apr;15(2):279-90.
Diman A, Boros J, Poulain F, et al. Nuclear respiratory factor 1 and endurance exercise promote human telomere transcription. Sci Adv. 2016 Jul 27;2(7):e1600031.
Xie L, Li W, Winters A, Yuan F, Jin K, Yang S. Methylene blue induces macroautophagy through 5' adenosine monophosphate-activated protein kinase pathway to protect neurons from serum deprivation. Front Cell Neurosci. 2013 May 3;7:56.
Gabriel D, Gordon LB, Djabali K. Temsirolimus Partially Rescues the Hutchinson-Gilford Progeria Cellular Phenotype. PLoS One. 2016 Dec 29;11(12):e0168988.
Berro R, Kehn K, de la Fuente C, et al. Acetylated Tat regulates human immunodeficiency virus type 1 splicing through its interaction with the splicing regulator p32. J Virol. 2006 Apr;80(7):3189-204.
Mehla R, Bivalkar-Mehla S, Zhang R, et al. Bryostatin modulates latent HIV-1 infection via PKC and AMPK signaling but inhibits acute infection in a receptor independent manner. PLoS One. 2010 Jun 16;5(6):e11160.
Expert-Bezançon A, Sureau A, Durosay P, et al. HnRNP A1 and the SR proteins ASF/SF2 and SC35 have antagonistic functions in splicing of beta tropomyosin exon 6B. J Biol Chem 2004 Sep 10;279(37):38249–59.
LaBranche H, Dupuis S, Ben-David Y, Bani MR, Wellinger RJ, Chabot B. Telomere elongation by hnRNP A1 and a derivative that interacts with telomeric repeats and telomerase. Nat Genet 1998 Jun;19(2):199–202.
Petersen-Mahrt SK, Estmer C, Ohrmalm C, Matthews DA, Russell WC, Akusjärvi G. The splicing factor-associated protein, p32, regulates RNA splicing by inhibiting ASF/SF2 RNA binding and phosphorylation. EMBO J 1999;18(4):1014–24.
Caputi M, Mayeda A, Krainer AR, Zahler AM. hnRNP A/B proteins are required for inhibition of HIV-1 pre-mRNA splicing. EMBO J. 1999 Jul 15;18(14):4060-7.
Zhu D1, Xu G, Ghandhi S, Hubbard K. Modulation of the expression of p16INK4a and p14ARF by hnRNP A1 and A2 RNA binding proteins: implications for cellular senescence. J Cell Physiol. 2002 Oct;193(1):19-25.
Wang H, Han L, Zhao G. hnRNP A1 antagonizes cellular senescence and senescence-associated secretory phenotype via regulation of SIRT1 mRNA stability. Aging Cell. 2016 Sep 9. doi: 10.1111/acel.12511. [Epub ahead of print].
Cantó C, Gerhart-Hines Z, Feige JN, et al. AMPK regulates energy expenditure by modulating NAD+ metabolism and SIRT1 activity. Nature. 2009 Apr 23;458(7241):1056-60.
Moshiri A, Puppo M, Rossi M, Gherzi R, Briata P. Resveratrol limits epithelial to mesenchymal transition through modulation of KHSRP/hnRNPA1-dependent alternative splicing in mammary gland cells. Biochim Biophys Acta. 2017 Mar;1860(3):291-298.
Chiang MC, Nicol CJ, Cheng YC. Resveratrol activation of AMPK-dependent pathways is neuroprotective in human neural stem cells against amyloid-beta-induced inflammation and oxidative stress. Neurochem Int. 2017 Oct 5. pii: S0197-0186(17)30362-5.
Dahabieh MS, Battivelli E, Verdin E. Understanding HIV latency: the road to an HIV cure. Annu Rev Med. 2015;66:407-21.
Sena LA, Li S, Jairaman A, et al. Mitochondria are required for antigen-specific T cell activation through reactive oxygen species signaling. Immunity. 2013 Feb 21;38(2):225-36.
Weng NP, Levine BL, June CH, Hodes RJ. Regulated expression of telomerase activity in human T lymphocyte development and activation. J Exp Med. 1996 Jun 1;183(6):2471-9.
Rao E, Zhang Y, Zhu G, et al. Deficiency of AMPK in CD8+ T cells suppresses their anti-tumor function by inducing protein phosphatase-mediated cell death. Oncotarget. 2015 Apr 10;6(10):7944-58.
Zeng X, Pan X, Xu X, et al. Resveratrol Reactivates Latent HIV through Increasing Histone Acetylation and Activating Heat Shock Factor 1. J Agric Food Chem. 2017 Jun 7;65(22):4384-4394.
G.M. Chew, D.C. Chow, S.A. Souza, et al. Impact of adjunctive metformin therapy on T cell exhaustion and viral persistence in a clinical trial of HIV-infected adults on suppressive ART. Journal of Virus Eradication 2017; 3 (Supplement 1): 6–19.
http://viruseradication.com/abstract-details.php?abstract_id=1188, last accessed March 14, 2018.
Finley J. Reactivation of latently infected HIV-1 viral reservoirs and correction of aberrant alternative splicing in the LMNA gene via AMPK activation: Common mechanism of action linking HIV-1 latency and Hutchinson-Gilford progeria syndrome. Med Hypotheses. 2015 Sep;85(3):320-32.
Finley J. Oocyte activation and latent HIV-1 reactivation: AMPK as a common mechanism of action linking the beginnings of life and the potential eradication of HIV-1. Med Hypotheses. 2016 Aug;93:34-47.
Calle-Guisado V, de Llera AH, Martin-Hidalgo D, et al. AMP-activated kinase in human spermatozoa: identification, intracellular localization, and key function in the regulation of sperm motility. Asian J Androl. 2017 Nov-Dec;19(6):707-714.
Auciello FR, Ross FA, Ikematsu N, Hardie DG. Oxidative stress activates AMPK in cultured cells primarily by increasing cellular AMP and/or ADP. FEBS Lett. 2014 Sep 17;588(18):3361-6.
0 notes
Text
Antibiotics produced by Bacteria activate Human Oocytes, creating Healthy Babies: AMPK links the Creation of Human Life with HIV, Progeria, & Cancer

CC-BY-SA-3.0 (http://creativecommons.org/licenses/by-sa/3.0/)], via Wikimedia Commons;By De Wood, Pooley, USDA, ARS, EMU. [Public domain], via Wikimedia Commons
A recent study published online in the journal Fertility and Sterility in September of 2017 systematically reviewed for the first time evidence for the effect of two compounds, ionomycin and A23187 (also known as calcimycin), on fertilization rates and pregnancy outcomes in infertile couples undergoing an in vitro fertilization procedure known as intracytoplasmic sperm injection (ICSI) [1]. ICSI involves the direct deposition of sperm into the oocyte cytoplasm, which typically leads to high rates of fertilization. However, fertilization failure despite repeated ICSI is likely caused by a failure of the oocyte to activate [1]. Physiological oocyte activation is accomplished by the delivery of a sperm-borne oocyte activating factor called phospholipase C zeta (PLCζ). PLCζ activates human oocytes by inducing an intracellular signaling cascade that ultimately results in increased calcium (Ca2+) oscillations in the oocyte, which drives oocyte activation to completion [1]. As oocyte activation is an indispensable prerequisite for the creation of all human life, every human being alive today and any human being that has ever lived began their existence as an activated oocyte [2]. Ionomycin and A23187 increase the levels of intracellular Ca2+ and are thus commonly known as Ca2+ ionophores [1]. The authors of the Fertility and Sterility study showed that over a total of 1,521 ICSI cycles, calcium ionophores including ionomycin and A23187 led to a statistically significant improvement in fertilization, cleavage, blastulation, implantation rates, overall pregnancy, and live-birth rates [1]. Ionomycin and A23187 have also been shown in several independent studies to effectively induce human oocyte activation, leading to the birth of normal, healthy children [3,4].
Strikingly, as described further below, both ionomycin and A23187 are antibiotics that are naturally produced by certain species within the bacterial genus Streptomyces [5,6]. Other structurally distinct compounds and methods have also been shown to induce human oocyte activation, including ethanol, puromycin (an antibiotic and protein synthesis inhibitor produced by Streptomyces alboniger), as well as mechanical manipulation and electrical stimulation, both of with have been reported to result in the creation of normal children [7-11]. Interestingly, as mouse oocytes are considered models for human oocytes, ionomycin, A23187, anisomycin (an antibiotic and protein synthesis inhibitor produced by Streptomyces griseolus), mycophenolic acid (an immunosuppressant produced by the fungus Penicillium brevicompactum), cycloheximide (a protein synthesis inhibitor produced by Streptomyces griseus), carvacrol (a secondary plant metabolite produced by Origanum vulgare{oregano}), and phorbol 12-myristate 13-acetate (PMA, a secondary plant metabolite produced by Croton tiglium) each induce activation of mouse oocytes [12-22]. Ionomycin, A23187, PMA, and reactive oxygen species (ROS) also induce the acrosome reaction in human sperm, a process characterized by the release of hydrolytic enzymes from the head of sperm which is necessary for oocyte penetration and thus indispensable for the creation of all human life outside of a clinical setting (ICSI bypasses the need for oocyte penetration) [23,24]. Additionally, although an over-production of ROS, similar to Ca2+, may lead to deleterious effects including cell death/apoptosis, low levels of ROS have been shown to act as signaling molecules and ROS is significantly increased on or immediately following mouse oocyte activation [25,26].
Furthermore, the master metabolic regulator AMPK is critical for oocyte meiotic resumption and maturation (a process that precedes and is essential for oocyte activation), is found located across the entire acrosome in the head of human sperm, and is activated by increases in ROS and Ca2+ [27-29]. Ionomycin, A23187, ethanol, puromycin, mechanical force, electrical stimulation, anisomycin, mycophenolic acid, carvacrol, and PMA also induce AMPK activation, indicating that a common mechanism of action links chemically distinct compounds with the creation of human life [30-39]. This common mechanism of action likely centers on the induction of cellular stress, mediated by indirect increases in intracellular Ca2+, ROS, and/or the AMP(ADP)/ATP ratio, etc. as I originally proposed in 2016 [40]. Because the bacterial-derived antibiotics ionomycin and A23187 induce both the acrosome reaction in human sperm and human oocyte activation, producing normal, healthy children, it can be said that “non-human organisms have the power to create human life or the power to end life.” As explained below, the beneficial effects of cellular stress induction (i.e. a “shock”) crosses species boundaries and may indeed play a role in facilitating natural selection, a process that underlies and drives evolution.
A number of bacterial species residing within the genus Streptomyces have proven to be extremely important and medicinally valuable as approximately 70% of clinically useful antibiotics are derived from Streptomyces [41]. The antibiotics ionomycin and A23187 are naturally produced by Streptomyces conglobatus and Streptomyces chartreusensis, respectively [5,6]. Other important examples include the antibiotic tetracycline (produced by Streptomyces aureofaciens), the immunosuppressant rapamycin (produced by Streptomyces hygroscopicus), and the anti-helminthic avermectins (produced by Streptomyces avermitilis) [42]. Many soil and aquatic-dwelling species of Streptomyces can be found in harsh environments and are characterized by a unique life cycle, including spore germination followed by vegetative mycelium production, aerial hyphae formation, sporulation (i.e. spore formation), and antibiotic production [43,44]. Curiously, just as cellular stress induction leads to the creation of human life and other beneficial effects in human cells (see below), stress induction also promotes the induction of aerial hyphae formation, sporulation, and antibiotic production in many Streptomyces species (spp.). Indeed, a decrease in the levels of ATP and bacterial growth is associated with sporulation, aerial hyphae formation, and antibiotic production [42,45]. A reduction in glucose/nutritional deprivation, the preferred sugar/carbon source for many Streptomyces spp., also significantly increases antibiotic production [46]. An increase in intracellular ROS and Ca2+ is associated with spore germination, aerial hyphae formation, and antibiotic production [47-49]. Other cellular stressors, including heat shock and ethanol, also significantly increase antibiotic production, provocatively indicating that the effects of cellular stress crosses species boundaries, enhancing bacterial survival and facilitating the creation of human life [50,51].
