Don't wanna be here? Send us removal request.
Text
Stress links the Creation of Gravity, the Universe, and all Human Life
Stress links the Creation of Gravity, the Universe, and all Human Life


Try Premium Free for 1 Month

Stress links the Creation of Gravity, the Universe, and all Human Life
Published on October 13, 2019
Edit article
View stats
Status is online
View On WordPress
0 notes
Text
Metformin target AMPK shown for the first time to be required for Long-Term Memory Formation: Hypothesis Substantiated

By Davidboyashi - Own work, CC BY-SA 4.0, https://commons.wikimedia.org/w/index.php?curid=48165329
A recently published study in the journal iScience in October of 2018 demonstrated for the first time that metabolic plasticity induced by AMPK activation is required for long-term potentiation (LTP) in the CA1 region of the hippocampus in vitro in mouse neurons as well as long-term memory formation in vivo in mice [1]. These results provide direct support and substantiate my previous hypothesis in which I first proposed that activation of AMPK will promote LTP specifically in the CA1 region of the hippocampus and promote memory formation in vivo [2]. AMPK activation is considered a primary mechanism through which the anti-diabetic drug metformin and numerous naturally-occurring compounds exert their therapeutic effects [3]. Learning and memory are generally considered the behavioral correlates of long-term potentiation (LTP), a form of synaptic plasticity associated with a persistent and long-lasting increase in synaptic strength in response to repetitive neuronal stimulation [2]. It is the Schaffer collateral-CA1 excitatory synapse (i.e. CA1 synapses) that has generated increased interest in recent years due to accumulating evidence that high frequency stimulation of CA1 synapses leads to a long–lasting increase in synaptic strength that underlies learning and memory [2]. In the iScience study, Marinangeli et al. initially demonstrated that synaptic activation of primary mouse neurons with a combination of bicuculline and 4-aminopyridine rapidly activated AMPK. AMPK activation was dependent on glutamate receptor activation, as the NMDA and AMPA receptor inhibitors MK-801 and NBQX, respectively, significantly reduced AMPK activation [1].
Inhibition of AMPK by compound C or a kinase-dead dominant-negative AMPK construct also significantly decreased ATP levels and the upregulation of glycolysis and mitochondrial respiration, indicating that AMPK is critical for maintaining neuronal energy levels in response to synaptic activation [1]. Interestingly, AMPK inhibition also significantly reduced the expression of the immediate-early genes Arc, cFos, and Egrl (important for learning and memory), indicating that AMPK activation is required for the expression of these genes following synaptic activation. Importantly, inhibition of AMPK by compound C severely impaired LTP in the CA1 region of the hippocampus induced by electrical theta burst stimulation [1]. The authors also determined if AMPK activation is necessary for long-term memory retention in vivo in mice. Bilateral injection of compound C in the hippocampus before inhibitory avoidance training significantly blocked long-term memory tested at 24 hours which persisted after retesting at 6 days, providing compelling evidence that AMPK activation is critical for CA1 LTP in vitro and long-term memory formation in vivo [1].
As noted above, I first proposed in April of 2018 that AMPK activation would promote LTP specifically in area CA1 of the hippocampus and enhance learning and memory in vivo [2]. Indeed, the authors of the iScience study performed precise experiments that verified and substantiated my hypothesis, as follows: “Knockdown or pharmacological inhibition of both AMPK catalytic subunits (AMPKα1 and AMPKα2) in hippocampal neurons (e.g. hippocampal CA1 pyramidal neurons) would be conducted to determine if AMPK activation is essential for the induction, expression, and/or maintenance of LTP in vitro or the facilitation of learning and memory in vivo.” [2]. I also proposed in this paper that cellular stress-induced AMPK activation links CA1 LTP with the reactivation of latent HIV-1, facilitating immune system detection and potential destruction of the virus [2]. Intriguingly, the iScience study showed that AMPK increased the expression of the immediate-early gene Egr1 whereas the AMPK activator resveratrol reactivates latent HIV-1 via upregulation of Egr-1 [3,4]. Egr-1 was shown to be downregulated during viral latency in HIV-1 infected ACH-2 cells and treatment with resveratrol caused viral reactivation as indicated by a dose-dependent increase in viral p24 expression, suggesting that AMPK activation may indeed facilitate reactivation and destruction of the virus [2,4].
The iScience study also showed that AMPK activation increased the expression of the immediate-early gene Arc. Arc plays a critical role in memory formation and has recently been shown to be derived from a transposable element, DNA sequences first described by Nobel laureate Barbara McClintock that comprise nearly half of the human genome and are able to transpose or move from one genomic location to another [5,6]. I also recently proposed for the first time that AMPK activation would promote beneficial activation and transposition of transposable elements (also known as “jumping genes”) located in the human brain, human sperm, and in human oocytes [6]. Indeed, the transposable element L1 is present in the hippocampus of the human brain and contributes to memory formation in vivo in mice [7,8]. Additionally, metformin promotes AMPK-dependent telomerase activation (critical for telomere maintenance) and induces activation of the endonuclease RAG1 (promotes DNA cleavage and transposition) via AMPK [9,10]. Similar to Arc, both RAG1 and telomerase are derived from transposable elements, providing further evidence that AMPK links learning and memory with potential HIV-1 eradication and transposable element activation and mobilization [2,6,11].
https://www.linkedin.com/pulse/metformin-target-ampk-shown-first-time-required-long-term-finley/
References:
Marinangeli C, Didier S, Ahmed T, et al. AMP-Activated Protein Kinase Is Essential for the Maintenance of Energy Levels during Synaptic Activation. iScience. 2018 Oct 12;9:1-13. doi: 10.1016/j.isci.2018.10.006. [Epub ahead of print].
Finley J. Facilitation of hippocampal long-term potentiation and reactivation of latent HIV-1 via AMPK activation: Common mechanism of action linking learning, memory, and the potential eradication of HIV-1. Med Hypotheses. 2018 Jul;116:61-73.
Hardie DG. AMPK: a target for drugs and natural products with effects on both diabetes and cancer. Diabetes 2013;62(7):2164–72.
Krishnan V, Zeichner SL. Host cell gene expression during human immunodeficiency virus type 1 latency and reactivation and effects of targeting genes that are differentially expressed in viral latency. J Virol 2004;78(17):9458–73.
Pastuzyn ED, Day CE, Kearns RB, et al. The Neuronal Gene Arc Encodes a Repurposed Retrotransposon Gag Protein that Mediates Intercellular RNA Transfer. Cell. 2018 Jan 11;172(1-2):275-288.e18.
Finley J. Transposable elements, placental development, and oocyte activation: Cellular stress and AMPK links jumping genes with the creation of human life. Med Hypotheses. 2018 Sep;118:44-54.
Coufal NG, Garcia-Perez JL, Peng GE, et al. L1 retrotransposition in human neural progenitor cells. Nature 2009;460(7259):1127–31.
Bachiller S, Del-Pozo-Martín Y, Carrión ÁM. L1 retrotransposition alters the hippocampal genomic landscape enabling memory formation. Brain Behav Immun 2017;64:65–70.
Karnewar S, Neeli PK, Panuganti D, et al. Metformin regulates mitochondrial biogenesis and senescence through AMPK mediated H3K79 methylation: relevance in age-associated vascular dysfunction. Biochim Biophys Acta 2018;1864(4 Pt A):1115–28.
Um JH, Brown AL, Singh SK, et al. Metabolic sensor AMPK directly phosphorylates RAG1 protein and regulates V(D)J recombination. Proc Natl Acad Sci USA 2013;110(24):9873–8.
Lander ES, Linton LM, Birren B, et al. Initial sequencing and analysis of the human genome. Nature 2001;409(6822):860–921.
0 notes
Text
New Harvard Study shows for the first time that meditation upregulates genes in the AMPK pathway similar to metformin
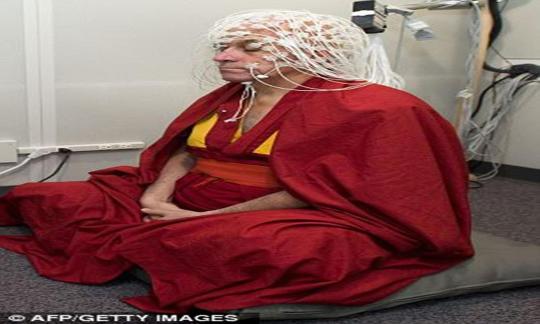
A new study published in 2018 by researchers at Harvard Medical School showed for the first time that a collection of meditative techniques that led to the relaxation response (RR) in human patients significantly upregulated genes in the AMPK signaling pathway [1]. The RR elicitation routine included diaphragmatic breathing, body scan, mantra repetition, mindfulness meditation, and passively ignoring intrusive thoughts [1]. An upregulation of genes in the AMPK signaling pathway was detected via blood samples taken from patients in which total RNA was isolated from peripheral blood mononuclear cells (PBMCs) [1].
This study has an interesting connection to a recently published study in 2018 in which a retreat that included meditation led to an increase in the RNA-binding protein HnRNPA1 [2]. I first hypothesized and proposed that metformin and AMPK activation would beneficially modulate the activity of HnRNPA1 [3,4] . Indeed, the well-studied AMPK activator resveratrol has recently been shown to upregulate HnRNPA1 [5]. Additionally, HnRNPA1 binds to telomerase and plays a critical role in telomere maintenance, promotes latent HIV-1 reactivation (facilitating immune system detection and virus destruction), and is necessary for the transposition or “jumping” of “jumping genes” in human cells [6-9]. Metformin has also been shown to activate human telomerase (hTERT) in an AMPK-dependent manner and reduce cellular makers associated with latent HIV-1 in infected patients [10-12]. This evidence lends substantial support to several hypotheses that I originally proposed linking metformin and AMPK activation with telomerase activation, “jumping genes” in the brain (important for learning and memory), virus destruction, aging deceleration, human life creation, and even consciousness itself [3,4,13-17]. Strikingly, AMPK activation may also play a critical role in the beneficial effects of meditation on the human brain.
Meditation has been shown to increase brain gamma waves, brain gray matter density, and beneficial transcriptome changes in energy metabolism [18-20]. Interestingly, the Dalai Lama has also described meditation as “hard work”, indicating that meditation is an active process that challenges or slightly stresses the brain, leading to upregulation of genes in the AMPK signaling pathway as shown in the Harvard study [1]. This “mental stressor or challenge” is very much similar to challenging or stressing the human body with exercise, which is well-known to induce AMPK activation, leading to several beneficial effects [21]. The “mental challenge of meditation” is also analogous to the stress or challenge placed on the brain of animals when exposed to a stimulating environment, leading to an enhancement of learning and memory (i.e. long-term potentiation) and the “jumping of genes” [15,16]. Additionally, methodologies and neurotransmitters that are critical for inducing long-term potentiation (e.g. glutamate, high-frequency stimulation) activate AMPK in neurons and nearly every anesthetic used clinically to induce and maintain general anesthesia, including propofol, activates AMPK and excites the brain in low doses (called paradoxical excitation) [15,16]. Nearly every neurotransmitter that plays a critical role in wakefulness, arousal, and cognition also activates AMPK, supporting my original hypothesis that AMPK activation likely plays a central role in promoting consciousness itself [16].
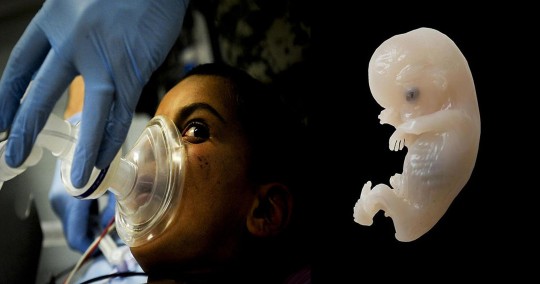
Source: By ISAF Headquarters Public Affairs Office (originally posted to Flickr as 100410-F-7713A-002) [CC BY 2.0 (https://creativecommons.org/licenses/by/2.0)], via Wikimedia Commons; By Anatomist90 [CC BY-SA 3.0 (https://creativecommons.org/licenses/by-sa/3.0) or GFDL (http://www.gnu.org/copyleft/fdl.html)], from Wikimedia Commons
References:
Bhasin MK, Denninger JW, Huffman JC, et al. Specific Transcriptome Changes Associated with Blood Pressure Reduction in Hypertensive Patients After Relaxation Response Training. J Altern Complement Med. 2018 May;24(5):486-504.
Conklin QA, King BG, Zanesco AP, et al. Insight meditation and telomere biology: The effects of intensive retreat and the moderating role of personality. Brain Behav Immun. 2018 May;70:233-245.
Finley J. Alteration of splice site selection in the LMNA gene and inhibition of progerin production via AMPK activation. Med Hypotheses. 2014 Nov;83(5):580-7.
Finley J. Reactivation of latently infected HIV-1 viral reservoirs and correction of aberrant alternative splicing in the LMNA gene via AMPK activation: Common mechanism of action linking HIV-1 latency and Hutchinson-Gilford progeria syndrome. Med Hypotheses. 2015 Sep;85(3):320-32.