The beneficial effects of low-level cellular stress induction also extends to plants, as many plants produce secondary metabolites partly for the purpose of self-defense, analogous to antibiotics. Similar to the harsh, stressful environments often inhabited by Streptomyces spp., the Great Basin Bristlecone Pine (Pinus Longaeva), considered the oldest living non-clonal organism on the planet ( >5000 years old), thrives in an exceptionally harsh environment, characterized by increased elevations and exposure to UV radiation, nutritionally-deprived soils, harsh temperatures, and mechanical stress due to wind variances, leading early researchers to conclude that it’s longevity is intimately associated with adversity [52-54]. Conversely, Pinus Longaeva species that are located in less stressful environments (i.e. lower elevations) are strongly associated with younger age classes (<875 years) [55]. Similarly, the Creosote bush (Larrea tridentate), considered one of the oldest living clonal organisms on the planet (>11,000 years old), also thrives in harsh environments including the Mohave Desert [56]. AMPK, which increases lifespan and healthspan in several model organisms, is the primary sensor of cellular stress in eukaryotic organisms (e.g. plants and humans) and the plant AMPK orthologue SnRK1 as well as Ca2+ and ROS are critical for seed germination, fertilization, root gravitropism, and secondary metabolite production [57-64]. The secondary plant metabolites PMA (which activates mouse oocytes and promotes the acrosome reaction in human sperm) and artemisinin (an anti-malarial drug) both activate AMPK and the antibiotic A23187 also increases production of the secondary metabolite resveratrol in grape cell cultures, again indicating that exposure to low-level stressors may promote extension of lifespan and initiate the creation of human life [17,23,39,65,66].
Organismal exposure to beneficial levels of stress may also play a critical role in evolution. As first noted by Charles Darwin, evolution is driven by natural selection, a process characterized by environmentally-induced phenotypic changes that may lead to inheritable survival and reproductive advantages [67]. From “On the Origin of Species by Means of Natural Selection, or the Preservation of Favoured Races in the Struggle for Life”, Darwin explained that “if there be, owing to the high geometrical powers of increase of each species, at some age, season, or year, a severe struggle for life, and this certainly cannot be disputed;……But if variations useful to any organic being do occur, assuredly individuals thus characterised will have the best chance of being preserved in the struggle for life;” [67]. This “struggle for life” Darwin spoke of is embodied by selective pressures which may be abiotic (i.e. light, wind, temperature, etc.) or biotic (predation, disease, competition, etc.) [68,69]. As alluded to above, such selective pressures are indeed sources of cellular stress, sensed by both prokaryotes and eukaryotes, that induce beneficial responses (at appropriate levels), leading to the acquisition of phenotypes conducive for continued survival. Both biotic (e.g. infection) and abiotic (e.g. heat) stressors/selective pressures activate AMPK (which is evolutionarily conserved among eukaryotes) in human cells [70,71]. A phenomenon often cited as an example of natural selection on a readily observable timescale is the development of bacterial resistance to antibiotics, resulting in problematic mutant strains that may be life-threatening for some individuals (i.e. the elderly and immunocompromised) [72]. Intriguingly, lethal levels of bactericidal antibiotics have been shown to kill microorganisms via the induction of ROS, sub-lethal levels of bactericidal antibiotics however increase mutagenesis and bacterial resistance via induction of lower levels of ROS, and heat as well as nutritional stress increase bacterial resistance to antibiotics, providing compelling evidence that continuous exposure to low levels of stress likely plays a significant role in natural selection and evolution [73-75].
Moreover, gravity itself likely functions as a cellular stressor/selective pressure that has influenced the development of organisms on Earth since the emergence of the very first lifeform. Gravity exerts its effects on living organisms via the application of force, which is experienced by human cells in the form of mechanical loading or stress [76]. The application of force or a mechanical load has recently been shown to activate AMPK and simulated microgravity (i.e. hind limb unloading in mice) significantly decreases AMPK activation [77,78]. Spaceflight also inhibits the activation of T cells (immune cells essential for adaptive immunity), whereas the application of force and AMPK activation promote T cell activation [79-81]. Interestingly, spaceflight has recently been shown to decrease the levels of the master antioxidant transcription factor Nrf2 and the heat shock-inducible protein HSP90a but increase the levels of the growth-promoting kinase mTOR in mice [82]. AMPK however inhibits mTOR but increases the phosphorylation, nuclear retention, and transcriptional activity of Nrf2 [57,83,84]. Also, HSP90 interacts with and maintains AMPK activity and HSP90 is necessary for progesterone-induced human sperm acrosome reaction [85,86]. Interestingly, rapamycin, an immunosuppressant produced by Streptomyces hygroscopicus, extends lifespan in genetically heterogeneous mice, activates AMPK in vivo in normal aged mice, and increases human sperm motility [42,87,88]. Simulated microgravity via the use of NASA-designed rotating wall vessels (RWVs) however drastically reduces rapamycin production (~90%) whereas the antibiotic gentamycin increases rapamycin production by Streptomyces hygroscopicus, providing further evidence that cellular stress, in the form of mechanical loading induced by gravity, is essential for development, function, and survival of Earth-bound organisms [89,90].
The induction of cellular stress also links seemingly dissimilar physiological and pathological states with the activation of AMPK. As discussed above, both ionomycin and ROS activate AMPK and promote oocyte meiotic resumption, a process that is AMPK-dependent and is essential for efficient oocyte activation [27,30,91]. ROS is also critical for ovulation, PMA and ionomycin both activate mouse oocytes, and ionomycin is extensively used during ICSI procedures, creating normal healthy children, suggesting that cellular stress-induced AMPK activation is also essential for oocyte activation [3,4,12,17,92]. The activation of oocytes and T cells share strikingly similar intracellular signaling mechanisms (e.g. PLC-PIP2-DAG-PKC-IP3-Ca2+) and ionomycin combined with PMA are extremely effective in activating T cells and are often used as positive controls in HIV-1 latency reversal studies [93-95]. Reactivating latent/dormant HIV-1 in CD4+ T cells, potentially facilitating immune system detection and virus destruction, is currently being pursued as a method for the potential eradication of HIV-1 (called the “shock and kill” approach) [96]. Similar to oocyte activation, both Ca2+ and ROS are critical for T cell activation (and hence latent HIV-1 reactivation) and other cellular stress-inducing compounds, including NDGA derived from the Creosote bush, butyrate derived from bacteria, as well as ROS and HSP90 have been shown to reactivate latent HIV-1 [26,93,94,97-101]. Interestingly, AMPK inhibition leads to cell death on T cell activation, knockdown of AMPK significantly decreases HIV-1 replication, and metformin (a well-studied AMPK activator derived from the French Lilac plant) increases butyrate production in human diabetic patients [81,102,103]. Perhaps most convincingly, early preliminary data showed that metformin significantly reduced several markers preferentially associated with cells latently infected with HIV-1 (e.g. PD-1, TIGIT, TIM-3) and also destabilized the latent HIV-1 reservoir in chronically-infected HIV patients, indicating that cellular-stress induced AMPK activation likely links the creation of human life with the potential eradication of HIV-1, as I originally proposed in 2016 [40,104,105].
AMPK activation may also link the disparate disease states of HIV-1 latency and Hutchinson-Gilford progeria syndrome (HGPS). HGPS is a genetic disorder caused by aberrant alternative splicing of the LMNA gene, generating a toxic protein called progerin that induces an accelerated aging phenotype and premature death at approximately 14 years of age [106]. Excessive activity of the gene splicing factor SRSF1 has been shown to prevent reactivation of latent HIV-1 and contribute to aberrant splicing of the LMNA gene in HGPS [107-109]. Metformin however has recently been shown to ameliorate the accelerated aging phenotype in cells derived from children with HGPS by reducing the levels of both SRSF1 and progerin and activating AMPK, as I first proposed in 2014 [110-112]. Interestingly, both Ca2+ and ROS induce autophagy (a process of disposing of damaged/toxic proteins and organelles) and rapamycin, which activates AMPK in vivo and increases intracellular Ca2+ levels, improves accelerated aging in progeria cells by inducing autophagic degradation of progerin [87,113-116]. Temsirolimus, an analog of rapamycin, also alleviated accelerated aging defects in progeria cells but also increased the levels of ROS and superoxide within the first hour of treatment [117]. Such evidence strongly suggests that cellular stress-induced AMPK activation links the reversal of HIV-1 latency and alleviation of accelerated cellular aging defects in HGPS.
Cellular stress-induced AMPK activation also links the potential elimination of cancer stem cells (CSCs) with HIV-1 latency reversal and viral eradication. CSCs, which are largely resistant to chemoradiation therapy, are a subpopulation of cancer cells that exhibit characteristics similar to embryonic stem cells (ESCs), including self-renewal, multi-lineage differentiation, & the ability to initiate tumorigenesis [118,119]. Mechanisms that sustain quiescence & promote self-renewal in adult stem cells (ASCs) & CSCs likely also function to maintain latency of HIV-1 in CD4+ memory T cells. Indeed, HIV-1 has been found to establish long-lasting latency in a recently discovered subset of CD4+ T cells that exhibit stem cell-like properties known as T memory stem (TSCM) cells and increases in Ca2+, ROS, and AMPK activation have been shown to promote T cell activation and ESC, ASC, and CSC differentiation [119,120]. Additionally, A23187 and PMA have been shown to promote CSC differentiation (causing CSCs to become more susceptible to chemoradiation) and metformin induces CSC differentiation and/or apoptosis in an AMPK-dependent manner in the deadliest of cancers, including glioblastoma and pancreatic cancer, providing support for my publication in 2017 in which I first proposed that CSC differentiation and/or apoptosis and HIV-1 latency reversal/viral eradication may be linked by cellular stress-induced AMPK activation [119,121-124].
In conclusion, the ability of non-human organisms including certain Streptomyces spp. to initiate the creation of human life is predicated on the induction of cellular stress, mediated by increases in intracellular ROS, Ca2+, AMP(ADP)/ATP ratio increase, etc. The beneficial effects of transient cellular stress induction, which may be likened to selective pressures, crosses species boundaries and may indeed play a role in facilitating natural selection, a process that underlies and drives evolution, as evidenced by stress-induced increases in antibiotic production by Streptomyces spp. and stress-induced mutagenesis and antibiotic resistance in various bacterial strains. Because AMPK, a primary sensor of cellular stress in eukaryotic cells that increases lifespan and healthspan, plays a critical role in oocyte meiotic resumption/maturation, T cell activation, and stem cell differentiation, the creation of human life, the potential eradication of HIV-1, amelioration of accelerated aging in HGPS cells, and CSC differentiation/apoptosis are likely linked by a “Shock to Live”, or a “Shock to Kill”.
https://www.linkedin.com/pulse/antibiotics-produced-bacteria-activate-human-oocytes-creating-finley/



References:
Murugesu S, Saso S, Jones BP, et al. Does the use of calcium ionophore during artificial oocyte activation demonstrate an effect on pregnancy rate? A meta-analysis. Fertil Steril. 2017 Sep;108(3):468-482.e3.
Tesarik J, Sousa M, Testart J. Human oocyte activation after intracytoplasmic sperm injection. Hum Reprod. 1994 Mar;9(3):511-8.
Deemeh MR, Tavalaee M, Nasr-Esfahani MH. Health of children born through artificial oocyte activation: a pilot study. Reprod Sci. 2015 Mar;22(3):322-8.
Eftekhar M, Janati S, Rahsepar M, Aflatoonian A. Effect of oocyte activation with calcium ionophore on ICSI outcomes in teratospermia: A randomized clinical trial. Iran J Reprod Med. 2013 Nov;11(11):875-82.
Liu WC, Slusarchyk DS, Astle G, Trejo WH, Brown WE, Meyers E. Ionomycin, a new polyether antibiotic. J Antibiot (Tokyo). 1978 Sep;31(9):815-9.