Moshiri A, Puppo M, Rossi M, Gherzi R, Briata P. Resveratrol limits epithelial to mesenchymal transition through modulation of KHSRP/hnRNPA1-dependent alternative splicing in mammary gland cells. Biochim Biophys Acta. 2017 Mar;1860(3):291-298.
Ford LP, Wright WE, Shay JW. A model for heterogeneous nuclear ribonucleoproteins in telomere and telomerase regulation. Oncogene. 2002 Jan 21;21(4):580-3.
Madsen JM, Stoltzfus CM. An exonic splicing silencer downstream of the splice site A2 is required for efficient human immunodeficiency virus type 1 replication. J Virol 2005;79(16):10478–86.
Goodier JL, Zhang L, Vetter MR, Kazazian Jr. HH. LINE-1 ORF1 protein localizes in stress granules with other RNA-binding proteins, including components of RNA interference RNA-induced silencing complex. Mol Cell Biol 2007;27(18):6469–83.
Pedersen I, Fung L, Guzman H, et al. miR-128-induced LINE-1 restriction is dependent on down-regulation of hnRNPA1. bioRxiv 195560; https://doi.org/10.1101/195560.
Karnewar S, Neeli PK, Panuganti D, et al. Metformin regulates mitochondrial biogenesis and senescence through AMPK mediated H3K79 methylation: relevance in age-associated vascular dysfunction. Biochim Biophys Acta 2018;1864(4 Pt A):1115–28.
Chew GM, Chow DC, Souza SA, et al. Impact of adjunctive metformin therapy on T cell exhaustion and viral persistence in a clinical trial of HIV-infected adults on suppressive ART. J Virus Eradication 2017;3(Suppl. 1):6–19.
Chew GM. AAA http://viruseradication.com/abstract-details.php?abstract_id=1188.
Finley J. Oocyte activation and latent HIV-1 reactivation: AMPK as a common mechanism of action linking the beginnings of life and the potential eradication of HIV-1. Med Hypotheses. 2016 Aug;93:34-47.
Finley J. Elimination of cancer stem cells and reactivation of latent HIV-1 via AMPK activation: Common mechanism of action linking inhibition of tumorigenesis and the potential eradication of HIV-1. Med Hypotheses. 2017 Jul;104:133-146.
Finley J. Facilitation of hippocampal long-term potentiation and reactivation of latent HIV-1 via AMPK activation: Common mechanism of action linking learning, memory, and the potential eradication of HIV-1. Med Hypotheses. 2018 Jul;116:61-73.
Finley J. Transposable elements, placental development, and oocyte activation: Cellular stress and AMPK links jumping genes with the creation of human life. Med Hypotheses. 2018 Sep;118:44-54.
Finley J. Cellular stress and AMPK activation as a common mechanism of action linking the effects of metformin and diverse compounds that alleviate accelerated aging defects in Hutchinson-Gilford progeria syndrome. Med Hypotheses. 2018 Sep;118:151-162.
Lutz A, Greischar LL, Rawlings NB, Ricard M, Davidson RJ. Long-term meditators self-induce high-amplitude gamma synchrony during mental practice. Proc Natl Acad Sci U S A. 2004 Nov 16;101(46):16369-73.
Hölzel BK, Carmody J, Vangel M, et al. Mindfulness practice leads to increases in regional brain gray matter density. Psychiatry Res. 2011 Jan 30;191(1):36-43.
Bhasin MK, Dusek JA, Chang BH, et al. Relaxation response induces temporal transcriptome changes in energy metabolism, insulin secretion and inflammatory pathways. PLoS One. 2013 May 1;8(5):e62817.
Richter EA, Ruderman NB. AMPK and the biochemistry of exercise: implications for human health and disease. Biochem J. 2009 Mar 1;418(2):261-75.
0 notes
Text
Metformin shares common mechanism with nearly every Anesthesia drug: AMPK links Consciousness with Jumping Genes & the Creation of Human Life

By ISAF Headquarters Public Affairs Office (originally posted to Flickr as 100410-F-7713A-002) [CC BY 2.0 (https://creativecommons.org/licenses/by/2.0)], via Wikimedia Commons; By Anatomist90 [CC BY-SA 3.0 (https://creativecommons.org/licenses/by-sa/3.0) or GFDL (http://www.gnu.org/copyleft/fdl.html)], from Wikimedia Commons
A recently published study in the journal PLoS One in May of 2018 demonstrated that the anesthetic drug propofol significantly increased intracellular calcium (Ca2+) levels, induced a burst of reactive oxygen species (ROS), and activated the master metabolic regulator AMPK in C2C12 cells [18]. Similar results were also obtained in a recent study published in April of 2018, wherein propofol also increased intracellular Ca2+ levels and activated AMPK in HeLa cells [105]. AMPK is an evolutionarily conserved protein that increases lifespan and healthspan in several model organisms [34]. Activation of AMPK is also the primary mechanism of action of the anti-diabetic drug metformin, a compound that has displayed wide-raging efficacy in multiple disparate disease states, including cancer, dementia, depression, frailty-related diseases, and cardiovascular diseases [34,106]. Interestingly, propofol is considered one of the most popular and widely-used intravenous anesthetic drugs in modern medicine to induce and maintain general anesthesia in humans [107]. Curiously, a recent study published in the journal Current Biology in June of 2018 by researchers from the University of Michigan demonstrated that the compound carbachol reversed anesthesia induced by the inhaled anesthetic sevoflurane and restored wake-like behavior and level of consciousness in rats [27]. Carbachol is a compound that binds to and stimulates acetylcholine receptors in the brain but also activates AMPK in human cells, similar to both metformin and propofol [27,108].
Each of these studies substantiates several novel proposals in a recently published paper I authored in June of 2018 in which I proposed for the first time that cellular stress-induced AMPK activation links consciousness and accelerated emergence from anesthesia with paradoxical excitation, hippocampal long-term potentiation (essential for learning and memory), alleviation of accelerated cellular aging in Hutchinson-Gilford progeria syndrome, oocyte activation and the sperm acrosome reaction (prerequisites for human life creation), and transposable element (i.e. “jumping genes”)-mediated promotion of learning, memory, and the creation of human life [1-6].
As further explained below, nearly every neurotransmitter that plays a critical role in promoting wakefulness, arousal, and consciousness activates AMPK (glutamate, acetylcholine, orexin-A, histamine, norepinephrine, dopamine, and serotonin) [7-17]. Several drugs that are commonly used to induce and maintain general anesthesia also activate AMPK in low doses (propofol, sevoflurane, isoflurane, ketamine, dexmedetomidine, and midazolam) [18-23]. Also, several compounds that have recently been shown to promote accelerated emergence from anesthesia also activate AMPK (carbachol, orexin-A, histamine, dopamine, dopamine D1 receptor agonists, nicotine, caffeine, and forskolin) [9-11,13,24-33].
AMPK, an evolutionarily conserved kinase that is activated by the induction of cellular stress (i.e. increases in intracellular reactive oxygen species [ROS], calcium [Ca2+], and/or an AMP(ADP)/ATP ratio increase), increases lifespan and healthspan in several model organisms (yeast, worms, flies, mice, etc.) [34]. In my prior publication, I first proposed that cellular stress-induced AMPK activation is critical for facilitation of hippocampal long-term potentiation (LTP), considered a cellular correlate for learning and memory [5]. Indeed, AMPK has been found localized in hippocampal CA1 pyramidal neurons and glutamate, NMDA, potassium chloride, and high frequency stimulation have been shown to induce AMPK activation in cortical and hippocampal neurons [7,35,36]. Although an increase in Ca2+ levels is critical for neuronal activation and LTP induction, inhibition of ROS significantly inhibits hippocampal CA1 LTP, indicating that cellular stress-induced AMPK activation may play a pivotal role in neuronal excitation [37-40].
In my most recent publication, I noted that forskolin activates both AMPK and the transposable element syncytin-1 (necessary for human placental formation), increases human oocyte fertilization rates when combined with the AMPK activator cilostamide, and promotes chemically-induced LTP in hippocampal slices [6,26,41-44]. Transposable elements (TEs) are found in human oocytes, human sperm, and in human neural progenitor cells within the hippocampus [45-48]. TEs are also activated and can be induced to transpose or “jump” from one genomic location to another by increases in Ca2+ or ROS [49-51]. Exercise was shown to enhance LINE-1 (L1) retrotransposition (a TE of the retrotransposon class) in the dentate gyrus of the hippocampus in mice and L1 expression and retrotransposition in the adult mouse hippocampus was reported to enable long-term memory formation [52,53]. Because forskolin and caffeine, both of which activate AMPK, have recently been shown to promote accelerated emergence from anesthesia in rats and caffeine activates both mouse oocytes (models for human oocytes) and TEs, I proposed that cellular stress-induced AMPK activation may represent a common mechanism linking consciousness with learning, memory, and the creation of human life [25,26,33,54,55].
A primary cellular target of hypnotic agents (e.g. propofol) used for the induction and maintenance of general anesthesia is the GABAA receptor [66]. The GABAA receptor is located throughout the brain (cortex, thalamus, brain stem, and striatum) and binding of propofol post-synaptically to GABAA receptors enhances neural inhibition by the primary inhibitory neurotransmitter GABA, contributing to a loss of consciousness [66]. Interestingly, the GABAA receptor antagonist bicuculline, which reverses propofol anesthesia, activates AMPK in mouse cortical neurons via Ca2+ influx and flumazenil (a GABAA receptor antagonist) induces preconditioning by increasing the levels of ROS [56-58]. Basheer et al. as well as researchers from the University of Pennsylvania showed that AMPK is activated during extended periods of wakefulness but is inhibited during sleep in the basal forebrain and cerebral cortex of rats and mice [59,60]. Decreases in AMPK activation during sleep were also associated with increases in ATP, which would decrease AMPK activation as increases in the AMP(ADP)/ATP ratio activates AMPK [34,59]. Creatine, which also activates AMPK, decreased total sleep time, NREM sleep, and NREM delta activity significantly in rats [61,62]. Combined use of the anesthetic agents ketamine and xylazine in rats also led to an ATP increase that positively and significantly correlated with EEG delta activity [63]. However, the sedative and α2-receptor agonist clonidine activates AMPK in mice and xylazine, an analog of clonidine, activates AMPK in the rat cerebral cortex, hippocampus, thalamus, and cerebellum, provocatively indicating that low-dose anesthetic administration may actually promote wakefulness, arousal, and consciousness through activation of AMPK [64,65].
Low dose anesthetic-induced AMPK activation may also explain the phenomenon of paradoxical excitation. Curiously, low doses of nearly every anesthetic drug have been shown to induce paradoxical excitation [66]. As the name implies, before inducing unconsciousness, general anesthetic administration may result in a temporary increase in neuronal excitation, characterized by an increase in beta activity on the electroencephalogram (EEG) and eccentric body movements [66,109]. Because AMPK is activated by cellular stress induction (ROS, Ca2+, AMP(ADP)/ATP ratio increase) and because ROS and Ca2+ increases are critical for activation of pyramidal neurons, it is likely that many anesthetics induce rapid neuronal activation and paradoxical excitation in low doses by promoting cellular stress-induced AMPK activation [34,37-40]. Indeed, propofol, one of the most commonly-used anesthetics to induce and maintain general anesthesia, activates AMPK via an increase in ROS and Ca2+, promotes hippocampal neural stem cell differentiation, and promotes neuronal viability [67-69]. Sevoflurane, a commonly-used inhaled anesthetic, activates AMPK via an increase in ROS, increases Ca2+ levels in mouse brain cells, and enhances memory in rats at low doses [70-72]. Ketamine also activates Ca2+ channels in rat cortical neurons, increases ROS levels in the brain of rats, enhances hippocampal CA1 LTP in rats, and also functions as an antidepressant by activating AMPK in the rat hippocampus in vivo [73-76]. Prominent beta activity on the EEG has also been observed just before return of consciousness in healthy adult volunteers anaesthetized with propofol or sevoflurane (similar to paradoxical excitation), suggesting that the decrease of an anesthetic to a low, stimulatory level after removal of anesthesia may explain the increase in beta activity just before return of consciousness as well as during paradoxical excitation [6,66,77]. Hence, low dose anesthetic-induced AMPK activation may potentially accelerate emergence from anesthesia as well as promote beneficial arousal in disorders of consciousness (e.g. minimally conscious state, persistent vegetative state, coma, etc.) [6].
As noted above, nearly every neurotransmitter that plays a critical role in promoting wakefulness, arousal, and consciousness activates AMPK (glutamate, acetylcholine, orexin, histamine, norepinephrine, dopamine, and serotonin) and commonly used drugs that induce and maintain general anesthesia also activate AMPK in low doses (propofol, sevoflurane, isoflurane, ketamine, dexmedetomidine, and midazolam) [7-23]. Compounds that have recently been shown to accelerate emergence from anesthesia also activate AMPK (carbachol, orexin-A, histamine, dopamine, dopamine D1 receptor agonists, nicotine, caffeine, and forskolin) [9-11,13,24-33]. Additionally, a recent study by Hambrecht-Wiedbusch et al. strikingly demonstrated that although sub-anesthetic doses of ketamine increased anesthetic depth and induced burst suppression during isoflurane anesthesia, ketamine paradoxically accelerated recovery of consciousness in rats [78]. Such evidence supports the notion that while larger doses of anesthetics are effective at inducing loss of consciousness, low-dose anesthetic administration may facilitate rapid, cellular stress-induced neuronal activation that is mediated by AMPK activation [6].
Although they do not have a nervous system, plants produce nearly every neurotransmitter that promotes wakefulness, arousal, and consciousness in humans, including glutamate, acetylcholine, histamine, norepinephrine, dopamine, and serotonin [79-82]. The production of these neurotransmitters in plants is often associated with the induction of cellular stress (i.e. via wounding, osmotic stress, etc.) and partly serves as a defense mechanism [79-82]. Fungal infection of certain rice cultivars for example increases the production of serotonin, which suppresses leaf damage and reduces biotic stress [83]. ROS and Ca2+ also play critical roles in the production of secondary metabolites, compounds that plants produce partly for the purpose of self defense [84,85]. Interestingly, several abiotic stressors including nutrient deficiency, salt, osmotic, oxidative, and ER stress activates autophagy in Arabidopsis in a SnRK1-dependent manner. SnRK1 is the plant ortholog of AMPK [86]. Such evidence suggests that a mechanism of cellular stress-induced AMPK activation by neurotransmitters may have been evolutionarily conserved to promote neuronal activation in the human brain.
Indeed, the well-studied AMPK activator metformin activates AMPK in hippocampal neurons in vivo and enhances neurogenesis in the subventricular zone and the subgranular zone of the dentate gyrus, indicating that metformin may enhance brain repair and recovery of consciousness in disorders of consciousness [24,87,88]. Metformin also alleviates accelerated cellular aging defects and activates AMPK in Hutchinson-Gilford progeria syndrome (HGPS), a genetic disorder characterized by an accelerated aging phenotype caused by faulty splicing of the LMNA gene that also occurs in normal human cells at low levels [1,89,90]. Interestingly, temsirolimus (an analog of the macrolide rapamycin), alleviates accelerated aging defects in HGPS cells but increases the levels of ROS in both normal and HGPS cells within the first hour of treatment [91]. Metformin also activates the telomere-lengthening enzyme telomerase (which is derived from a transposable element) in an AMPK-dependent manner [92]. Cellular stress and AMPK activation also promotes oocyte maturation (precedes and is critical for oocyte activation), the acrosome reaction in human sperm (necessary for oocyte penetration and fertilization), and human placental development [26,93-95]. Forskolin and caffeine also induce the acrosome reaction in human sperm [96,110].
Lastly, increases in ROS, Ca2+, and AMPK activation are also critical for T cell activation and hence latent HIV-1 reactivation, a method currently pursued by HIV-1 cure researchers to reactivate dormant HIV-1 residing in T cells to facilitate virus detection and destruction by the immune system (called the “shock and kill” approach) [5,97-101]. Strikingly, forskolin reactivates latent HIV-1 in human U1 cells, a myelo-monocytic cell line used as a model for HIV-1 latency [102]. Early data has also demonstrated that metformin destabilized the latent HIV-1 reservoir in patients chronically infected with HIV-1 and significantly reduced cellular markers positively associated with T cells latently infected with HIV-1 [103,104]. Such evidence provides a compelling indication that cellular stress-induced AMPK activation links transposable elements and alleviation of accelerated cellular aging with potential HIV-1 eradication, consciousness, and the creation of human life, all hypotheses that I originally proposed [1-6].
https://www.linkedin.com/pulse/metformin-shares-common-mechanism-nearly-every-drug-ampk-finley/


References
Finley J. Alteration of splice site selection in the LMNA gene and inhibition of progerin production via AMPK activation. Med Hypotheses. 2014 Nov;83(5):580-7.
Finley J. Reactivation of latently infected HIV-1 viral reservoirs and correction of aberrant alternative splicing in the LMNA gene via AMPK activation: Common mechanism of action linking HIV-1 latency and Hutchinson-Gilford progeria syndrome. Med Hypotheses. 2015 Sep;85(3):320-32.
Finley J. Oocyte activation and latent HIV-1 reactivation: AMPK as a common mechanism of action linking the beginnings of life and the potential eradication of HIV-1. Med Hypotheses. 2016 Aug;93:34-47.
Finley J. Elimination of cancer stem cells and reactivation of latent HIV-1 via AMPK activation: Common mechanism of action linking inhibition of tumorigenesis and the potential eradication of HIV-1. Med Hypotheses. 2017 Jul;104:133-146.
Finley J. Facilitation of hippocampal long-term potentiation and reactivation of latent HIV-1 via AMPK activation: Common mechanism of action linking learning, memory, and the potential eradication of HIV-1. Med Hypotheses. 2018 Jul;116:61-73.
Finley J. Transposable elements, placental development, and oocyte activation: Cellular stress and AMPK links jumping genes with the creation of human life. Med Hypotheses. 2018.
Terunuma M, Vargas KJ, Wilkins ME, et al. Prolonged activation of NMDA receptors promotes dephosphorylation and alters postendocytic sorting of GABAB receptors. Proc. Natl. Acad. Sci. U.S.A. 2010;107(31):13918–23.
Zhao M, Sun L, Yu XJ, et al. Acetylcholine mediates AMPK-dependent autophagic cytoprotection in H9c2 cells during hypoxia/reoxygenation injury. Cell Physiol Biochem. 2013;32(3):601-13.
Merlin J, Evans BA, Csikasz RI, Bengtsson T, Summers RJ, Hutchinson DS. The M3-muscarinic acetylcholine receptor stimulates glucose uptake in L6 skeletal muscle cells by a CaMKK-AMPK-dependent mechanism. Cell Signal. 2010 Jul;22(7):1104-13.
Wu WN, Wu PF, Zhou J, et al. Orexin-A activates hypothalamic AMP-activated protein kinase signaling through a Ca²+-dependent mechanism involving voltage-gated L-type calcium channel. Mol Pharmacol. 2013 Dec;84(6):876-87.
Thors B, Halldórsson H, Thorgeirsson G. eNOS activation mediated by AMPK after stimulation of endothelial cells with histamine or thrombin is dependent on LKB1. Biochim Biophys Acta. 2011 Feb;1813(2):322-31.
Hutchinson DS, Chernogubova E, Dallner OS, Cannon B, Bengtsson T. Beta-adrenoceptors, but not alpha-adrenoceptors, stimulate AMP-activated protein kinase in brown adipocytes independently of uncoupling protein-1. Diabetologia. 2005 Nov;48(11):2386-95.
Bone NB, Liu Z, Pittet JF, Zmijewski JW. Frontline Science: D1 dopaminergic receptor signaling activates the AMPK-bioenergetic pathway in macrophages and alveolar epithelial cells and reduces endotoxin-induced ALI. J Leukoc Biol. 2017 Feb;101(2):357-365.
Laporta J, Peters TL, Merriman KE, Vezina CM, Hernandez LL. Serotonin (5-HT) affects expression of liver metabolic enzymes and mammary gland glucose transporters during the transition from pregnancy to lactation. PLoS One. 2013;8(2):e57847.
Jiang X, Lu W, Shen X, et al. Repurposing sertraline sensitizes non-small cell lung cancer cells to erlotinib by inducing autophagy. JCI Insight. 2018 Jun 7;3(11). pii: 98921.
Sun D, Zhu L, Zhao Y, et al. Fluoxetine induces autophagic cell death via eEF2K-AMPK-mTOR-ULK complex axis in triple negative breast cancer. Cell Prolif. 2018 Apr;51(2):e12402.
Jeong J, Park M, Yoon JS, et al. Requirement of AMPK activation for neuronal metabolic-enhancing effects of antidepressant paroxetine. Neuroreport. 2015 May 6;26(7):424-8.
Chen X, Li LY, Jiang JL, et al. Propofol elicits autophagy via endoplasmic reticulum stress and calcium exchange in C2C12 myoblast cell line. PLoS One. 2018 May 24;13(5):e0197934.
Lamberts RR, Onderwater G, Hamdani N, et al. Reactive oxygen species-induced stimulation of 5'AMP-activated protein kinase mediates sevoflurane-induced cardioprotection. Circulation. 2009 Sep 15;120(11 Suppl):S10-5.
Rao Z, Pan X, Zhang H, et al. Isoflurane Preconditioning Alleviated Murine Liver Ischemia and Reperfusion Injury by Restoring AMPK/mTOR-Mediated Autophagy. Anesth Analg. 2017 Oct;125(4):1355-1363.
Xu SX, Zhou ZQ, Li XM, Ji MH, Zhang GF, Yang JJ. The activation of adenosine monophosphate-activated protein kinase in rat hippocampus contributes to the rapid antidepressant effect of ketamine. Behav Brain Res. 2013 Sep 15;253:305-9.
Sun Y, Jiang C, Jiang J, Qiu L. Dexmedetomidine protects mice against myocardium ischaemic/reperfusion injury by activating an AMPK/PI3K/Akt/eNOS pathway. Clin Exp Pharmacol Physiol. 2017 Sep;44(9):946-953.
Shindo S, Numazawa S, Yoshida T. A physiological role of AMP-activated protein kinase in phenobarbital-mediated constitutive androstane receptor activation and CYP2B induction. Biochem J. 2007 Feb 1;401(3):735-41.
Brynildsen JK, Lee BG, Perron IJ, Jin S, Kim SF, Blendy JA. Activation of AMPK by metformin improves withdrawal signs precipitated by nicotine withdrawal. Proc Natl Acad Sci U S A. 2018 Apr 17;115(16):4282-4287.
Jensen TE, Rose AJ, Hellsten Y, Wojtaszewski JF, Richter EA. Caffeine-induced Ca(2+) release increases AMPK-dependent glucose uptake in rodent soleus muscle. Am J Physiol Endocrinol Metab. 2007 Jul;293(1):E286-92.
Egawa M, Kamata H, Kushiyama A, et al. Long-term forskolin stimulation induces AMPK activation and thereby enhances tight junction formation in human placental trophoblast BeWo cells. Placenta 2008;29(12):1003–8.
Pal D, Dean JG, Liu T, et al. Differential Role of Prefrontal and Parietal Cortices in Controlling Level of Consciousness. Curr Biol. 2018 Jun 12. pii: S0960-9822(18)30627-4.
Zhang LN1, Li ZJ, Tong L, et al. Orexin-A facilitates emergence from propofol anesthesia in the rat. Anesth Analg. 2012 Oct;115(4):789-96.
Luo T, Leung LS. Basal forebrain histaminergic transmission modulates electroencephalographic activity and emergence from isoflurane anesthesia. Anesthesiology. 2009 Oct;111(4):725-33.
Chemali JJ, Van Dort CJ, Brown EN, Solt K. Active emergence from propofol general anesthesia is induced by methylphenidate. Anesthesiology. 2012 May;116(5):998-1005.
Taylor NE, Chemali JJ, Brown EN, Solt K. Activation of D1 dopamine receptors induces emergence from isoflurane general anesthesia. Anesthesiology. 2013 Jan;118(1):30-9.
Alkire MT, McReynolds JR, Hahn EL, Trivedi AN. Thalamic microinjection of nicotine reverses sevoflurane-induced loss of righting reflex in the rat. Anesthesiology. 2007 Aug;107(2):264-72.
Wang Q, Fong R, Mason P, Fox AP, Xie Z. Caffeine accelerates recovery from general anesthesia. J Neurophysiol. 2014 Mar;111(6):1331-40.
Salminen A, Kaarniranta K. AMP-activated protein kinase (AMPK) controls the aging process via an integrated signaling network. Ageing Res Rev 2012;11(2):230–41.
Potter WB, O'Riordan KJ, Barnett D, et al. Metabolic regulation of neuronal plasticity by the energy sensor AMPK. PLoS One. 2010 Feb 1;5(2):e8996.
Yu DF, Shen ZC, Wu PF, et al. HFS-triggered AMPK activation phosphorylates GSK3β and induces E-LTP in rat hippocampus in vivo. CNS Neurosci. Ther.2016;22(6):525–31.
Volianskis A, France G, Jensen MS, et al. Long-term potentiation and the role of Nmethyl-D-aspartate receptors. Brain Res. 2015;24(1621):5–16.
Bindokas VP, Jordán J, Lee CC, Miller RJ. Superoxide production in rat hippocampal neurons: selective imaging with hydroethidine. J. Neurosci. 1996 Feb 15;16(4):1324–36.
Klann E. Cell-permeable scavengers of superoxide prevent long-term potentiation in hippocampal area CA1. J. Neurophysiol. 1998;80(1):452–7.
Thiels E, Urban NN, Gonzalez-Burgos GR, et al. Impairment of long-term potentiation and associative memory in mice that overexpress extracellular superoxide dismutase. J. Neurosci. 2000;20(20):7631–9.
Kudo Y, Boyd CA, Sargent IL, Redman CW. Hypoxia alters expression and function of syncytin and its receptor during trophoblast cell fusion of human placental BeWo cells: implications for impaired trophoblast syncytialisation in preeclampsia. Biochim Biophys Acta 2003;1638(1):63–71.
Shu YM, Zeng HT, Ren Z, et al. Effects of cilostamide and forskolin on the meiotic resumption and embryonic development of immature human oocytes. Hum Reprod 2008;23(3):504–13.