Reed PW, Lardy HA. A23187: a divalent cation ionophore. J Biol Chem. 1972 Nov 10;247(21):6970-7.
Zhang Z, Wang T, Hao Y, et al. Effects of trehalose vitrification and artificial oocyte activation on the development competence of human immature oocytes. Cryobiology. 2017 Feb;74:43-49.
De Sutter P, Dozortsev D, Cieslak J, Wolf G, Verlinsky Y, Dyban A. Parthenogenetic activation of human oocytes by puromycin. J Assist Reprod Genet. 1992 Aug;9(4):328-37.
Sankaran L, Pogell BM. Biosynthesis of puromycin in Streptomyces alboniger: regulation and properties of O-demethylpuromycin O-methyltransferase. Antimicrob Agents Chemother. 1975 Dec;8(6):721-32.
Tesarik J, Rienzi L, Ubaldi F, Mendoza C, Greco E. Use of a modified intracytoplasmic sperm injection technique to overcome sperm-borne and oocyte-borne oocyte activation failures. Fertil Steril. 2002 Sep;78(3):619-24.
Mansour R, Fahmy I, Tawab NA, et al. Electrical activation of oocytes after intracytoplasmic sperm injection: a controlled randomized study. Fertil Steril. 2009 Jan;91(1):133-9.
Nikiforaki D, Vanden Meerschaut F, de Roo C, et al. Effect of two assisted oocyte activation protocols used to overcome fertilization failure on the activation potential and calcium releasing pattern. Fertil Steril. 2016 Mar;105(3):798-806.e2.
Liu H1, Zhang J, Krey LC, Grifo JA. In-vitro development of mouse zygotes following reconstruction by sequential transfer of germinal vesicles and haploid pronuclei. Hum Reprod. 2000 Sep;15(9):1997-2002.
Downs SM. Stimulation of parthenogenesis in mouse ovarian follicles by inhibitors of inosine monophosphate dehydrogenase. Biol Reprod. 1990 Sep;43(3):427-36.
Siracusa G, Whittingham DG, Molinaro M, Vivarelli E. Parthenogenetic activation of mouse oocytes induced by inhibitors of protein synthesis. J Embryol Exp Morphol. 1978 Feb;43:157-66.
Carvacho I, Lee HC, Fissore RA, Clapham DE. TRPV3 channels mediate strontium-induced mouse-egg activation. Cell Rep. 2013 Dec 12;5(5):1375-86.
Colonna R, Tatone C, Malgaroli A, Eusebi F, Mangia F. Effects of protein kinase C stimulation and free Ca2+ rise in mammalian egg activation. Gamete Res. 1989 Oct;24(2):171-83.
Tang Z, Xing F, Chen D, et al. In vivo toxicological evaluation of Anisomycin. Toxicol Lett. 2012 Jan 5;208(1):1-11.
Regueira TB, Kildegaard KR, Hansen BG, Mortensen UH, Hertweck C, Nielsen J. Molecular basis for mycophenolic acid biosynthesis in Penicillium brevicompactum. Appl Environ Microbiol. 2011 May;77(9):3035-43.
K'ominek LA. Cycloheximide production by Streptomyces griseus: control mechanisms of cycloheximide biosynthesis. Antimicrob Agents Chemother. 1975 Jun;7(6):856-6.
Ultee A, Slump RA, Steging G, Smid EJ. Antimicrobial activity of carvacrol toward Bacillus cereus on rice. J Food Prot. 2000 May;63(5):620-4.
Pal PK, Nandi MK, Singh NK. Detoxification of Croton tiglium L. seeds by Ayurvedic process of Śodhana. Anc Sci Life. 2014 Jan;33(3):157-61.
Rotem R, Paz GF, Homonnai ZT, et al. Ca(2+)-independent induction of acrosome reaction by protein kinase C in human sperm. Endocrinology. 1992 Nov;131(5):2235-43.
de Lamirande E, Tsai C, Harakat A, Gagnon C. Involvement of reactive oxygen species in human sperm arcosome reaction induced by A23187, lysophosphatidylcholine, and biological fluid ultrafiltrates. J Androl. 1998 Sep-Oct;19(5):585-94.
Görlach A, Bertram K, Hudecova S, Krizanova O. Calcium and ROS: A mutual interplay. Redox Biol. 2015 Dec;6:260-71.
Nasr-Esfahani MM, Johnson MH. The origin of reactive oxygen species in mouse embryos cultured in vitro. Development. 1991 Oct;113(2):551-60.
LaRosa C, Downs SM. Stress stimulates AMP-activated protein kinase and meiotic resumption in mouse oocytes. Biol Reprod. 2006 Mar;74(3):585-92.
Calle-Guisado V, de Llera AH, Martin-Hidalgo D, et al. AMP-activated kinase in human spermatozoa: identification, intracellular localization, and key function in the regulation of sperm motility. Asian J Androl. 2016 Sep 27.
Mungai PT, Waypa GB, Jairaman A, et al. Hypoxia triggers AMPK activation through reactive oxygen species-mediated activation of calcium release-activated calcium channels. Mol Cell Biol. 2011 Sep;31(17):3531-45.
Tamas P, Hawley SA, Clarke RG, et al. Regulation of the energy sensor AMP-activated protein kinase by antigen receptor and Ca2+ in T lymphocytes. J Exp Med 2006;203(7):1665–70.
Hawley SA, Pan DA, Mustard KJ, et al. Calmodulin-dependent protein kinase kinase-beta is an alternative upstream kinase for AMP-activated protein kinase. Cell Metab. 2005 Jul;2(1):9-19.
Nammi S, Roufogalis BD. Light-to-moderate ethanol feeding augments AMPK-α phosphorylation and attenuates SREBP-1 expression in the liver of rats. J Pharm Pharm Sci. 2013;16(2):342-51.
Koh W, Jeong SJ, Lee HJ, et al. Melatonin promotes puromycin-induced apoptosis with activation of caspase-3 and 5’-adenosine monophosphate-activated kinase-alpha in human leukemia HL-60 cells. J Pineal Res. 2011 May;50(4):367-73.
Bays JL, Campbell HK, Heidema C, Sebbagh M, DeMali KA. Linking E-cadherin mechanotransduction to cell metabolism through force-mediated activation of AMPK. Nat Cell Biol. 2017 Jun;19(6):724-731.
Hutber CA, Hardie DG, Winder WW. Electrical stimulation inactivates muscle acetyl-CoA carboxylase and increases AMP-activated protein kinase. Am J Physiol. 1997 Feb;272(2 Pt 1):E262-6.
Ohsaka Y, Nishino H, Nomura Y. Induction of phospho-Thr-172 AMPK in 3T3-L1 adipocytes exposed to cold or treated with anisomycin, mithramycin A, and ionic compounds. Cryo Letters. 2010 May-Jun;31(3):218-29.
Fernández-Ramos AA, Marchetti-Laurent C, Poindessous V, et al. A comprehensive characterization of the impact of mycophenolic acid on the metabolism of Jurkat T cells. Sci Rep. 2017 Sep 5;7(1):10550.
Kim E, Choi Y, Jang J, Park T. Carvacrol Protects against Hepatic Steatosis in Mice Fed a High-Fat Diet by Enhancing SIRT1-AMPK Signaling. Evid Based Complement Alternat Med. 2013;2013:290104.
Zogovic N, Tovilovic-Kovacevic G, Misirkic-Marjanovic M, et al. Coordinated activation of AMP-activated protein kinase, extracellular signal-regulated kinase, and autophagy regulates phorbol myristate acetate-induced differentiation of SH-SY5Y neuroblastoma cells. J Neurochem. 2015 Apr;133(2):223-32.
Finley J. Oocyte activation and latent HIV-1 reactivation: AMPK as a common mechanism of action linking the beginnings of life and the potential eradication of HIV-1. Med Hypotheses. 2016 Aug;93:34-47.
Kitani S1, Miyamoto KT, Takamatsu S, et al. Avenolide, a Streptomyces hormone controlling antibiotic production in Streptomyces avermitilis. Proc Natl Acad Sci U S A. 2011 Sep 27;108(39):16410-5.
Challis GL, Hopwood DA. Synergy and contingency as driving forces for the evolution of multiple secondary metabolite production by Streptomyces species. Proc Natl Acad Sci U S A. 2003 Nov 25;100 Suppl 2:14555-61.
Yagüe P, López-García MT, Rioseras B, Sánchez J, Manteca A. Pre-sporulation stages of Streptomyces differentiation: state-of-the-art and future perspectives. FEMS Microbiol Lett. 2013 May;342(2):79-88.
Seipke RF, Kaltenpoth M, Hutchings MI. Streptomyces as symbionts: an emerging and widespread theme? FEMS Microbiol Rev. 2012 Jul;36(4):862-76.
Meng L, Li M, Yang SH, Kim TJ, Suh JW. Intracellular ATP levels affect secondary metabolite production in Streptomyces spp. Biosci Biotechnol Biochem. 2011;75(8):1576-81.
Sánchez S, Chávez A, Forero A, et al. Carbon source regulation of antibiotic production. J Antibiot (Tokyo). 2010 Aug;63(8):442-59.
Wang SL, Fan KQ, Yang X, Lin ZX, Xu XP, Yang KQ. CabC, an EF-hand calcium-binding protein, is involved in Ca2+-mediated regulation of spore germination and aerial hypha formation in Streptomyces coelicolor. J Bacteriol. 2008 Jun;190(11):4061-8.
Wang D, Wei L, Zhang Y, Zhang M, Gu S. Physicochemical and microbial responses of Streptomyces natalensis HW-2 to fungal elicitor. Appl Microbiol Biotechnol. 2017 Jul 28. doi: 10.1007/s00253-017-8440-0. [Epub ahead of print].
Wei ZH, Bai L, Deng Z, Zhong JJ. Enhanced production of validamycin A by H2O2-induced reactive oxygen species in fermentation of Streptomyces hygroscopicus 5008. Bioresour Technol. 2011 Jan;102(2):1783-7.
Doull JL, Ayer SW, Singh AK, Thibault P. Production of a novel polyketide antibiotic, jadomycin B, by Streptomyces venezuelae following heat shock. J Antibiot (Tokyo). 1993 May;46(5):869-71.
Zhou WW1, Ma B, Tang YJ, Zhong JJ, Zheng X. Enhancement of validamycin A production by addition of ethanol in fermentation of Streptomyces hygroscopicus 5008. Bioresour Technol. 2012 Jun;114:616-21.
Flanary BE, Kletetschka G. Analysis of telomere length and telomerase activity in tree species of various lifespans, and with age in the bristlecone pine Pinus longaeva. Rejuvenation Res. 2006 Spring;9(1):61-3.
R. S. BeasleyJ. O. Klemmedson. Recognizing site adversity and drought-sensitive trees in stands of bristlecone pine (Pinus longaeva). January 1973, Volume 27, Issue 1, pp 141–146. doi: 10.1007/BF02862228.
Schulman E. Longevity under Adversity in Conifers. Science. 1954 Mar 26;119(3091):396-9.
Hiebert, R. D.; Hamrick, J. L. 1984. An ecological study of bristlecone pine (Pinus longaeva) in Utah and eastern Nevada. The Great Basin Naturalist. 44(3): 487-494.
Jorquera MA, Shaharoona B, Nadeem SM, de la Luz Mora M, Crowley DE. Plant growth-promoting rhizobacteria associated with ancient clones of creosote bush (Larrea tridentata). Microb Ecol. 2012 Nov;64(4):1008-17.
Salminen A, Kaarniranta K. AMP-activated protein kinase (AMPK) controls the aging process via an integrated signaling network. Ageing Res Rev. 2012 Apr;11(2):230-41.
Leymarie J, Vitkauskaité G, Hoang HH, et al. Role of reactive oxygen species in the regulation of Arabidopsis seed dormancy. Plant Cell Physiol. 2012 Jan;53(1):96-106.
Pang X, Halaly T, Crane O, et al. Involvement of calcium signalling in dormancy release of grape buds. J Exp Bot. 2007;58(12):3249-62.