Chung YW, Ahmad F, Tang Y, et al. White to beige conversion in PDE3B KO adipose tissue through activation of AMPK signaling and mitochondrial function. Sci Rep 2017;13(7):40445.
Otmakhov N, Khibnik L, Otmakhova N, et al. Forskolin-induced LTP in the CA1 hippocampal region is NMDA receptor dependent. J Neurophysiol 2004;91(5):1955–62.
Bjerregaard B, Lemmen JG, Petersen MR, et al. Syncytin-1 and its receptor is present in human gametes. J Assist Reprod Genet 2014;31(5):533–9.
Georgiou I, Noutsopoulos D, Dimitriadou E, et al. Retrotransposon RNA expression and evidence for retrotransposition events in human oocytes. Hum Mol Genet 2009;18(7):1221–8.
Lazaros L, Kitsou C, Kostoulas C, et al. Retrotransposon expression and incorporation of cloned human and mouse retroelements in human spermatozoa. Fertil Steril 2017;107(3):821–30.
Coufal NG, Garcia-Perez JL, Peng GE, et al. L1 retrotransposition in human neural progenitor cells. Nature 2009;460(7259):1127–31.
Rodland KD, Muldoon LL, Lenormand P, Magun BE. Modulation of RNA expression by intracellular calcium. Existence of a threshold calcium concentration for induction of VL30 RNA by epidermal growth factor, endothelin, and protein kinase C. J Biol Chem 1990;265(19):11000–7.
Markopoulos G, Noutsopoulos D, Mantziou S, et al. Arsenic induces VL30 retrotransposition: the involvement of oxidative stress and heat-shock protein 70. Toxicol Sci. 2013 Aug;134(2):312-22.
Giorgi G, Marcantonio P, Del Re B. LINE-1 retrotransposition in human neuroblastoma cells is affected by oxidative stress. Cell Tissue Res 2011;346(3):383–91.
Muotri AR, Zhao C, Marchetto MC, Gage FH. Environmental influence on L1 retrotransposons in the adult hippocampus. Hippocampus 2009;19(10):1002–7.
Bachiller S, Del-Pozo-Martín Y, Carrión ÁM. L1 retrotransposition alters the hippocampal genomic landscape enabling memory formation. Brain Behav Immun 2017;64:65–70.
Scott L, Smith S. Human sperm motility-enhancing agents have detrimental effects on mouse oocytes and embryos. Fertil Steril. 1995 Jan;63(1):166-75.
Liu C, Chen Y, Li S, et al. Activation of elements in HERV-W family by caffeine and aspirin. Virus Genes. 2013 Oct;47(2):219-27.
Kenney JW, Sorokina O, Genheden M, Sorokin A, Armstrong JD, Proud CG. Dynamics of elongation factor 2 kinase regulation in cortical neurons in response to synaptic activity. J Neurosci. 2015 Feb 18;35(7):3034-47.
Irifune M, Sugimura M, Takarada T, et al. Propofol anaesthesia in mice is potentiated by muscimol and reversed by bicuculline. Br J Anaesth. 1999 Oct;83(4):665-7.
Zhang Q, Yao Z. Flumazenil preconditions cardiomyocytes via oxygen radicals and K(ATP) channels. Am J Physiol Heart Circ Physiol. 2000 Oct;279(4):H1858-63.
Dworak M, McCarley RW, Kim T, Kalinchuk AV, Basheer R. Sleep and brain energy levels: ATP changes during sleep. J Neurosci. 2010 Jun 30;30(26):9007-16.
Nikonova EV, Naidoo N, Zhang L, et al. Changes in components of energy regulation in mouse cortex with increases in wakefulness. Sleep. 2010 Jul;33(7):889-900.
Ceddia RB, Sweeney G. Creatine supplementation increases glucose oxidation and AMPK phosphorylation and reduces lactate production in L6 rat skeletal muscle cells. J Physiol. 2004 Mar 1;555(Pt 2):409-21.
Dworak M, Kim T, Mccarley RW, Basheer R. Creatine supplementation reduces sleep need and homeostatic sleep pressure in rats. J Sleep Res. 2017 Jun;26(3):377-385.
Dworak M, McCarley RW, Kim T, Basheer R. Delta oscillations induced by ketamine increase energy levels in sleep-wake related brain regions. Neuroscience. 2011 Dec 1;197:72-9.
Kim SS, Park SH, Lee JR, Jung JS, Suh HW. The activation of α2-adrenergic receptor in the spinal cord lowers sepsis-induced mortality. Korean J Physiol Pharmacol. 2017 Sep;21(5):495-507.
Shi XX, Yin BS, Yang P, et al. Xylazine Activates Adenosine Monophosphate-Activated Protein Kinase Pathway in the Central Nervous System of Rats. PLoS One. 2016 Apr 6;11(4):e0153169.
Brown EN, Lydic R, Schiff ND. General anesthesia, sleep, and coma. N Engl J Med 2010;363(27):2638–50.
Chen X, Li LY, Jiang JL, et al. Propofol elicits autophagy via endoplasmic reticulum stress and calcium exchange in C2C12 myoblast cell line. PLoS One. 2018 May 24;13(5):e0197934.
Sall JW, Stratmann G, Leong J, Woodward E, Bickler PE. Propofol at clinically relevant concentrations increases neuronal differentiation but is not toxic to hippocampal neural precursor cells in vitro. Anesthesiology. 2012 Nov;117(5):1080-90.
Wu GJ, Chen WF, Hung HC, et al. Effects of propofol on proliferation and anti-apoptosis of neuroblastoma SH-SY5Y cell line: new insights into neuroprotection. Brain Res. 2011 Apr 12;1384:42-50.
Lamberts RR, Onderwater G, Hamdani N, et al. Reactive oxygen species-induced stimulation of 5'AMP-activated protein kinase mediates sevoflurane-induced cardioprotection. Circulation. 2009 Sep 15;120(11 Suppl):S10-5.
Pinheiro AC, Gomez RS, Guatimosim C, Silva JH, Prado MA, Gomez MV. The effect of sevoflurane on intracellular calcium concentration from cholinergic cells. Brain Res Bull. 2006 Mar 31;69(2):147-52.
Alkire MT, Nathan SV, McReynolds JR. Memory enhancing effect of low-dose sevoflurane does not occur in basolateral amygdala-lesioned rats. Anesthesiology. 2005 Dec;103(6):1167-73.
Lepack AE, Fuchikami M, Dwyer JM, Banasr M, Duman RS. BDNF release is required for the behavioral actions of ketamine. Int J Neuropsychopharmacol. 2014 Oct 31;18(1). pii: pyu033.
de Oliveira L, Spiazzi CM, Bortolin T, et al. Different sub-anesthetic doses of ketamine increase oxidative stress in the brain of rats. Prog Neuropsychopharmacol Biol Psychiatry. 2009 Aug 31;33(6):1003-8.
Widman AJ, Stewart AE, Erb EM, Gardner E, McMahon LL. Intravascular Ketamine Increases Theta-Burst but Not High Frequency Tetanus Induced LTP at CA3-CA1 Synapses Within Three Hours and Devoid of an Increase in Spine Density. Front Synaptic Neurosci. 2018 May 30;10:8.
Xu SX, Zhou ZQ, Li XM, Ji MH, Zhang GF, Yang JJ. The activation of adenosine monophosphate-activated protein kinase in rat hippocampus contributes to the rapid antidepressant effect of ketamine. Behav Brain Res. 2013 Sep 15;253:305-9.
Gugino LD, Chabot RJ, Prichep LS, John ER, Formanek V, Aglio LS. Quantitative EEG changes associated with loss and return of consciousness in healthy adult volunteers anaesthetized with propofol or sevoflurane. Br J Anaesth 2001;87(3):421–8.
Hambrecht-Wiedbusch VS, Li D, Mashour GA. Paradoxical Emergence: Administration of Subanesthetic Ketamine during Isoflurane Anesthesia Induces Burst Suppression but Accelerates Recovery. Anesthesiology. 2017 Mar;126(3):482-494.
Kulma A, Szopa J. Catecholamines are active compounds in plants. Plant Science Volume 172, Issue 3, March 2007, Pages 433-440.
Roshchina V.V. (2010) Evolutionary Considerations of Neurotransmitters in Microbial, Plant, and Animal Cells. In: Lyte M., Freestone P. (eds) Microbial Endocrinology. Springer, New York, NY.
Murch S.J. (2006) Neurotransmitters, Neuroregulators and Neurotoxins in Plants. In: Baluška F., Mancuso S., Volkmann D. (eds) Communication in Plants. Springer, Berlin, Heidelberg.
Skopelitis DS, Paranychianakis NV, Paschalidis KA, et al. Abiotic stress generates ROS that signal expression of anionic glutamate dehydrogenases to form glutamate for proline synthesis in tobacco and grapevine. Plant Cell. 2006 Oct;18(10):2767-81.
Hayashi K, Fujita Y, Ashizawa T, Suzuki F, Nagamura Y, Hayano-Saito Y. Serotonin attenuates biotic stress and leads to lesion browning caused by a hypersensitive response to Magnaporthe oryzae penetration in rice. Plant J. 2016 Jan;85(1):46-56.
Jacobo-Velázquez DA, González-Agüero M, Cisneros-Zevallos L. Cross-talk between signaling pathways: the link between plant secondary metabolite production and wounding stress response. Sci Rep. 2015 Feb 25;5:8608.
Blume B, Nürnberger T, Nass N, Scheel D. Receptor-mediated increase in cytoplasmic free calcium required for activation of pathogen defense in parsley. Plant Cell. 2000 Aug;12(8):1425-40.
Soto-Burgos J, Bassham DC. SnRK1 activates autophagy via the TOR signaling pathway in Arabidopsis thaliana. PLoS One. 2017 Aug 4;12(8):e0182591.
Dadwal P, Mahmud N, Sinai L, et al. Activating Endogenous Neural Precursor Cells Using Metformin Leads to Neural Repair and Functional Recovery in a Model of Childhood Brain Injury. Stem Cell Reports. 2015 Aug 11;5(2):166-73.
Ahmed S, Mahmood Z, Javed A, et al. Effect of metformin on adult hippocampal neurogenesis: comparison with donepezil and links to cognition. J Mol Neurosci 2017;62(1):88–98.
Egesipe AL, Blondel S, Cicero AL, et al. Metformin decreases progerin expression and alleviates pathological defects of Hutchinson-Gilford progeria syndrome cells. NPJ Aging Mech Dis 2016;10(2):16026.
Park SK, Shin OS. Metformin alleviates ageing cellular phenotypes in Hutchinson-Gilford progeria syndrome dermal fibroblasts. Exp Dermatol 2017;26(10):889–95.
Gabriel D, Gordon LB, Djabali K. Temsirolimus partially rescues the Hutchinson-Gilford progeria cellular phenotype. PLoS One 2016;11(12):e0168988.
Karnewar S, Neeli PK, Panuganti D, et al. Metformin regulates mitochondrial biogenesis and senescence through AMPK mediated H3K79 methylation: relevance in age-associated vascular dysfunction. Biochim Biophys Acta 2018;1864(4 Pt A):1115–28.
LaRosa C, Downs SM. Stress stimulates AMP-activated protein kinase and meiotic resumption in mouse oocytes. Biol Reprod 2006;74(3):585–92.
Calle-Guisado V, de Llera AH, Martin-Hidalgo D, et al. AMP-activated kinase in human spermatozoa: identification, intracellular localization, and key function in the regulation of sperm motility. Asian J Androl 2017;19(6):707–14.
de Lamirande E, Tsai C, Harakat A, Gagnon C. Involvement of reactive oxygen species in human sperm arcosome reaction induced by A23187, lysophosphatidylcholine, and biological fluid ultrafiltrates. J Androl 1998;19(5):585–94.
De Jonge CJ, Han HL, Lawrie H, Mack SR, Zaneveld LJ. Modulation of the human sperm acrosome reaction by effectors of the adenylate cyclase/cyclic AMP second messenger pathway. J Exp Zool 1991;258(1):113–25.
Dahabieh MS, Battivelli E, Verdin E. Understanding HIV latency: the road to an HIV cure. Annu Rev Med 2015;66:407–21.
Spina CA, Anderson J, Archin NM, et al. An in-depth comparison of latent HIV-1 reactivation in multiple cell model systems and resting CD4+ T cells from aviremic patients. PLoS Pathog 2013;9(12):e1003834.
Sena LA, Li S, Jairaman A, et al. Mitochondria are required for antigen-specific T cell activation through reactive oxygen species signaling. Immunity 2013;38(2):225–36.
Rao E, Zhang Y, Zhu G, et al. Deficiency of AMPK in CD8+ T cells suppresses their anti-tumor function by inducing protein phosphatase-mediated cell death. Oncotarget 2015;6(10):7944–58.
Zhou H, Xu M, Huang Q, et al. Genome-scale RNAi screen for host factors required for HIV replication. Cell Host Microbe 2008;4(5):495–504.
Chowdhury MI, Koyanagi Y, Horiuchi S, et al. cAMP stimulates human immunodeficiency virus (HIV-1) from latently infected cells of monocyte-macrophage lineage: synergism with TNF-alpha. Virology 1993;194(1):345–9.