Duan Q, Kita D, Johnson EA, et al. Reactive oxygen species mediate pollen tube rupture to release sperm for fertilization in Arabidopsis. Nat Commun. 2014;5:3129.
Gao XQ, Liu CZ, Li DD, et al. The Arabidopsis KINβγ Subunit of the SnRK1 Complex Regulates Pollen Hydration on the Stigma by Mediating the Level of Reactive Oxygen Species in Pollen. PLoS Genet. 2016 Jul 29;12(7):e1006228.
Joo JH, Bae YS, Lee JS. Role of auxin-induced reactive oxygen species in root gravitropism. Plant Physiol. 2001 Jul;126(3):1055-60.
Lee JS, Mulkey TJ, Evans ML. Reversible loss of gravitropic sensitivity in maize roots after tip application of calcium chelators. Science. 1983 Jun 24;220(4604):1375-6.
Zhao J, Davis LC, Verpoorte R. Elicitor signal transduction leading to production of plant secondary metabolites. Biotechnol Adv. 2005 Jun;23(4):283-333.
Wang H, Sharma L, Lu J, Finch P, Fletcher S, Prochownik EV. Structurally diverse c-Myc inhibitors share a common mechanism of action involving ATP depletion. Oncotarget. 2015 Jun 30;6(18):15857-70.
K.V. Kiselev, O.A. Shumakova, A.Y. Manyakhin, A.N. Mazeika. Influence of calcium influx induced by the calcium ionophore, A23187, on resveratrol content and the expression of CDPK and STS genes in the cell cultures of Vitis amurensis. Plant Growth Regulation. December 2012, Volume 68, Issue 3, pp 371–381. DOI: 10.1007/s10725-012-9725-z.
Darwin, Charles (1859). On the origin of species by means of natural selection, or the preservation of favoured races in the struggle for life. London: Murray. [1st ed.].
Sobral M, Veiga T, Domínguez P, Guitián JA, Guitián P, Guitián JM. Selective Pressures Explain Differences in Flower Color among Gentiana lutea Populations. PLoS One. 2015 Jul 14;10(7):e0132522.
Lefebvre V, Kiani SP, Durand-Tardif M. A focus on natural variation for abiotic constraints response in the model species Arabidopsis thaliana. Int J Mol Sci. 2009 Aug 13;10(8):3547-82.
Moser TS, Schieffer D, Cherry S. AMP-activated kinase restricts Rift Valley fever virus infection by inhibiting fatty acid synthesis. PLoS Pathog. 2012;8(4):e1002661.
Goto A, Egawa T, Sakon I, et al. Heat stress acutely activates insulin-independent glucose transport and 5’-AMP-activated protein kinase prior to an increase in HSP72 protein in rat skeletal muscle. Physiol Rep. 2015 Nov;3(11). pii: e12601.
Maclean RC, Hall AR, Perron GG, Buckling A. The evolution of antibiotic resistance: insight into the roles of molecular mechanisms of resistance and treatment context. Discov Med. 2010 Aug;10(51):112-8.
Dwyer DJ, Belenky PA, Yang JH, et al. Antibiotics induce redox-related physiological alterations as part of their lethality. Proc Natl Acad Sci U S A. 2014 May 20;111(20):E2100-9.
Kohanski MA, DePristo MA, Collins JJ. Sublethal antibiotic treatment leads to multidrug resistance via radical-induced mutagenesis. Mol Cell. 2010 Feb 12;37(3):311-20.
Poole K. Bacterial stress responses as determinants of antimicrobial resistance. J Antimicrob Chemother. 2012 Sep;67(9):2069-89.
Ito M, Arakawa T, Okayama M, Shitara A, Mizoguchi I, Takuma T. Gravity loading induces adenosine triphosphate release and phosphorylation of extracellular signal-regulated kinases in human periodontal ligament cells. J Investig Clin Dent. 2014 Nov;5(4):266-74.
Bays JL, Campbell HK, Heidema C, Sebbagh M, DeMali KA. Linking E-cadherin mechanotransduction to cell metabolism through force-mediated activation of AMPK. Nat Cell Biol. 2017 Jun;19(6):724-731.
Zhong G, Li Y, Li H, et al. Simulated Microgravity and Recovery-Induced Remodeling of the Left and Right Ventricle. Front Physiol. 2016 Jun 29;7:274.
Chang TT, Walther I, Li CF, et al. The Rel/NF-jB pathway and transcription of immediate early genes in T cell activation are inhibited by microgravity. J Leukoc Biol 2012;92(6):1133–45.
Li YC, Chen BM, Wu PC, et al. Cutting Edge: mechanical forces acting on T cells immobilized via the TCR complex can trigger TCR signaling. J Immunol 2010;184(11):5959–63.
Ouyang Z, Wang X, Meng Q, et al. Suppression of adenosine monophosphate-activated protein kinase selectively triggers apoptosis in activated T cells and ameliorates immune diseases. Biochem Biophys Res Commun. 2017 May 27;487(2):223-229.
Blaber EA, Pecaut MJ, Jonscher KR. Spaceflight Activates Autophagy Programs and the Proteasome in Mouse Liver. Int J Mol Sci. 2017 Sep 27;18(10). pii: E2062.
Mo C, Wang L, Zhang J, et al. The crosstalk between Nrf2 and AMPK signal pathways is important for the anti-inflammatory effect of berberine in LPS-stimulated macrophages and endotoxin-shocked mice. Antioxid Redox Signal. 2014 Feb 1;20(4):574-88.
Joo MS, Kim WD, Lee KY, Kim JH, Koo JH, Kim SG. AMPK facilitates nuclear accumulation of Nrf2 by phosphorylating at serine 550. Mol Cell Biol. 2016 Jun 29;36(14):1931-42.
Zhang L, Yi Y, Guo Q, et al. Hsp90 interacts with AMPK and mediates acetyl-CoA carboxylase phosphorylation. Cell Signal. 2012 Apr;24(4):859-65.
Sagare-Patil V, Bhilawadikar R, Galvankar M, Zaveri K, Hinduja I, Modi D. Progesterone requires heat shock protein 90 (HSP90) in human sperm to regulate motility and acrosome reaction. J Assist Reprod Genet. 2017 Apr;34(4):495-503.
Lesniewski LA, Seals DR4, Walker AE, et al. Dietary rapamycin supplementation reverses age-related vascular dysfunction and oxidative stress, while modulating nutrient-sensing, cell cycle, and senescence pathways. Aging Cell. 2017 Feb;16(1):17-26.
Aparicio IM, Espino J, Bejarano I, et al. Autophagy-related proteins are functionally active in human spermatozoa and may be involved in the regulation of cell survival and motility. Sci Rep. 2016 Sep 16;6:33647.
Fang A, Pierson DL, Mishra SK, Demain AL. Growth of Steptomyces hygroscopicus in rotating-wall bioreactor under simulated microgravity inhibits rapamycin production. Appl Microbiol Biotechnol. 2000 Jul;54(1):33-6.
Cheng YR, Huang J, Qiang H, Lin WL, Demain AL. Mutagenesis of the rapamycin producer Streptomyces hygroscopicus FC904. J Antibiot (Tokyo). 2001 Nov;54(11):967-72.
Heindryckx B, Lierman S, Combelles CM, Cuvelier CA, Gerris J, De Sutter P. Aberrant spindle structures responsible for recurrent human metaphase I oocyte arrest with attempts to induce meiosis artificially. Hum Reprod. 2011 Apr;26(4):791-800.
Shkolnik K, Tadmor A, Ben-Dor S, Nevo N, Galiani D, Dekel N. Reactive oxygen species are indispensable in ovulation. Proc Natl Acad Sci U S A. 2011 Jan 25;108(4):1462-7.
Amdani SN, Jones C, Coward K. Phospholipase C zeta (PLC ζ): oocyte activation and clinical links to male factor infertility. Adv Biol Regul 2013;53(3):292–308.
Smith-Garvin JE, Koretzky GA, Jordan MS. T cell activation. Annu Rev Immunol 2009;27:591–619.
Spina CA, Anderson J, Archin NM, et al. An in-depth comparison of latent HIV-1 reactivation in multiple cell model systems and resting CD4+ T cells from aviremic patients. PLoS Pathog 2013;9(12):e1003834.
Marsden MD, Zack JA. HIV/AIDS eradication. Bioorg Med Chem Lett 2013;23(14):4003–10.
Sena LA, Li S, Jairaman A, et al. Mitochondria are required for antigen-specific T cell activation through reactive oxygen species signaling. Immunity. 2013 Feb 21;38(2):225-36.
Barquero AA, Dávola ME, Riva DA, Mersich SE, Alché LE. Naturally occurring compounds elicit HIV-1 replication in chronically infected promonocytic cells. Biomed Res Int. 2014;2014:989101.
Imai K, Ochiai K, Okamoto T. Reactivation of latent HIV-1 infection by the periodontopathic bacterium Porphyromonas gingivalis involves histone modification. J Immunol. 2009 Mar 15;182(6):3688-95.
Piette J, Legrand-Poels S. HIV-1 reactivation after an oxidative stress mediated by different reactive oxygen species. Chem Biol Interact. 1994 Jun;91(2-3):79-89.
Anderson I, Low JS, Weston S, et al. Heat shock protein 90 controls HIV-1 reactivation from latency. Proc Natl Acad Sci U S A. 2014 Apr 15;111(15):E1528-37.
Zhou H, Xu M, Huang Q, et al. Genome-scale RNAi screen for host factors required for HIV replication. Cell Host Microbe. 2008 Nov 13;4(5):495-504.
Wu H, Esteve E, Tremaroli V, et al. Metformin alters the gut microbiome of individuals with treatment-naive type 2 diabetes, contributing to the therapeutic effects of the drug. Nat Med. 2017 Jul;23(7):850-858.
G.M. Chew, D.C. Chow, S.A. Souza, et al. Impact of adjunctive metformin therapy on T cell exhaustion and viral persistence in a clinical trial of HIV-infected adults on suppressive ART. Journal of Virus Eradication 2017; 3 (Supplement 1): 6–19.
http://viruseradication.com/supplement-details/Abstracts_of_the_IAS_HIV_Cure_and_Cancer_Forum_2017/
Ullrich NJ, Gordon LB. Hutchinson-Gilford progeria syndrome. Handb Clin Neurol. 2015;132:249-64.
Berro R, Kehn K, de la Fuente C, et al. Acetylated Tat regulates human immunodeficiency virus type 1 splicing through its interaction with the splicing regulator p32. J Virol 2006;80(7):3189–204.
Paz S, Lu ML, Takata H, Trautmann L, Caputi M. SRSF1 RNA Recognition Motifs Are Strong Inhibitors of HIV-1 Replication. J Virol. 2015 Jun;89(12):6275-86.
Lopez-Mejia IC, Vautrot V, De Toledo M, et al. A conserved splicing mechanism of the LMNA gene controls premature aging. Hum Mol Genet. 2011 Dec 1;20(23):4540-55.
Park SK, Shin OS. Metformin alleviates ageing cellular phenotypes in Hutchinson-Gilford progeria syndrome dermal fibroblasts. Exp Dermatol. 2017 Oct;26(10):889-895.
Egesipe AL, Blondel S, Cicero AL, et al. Metformin decreases progerin expression and alleviates pathological defects of Hutchinson-Gilford progeria syndrome cells. NPJ Aging Mech Dis. 2016 Nov 10;2:16026.
Finley J. Alteration of splice site selection in the LMNA gene and inhibition of progerin production via AMPK activation. Med Hypotheses. 2014 Nov;83(5):580-7.
Decuypere JP, Welkenhuyzen K, Luyten T, et al. Ins(1,4,5)P3 receptor-mediated Ca2+ signaling and autophagy induction are interrelated. Autophagy. 2011 Dec;7(12):1472-89.
Cao Y, Fang Y, Cai J, et al. ROS functions as an upstream trigger for autophagy to drive hematopoietic stem cell differentiation. Hematology. 2016 Dec;21(10):613-618.