Chew GM, Chow DC, Souza SA, et al. Impact of adjunctive metformin therapy on T cell exhaustion and viral persistence in a clinical trial of HIV-infected adults on suppressive ART. J Virus Eradication 2017;3(Suppl. 1):6–19.
Chew GM, Chow DC, Souza SA, et al. http://viruseradication.com/abstract-details.php?abstract_id=1188, last accessed June 28, 2018.
Chen X, Li K, Zhao G. Propofol Inhibits HeLa Cells by Impairing Autophagic Flux via AMP-Activated Protein Kinase (AMPK) Activation and Endoplasmic Reticulum Stress Regulated by Calcium. Med Sci Monit. 2018 Apr 18;24:2339-2349.
Wang CP, Lorenzo C, Habib SL, Jo B, Espinoza SE. Differential effects of metformin on age related comorbidities in older men with type 2 diabetes. J. Diabetes Complications 2017;31(4):679–86.
Chidambaran V, Costandi A, D'Mello A. Propofol: a review of its role in pediatric anesthesia and sedation. CNS Drugs. 2015 Jul;29(7):543-63.
Olianas MC, Dedoni S, Onali P. Involvement of store-operated Ca(2+) entry in activation of AMP-activated protein kinase and stimulation of glucose uptake by M3 muscarinic acetylcholine receptors in human neuroblastoma cells. Biochim Biophys Acta. 2014 Dec;1843(12):3004-17.
McCarthy MM, Brown EN, Kopell N. Potential network mechanisms mediating electroencephalographic beta rhythm changes during propofol-induced paradoxical excitation. J Neurosci 2008;28(50):13488–504.
Tesarik J, Mendoza C, Carreras A. Effects of phosphodiesterase inhibitors caffeine and pentoxifylline on spontaneous and stimulus-induced acrosome reactions in human sperm. Fertil Steril. 1992 Dec;58(6):1185-90.
0 notes
Text
Metformin & AMPK Link Nobel Prize-winning Telomeres & Jumping Genes with Learning, HIV, & the Creation of Human Life

Nobel Prize winners, from left to right: Elizabeth Blackburn (discovered telomerase), Barbara McClintock (discovered “jumping genes”), and Françoise Barré-Sinoussi (discovered HIV). By Science History Institute, CC BY-SA 3.0, https://commons.wikimedia.org/w/index.php?curid=30112731; By Smithsonian Institution/Science Service; Restored by Adam Cuerden - Flickr: Barbara McClintock (1902-1992), Public Domain, https://commons.wikimedia.org/w/index.php?curid=25629182; By Prolineserver (talk) - Own work, GFDL 1.2, https://commons.wikimedia.org/w/index.php?curid=5395403
A recently published study in the journal Biochimica et Biophysica Acta (BBA) - Molecular Basis of Disease in 2018 demonstrated for the first time that chronic treatment with the anti-diabetic drug metformin activated human telomerase in human aortic endothelial cells (HAECs) and significantly delayed endothelial senescence in an AMPK-dependent manner [11]. Telomeres are specialized regions of repetitive nucleotide sequences located at the ends of eukaryotic chromosomes that protect chromosomal ends from deterioration [63]. However, continuous cell division leads to telomere shortening, impeding the replenishment of tissues and triggering cellular senescence (i.e. cells cease to divide). Although human telomeres shorten with age, telomeres may be lengthened by the enzyme telomerase [64].
This study substantiates and confirms several novel proposals in a recently published paper I authored in April of 2018 in which I first proposed that because telomerase is derived from a “jumping gene” (see below for discussion), metformin would activate telomerase via AMPK [6]. My paper also highlights a novel link between hippocampal long-term potentiation (essential for learning and memory), alleviation of accelerated cellular aging in Hutchinson-Gilford progeria syndrome, oocyte activation and the sperm acrosome reaction (prerequisites for human life creation), and transposable element (i.e. “jumping genes”)-mediated promotion of learning, memory, and the creation of human life [1-7]. Indeed, these novel proposals also link several Nobel Prize-winning discoveries, including the discovery of telomerase by Elizabeth Blackburn (photo-left), the discovery of “jumping genes” by Barbara McClintock (photo-middle), and the discovery of HIV by Françoise Barré-Sinoussi (photo-right).
The link between such disparate physiological and pathophysiological phenomena is cellular stress-induced modulation of energy metabolism, leading to the activation of the master metabolic regulator AMPK, a kinase that increases lifespan and healthspan in several model organisms [12]. I was the first to propose and publish (2014) that an increase in beneficial levels of cellular stress (e.g. increases in the levels reactive oxygen species [ROS], calcium [Ca2+], and/or an AMP(ADP)/ATP ratio increase, etc.) and activation of AMPK by compounds including metformin would alleviate accelerated cellular aging defects in children diagnosed with the genetic disorder Hutchinson-Gilford progeria syndrome (HGPS), a disease characterized by an accelerated aging phenotype and death at ~14.6 years of age [1]. This hypothesis was substantiated in 2016 and 2017, with metformin activating AMPK in cells taken from HGPS kids and ameliorating accelerated cellular aging defects (e.g. correcting nuclear morphology, decrease in senescence markers, etc.) [8,9]. Additionally, transfection of telomerase been shown to reverse senescence in HGPS cells [65]. As metformin activates telomerase and normal humans make the same toxic protein (called progerin) that leads to accelerated aging in HGPS kids (just at lower amounts that accumulate with age), AMPK activation may also play a significant role in ameliorating diseases associated with physiological aging [10,11].
AMPK also links HGPS with potential virus eradication. I first proposed in 2015 that AMPK activation links alleviation of accelerated aging in HGPS with the potential eradication of HIV-1 via the “shock and kill” approach, a method currently being pursued by HIV cure researchers to possibly eradicate HIV-1 [2,13]. The same gene splicing factor that promotes accelerated aging in HGPS (called SRSF1 or ASF/SF2) also inhibits reactivation of latent HIV-1 (i.e. “shock”), preventing immune system detection and virus destruction (i.e. “kill”) [8,14]. Metformin was shown to slow aging in HGPS cells by decreasing this splicing factor, as I originally predicted in 2014, and several compounds that potently induce latent HIV-1 reactivation in T cells from infected patients, including PMA (a phorbol ester) combined with ionomycin, each activate AMPK [1,8,15-17]. AMPKα1 deletion leads to a decrease in primary T cell responses to bacterial and viral infections in vivo, AMPK knockdown leads to cell death on T cell activation, and metformin has recently been shown to inhibit Zika and Dengue viruses, the malaria parasite, and Legionella pneumophila [18-23]. Intriguingly, early data presented at the International AIDS Conference in 2017 demonstrated that metformin destabilized the latent HIV-1 reservoir in chronically-infected HIV patients and decreased the percentage of CD4+ T cells expressing the immune checkpoint receptors PD-1, TIGIT, and TIM-3, each markers associated with T cells latently infected with HIV-1, indicating that AMPK activation may indeed contribute to a cure for HIV-1 [24,25].
As I first proposed in 2016 and 2017, the induction of cellular stress and AMPK activation also links HGPS and potential HIV-1 eradication with oocyte activation and the sperm acrosome reaction, prerequisites for the creation of human life [3,4]. Increases in both ROS and Ca2+ are critical for T cell activation (and hence latent HIV-1 reactivation) and ROS is transiently increased in HGPS cells when treated with a rapamycin analog to alleviate accelerated aging [26-28]. Stress-induced activation of AMPK by AICAR and other compounds promotes oocyte maturation, which precedes and is essential for efficient oocyte activation [29,30]. Oocyte activation is indispensable for the creation of all human life and PMA and ionomycin, which collectively reactivates latent HIV-1, activates mouse and human oocytes, respectively [31,32]. AMPK is also found in the acrosome of the human sperm head and ionomycin induces the acrosome reaction in human sperm, a process necessary for oocyte penetration and fertilization [33,34]. Ionomycin is also used extensively during fertility procedures to activate human oocytes (i.e. “shock”), creating normal, healthy children (i.e. “live”) [32]. Interestingly, ionomycin is a narrow spectrum antibiotic produced by certain species within the bacterial genus Streptomyces, from which ~70 percent of clinically useful antibiotics are derived [35,36]. Cellular stress, mediated by increases in ROS, Ca2+, and/or an AMP(ADP)/ATP ratio increase, etc. also enhances antibiotic production in many Streptomyces strains, reinforcing the notion that the beneficial effects of cellular stress induction crosses species boundaries [37,38].
Cellular stress induction and AMPK activation also link HGPS, potential HIV-1 eradication, and human life creation with learning and memory, a hypothesis I originally proposed in 2018 [6]. Hippocampal long-term potentiation (LTP) is considered the cellular correlate of learning and memory and AMPK has been found localized in hippocampal CA1 dendrites and is activated in neurons by metformin, AICAR, ionomycin, and glutamate, a neurotransmitter essential for hippocampal LTP induction [39-41]. The glutamate receptors AMPAR and NMDAR are found on and modulate T cell activation, AMPK activation increases synthesis and membrane insertion of AMPARs (critical for LTP expression), PMA enhances hippocampal CA1 LTP, and inhibition of ROS significantly impairs hippocampal CA1 LTP [42-46]. Also, neuronal depolarization decreases the recruitment efficiency of SRSF1 to nascent RNAs and promotes SRSF1 nuclear speckle accumulation [6]. SRSF1, a gene splicing factor that is inhibited by metformin, enhances progerin production in HGPS cells and prevents latent HIV-1 reactivation [2,8]. Metformin also significantly reduces pathology-associated reductions in LTP in animal models in vivo, indicating that learning and memory are linked to HGPS, potential HIV-1 eradication, and human life creation via the induction of beneficial levels of cellular stress [47].
Lastly, cellular stress and AMPK activation also links the activation and mobilization of transposable elements (i.e. “jumping genes”) with telomerase activation, potential HIV-1 eradication, learning and memory, and the creation of human life, a hypothesis I originally proposed in 2018 [6]. Transposable elements (TEs) are DNA sequences first described by Nobel laureate Barbara McClintock that comprise nearly half of the human genome, are able to transpose or move from one genomic location to another, and have played an extensive role in human genome evolution [48-50]. Strikingly, McClintock also described in her Nobel Prize speech that a genome “shock” seemed to promote TE activation and mobilization [50]. As first noted in my recently published paper, this “shock” is the same “shock” that HIV cure researchers are using during the “shock and kill” approach to reactivate latent HIV-1 to potentially effectuate a cure [6]. Indeed, several forms of cellular stress, including heat shock and radiation, have been convincingly shown to activate and enhance TE mobilization in several model organisms and in human cells [51-53]. This same “shock” McClintock referred to, mediated by increases in ROS, Ca2+, and/or an AMP(ADP)/ATP ratio, etc. is also what leads to the creation of human life, as the antibiotic ionomycin activates AMPK, promotes TE activation, and induces human oocyte activation [17,32,54]. LINE-1 (L1), a member of the retrotransposon class of TEs, is active and capable of mobilization in human oocytes, human sperm, and in human neural progenitor cells [55-57]. Inhibition of L1 impairs both oocyte maturation in vitro and long-term memory formation in vivo in mice [58,59]. L1 has also been detected in the human brain and is capable of mobilization in human neurons [57]. As noted above, AMPK is critical for oocyte maturation and metformin promotes hippocampal neurogenesis and spatial memory formation [29,60]. The landmark initial sequencing of the human genome also noted that both telomerase and RAG1 (promotes DNA cleavage and transposition in human cells) are derived from TEs [49]. Because metformin activates both telomerase and RAG1 via AMPK, it is likely that cellular stress-induced AMPK activation facilitates beneficial TE activation and mobilization (i.e. learning and memory associated with L1 mobilization), linking human genome evolution and the creation of human life with hippocampal LTP, HGPS, and potential HIV-1 eradication [61,62].
https://www.linkedin.com/pulse/metformin-ampk-link-nobel-prize-winning-telomeres-jumping-finley/


References
Finley J. Alteration of splice site selection in the LMNA gene and inhibition of progerin production via AMPK activation. Med Hypotheses. 2014 Nov;83(5):580-7.
Finley J. Reactivation of latently infected HIV-1 viral reservoirs and correction of aberrant alternative splicing in the LMNA gene via AMPK activation: Common mechanism of action linking HIV-1 latency and Hutchinson-Gilford progeria syndrome. Med Hypotheses. 2015 Sep;85(3):320-32.
Finley J. Oocyte activation and latent HIV-1 reactivation: AMPK as a common mechanism of action linking the beginnings of life and the potential eradication of HIV-1. Med Hypotheses. 2016 Aug;93:34-47.
Finley J. Elimination of cancer stem cells and reactivation of latent HIV-1 via AMPK activation: Common mechanism of action linking inhibition of tumorigenesis and the potential eradication of HIV-1. Med Hypotheses. 2017 Jul;104:133-146.
Finley J. AMPK activation as a common mechanism of action linking the effects of diverse compounds that ameliorate accelerated cellular aging defects in Hutchinson-Gilford progeria syndrome. Med Hypotheses. Manuscript submitted.