Decuypere JP, Kindt D, Luyten T, et al. mTOR-Controlled Autophagy Requires Intracellular Ca(2+) Signaling. PLoS One. 2013;8(4):e61020.
Cao K, Graziotto JJ, Blair CD, et al. Rapamycin reverses cellular phenotypes and enhances mutant protein clearance in Hutchinson-Gilford progeria syndrome cells. Sci Transl Med. 2011 Jun 29;3(89):89ra58.
Gabriel D, Gordon LB, Djabali K. Temsirolimus Partially Rescues the Hutchinson-Gilford Progeria Cellular Phenotype. PLoS One. 2016 Dec 29;11(12):e0168988.
Moncharmont C, Levy A, Gilormini M, et al. Targeting a cornerstone of radiation resistance: cancer stem cell. Cancer Lett 2012;322(2):139–47.
Finley J. Elimination of cancer stem cells and reactivation of latent HIV-1 via AMPK activation: Common mechanism of action linking inhibition of tumorigenesis and the potential eradication of HIV-1. Med Hypotheses. 2017 Jul;104:133-146.
Buzon MJ, Sun H, Li C, et al. HIV-1 persistence in CD4+ T cells with stem cell-like properties. Nat Med 2014;20(2):139–42.
Wee S, Niklasson M, Marinescu VD, et al. Selective calcium sensitivity in immature glioma cancer stem cells. PLoS One. 2014 Dec 22;9(12):e115698.
Hayun M, Okun E, Hayun R, Gafter U, et al. Synergistic effect of AS101 and Bryostatin-1 on myeloid leukemia cell differentiation in vitro and in an animal model. Leukemia 2007;21(7):1504–13.
Sato A, Sunayama J, Okada M, et al. Glioma-initiating cell elimination by metformin activation of FOXO3 via AMPK. Stem Cells Transl Med 2012;1(11):811–24.
Fasih A, Elbaz HA, Hüttemann M, Konski AA, Zielske SP. Radiosensitization of pancreatic cancer cells by metformin through the AMPK pathway. Radiat Res 2014;182(1):50–9.
#ampk#progeria#cancer stem cell#HIV/AIDS#oocyte#sperm#acrosome reaction#gut bacteria#metformin#fertilization#reproduction#evolution#charles darwin
0 notes
Text
Antibiotics produced by Bacteria activate Human Oocytes, creating Healthy Babies: AMPK links the Creation of Human Life with HIV, Progeria, & Cancer
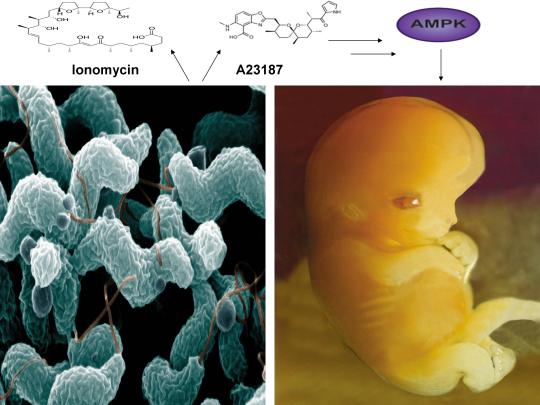
CC-BY-SA-3.0 (http://creativecommons.org/licenses/by-sa/3.0/)], via Wikimedia Commons;By De Wood, Pooley, USDA, ARS, EMU. [Public domain], via Wikimedia Commons
A recent study published online in the journal Fertility and Sterility in September of 2017 systematically reviewed for the first time evidence for the effect of two compounds, ionomycin and A23187 (also known as calcimycin), on fertilization rates and pregnancy outcomes in infertile couples undergoing an in vitro fertilization procedure known as intracytoplasmic sperm injection (ICSI) [1]. ICSI involves the direct deposition of sperm into the oocyte cytoplasm, which typically leads to high rates of fertilization. However, fertilization failure despite repeated ICSI is likely caused by a failure of the oocyte to activate [1]. Physiological oocyte activation is accomplished by the delivery of a sperm-borne oocyte activating factor called phospholipase C zeta (PLCζ). PLCζ activates human oocytes by inducing an intracellular signaling cascade that ultimately results in increased calcium (Ca2+) oscillations in the oocyte, which drives oocyte activation to completion [1]. As oocyte activation is an indispensable prerequisite for the creation of all human life, every human being alive today and any human being that has ever lived began their existence as an activated oocyte [2]. Ionomycin and A23187 increase the levels of intracellular Ca2+ and are thus commonly known as Ca2+ ionophores [1]. The authors of the Fertility and Sterility study showed that over a total of 1,521 ICSI cycles, calcium ionophores including ionomycin and A23187 led to a statistically significant improvement in fertilization, cleavage, blastulation, implantation rates, overall pregnancy, and live-birth rates [1]. Ionomycin and A23187 have also been shown in several independent studies to effectively induce human oocyte activation, leading to the birth of normal, healthy children [3,4].
Strikingly, as described further below, both ionomycin and A23187 are antibiotics that are naturally produced by certain species within the bacterial genus Streptomyces [5,6]. Other structurally distinct compounds and methods have also been shown to induce human oocyte activation, including ethanol, puromycin (an antibiotic and protein synthesis inhibitor produced by Streptomyces alboniger), as well as mechanical manipulation and electrical stimulation, both of with have been reported to result in the creation of normal children [7-11]. Interestingly, as mouse oocytes are considered models for human oocytes, ionomycin, A23187, anisomycin (an antibiotic and protein synthesis inhibitor produced by Streptomyces griseolus), mycophenolic acid (an immunosuppressant produced by the fungus Penicillium brevicompactum), cycloheximide (a protein synthesis inhibitor produced by Streptomyces griseus), carvacrol (a secondary plant metabolite produced by Origanum vulgare{oregano}), and phorbol 12-myristate 13-acetate (PMA, a secondary plant metabolite produced by Croton tiglium) each induce activation of mouse oocytes [12-22]. Ionomycin, A23187, PMA, and reactive oxygen species (ROS) also induce the acrosome reaction in human sperm, a process characterized by the release of hydrolytic enzymes from the head of sperm which is necessary for oocyte penetration and thus indispensable for the creation of all human life outside of a clinical setting (ICSI bypasses the need for oocyte penetration) [23,24]. Additionally, although an over-production of ROS, similar to Ca2+, may lead to deleterious effects including cell death/apoptosis, low levels of ROS have been shown to act as signaling molecules and ROS is significantly increased on or immediately following mouse oocyte activation [25,26].
Furthermore, the master metabolic regulator AMPK is critical for oocyte meiotic resumption and maturation (a process that precedes and is essential for oocyte activation), is found located across the entire acrosome in the head of human sperm, and is activated by increases in ROS and Ca2+ [27-29]. Ionomycin, A23187, ethanol, puromycin, mechanical force, electrical stimulation, anisomycin, mycophenolic acid, carvacrol, and PMA also induce AMPK activation, indicating that a common mechanism of action links chemically distinct compounds with the creation of human life [30-39]. This common mechanism of action likely centers on the induction of cellular stress, mediated by indirect increases in intracellular Ca2+, ROS, and/or the AMP(ADP)/ATP ratio, etc. as I originally proposed in 2016 [40]. Because the bacterial-derived antibiotics ionomycin and A23187 induce both the acrosome reaction in human sperm and human oocyte activation, producing normal, healthy children, it can be said that “non-human organisms have the power to create human life or the power to end life.” As explained below, the beneficial effects of cellular stress induction (i.e. a “shock”) crosses species boundaries and may indeed play a role in facilitating natural selection, a process that underlies and drives evolution.
A number of bacterial species residing within the genus Streptomyces have proven to be extremely important and medicinally valuable as approximately 70% of clinically useful antibiotics are derived from Streptomyces [41]. The antibiotics ionomycin and A23187 are naturally produced by Streptomyces conglobatus and Streptomyces chartreusensis, respectively [5,6]. Other important examples include the antibiotic tetracycline (produced by Streptomyces aureofaciens), the immunosuppressant rapamycin (produced by Streptomyces hygroscopicus), and the anti-helminthic avermectins (produced by Streptomyces avermitilis) [42]. Many soil and aquatic-dwelling species of Streptomyces can be found in harsh environments and are characterized by a unique life cycle, including spore germination followed by vegetative mycelium production, aerial hyphae formation, sporulation (i.e. spore formation), and antibiotic production [43,44]. Curiously, just as cellular stress induction leads to the creation of human life and other beneficial effects in human cells (see below), stress induction also promotes the induction of aerial hyphae formation, sporulation, and antibiotic production in many Streptomyces species (spp.). Indeed, a decrease in the levels of ATP and bacterial growth is associated with sporulation, aerial hyphae formation, and antibiotic production [42,45]. A reduction in glucose/nutritional deprivation, the preferred sugar/carbon source for many Streptomyces spp., also significantly increases antibiotic production [46]. An increase in intracellular ROS and Ca2+ is associated with spore germination, aerial hyphae formation, and antibiotic production [47-49]. Other cellular stressors, including heat shock and ethanol, also significantly increase antibiotic production, provocatively indicating that the effects of cellular stress crosses species boundaries, enhancing bacterial survival and facilitating the creation of human life [50,51].
The beneficial effects of low-level cellular stress induction also extends to plants, as many plants produce secondary metabolites partly for the purpose of self-defense, analogous to antibiotics. Similar to the harsh, stressful environments often inhabited by Streptomyces spp., the Great Basin Bristlecone Pine (Pinus Longaeva), considered the oldest living non-clonal organism on the planet ( >5000 years old), thrives in an exceptionally harsh environment, characterized by increased elevations and exposure to UV radiation, nutritionally-deprived soils, harsh temperatures, and mechanical stress due to wind variances, leading early researchers to conclude that it’s longevity is intimately associated with adversity [52-54]. Conversely, Pinus Longaeva species that are located in less stressful environments (i.e. lower elevations) are strongly associated with younger age classes (<875 years) [55]. Similarly, the Creosote bush (Larrea tridentate), considered one of the oldest living clonal organisms on the planet (>11,000 years old), also thrives in harsh environments including the Mohave Desert [56]. AMPK, which increases lifespan and healthspan in several model organisms, is the primary sensor of cellular stress in eukaryotic organisms (e.g. plants and humans) and the plant AMPK orthologue SnRK1 as well as Ca2+ and ROS are critical for seed germination, fertilization, root gravitropism, and secondary metabolite production [57-64]. The secondary plant metabolites PMA (which activates mouse oocytes and promotes the acrosome reaction in human sperm) and artemisinin (an anti-malarial drug) both activate AMPK and the antibiotic A23187 also increases production of the secondary metabolite resveratrol in grape cell cultures, again indicating that exposure to low-level stressors may promote extension of lifespan and initiate the creation of human life [17,23,39,65,66].
Organismal exposure to beneficial levels of stress may also play a critical role in evolution. As first noted by Charles Darwin, evolution is driven by natural selection, a process characterized by environmentally-induced phenotypic changes that may lead to inheritable survival and reproductive advantages [67]. From “On the Origin of Species by Means of Natural Selection, or the Preservation of Favoured Races in the Struggle for Life”, Darwin explained that “if there be, owing to the high geometrical powers of increase of each species, at some age, season, or year, a severe struggle for life, and this certainly cannot be disputed;……But if variations useful to any organic being do occur, assuredly individuals thus characterised will have the best chance of being preserved in the struggle for life;” [67]. This “struggle for life” Darwin spoke of is embodied by selective pressures which may be abiotic (i.e. light, wind, temperature, etc.) or biotic (predation, disease, competition, etc.) [68,69]. As alluded to above, such selective pressures are indeed sources of cellular stress, sensed by both prokaryotes and eukaryotes, that induce beneficial responses (at appropriate levels), leading to the acquisition of phenotypes conducive for continued survival. Both biotic (e.g. infection) and abiotic (e.g. heat) stressors/selective pressures activate AMPK (which is evolutionarily conserved among eukaryotes) in human cells [70,71]. A phenomenon often cited as an example of natural selection on a readily observable timescale is the development of bacterial resistance to antibiotics, resulting in problematic mutant strains that may be life-threatening for some individuals (i.e. the elderly and immunocompromised) [72]. Intriguingly, lethal levels of bactericidal antibiotics have been shown to kill microorganisms via the induction of ROS, sub-lethal levels of bactericidal antibiotics however increase mutagenesis and bacterial resistance via induction of lower levels of ROS, and heat as well as nutritional stress increase bacterial resistance to antibiotics, providing compelling evidence that continuous exposure to low levels of stress likely plays a significant role in natural selection and evolution [73-75].