Finley J. Facilitation of hippocampal long-term potentiation and reactivation of latent HIV-1 via AMPK activation: Common mechanism of action linking learning, memory, and the potential eradication of HIV-1. Med Hypotheses. 2018 Jul;116: 61-73.
Finley J. Transposable elements, placental development, and oocyte activation: Cellular stress and AMPK links jumping genes with the creation of human life. Med Hypotheses. Under Review.
Egesipe AL, Blondel S, Cicero AL, et al. Metformin decreases progerin expression and alleviates pathological defects of Hutchinson-Gilford progeria syndrome cells. NPJ Aging Mech Dis. 2016 Nov 10;2:16026.
Park SK, Shin OS. Metformin alleviates ageing cellular phenotypes in Hutchinson-Gilford progeria syndrome dermal fibroblasts. Exp Dermatol. 2017 Oct;26(10):889-895.
McClintock D, Ratner D, Lokuge M, et al. The mutant form of lamin A that causes Hutchinson-Gilford progeria is a biomarker of cellular aging in human skin. PLoS One. 2007 Dec 5;2(12):e1269.
Karnewar S, Neeli PK, Panuganti D, et al. Metformin regulates mitochondrial biogenesis and senescence through AMPK mediated H3K79 methylation: Relevance in age-associated vascular dysfunction. Biochim Biophys Acta. 2018 Apr;1864(4 Pt A):1115-1128.
Salminen A, Kaarniranta K. AMP-activated protein kinase (AMPK) controls the aging process via an integrated signaling network. Ageing Res Rev. 2012 Apr;11(2):230-41.
Kim Y, Anderson JL, Lewin SR. Getting the "Kill" into "Shock and Kill": Strategies to Eliminate Latent HIV. Cell Host Microbe. 2018 Jan 10;23(1):14-26.
Berro R, Kehn K, de la Fuente C, et al. Acetylated Tat regulates human immunodeficiency virus type 1 splicing through its interaction with the splicing regulator p32. J Virol 2006;80(7):3189–204.
Spina CA, Anderson J, Archin NM, et al. An in-depth comparison of latent HIV-1 reactivation in multiple cell model systems and resting CD4+ T cells from aviremic patients. PLoS Pathog 2013;9(12):e1003834.
Zogovic N, Tovilovic-Kovacevic G, Misirkic-Marjanovic M, et al. Coordinated activation of AMP-activated protein kinase, extracellular signal-regulated kinase, and autophagy regulates phorbol myristate acetate-induced differentiation of SH-SY5Y neuroblastoma cells. J Neurochem 2015;133 (2):223–32.
Tamás P, Hawley SA, Clarke RG, et al. Regulation of the energy sensor AMP-activated protein kinase by antigen receptor and Ca2+ in T lymphocytes. J Exp Med. 2006 Jul 10;203(7):1665-70.
Blagih J, Coulombe F, Vincent EE, et al. The energy sensor AMPK regulates T cell metabolic adaptation and effector responses in vivo. Immunity. 2015 Jan 20;42(1):41-54.
Rao E, Zhang Y, Zhu G, et al. Deficiency of AMPK in CD8+ T cells suppresses their anti-tumor function by inducing protein phosphatase-mediated cell death. Oncotarget. 2015 Apr 10;6(10):7944-58.
Cheng F, Ramos da Silva S, Huang IC, Jung JU, Gao SJ. Suppression of Zika virus infection and replication in endothelial cells and astrocytes by PKA inhibitor PKI 14-22. J Virol. 2017 Dec 6. pii: JVI.02019-17.
Soto-Acosta R, Bautista-Carbajal P, Cervantes-Salazar M, Angel-Ambrocio AH, Del Angel RM. DENV up-regulates the HMG-CoA reductase activity through the impairment of AMPK phosphorylation: A potential antiviral target. PLoS Pathog. 2017 Apr 6;13(4):e1006257.
Ruivo MT, Vera IM, Sales-Dias J, et al. Host AMPK Is a Modulator of Plasmodium Liver Infection. Cell Rep. 2016 Sep 6;16(10):2539-45.
Kajiwara C, Kusaka Y, Kimura S, et al. Metformin Mediates Protection against Legionella Pneumonia through Activation of AMPK and Mitochondrial Reactive Oxygen Species. J Immunol. 2017 Dec 15. pii: ji1700474.
G.M. Chew, D.C. Chow, S.A. Souza, et al. Impact of adjunctive metformin therapy on T cell exhaustion and viral persistence in a clinical trial of HIV-infected adults on suppressive ART. Journal of Virus Eradication 2017; 3 (Supplement 1): 6–19.
http://viruseradication.com/abstract-details.php?abstract_id=1188, last accessed March 14, 2018.
Oh-hora M, Rao A. Calcium signaling in lymphocytes. Curr Opin Immunol 2008;20(3):250–8.
Sena LA, Li S, Jairaman A, et al. Mitochondria are required for antigen-specific T cell activation through reactive oxygen species signaling. Immunity. 2013 Feb 21;38(2):225-36.
Gabriel D, Gordon LB, Djabali K. Temsirolimus Partially Rescues the Hutchinson-Gilford Progeria Cellular Phenotype. PLoS One. 2016 Dec 29;11(12):e0168988.
Chen J, Hudson E, Chi MM, et al. AMPK regulation of mouse oocyte meiotic resumption in vitro. Dev Biol. 2006 Mar 15;291(2):227-38.
LaRosa C, Downs SM. Stress stimulates AMP-activated protein kinase and meiotic resumption in mouse oocytes. Biol Reprod. 2006 Mar;74(3):585-92.
Uranga JA, Pedersen RA, Arechaga J. Parthenogenetic activation of mouse oocytes using calcium ionophores and protein kinase C stimulators. Int J Dev Biol. 1996 Apr;40(2):515-9.
Deemeh MR, Tavalaee M, Nasr-Esfahani MH. Health of children born through artificial oocyte activation: a pilot study. Reprod Sci. 2015 Mar;22(3):322-8.
Calle-Guisado V, de Llera AH, Martin-Hidalgo D, et al. AMP-activated kinase in human spermatozoa: identification, intracellular localization, and key function in the regulation of sperm motility. Asian J Androl. 2017 Nov-Dec;19(6):707-714.
Sánchez-Cárdenas C, Servín-Vences MR, José O, Treviño CL, Hernández-Cruz A, Darszon A. Acrosome reaction and Ca²+ imaging in single human spermatozoa: new regulatory roles of [Ca²+]i. Biol Reprod. 2014 Sep;91(3):67.
Liu WC, Slusarchyk DS, Astle G, Trejo WH, Brown WE, Meyers E. Ionomycin, a new polyether antibiotic. J Antibiot (Tokyo). 1978 Sep;31(9):815-9.
Kitani S, Miyamoto KT, Takamatsu S, et al. Avenolide, a Streptomyces hormone controlling antibiotic production in Streptomyces avermitilis. Proc Natl Acad Sci U S A. 2011 Sep 27;108(39):16410-5.
Meng L, Li M, Yang SH, Kim TJ, Suh JW. Intracellular ATP levels affect secondary metabolite production in Streptomyces spp. Biosci Biotechnol Biochem. 2011;75(8):1576-81.
Wang D, Wei L, Zhang Y, Zhang M, Gu S. Physicochemical and microbial responses of Streptomyces natalensis HW-2 to fungal elicitor. Appl Microbiol Biotechnol. 2017 Sep;101(17):6705-6712.
Potter WB, O'Riordan KJ, Barnett D, et al. Metabolic regulation of neuronal plasticity by the energy sensor AMPK. PLoS One. 2010 Feb 1;5(2):e8996.
Sample V, Ramamurthy S, Gorshkov K, Ronnett GV, Zhang J. Polarized activities of AMPK and BRSK in primary hippocampal neurons. Mol Biol Cell. 2015 May 15;26(10):1935-46
Terunuma M, Vargas KJ, Wilkins ME, et al. Prolonged activation of NMDA receptors promotes dephosphorylation and alters postendocytic sorting of GABAB receptors. Proc Natl Acad Sci U S A. 2010 Aug 3;107(31):13918-23.
Ganor Y, Besser M, Ben-Zakay N, Unger T, Levite M. Human T cells express a functional ionotropic glutamate receptor GluR3, and glutamate by itself triggers integrin-mediated adhesion to laminin and fibronectin and chemotactic migration. J Immunol. 2003 Apr 15;170(8):4362-72.
Miglio G, Varsaldi F, Lombardi G. Human T lymphocytes express N-methyl-D-aspartate receptors functionally active in controlling T cell activation. Biochem Biophys Res Commun. 2005 Dec 30;338(4):1875-83.
Wang G, Amato S, Gilbert J, Man HY. Resveratrol up-regulates AMPA receptor expression via AMP-activated protein kinase-mediated protein translation. Neuropharmacology. 2015 Aug;95:144-53.
Kim EC, Lee MJ, Shin SY, et al. Phorbol 12-Myristate 13-Acetate Enhances Long-Term Potentiation in the Hippocampus through Activation of Protein Kinase Cδ and ε. Korean J Physiol Pharmacol. 2013 Feb;17(1):51-6.
Klann E. Cell-permeable scavengers of superoxide prevent long-term potentiation in hippocampal area CA1. J Neurophysiol. 1998 Jul;80(1):452-7.
Asadbegi M, Yaghmaei P, Salehi I, Ebrahim-Habibi A, Komaki A. Neuroprotective effects of metformin against Aβ-mediated inhibition of long-term potentiation in rats fed a high-fat diet. Brain Res Bull. 2016 Mar;121:178-85.
Muñoz-López M, García-Pérez JL. DNA transposons: nature and applications in genomics. Curr Genomics. 2010 Apr;11(2):115-28.
Lander ES, Linton LM, Birren B, et al. Initial sequencing and analysis of the human genome. Nature. 2001 Feb 15;409(6822):860-921.
McClintock B. The significance of responses of the genome to challenge. Science. 1984 Nov 16;226(4676):792-801.
Stanley D, Fraser S, Stanley GA, Chambers PJ. Retrotransposon expression in ethanol-stressed Saccharomyces cerevisiae. Appl Microbiol Biotechnol. 2010 Jul;87(4):1447-54.
Vasilyeva LA, Bubenshchikova EV, Ratner VA. Heavy heat shock induced retrotransposon transposition in Drosophila. Genet Res. 1999 Oct;74(2):111-9.
Farkash EA, Kao GD, Horman SR, Prak ET. Gamma radiation increases endonuclease-dependent L1 retrotransposition in a cultured cell assay. Nucleic Acids Res. 2006 Feb 28;34(4):1196-204.
Magun BE, Rodland KD. Transient inhibition of protein synthesis induces the immediate early gene VL30: alternative mechanism for thapsigargin-induced gene expression. Cell Growth Differ. 1995 Jul;6(7):891-7.
Georgiou I, Noutsopoulos D, Dimitriadou E, et al. Retrotransposon RNA expression and evidence for retrotransposition events in human oocytes. Hum Mol Genet. 2009 Apr 1;18(7):1221-8.
Lazaros L, Kitsou C, Kostoulas C, et al. Retrotransposon expression and incorporation of cloned human and mouse retroelements in human spermatozoa. Fertil Steril. 2017 Mar;107(3):821-830.
Coufal NG, Garcia-Perez JL, Peng GE, et al. L1 retrotransposition in human neural progenitor cells. Nature. 2009 Aug 27;460(7259):1127-31.
Luo YB, Zhang L, Lin ZL, et al. Distinct subcellular localization and potential role of LINE1-ORF1P in meiotic oocytes. Histochem Cell Biol. 2016 Jan;145(1):93-104.
Bachiller S, Del-Pozo-Martín Y, Carrión ÁM. L1 retrotransposition alters the hippocampal genomic landscape enabling memory formation. Brain Behav Immun. 2017 Aug;64:65-70.
Ahmed S, Mahmood Z, Javed A, et al. Effect of Metformin on Adult Hippocampal Neurogenesis: Comparison with Donepezil and Links to Cognition. J Mol Neurosci. 2017 May;62(1):88-98.
Karnewar S, Neeli PK, Panuganti D, et al. Metformin regulates mitochondrial biogenesis and senescence through AMPK mediated H3K79 methylation: Relevance in age-associated vascular dysfunction. Biochim Biophys Acta. 2018 Apr;1864(4 Pt A):1115-1128.
Um JH, Brown AL, Singh SK, et al. Metabolic sensor AMPK directly phosphorylates RAG1 protein and regulates V(D)J recombination. Proc Natl Acad Sci U S A. 2013 Jun 11;110(24):9873-8.
O'Sullivan RJ, Karlseder J. Telomeres: protecting chromosomes against genome instability. Nat Rev Mol Cell Biol. 2010 Mar;11(3):171-81.
Cohen SB, Graham ME, Lovrecz GO, Bache N, Robinson PJ, Reddel RR. Protein composition of catalytically active human telomerase from immortal cells. Science. 2007 Mar 30;315(5820):1850-3.
Li Y, Zhou G, Bruno IG, Cooke JP. Telomerase mRNA Reverses Senescence in Progeria Cells. J Am Coll Cardiol. 2017 Aug 8;70(6):804-805.