Moreover, gravity itself likely functions as a cellular stressor/selective pressure that has influenced the development of organisms on Earth since the emergence of the very first lifeform. Gravity exerts its effects on living organisms via the application of force, which is experienced by human cells in the form of mechanical loading or stress [76]. The application of force or a mechanical load has recently been shown to activate AMPK and simulated microgravity (i.e. hind limb unloading in mice) significantly decreases AMPK activation [77,78]. Spaceflight also inhibits the activation of T cells (immune cells essential for adaptive immunity), whereas the application of force and AMPK activation promote T cell activation [79-81]. Interestingly, spaceflight has recently been shown to decrease the levels of the master antioxidant transcription factor Nrf2 and the heat shock-inducible protein HSP90a but increase the levels of the growth-promoting kinase mTOR in mice [82]. AMPK however inhibits mTOR but increases the phosphorylation, nuclear retention, and transcriptional activity of Nrf2 [57,83,84]. Also, HSP90 interacts with and maintains AMPK activity and HSP90 is necessary for progesterone-induced human sperm acrosome reaction [85,86]. Interestingly, rapamycin, an immunosuppressant produced by Streptomyces hygroscopicus, extends lifespan in genetically heterogeneous mice, activates AMPK in vivo in normal aged mice, and increases human sperm motility [42,87,88]. Simulated microgravity via the use of NASA-designed rotating wall vessels (RWVs) however drastically reduces rapamycin production (~90%) whereas the antibiotic gentamycin increases rapamycin production by Streptomyces hygroscopicus, providing further evidence that cellular stress, in the form of mechanical loading induced by gravity, is essential for development, function, and survival of Earth-bound organisms [89,90].
The induction of cellular stress also links seemingly dissimilar physiological and pathological states with the activation of AMPK. As discussed above, both ionomycin and ROS activate AMPK and promote oocyte meiotic resumption, a process that is AMPK-dependent and is essential for efficient oocyte activation [27,30,91]. ROS is also critical for ovulation, PMA and ionomycin both activate mouse oocytes, and ionomycin is extensively used during ICSI procedures, creating normal healthy children, suggesting that cellular stress-induced AMPK activation is also essential for oocyte activation [3,4,12,17,92]. The activation of oocytes and T cells share strikingly similar intracellular signaling mechanisms (e.g. PLC-PIP2-DAG-PKC-IP3-Ca2+) and ionomycin combined with PMA are extremely effective in activating T cells and are often used as positive controls in HIV-1 latency reversal studies [93-95]. Reactivating latent/dormant HIV-1 in CD4+ T cells, potentially facilitating immune system detection and virus destruction, is currently being pursued as a method for the potential eradication of HIV-1 (called the “shock and kill” approach) [96]. Similar to oocyte activation, both Ca2+ and ROS are critical for T cell activation (and hence latent HIV-1 reactivation) and other cellular stress-inducing compounds, including NDGA derived from the Creosote bush, butyrate derived from bacteria, as well as ROS and HSP90 have been shown to reactivate latent HIV-1 [26,93,94,97-101]. Interestingly, AMPK inhibition leads to cell death on T cell activation, knockdown of AMPK significantly decreases HIV-1 replication, and metformin (a well-studied AMPK activator derived from the French Lilac plant) increases butyrate production in human diabetic patients [81,102,103]. Perhaps most convincingly, early preliminary data showed that metformin significantly reduced several markers preferentially associated with cells latently infected with HIV-1 (e.g. PD-1, TIGIT, TIM-3) and also destabilized the latent HIV-1 reservoir in chronically-infected HIV patients, indicating that cellular-stress induced AMPK activation likely links the creation of human life with the potential eradication of HIV-1, as I originally proposed in 2016 [40,104,105].
AMPK activation may also link the disparate disease states of HIV-1 latency and Hutchinson-Gilford progeria syndrome (HGPS). HGPS is a genetic disorder caused by aberrant alternative splicing of the LMNA gene, generating a toxic protein called progerin that induces an accelerated aging phenotype and premature death at approximately 14 years of age [106]. Excessive activity of the gene splicing factor SRSF1 has been shown to prevent reactivation of latent HIV-1 and contribute to aberrant splicing of the LMNA gene in HGPS [107-109]. Metformin however has recently been shown to ameliorate the accelerated aging phenotype in cells derived from children with HGPS by reducing the levels of both SRSF1 and progerin and activating AMPK, as I first proposed in 2014 [110-112]. Interestingly, both Ca2+ and ROS induce autophagy (a process of disposing of damaged/toxic proteins and organelles) and rapamycin, which activates AMPK in vivo and increases intracellular Ca2+ levels, improves accelerated aging in progeria cells by inducing autophagic degradation of progerin [87,113-116]. Temsirolimus, an analog of rapamycin, also alleviated accelerated aging defects in progeria cells but also increased the levels of ROS and superoxide within the first hour of treatment [117]. Such evidence strongly suggests that cellular stress-induced AMPK activation links the reversal of HIV-1 latency and alleviation of accelerated cellular aging defects in HGPS.
Cellular stress-induced AMPK activation also links the potential elimination of cancer stem cells (CSCs) with HIV-1 latency reversal and viral eradication. CSCs, which are largely resistant to chemoradiation therapy, are a subpopulation of cancer cells that exhibit characteristics similar to embryonic stem cells (ESCs), including self-renewal, multi-lineage differentiation, & the ability to initiate tumorigenesis [118,119]. Mechanisms that sustain quiescence & promote self-renewal in adult stem cells (ASCs) & CSCs likely also function to maintain latency of HIV-1 in CD4+ memory T cells. Indeed, HIV-1 has been found to establish long-lasting latency in a recently discovered subset of CD4+ T cells that exhibit stem cell-like properties known as T memory stem (TSCM) cells and increases in Ca2+, ROS, and AMPK activation have been shown to promote T cell activation and ESC, ASC, and CSC differentiation [119,120]. Additionally, A23187 and PMA have been shown to promote CSC differentiation (causing CSCs to become more susceptible to chemoradiation) and metformin induces CSC differentiation and/or apoptosis in an AMPK-dependent manner in the deadliest of cancers, including glioblastoma and pancreatic cancer, providing support for my publication in 2017 in which I first proposed that CSC differentiation and/or apoptosis and HIV-1 latency reversal/viral eradication may be linked by cellular stress-induced AMPK activation [119,121-124].
In conclusion, the ability of non-human organisms including certain Streptomyces spp. to initiate the creation of human life is predicated on the induction of cellular stress, mediated by increases in intracellular ROS, Ca2+, AMP(ADP)/ATP ratio increase, etc. The beneficial effects of transient cellular stress induction, which may be likened to selective pressures, crosses species boundaries and may indeed play a role in facilitating natural selection, a process that underlies and drives evolution, as evidenced by stress-induced increases in antibiotic production by Streptomyces spp. and stress-induced mutagenesis and antibiotic resistance in various bacterial strains. Because AMPK, a primary sensor of cellular stress in eukaryotic cells that increases lifespan and healthspan, plays a critical role in oocyte meiotic resumption/maturation, T cell activation, and stem cell differentiation, the creation of human life, the potential eradication of HIV-1, amelioration of accelerated aging in HGPS cells, and CSC differentiation/apoptosis are likely linked by a “Shock to Live”, or a “Shock to Kill”.
https://www.linkedin.com/pulse/antibiotics-produced-bacteria-activate-human-oocytes-creating-finley/


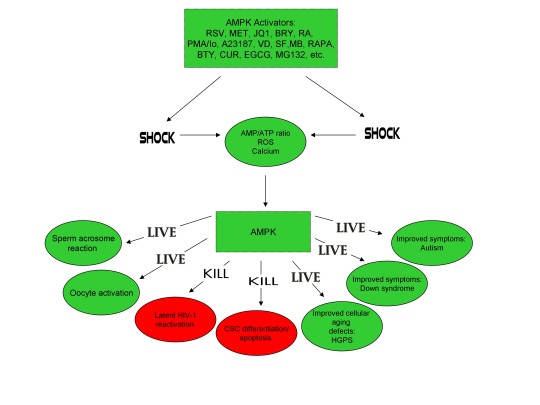
References:
Murugesu S, Saso S, Jones BP, et al. Does the use of calcium ionophore during artificial oocyte activation demonstrate an effect on pregnancy rate? A meta-analysis. Fertil Steril. 2017 Sep;108(3):468-482.e3.
Tesarik J, Sousa M, Testart J. Human oocyte activation after intracytoplasmic sperm injection. Hum Reprod. 1994 Mar;9(3):511-8.
Deemeh MR, Tavalaee M, Nasr-Esfahani MH. Health of children born through artificial oocyte activation: a pilot study. Reprod Sci. 2015 Mar;22(3):322-8.
Eftekhar M, Janati S, Rahsepar M, Aflatoonian A. Effect of oocyte activation with calcium ionophore on ICSI outcomes in teratospermia: A randomized clinical trial. Iran J Reprod Med. 2013 Nov;11(11):875-82.
Liu WC, Slusarchyk DS, Astle G, Trejo WH, Brown WE, Meyers E. Ionomycin, a new polyether antibiotic. J Antibiot (Tokyo). 1978 Sep;31(9):815-9.
Reed PW, Lardy HA. A23187: a divalent cation ionophore. J Biol Chem. 1972 Nov 10;247(21):6970-7.
Zhang Z, Wang T, Hao Y, et al. Effects of trehalose vitrification and artificial oocyte activation on the development competence of human immature oocytes. Cryobiology. 2017 Feb;74:43-49.
De Sutter P, Dozortsev D, Cieslak J, Wolf G, Verlinsky Y, Dyban A. Parthenogenetic activation of human oocytes by puromycin. J Assist Reprod Genet. 1992 Aug;9(4):328-37.
Sankaran L, Pogell BM. Biosynthesis of puromycin in Streptomyces alboniger: regulation and properties of O-demethylpuromycin O-methyltransferase. Antimicrob Agents Chemother. 1975 Dec;8(6):721-32.
Tesarik J, Rienzi L, Ubaldi F, Mendoza C, Greco E. Use of a modified intracytoplasmic sperm injection technique to overcome sperm-borne and oocyte-borne oocyte activation failures. Fertil Steril. 2002 Sep;78(3):619-24.
Mansour R, Fahmy I, Tawab NA, et al. Electrical activation of oocytes after intracytoplasmic sperm injection: a controlled randomized study. Fertil Steril. 2009 Jan;91(1):133-9.
Nikiforaki D, Vanden Meerschaut F, de Roo C, et al. Effect of two assisted oocyte activation protocols used to overcome fertilization failure on the activation potential and calcium releasing pattern. Fertil Steril. 2016 Mar;105(3):798-806.e2.
Liu H1, Zhang J, Krey LC, Grifo JA. In-vitro development of mouse zygotes following reconstruction by sequential transfer of germinal vesicles and haploid pronuclei. Hum Reprod. 2000 Sep;15(9):1997-2002.
Downs SM. Stimulation of parthenogenesis in mouse ovarian follicles by inhibitors of inosine monophosphate dehydrogenase. Biol Reprod. 1990 Sep;43(3):427-36.
Siracusa G, Whittingham DG, Molinaro M, Vivarelli E. Parthenogenetic activation of mouse oocytes induced by inhibitors of protein synthesis. J Embryol Exp Morphol. 1978 Feb;43:157-66.
Carvacho I, Lee HC, Fissore RA, Clapham DE. TRPV3 channels mediate strontium-induced mouse-egg activation. Cell Rep. 2013 Dec 12;5(5):1375-86.
Colonna R, Tatone C, Malgaroli A, Eusebi F, Mangia F. Effects of protein kinase C stimulation and free Ca2+ rise in mammalian egg activation. Gamete Res. 1989 Oct;24(2):171-83.
Tang Z, Xing F, Chen D, et al. In vivo toxicological evaluation of Anisomycin. Toxicol Lett. 2012 Jan 5;208(1):1-11.
Regueira TB, Kildegaard KR, Hansen BG, Mortensen UH, Hertweck C, Nielsen J. Molecular basis for mycophenolic acid biosynthesis in Penicillium brevicompactum. Appl Environ Microbiol. 2011 May;77(9):3035-43.
K'ominek LA. Cycloheximide production by Streptomyces griseus: control mechanisms of cycloheximide biosynthesis. Antimicrob Agents Chemother. 1975 Jun;7(6):856-6.
Ultee A, Slump RA, Steging G, Smid EJ. Antimicrobial activity of carvacrol toward Bacillus cereus on rice. J Food Prot. 2000 May;63(5):620-4.
Pal PK, Nandi MK, Singh NK. Detoxification of Croton tiglium L. seeds by Ayurvedic process of Śodhana. Anc Sci Life. 2014 Jan;33(3):157-61.
Rotem R, Paz GF, Homonnai ZT, et al. Ca(2+)-independent induction of acrosome reaction by protein kinase C in human sperm. Endocrinology. 1992 Nov;131(5):2235-43.
de Lamirande E, Tsai C, Harakat A, Gagnon C. Involvement of reactive oxygen species in human sperm arcosome reaction induced by A23187, lysophosphatidylcholine, and biological fluid ultrafiltrates. J Androl. 1998 Sep-Oct;19(5):585-94.
Görlach A, Bertram K, Hudecova S, Krizanova O. Calcium and ROS: A mutual interplay. Redox Biol. 2015 Dec;6:260-71.
Nasr-Esfahani MM, Johnson MH. The origin of reactive oxygen species in mouse embryos cultured in vitro. Development. 1991 Oct;113(2):551-60.
LaRosa C, Downs SM. Stress stimulates AMP-activated protein kinase and meiotic resumption in mouse oocytes. Biol Reprod. 2006 Mar;74(3):585-92.
Calle-Guisado V, de Llera AH, Martin-Hidalgo D, et al. AMP-activated kinase in human spermatozoa: identification, intracellular localization, and key function in the regulation of sperm motility. Asian J Androl. 2016 Sep 27.
Mungai PT, Waypa GB, Jairaman A, et al. Hypoxia triggers AMPK activation through reactive oxygen species-mediated activation of calcium release-activated calcium channels. Mol Cell Biol. 2011 Sep;31(17):3531-45.
Tamas P, Hawley SA, Clarke RG, et al. Regulation of the energy sensor AMP-activated protein kinase by antigen receptor and Ca2+ in T lymphocytes. J Exp Med 2006;203(7):1665–70.
Hawley SA, Pan DA, Mustard KJ, et al. Calmodulin-dependent protein kinase kinase-beta is an alternative upstream kinase for AMP-activated protein kinase. Cell Metab. 2005 Jul;2(1):9-19.
Nammi S, Roufogalis BD. Light-to-moderate ethanol feeding augments AMPK-α phosphorylation and attenuates SREBP-1 expression in the liver of rats. J Pharm Pharm Sci. 2013;16(2):342-51.
Koh W, Jeong SJ, Lee HJ, et al. Melatonin promotes puromycin-induced apoptosis with activation of caspase-3 and 5’-adenosine monophosphate-activated kinase-alpha in human leukemia HL-60 cells. J Pineal Res. 2011 May;50(4):367-73.
Bays JL, Campbell HK, Heidema C, Sebbagh M, DeMali KA. Linking E-cadherin mechanotransduction to cell metabolism through force-mediated activation of AMPK. Nat Cell Biol. 2017 Jun;19(6):724-731.
Hutber CA, Hardie DG, Winder WW. Electrical stimulation inactivates muscle acetyl-CoA carboxylase and increases AMP-activated protein kinase. Am J Physiol. 1997 Feb;272(2 Pt 1):E262-6.
Ohsaka Y, Nishino H, Nomura Y. Induction of phospho-Thr-172 AMPK in 3T3-L1 adipocytes exposed to cold or treated with anisomycin, mithramycin A, and ionic compounds. Cryo Letters. 2010 May-Jun;31(3):218-29.
Fernández-Ramos AA, Marchetti-Laurent C, Poindessous V, et al. A comprehensive characterization of the impact of mycophenolic acid on the metabolism of Jurkat T cells. Sci Rep. 2017 Sep 5;7(1):10550.
Kim E, Choi Y, Jang J, Park T. Carvacrol Protects against Hepatic Steatosis in Mice Fed a High-Fat Diet by Enhancing SIRT1-AMPK Signaling. Evid Based Complement Alternat Med. 2013;2013:290104.
Zogovic N, Tovilovic-Kovacevic G, Misirkic-Marjanovic M, et al. Coordinated activation of AMP-activated protein kinase, extracellular signal-regulated kinase, and autophagy regulates phorbol myristate acetate-induced differentiation of SH-SY5Y neuroblastoma cells. J Neurochem. 2015 Apr;133(2):223-32.
Finley J. Oocyte activation and latent HIV-1 reactivation: AMPK as a common mechanism of action linking the beginnings of life and the potential eradication of HIV-1. Med Hypotheses. 2016 Aug;93:34-47.
Kitani S1, Miyamoto KT, Takamatsu S, et al. Avenolide, a Streptomyces hormone controlling antibiotic production in Streptomyces avermitilis. Proc Natl Acad Sci U S A. 2011 Sep 27;108(39):16410-5.
Challis GL, Hopwood DA. Synergy and contingency as driving forces for the evolution of multiple secondary metabolite production by Streptomyces species. Proc Natl Acad Sci U S A. 2003 Nov 25;100 Suppl 2:14555-61.
Yagüe P, López-García MT, Rioseras B, Sánchez J, Manteca A. Pre-sporulation stages of Streptomyces differentiation: state-of-the-art and future perspectives. FEMS Microbiol Lett. 2013 May;342(2):79-88.
Seipke RF, Kaltenpoth M, Hutchings MI. Streptomyces as symbionts: an emerging and widespread theme? FEMS Microbiol Rev. 2012 Jul;36(4):862-76.
Meng L, Li M, Yang SH, Kim TJ, Suh JW. Intracellular ATP levels affect secondary metabolite production in Streptomyces spp. Biosci Biotechnol Biochem. 2011;75(8):1576-81.
Sánchez S, Chávez A, Forero A, et al. Carbon source regulation of antibiotic production. J Antibiot (Tokyo). 2010 Aug;63(8):442-59.
Wang SL, Fan KQ, Yang X, Lin ZX, Xu XP, Yang KQ. CabC, an EF-hand calcium-binding protein, is involved in Ca2+-mediated regulation of spore germination and aerial hypha formation in Streptomyces coelicolor. J Bacteriol. 2008 Jun;190(11):4061-8.
Wang D, Wei L, Zhang Y, Zhang M, Gu S. Physicochemical and microbial responses of Streptomyces natalensis HW-2 to fungal elicitor. Appl Microbiol Biotechnol. 2017 Jul 28. doi: 10.1007/s00253-017-8440-0. [Epub ahead of print].
Wei ZH, Bai L, Deng Z, Zhong JJ. Enhanced production of validamycin A by H2O2-induced reactive oxygen species in fermentation of Streptomyces hygroscopicus 5008. Bioresour Technol. 2011 Jan;102(2):1783-7.
Doull JL, Ayer SW, Singh AK, Thibault P. Production of a novel polyketide antibiotic, jadomycin B, by Streptomyces venezuelae following heat shock. J Antibiot (Tokyo). 1993 May;46(5):869-71.
Zhou WW1, Ma B, Tang YJ, Zhong JJ, Zheng X. Enhancement of validamycin A production by addition of ethanol in fermentation of Streptomyces hygroscopicus 5008. Bioresour Technol. 2012 Jun;114:616-21.
Flanary BE, Kletetschka G. Analysis of telomere length and telomerase activity in tree species of various lifespans, and with age in the bristlecone pine Pinus longaeva. Rejuvenation Res. 2006 Spring;9(1):61-3.
R. S. BeasleyJ. O. Klemmedson. Recognizing site adversity and drought-sensitive trees in stands of bristlecone pine (Pinus longaeva). January 1973, Volume 27, Issue 1, pp 141–146. doi: 10.1007/BF02862228.
Schulman E. Longevity under Adversity in Conifers. Science. 1954 Mar 26;119(3091):396-9.
Hiebert, R. D.; Hamrick, J. L. 1984. An ecological study of bristlecone pine (Pinus longaeva) in Utah and eastern Nevada. The Great Basin Naturalist. 44(3): 487-494.
Jorquera MA, Shaharoona B, Nadeem SM, de la Luz Mora M, Crowley DE. Plant growth-promoting rhizobacteria associated with ancient clones of creosote bush (Larrea tridentata). Microb Ecol. 2012 Nov;64(4):1008-17.
Salminen A, Kaarniranta K. AMP-activated protein kinase (AMPK) controls the aging process via an integrated signaling network. Ageing Res Rev. 2012 Apr;11(2):230-41.
Leymarie J, Vitkauskaité G, Hoang HH, et al. Role of reactive oxygen species in the regulation of Arabidopsis seed dormancy. Plant Cell Physiol. 2012 Jan;53(1):96-106.
Pang X, Halaly T, Crane O, et al. Involvement of calcium signalling in dormancy release of grape buds. J Exp Bot. 2007;58(12):3249-62.
Duan Q, Kita D, Johnson EA, et al. Reactive oxygen species mediate pollen tube rupture to release sperm for fertilization in Arabidopsis. Nat Commun. 2014;5:3129.
Gao XQ, Liu CZ, Li DD, et al. The Arabidopsis KINβγ Subunit of the SnRK1 Complex Regulates Pollen Hydration on the Stigma by Mediating the Level of Reactive Oxygen Species in Pollen. PLoS Genet. 2016 Jul 29;12(7):e1006228.
Joo JH, Bae YS, Lee JS. Role of auxin-induced reactive oxygen species in root gravitropism. Plant Physiol. 2001 Jul;126(3):1055-60.
Lee JS, Mulkey TJ, Evans ML. Reversible loss of gravitropic sensitivity in maize roots after tip application of calcium chelators. Science. 1983 Jun 24;220(4604):1375-6.
Zhao J, Davis LC, Verpoorte R. Elicitor signal transduction leading to production of plant secondary metabolites. Biotechnol Adv. 2005 Jun;23(4):283-333.
Wang H, Sharma L, Lu J, Finch P, Fletcher S, Prochownik EV. Structurally diverse c-Myc inhibitors share a common mechanism of action involving ATP depletion. Oncotarget. 2015 Jun 30;6(18):15857-70.
K.V. Kiselev, O.A. Shumakova, A.Y. Manyakhin, A.N. Mazeika. Influence of calcium influx induced by the calcium ionophore, A23187, on resveratrol content and the expression of CDPK and STS genes in the cell cultures of Vitis amurensis. Plant Growth Regulation. December 2012, Volume 68, Issue 3, pp 371–381. DOI: 10.1007/s10725-012-9725-z.
Darwin, Charles (1859). On the origin of species by means of natural selection, or the preservation of favoured races in the struggle for life. London: Murray. [1st ed.].
Sobral M, Veiga T, Domínguez P, Guitián JA, Guitián P, Guitián JM. Selective Pressures Explain Differences in Flower Color among Gentiana lutea Populations. PLoS One. 2015 Jul 14;10(7):e0132522.
Lefebvre V, Kiani SP, Durand-Tardif M. A focus on natural variation for abiotic constraints response in the model species Arabidopsis thaliana. Int J Mol Sci. 2009 Aug 13;10(8):3547-82.