0 notes
Text
Metformin shown for the first time to activate Telomere enzyme Telomerase in human cells via AMPK: Link between Progeria and HIV

Goldsmith Content Providers: CDC/ C. Goldsmith, P. Feorino, E. L. Palmer, W. R. McManus [Public domain], via Wikimedia Commons;The Cell Nucleus and Aging: Tantalizing Clues and Hopeful Promises. Scaffidi P, Gordon L, Misteli T. PLoS Biology Vol. 3/11/2005, e39
A recently published study in the journal Biochimica et Biophysica Acta (BBA) - Molecular Basis of Disease in 2018 demonstrated for the first time that chronic treatment with the anti-diabetic drug metformin activated human telomerase reverse transcriptase (hTERT) in human aortic endothelial cells (HAECs) and significantly delayed endothelial senescence in an AMPK-dependent manner [1]. AMPK is activated by the induction of cellular stress, mediated by increases in intracellular calcium (Ca2+), reactive oxygen species (ROS), and/or an AMP(ADP)/ATP ratio increase, etc. [41]. Telomeres are specialized regions of repetitive nucleotide sequences located at the ends of eukaryotic chromosomes that protect chromosomal ends from deterioration [2]. However, continuous cell division leads to telomere shortening, impeding the replenishment of tissues and triggering cellular senescence (i.e. cells cease to divide). Although human telomeres shorten with age, telomeres may be lengthened by the enzyme telomerase, a ribonucleoprotein that consists of the catalytic subunit hTERT, telomerase RNA, and the nucleolar protein dyskerin [2,3]. hTERT, which is considering limiting for telomerase activity, is a protein that exhibits reverse transcriptase activity and synthesizes telomeric DNA from an RNA template [4].
The authors of the study initially demonstrated that both metformin and the AMPK activator AICAR significantly increased hTERT levels and enhanced AMPK activation in human aortic endothelial cells (HAECs) [1]. Importantly, inhibition or knockdown of AMPK with compound C or siAMPKα inhibited the metformin-induced increase in hTERT while metformin failed to reverse siAMPKα-induced senescence in HAECs, indicating that hTERT expression is regulated by AMPK activation in endothelial cells. Indeed, continuous culturing of HAECs in the presence of metformin significantly increased hTERT protein levels and activity, reduced the expression of the senescence markers p53, p21, p27, and p16, and reduced senescence-associated beta-galactosidase (SA-β-gal, a biomarker of cellular senescence) staining in HAECs, again indicating that metformin delays cellular senescence and increases hTERT levels via AMPK activation [1]. Strikingly, using ApoE-/- mice (which spontaneously develop atherosclerosis and age faster compared to normal mice), the authors also showed that chronic low-dose metformin administration for fourteen months in drinking water enhanced the levels of activated AMPK and Pgc-1α observed in the endothelial layer of the aorta [1]. Metformin also increased the transcript and protein levels of Tert, decreased senescence markers (p16, p21, p27, p53) in the total aortic homogenate, and significantly reduced SA-β-gal staining of aorta compared to untreated ApoE-/- mice, demonstrating that metformin-induced AMPK activation delays vascular aging and protects from age-associated atherosclerosis in ApoE-/- mice [1].
Interestingly, telomere length has been shown to be significantly reduced in cells derived from patients with the accelerated aging disorder Hutchinson-Gilford progeria syndrome (HGPS) and telomere shortening in normal cells that occurs during cellular senescence activates progerin production, a toxic protein that leads to an accelerating aging phenotype in children with HGPS via aberrant alternative splicing of the LMNA gene [5]. Normal lamin A plays a critical role in supporting nuclear architecture and morphology. Lamin A binding to subtelomeric repeats also localizes telomeres to the nuclear periphery and loss of lamin A leads to defects in telomeric heterochromatin, altered nuclear distribution and shortening of telomeres, inefficient processing of dysfunctional telomeres by non-homologus end joining, and increased genomic instability [6]. Telomere length has been found to be significantly reduced in fibroblasts derived from HGPS patients and a recent study also confirmed that in normal human fibroblasts, progressive telomere damage that occurs during cellular senescence activates progerin production and also leads to extensive changes in alternative splicing of many other genes, highlighting a striking similarity between normal aging and accelerated aging in HGPS patients [5,7]. Indeed, transfection of HGPS fibroblasts with human telomerase (hTERT) mRNA restored cell proliferation, reduced cell loss, extended cellular lifespan, increased telomerase activity and telomere length, and reduced SA-β-gal staining compared to HGPS cells expressing catalytically inactive hTERT mRNA [8]. Because metformin also increases hTERT expression and inhibits senescence in human cells, it is likely that cellular stress-induced AMPK activation, mediated by increases in intracellular calcium (Ca2+), reactive oxygen species (ROS), and/or an AMP(ADP)/ATP ratio increase, etc., represents a central node linking structurally diverse compounds and methodologies that alleviate accelerated aging in HGPS cells.
As the splicing factor SRSF1 has been shown to increase progerin production by promoting the use of a cryptic splice located in the LMNA gene, metformin was recently shown to significantly reduce the expression of SRSF1 and progerin, activate AMPK, and improve nuclear architecture in HGPS cells, indicating that AMPK activation by metformin beneficially alters gene splicing in HGPS cells by modulating SRSF1, a hypothesis that I first proposed and published in 2014 [9-11]. Also, p32, a splicing-associated protein that is an endogenous inhibitor of SRSF1 and is critical for the maintenance of mitochondrial functionality and oxidative phosphorylation, has also been shown to be essential for rapamycin- or starvation-induced autophagy mediated by ULK1 [12,13]. Because rapamycin, also an AMPK activator in vivo, improves accelerated aging defects in HGPS cells by reducing progerin levels via induction of autophagy, AMPK activation likely also beneficially modulates the activity of p32, leading to inhibition of SRSF1 splicing activity and enhancement of mitochondrial functionality [14,15]. Moreover, PGC-1α, which is activated by AMPK and metformin and promotes telomere transcription, is downregulated in HGPS cells, leading to significant mitochondrial dysfunction [1,16,17]. Methylene blue, which activates AMPK in vivo, was shown to increase PGC-1α levels, induce progerin solubility, and alleviate accelerated aging defects in HGPS cells [16,18]. Additionally, the rapamycin analog temsirolimus alleviated accelerated aging defects in HGPS cells but transiently increased ROS and superoxide anion levels in both HGPS and normal cells within the first hour of treatment, again indicating that cellular stress-induced AMPK activation represents a common mechanism for inhibiting senescence and ameliorating symptoms associated with accelerated aging [19].
The inhibition of SRSF1 and the promotion of hTERT expression and telomere transcription by metformin via AMPK activation also link HGPS and telomere integrity with HIV-1 latency. Increased splicing activity of SRSF1 inhibits reactivation of latent HIV-1 residing in infected immune cells, preventing immune system detection and destruction of the virus [20]. Reactivation of latent HIV-1 (i.e. the “shock and kill” approach) leads to a reduction in SRSF1 but an increase in p32 activity and bryostatin-1 (a PKC modulator) has been shown to reactivate latent HIV-1 via AMPK activation [20,21]. Interestingly, p32 modulation via AMPK activation may also enhance and stabilize the splicing activities of hnRNPA1, a heteroribonuclear protein that associates with p32, antagonizes the splicing function of SRSF1, prevents splicing of the HIV-1 genome (promoting viral reactivation), and participates in the maintenance and preservation of telomeres [22-25]. hnRNPA1 is also decreased in senescent human fibroblasts and antagonizes cellular senescence and the senescence-associated secretory phenotype (SASP) via increasing SIRT1 expression [26,27]. Resveratrol, a plant-derived polyphenol that activates AMPK, increases hnRNPA1 protein expression and SIRT1, a histone deacetylase that plays a role in a number of age related diseases and in the extension of lifespan, is also activated by AMPK [28-30]. Also, T cell activation, an efficient method for reactivating latent HIV-1, is dependent on increases in intracellular Ca2+ and ROS, telomerase is transiently increased on T cell activation, and AMPK knockdown leads to T cell death during in vitro activation [31-34]. Furthermore, resveratrol reactivates latent HIV-1 and preliminary data demonstrated that metformin decreased the percentage of CD4+ T cells expressing PD-1, TIGIT, and TIM-3, each markers associated with T cells latently infected with HIV-1, in chronically-infected HIV-1 patients [35-37]. Such evidence strongly suggests that cellular stress-induced AMPK activation, mediated by increases in intracellular calcium (Ca2+), reactive oxygen species (ROS), and/or an AMP (ADP)/ATP ratio increase, etc. links the alleviation of accelerated cellular aging defects in HGPS with the potential eradication of HIV-1, a hypothesis that I first proposed in 2015 [38].
The evidence presented in the Biochimica et Biophysica Acta (BBA) - Molecular Basis of Disease publication further substantiates that AMPK activation represents a central node that connects the therapeutic benefits of chemically distinct compounds in diseases as seemingly dissimilar as HGPS and HIV-1 latency. Indeed, AMPK activators including metformin increase hTERT expression, promote telomere transcription and integrity, decrease the splicing activity of SRSF1 that increases progerin production but prevents latent HIV-1 reactivation, and potentially beneficially modulates the activity of the SRSF1 inhibitor p32 and the ribonucleoprotein hnRNPA1. As AMPK activators (e.g. ionomycin) induce human oocyte activation (giving rise to normal healthy children) and AMPK is localized throughout the entire acrosome in human sperm (likely promoting the acrosome reaction), AMPK activation links normal human aging, Progeria, and HIV-1 latency with the creation of all human life (i.e. the “shock and live” approach) [11,38-40].
https://www.linkedin.com/pulse/metformin-shown-first-time-activate-telomere-enzyme-human-finley/?published=t
References:
Karnewar S, Neeli PK, Panuganti D, et al. Metformin regulates mitochondrial biogenesis and senescence through AMPK mediated H3K79 methylation: Relevance in age-associated vascular dysfunction. Biochim Biophys Acta. 2018 Apr;1864(4 Pt A):1115-1128.
O'Sullivan RJ, Karlseder J. Telomeres: protecting chromosomes against genome instability. Nat Rev Mol Cell Biol. 2010 Mar;11(3):171-81.
Cohen SB, Graham ME, Lovrecz GO, Bache N, Robinson PJ, Reddel RR. Protein composition of catalytically active human telomerase from immortal cells. Science. 2007 Mar 30;315(5820):1850-3.
Cong YS, Wen J, Bacchetti S. The human telomerase catalytic subunit hTERT: organization of the gene and characterization of the promoter. Hum Mol Genet. 1999 Jan;8(1):137-42.
Cao K, Blair CD, Faddah DA, et al. Progerin and telomere dysfunction collaborate to trigger cellular senescence in normal human fibroblasts. J Clin Invest. 2011 Jul;121(7):2833-44.
Gonzalez-Suarez I, Redwood AB, Perkins SM, et al. Novel roles for A-type lamins in telomere biology and the DNA damage response pathway. EMBO J 2009;28(16):2414–27.
Decker ML, Chavez E, Vulto I, Lansdorp PM. Telomere length in Hutchinson-Gilford progeria syndrome. Mech Ageing Dev. 2009 Jun;130(6):377-83.
Li Y, Zhou G, Bruno IG, Cooke JP. Telomerase mRNA Reverses Senescence in Progeria Cells. J Am Coll Cardiol. 2017 Aug 8;70(6):804-805.
Egesipe AL, Blondel S1, Cicero AL, et al. Metformin decreases progerin expression and alleviates pathological defects of Hutchinson-Gilford progeria syndrome cells. NPJ Aging Mech Dis. 2016 Nov 10;2:16026.
Park SK, Shin OS. Metformin alleviates ageing cellular phenotypes in Hutchinson-Gilford progeria syndrome dermal fibroblasts. Exp Dermatol. 2017 Oct;26(10):889-895.
Finley J. Alteration of splice site selection in the LMNA gene and inhibition of progerin production via AMPK activation. Med Hypotheses. 2014 Nov;83(5):580-7.
P32/gC1qR is indispensable for fetal development and mitochondrial translation: importance of its RNA-binding ability. Nucl Acids Res 2012;40(19):9717–37.
Jiao H, Su GQ, Dong W, et al. Chaperone-like protein p32 regulates ULK1 stability and autophagy. Cell Death Differ. 2015 Nov;22(11):1812-23.
Cao K, Graziotto JJ, Blair CD, et al. Rapamycin reverses cellular phenotypes and enhances mutant protein clearance in Hutchinson-Gilford progeria syndrome cells. Sci Transl Med. 2011 Jun 29;3(89):89ra58.
Chiao YA, Kolwicz SC, Basisty N, et al. Rapamycin transiently induces mitochondrial remodeling to reprogram energy metabolism in old hearts. Aging (Albany NY). 2016 Feb;8(2):314-27.
Xiong ZM, Choi JY, Wang K, et al. Methylene blue alleviates nuclear and mitochondrial abnormalities in progeria. Aging Cell. 2016 Apr;15(2):279-90.
Diman A, Boros J, Poulain F, et al. Nuclear respiratory factor 1 and endurance exercise promote human telomere transcription. Sci Adv. 2016 Jul 27;2(7):e1600031.
Xie L, Li W, Winters A, Yuan F, Jin K, Yang S. Methylene blue induces macroautophagy through 5' adenosine monophosphate-activated protein kinase pathway to protect neurons from serum deprivation. Front Cell Neurosci. 2013 May 3;7:56.