Moser TS, Schieffer D, Cherry S. AMP-activated kinase restricts Rift Valley fever virus infection by inhibiting fatty acid synthesis. PLoS Pathog. 2012;8(4):e1002661.
Goto A, Egawa T, Sakon I, et al. Heat stress acutely activates insulin-independent glucose transport and 5’-AMP-activated protein kinase prior to an increase in HSP72 protein in rat skeletal muscle. Physiol Rep. 2015 Nov;3(11). pii: e12601.
Maclean RC, Hall AR, Perron GG, Buckling A. The evolution of antibiotic resistance: insight into the roles of molecular mechanisms of resistance and treatment context. Discov Med. 2010 Aug;10(51):112-8.
Dwyer DJ, Belenky PA, Yang JH, et al. Antibiotics induce redox-related physiological alterations as part of their lethality. Proc Natl Acad Sci U S A. 2014 May 20;111(20):E2100-9.
Kohanski MA, DePristo MA, Collins JJ. Sublethal antibiotic treatment leads to multidrug resistance via radical-induced mutagenesis. Mol Cell. 2010 Feb 12;37(3):311-20.
Poole K. Bacterial stress responses as determinants of antimicrobial resistance. J Antimicrob Chemother. 2012 Sep;67(9):2069-89.
Ito M, Arakawa T, Okayama M, Shitara A, Mizoguchi I, Takuma T. Gravity loading induces adenosine triphosphate release and phosphorylation of extracellular signal-regulated kinases in human periodontal ligament cells. J Investig Clin Dent. 2014 Nov;5(4):266-74.
Bays JL, Campbell HK, Heidema C, Sebbagh M, DeMali KA. Linking E-cadherin mechanotransduction to cell metabolism through force-mediated activation of AMPK. Nat Cell Biol. 2017 Jun;19(6):724-731.
Zhong G, Li Y, Li H, et al. Simulated Microgravity and Recovery-Induced Remodeling of the Left and Right Ventricle. Front Physiol. 2016 Jun 29;7:274.
Chang TT, Walther I, Li CF, et al. The Rel/NF-jB pathway and transcription of immediate early genes in T cell activation are inhibited by microgravity. J Leukoc Biol 2012;92(6):1133–45.
Li YC, Chen BM, Wu PC, et al. Cutting Edge: mechanical forces acting on T cells immobilized via the TCR complex can trigger TCR signaling. J Immunol 2010;184(11):5959–63.
Ouyang Z, Wang X, Meng Q, et al. Suppression of adenosine monophosphate-activated protein kinase selectively triggers apoptosis in activated T cells and ameliorates immune diseases. Biochem Biophys Res Commun. 2017 May 27;487(2):223-229.
Blaber EA, Pecaut MJ, Jonscher KR. Spaceflight Activates Autophagy Programs and the Proteasome in Mouse Liver. Int J Mol Sci. 2017 Sep 27;18(10). pii: E2062.
Mo C, Wang L, Zhang J, et al. The crosstalk between Nrf2 and AMPK signal pathways is important for the anti-inflammatory effect of berberine in LPS-stimulated macrophages and endotoxin-shocked mice. Antioxid Redox Signal. 2014 Feb 1;20(4):574-88.
Joo MS, Kim WD, Lee KY, Kim JH, Koo JH, Kim SG. AMPK facilitates nuclear accumulation of Nrf2 by phosphorylating at serine 550. Mol Cell Biol. 2016 Jun 29;36(14):1931-42.
Zhang L, Yi Y, Guo Q, et al. Hsp90 interacts with AMPK and mediates acetyl-CoA carboxylase phosphorylation. Cell Signal. 2012 Apr;24(4):859-65.
Sagare-Patil V, Bhilawadikar R, Galvankar M, Zaveri K, Hinduja I, Modi D. Progesterone requires heat shock protein 90 (HSP90) in human sperm to regulate motility and acrosome reaction. J Assist Reprod Genet. 2017 Apr;34(4):495-503.
Lesniewski LA, Seals DR4, Walker AE, et al. Dietary rapamycin supplementation reverses age-related vascular dysfunction and oxidative stress, while modulating nutrient-sensing, cell cycle, and senescence pathways. Aging Cell. 2017 Feb;16(1):17-26.
Aparicio IM, Espino J, Bejarano I, et al. Autophagy-related proteins are functionally active in human spermatozoa and may be involved in the regulation of cell survival and motility. Sci Rep. 2016 Sep 16;6:33647.
Fang A, Pierson DL, Mishra SK, Demain AL. Growth of Steptomyces hygroscopicus in rotating-wall bioreactor under simulated microgravity inhibits rapamycin production. Appl Microbiol Biotechnol. 2000 Jul;54(1):33-6.
Cheng YR, Huang J, Qiang H, Lin WL, Demain AL. Mutagenesis of the rapamycin producer Streptomyces hygroscopicus FC904. J Antibiot (Tokyo). 2001 Nov;54(11):967-72.
Heindryckx B, Lierman S, Combelles CM, Cuvelier CA, Gerris J, De Sutter P. Aberrant spindle structures responsible for recurrent human metaphase I oocyte arrest with attempts to induce meiosis artificially. Hum Reprod. 2011 Apr;26(4):791-800.
Shkolnik K, Tadmor A, Ben-Dor S, Nevo N, Galiani D, Dekel N. Reactive oxygen species are indispensable in ovulation. Proc Natl Acad Sci U S A. 2011 Jan 25;108(4):1462-7.
Amdani SN, Jones C, Coward K. Phospholipase C zeta (PLC ζ): oocyte activation and clinical links to male factor infertility. Adv Biol Regul 2013;53(3):292–308.
Smith-Garvin JE, Koretzky GA, Jordan MS. T cell activation. Annu Rev Immunol 2009;27:591–619.
Spina CA, Anderson J, Archin NM, et al. An in-depth comparison of latent HIV-1 reactivation in multiple cell model systems and resting CD4+ T cells from aviremic patients. PLoS Pathog 2013;9(12):e1003834.
Marsden MD, Zack JA. HIV/AIDS eradication. Bioorg Med Chem Lett 2013;23(14):4003–10.
Sena LA, Li S, Jairaman A, et al. Mitochondria are required for antigen-specific T cell activation through reactive oxygen species signaling. Immunity. 2013 Feb 21;38(2):225-36.
Barquero AA, Dávola ME, Riva DA, Mersich SE, Alché LE. Naturally occurring compounds elicit HIV-1 replication in chronically infected promonocytic cells. Biomed Res Int. 2014;2014:989101.
Imai K, Ochiai K, Okamoto T. Reactivation of latent HIV-1 infection by the periodontopathic bacterium Porphyromonas gingivalis involves histone modification. J Immunol. 2009 Mar 15;182(6):3688-95.
Piette J, Legrand-Poels S. HIV-1 reactivation after an oxidative stress mediated by different reactive oxygen species. Chem Biol Interact. 1994 Jun;91(2-3):79-89.
Anderson I, Low JS, Weston S, et al. Heat shock protein 90 controls HIV-1 reactivation from latency. Proc Natl Acad Sci U S A. 2014 Apr 15;111(15):E1528-37.
Zhou H, Xu M, Huang Q, et al. Genome-scale RNAi screen for host factors required for HIV replication. Cell Host Microbe. 2008 Nov 13;4(5):495-504.
Wu H, Esteve E, Tremaroli V, et al. Metformin alters the gut microbiome of individuals with treatment-naive type 2 diabetes, contributing to the therapeutic effects of the drug. Nat Med. 2017 Jul;23(7):850-858.
G.M. Chew, D.C. Chow, S.A. Souza, et al. Impact of adjunctive metformin therapy on T cell exhaustion and viral persistence in a clinical trial of HIV-infected adults on suppressive ART. Journal of Virus Eradication 2017; 3 (Supplement 1): 6–19.
http://viruseradication.com/supplement-details/Abstracts_of_the_IAS_HIV_Cure_and_Cancer_Forum_2017/
Ullrich NJ, Gordon LB. Hutchinson-Gilford progeria syndrome. Handb Clin Neurol. 2015;132:249-64.
Berro R, Kehn K, de la Fuente C, et al. Acetylated Tat regulates human immunodeficiency virus type 1 splicing through its interaction with the splicing regulator p32. J Virol 2006;80(7):3189–204.
Paz S, Lu ML, Takata H, Trautmann L, Caputi M. SRSF1 RNA Recognition Motifs Are Strong Inhibitors of HIV-1 Replication. J Virol. 2015 Jun;89(12):6275-86.
Lopez-Mejia IC, Vautrot V, De Toledo M, et al. A conserved splicing mechanism of the LMNA gene controls premature aging. Hum Mol Genet. 2011 Dec 1;20(23):4540-55.
Park SK, Shin OS. Metformin alleviates ageing cellular phenotypes in Hutchinson-Gilford progeria syndrome dermal fibroblasts. Exp Dermatol. 2017 Oct;26(10):889-895.
Egesipe AL, Blondel S, Cicero AL, et al. Metformin decreases progerin expression and alleviates pathological defects of Hutchinson-Gilford progeria syndrome cells. NPJ Aging Mech Dis. 2016 Nov 10;2:16026.
Finley J. Alteration of splice site selection in the LMNA gene and inhibition of progerin production via AMPK activation. Med Hypotheses. 2014 Nov;83(5):580-7.
Decuypere JP, Welkenhuyzen K, Luyten T, et al. Ins(1,4,5)P3 receptor-mediated Ca2+ signaling and autophagy induction are interrelated. Autophagy. 2011 Dec;7(12):1472-89.
Cao Y, Fang Y, Cai J, et al. ROS functions as an upstream trigger for autophagy to drive hematopoietic stem cell differentiation. Hematology. 2016 Dec;21(10):613-618.
Decuypere JP, Kindt D, Luyten T, et al. mTOR-Controlled Autophagy Requires Intracellular Ca(2+) Signaling. PLoS One. 2013;8(4):e61020.
Cao K, Graziotto JJ, Blair CD, et al. Rapamycin reverses cellular phenotypes and enhances mutant protein clearance in Hutchinson-Gilford progeria syndrome cells. Sci Transl Med. 2011 Jun 29;3(89):89ra58.
Gabriel D, Gordon LB, Djabali K. Temsirolimus Partially Rescues the Hutchinson-Gilford Progeria Cellular Phenotype. PLoS One. 2016 Dec 29;11(12):e0168988.
Moncharmont C, Levy A, Gilormini M, et al. Targeting a cornerstone of radiation resistance: cancer stem cell. Cancer Lett 2012;322(2):139–47.
Finley J. Elimination of cancer stem cells and reactivation of latent HIV-1 via AMPK activation: Common mechanism of action linking inhibition of tumorigenesis and the potential eradication of HIV-1. Med Hypotheses. 2017 Jul;104:133-146.
Buzon MJ, Sun H, Li C, et al. HIV-1 persistence in CD4+ T cells with stem cell-like properties. Nat Med 2014;20(2):139–42.
Wee S, Niklasson M, Marinescu VD, et al. Selective calcium sensitivity in immature glioma cancer stem cells. PLoS One. 2014 Dec 22;9(12):e115698.
Hayun M, Okun E, Hayun R, Gafter U, et al. Synergistic effect of AS101 and Bryostatin-1 on myeloid leukemia cell differentiation in vitro and in an animal model. Leukemia 2007;21(7):1504–13.
Sato A, Sunayama J, Okada M, et al. Glioma-initiating cell elimination by metformin activation of FOXO3 via AMPK. Stem Cells Transl Med 2012;1(11):811–24.
Fasih A, Elbaz HA, Hüttemann M, Konski AA, Zielske SP. Radiosensitization of pancreatic cancer cells by metformin through the AMPK pathway. Radiat Res 2014;182(1):50–9
#ampk#progeria#cancer stem cell#HIV/AIDS#oocyte#sperm#acrosome reaction#fertilization#metformin#gut bacteria#evolution#reproduction#charles darwin
0 notes