Gabriel D, Gordon LB, Djabali K. Temsirolimus Partially Rescues the Hutchinson-Gilford Progeria Cellular Phenotype. PLoS One. 2016 Dec 29;11(12):e0168988.
Berro R, Kehn K, de la Fuente C, et al. Acetylated Tat regulates human immunodeficiency virus type 1 splicing through its interaction with the splicing regulator p32. J Virol. 2006 Apr;80(7):3189-204.
Mehla R, Bivalkar-Mehla S, Zhang R, et al. Bryostatin modulates latent HIV-1 infection via PKC and AMPK signaling but inhibits acute infection in a receptor independent manner. PLoS One. 2010 Jun 16;5(6):e11160.
Expert-Bezançon A, Sureau A, Durosay P, et al. HnRNP A1 and the SR proteins ASF/SF2 and SC35 have antagonistic functions in splicing of beta tropomyosin exon 6B. J Biol Chem 2004 Sep 10;279(37):38249–59.
LaBranche H, Dupuis S, Ben-David Y, Bani MR, Wellinger RJ, Chabot B. Telomere elongation by hnRNP A1 and a derivative that interacts with telomeric repeats and telomerase. Nat Genet 1998 Jun;19(2):199–202.
Petersen-Mahrt SK, Estmer C, Ohrmalm C, Matthews DA, Russell WC, Akusjärvi G. The splicing factor-associated protein, p32, regulates RNA splicing by inhibiting ASF/SF2 RNA binding and phosphorylation. EMBO J 1999;18(4):1014–24.
Caputi M, Mayeda A, Krainer AR, Zahler AM. hnRNP A/B proteins are required for inhibition of HIV-1 pre-mRNA splicing. EMBO J. 1999 Jul 15;18(14):4060-7.
Zhu D1, Xu G, Ghandhi S, Hubbard K. Modulation of the expression of p16INK4a and p14ARF by hnRNP A1 and A2 RNA binding proteins: implications for cellular senescence. J Cell Physiol. 2002 Oct;193(1):19-25.
Wang H, Han L, Zhao G. hnRNP A1 antagonizes cellular senescence and senescence-associated secretory phenotype via regulation of SIRT1 mRNA stability. Aging Cell. 2016 Sep 9. doi: 10.1111/acel.12511. [Epub ahead of print].
Cantó C, Gerhart-Hines Z, Feige JN, et al. AMPK regulates energy expenditure by modulating NAD+ metabolism and SIRT1 activity. Nature. 2009 Apr 23;458(7241):1056-60.
Moshiri A, Puppo M, Rossi M, Gherzi R, Briata P. Resveratrol limits epithelial to mesenchymal transition through modulation of KHSRP/hnRNPA1-dependent alternative splicing in mammary gland cells. Biochim Biophys Acta. 2017 Mar;1860(3):291-298.
Chiang MC, Nicol CJ, Cheng YC. Resveratrol activation of AMPK-dependent pathways is neuroprotective in human neural stem cells against amyloid-beta-induced inflammation and oxidative stress. Neurochem Int. 2017 Oct 5. pii: S0197-0186(17)30362-5.
Dahabieh MS, Battivelli E, Verdin E. Understanding HIV latency: the road to an HIV cure. Annu Rev Med. 2015;66:407-21.
Sena LA, Li S, Jairaman A, et al. Mitochondria are required for antigen-specific T cell activation through reactive oxygen species signaling. Immunity. 2013 Feb 21;38(2):225-36.
Weng NP, Levine BL, June CH, Hodes RJ. Regulated expression of telomerase activity in human T lymphocyte development and activation. J Exp Med. 1996 Jun 1;183(6):2471-9.
Rao E, Zhang Y, Zhu G, et al. Deficiency of AMPK in CD8+ T cells suppresses their anti-tumor function by inducing protein phosphatase-mediated cell death. Oncotarget. 2015 Apr 10;6(10):7944-58.
Zeng X, Pan X, Xu X, et al. Resveratrol Reactivates Latent HIV through Increasing Histone Acetylation and Activating Heat Shock Factor 1. J Agric Food Chem. 2017 Jun 7;65(22):4384-4394.
G.M. Chew, D.C. Chow, S.A. Souza, et al. Impact of adjunctive metformin therapy on T cell exhaustion and viral persistence in a clinical trial of HIV-infected adults on suppressive ART. Journal of Virus Eradication 2017; 3 (Supplement 1): 6–19.
http://viruseradication.com/abstract-details.php?abstract_id=1188, last accessed March 14, 2018.
Finley J. Reactivation of latently infected HIV-1 viral reservoirs and correction of aberrant alternative splicing in the LMNA gene via AMPK activation: Common mechanism of action linking HIV-1 latency and Hutchinson-Gilford progeria syndrome. Med Hypotheses. 2015 Sep;85(3):320-32.
Finley J. Oocyte activation and latent HIV-1 reactivation: AMPK as a common mechanism of action linking the beginnings of life and the potential eradication of HIV-1. Med Hypotheses. 2016 Aug;93:34-47.
Calle-Guisado V, de Llera AH, Martin-Hidalgo D, et al. AMP-activated kinase in human spermatozoa: identification, intracellular localization, and key function in the regulation of sperm motility. Asian J Androl. 2017 Nov-Dec;19(6):707-714.
Auciello FR, Ross FA, Ikematsu N, Hardie DG. Oxidative stress activates AMPK in cultured cells primarily by increasing cellular AMP and/or ADP. FEBS Lett. 2014 Sep 17;588(18):3361-6.
0 notes
Text
Metformin shown for the first time to inhibit Legionella infection via AMPK : AMPK links pathogen destruction with Progeria & Human Life Creation

CC-BY-SA-3.0 http://creativecommons.org/licenses/by-sa/3.0/)], via Wikimedia Commons; By CDC/ Dr. Barry S. Fields [Public domain], via Wikimedia Commons
In line with recent evidence demonstrating that the AMPK activator metformin inhibits Zika virus, Dengue virus, and malaria parasite replication (see below), a study published in The Journal of Immunology in December of 2017 showed for the first time that the anti-diabetic drug metformin significantly suppressed the growth of the bacterium Legionella pneumophila (L. pneumophila) in immune cells derived from both mice and humans by activating AMPK [1]. L. pneumophila is a Gram-negative bacterium that is typically found in water-associated environments and may contaminate hot water tanks and air-conditioning units for large buildings and is the causative agent of Legionnaires' disease [1,2]. Legionnaires' disease is a form of atypical pneumonia characterized by fever, cough, and shortness of breath and is usually acquired by inhalation of small air-borne water droplets [2]. According to the Centers for Disease Control and Prevention (CDC), “the bacterium is named after a 1976 outbreak, during which some people who went to a Philadelphia convention of the American Legion suffered from a new type of pneumonia (lung infection) that became known as Legionnaires’ disease.” [3]. Interestingly, the authors of the study observed that metformin increased the production of mitochondrial reactive oxygen species (ROS) in L. pneumophila-infected immune cells and that inhibition of both AMPK activation and ROS production negated metformin-mediated growth suppression of L. pneumophila [1]. Most importantly, metformin significantly reduced bacterial number, activated AMPK, and increased ROS in the lungs of infected mice, thus improving survival and indicating that metformin inhibits L. pneumophila replication in vivo in an AMPK-dependent manner. Metformin also increased mitochondrial ROS in uninfected immune cells, suggesting that cellular stress induced AMPK activation (via increases in ROS, calcium[Ca2+], and/or an AMP/ATP ratio increase, etc) is critical for mounting an effective immune response to bacteria, viruses, and other pathogens [1].
Strikingly, metformin activates AMPK and alleviates accelerating aging defects in cells from children with the genetic disorder Hutchinson-Gilford progeria syndrome (HGPS), promotes the differentiation and/or apoptosis of cancer stem cells in an AMPK-dependent manner, and destabilizes the latent HIV-1 reservoir in chronically-infected HIV-1 patients (facilitating virus elimination and potentially contributing to an HIV-1 cure). Also, because AMPK is critical for oocyte maturation and bacteria-derived antibiotics (e.g. ionomycin, A23187) that activate AMPK are used extensively to activate human oocytes to create normal, healthy babies, it is likely that stress-induced AMPK activation (e.g. via ROS, intracellular Ca2+, and/or AMP/ATP ratio increase, etc.) represents a common mechanism linking pathogen elimination with HGPS, caner stem cell elimination, HIV-1 eradication, and the creation of all human life, as I originally proposed in several recent publications (see below) [4-7].
As noted above, in addition to inhibition of L. pneumophila replication, metformin also potently inhibited ZIKV replication in HUVECs and AMPK activation has recently been found to exert significant antiviral effects against Rift Valley Fever virus as well as multiple arbovirus family members including the Flavivirus Kunjin virus, the Togavirus Sindbis virus, and the Rhabdovirus Vesicular stomatitis virus [8,9]. Interestingly, as both ZIKV and dengue virus (DENV) are transmitted by the same mosquito vector, a study recently published in the journal PLoS Pathogens in April of 2017 demonstrated for the first time that metformin exerted significant antiviral effects in DENV-infected human liver cells that was dependent on activation of AMPK [10]. The authors showed that an increase in HMG-CoA reductase (HMGCR) activity, a target of AMPK, was associated with DENV-infected cells, AMPK activation was reduced in DENV-infected cells at 12 and 24 hours post infection (hpi), and metformin significantly decreased the number of infected cells, viral yield, and viral genome copies, leading the authors to conclude that metformin-induced AMPK activation generates a strong antiviral effect against DENV [10].
Recent efforts funded by the U.S. and British governments, the Bill & Melinda Gates Foundation, and the Google health spin-off Verily have sought to decrease the spread of dengue and Zika viruses through the coordinated release of female and/or male mosquitoes (called Aedes aegypti) that were purposely infected with a bacterium that inhibits the mosquito’s ability to transmit the two viruses to humans [11,12]. Studies have shown that this bacterium, called Wolbachia, enhances the mosquito’s immune response by increasing the levels of reactive oxygen species (ROS), thus enhancing inhibition of DENV replication [13]. Because AMPK is activated by cellular stress (e.g. ROS increase, intracellular calcium [Ca2+] increase, AMP/ATP ratio increase, etc.), has been found in Aedes aegypti (Ae. aegypti), and AMPK activation by stress-inducing compounds (e.g. resveratrol) increased average life span and enhanced the immune response in Ae. aegypti in an AMPK-dependent manner, the recent finding that metformin also inhibits DENV replication in human cells in an AMPK-dependent manner provides compelling evidence that the anti-viral and antimicrobial effects of AMPK activation likely crosses species boundaries [14].
Moreover, a study published in the journal Cell Reports in September of 2016 by researchers from the Massachusetts Institute of Technology (MIT) and the University of Lisbon also showed for the first time that metformin and other AMPK activators significantly reduced parasite load in human liver cells of different species of Plasmodium, a protozoan parasite that is the etiological agent of malaria [15]. Importantly, the authors also showed that AMPK activation inhibits growth and replication of different Plasmodium spp. (species) and AMPK activators as well as dietary restriction, which activates AMPK, reduces Plasmodium berghei (malaria-causing species in rodents often used as a model for the study of human malaria) infection in mice [15]. The AMPK-activating compounds salicylate and A769662 also reduced P. berghei and P. falciparum (malaria parasite that infects humans) merozoite formation (infectious parasites generated through replication in erythrocytes) in vitro while salicylate decreased parasitaemia in mice in vivo [16].
Cellular stress-induced AMPK activation has also been shown to exert antiviral effects against HIV-1. AMPK activation and several AMPK-activating compounds, including EGCG, curcumin, tanshinone II A (derived from the plant Salvia miltiorrhiza), bryostatin-1 (isolated from the marine organism Bugula neritina), and resveratrol (found in grapes and in the plant Polygonum cuspidatum) have been shown to exhibit antiviral activity in vitro against HIV-1 [17-21].
An active area among HIV-1 cure researchers, known as the “shock and kill” approach, involves reactivating (i.e. “shock”) a T cell (or another immune cell) that harbors dormant HIV-1, hence reactivating the virus itself and thus inducing destruction of the T cell along with the virus or enhancing recognition and destruction of the virus-infected T cell by the immune system (i.e. “kill”) [5]. Strikingly, AMPK is also critical for the activation of T cells and the mounting of an effective immune response to eliminate viruses, bacteria, and cancer cells [6,22,23]. A recent study demonstrated that metformin, when combined with the protein kinase C modulator bryostatin, induced reactivation of latent HIV-1 in a monocytic cell line in an AMPK-dependent manner. Bryostatin was also shown to induce phosphorylation and activation of AMPK in that study, implying that bryostatin is an indirect AMPK activator as well [20]. Furthermore, the calcium ionophores ionomycin and A23187, both of which activate AMPK and induce human oocyte activation, are often combined with phorbol 12-myristate 13-acetate (PMA) and are extremely efficient in promoting T cell activation-induced latent HIV-1 reactivation [24-26].
Perhaps most convincingly, at the International AIDS Society’s (IAS) HIV Cure and Cancer Forum held in Paris, France in July of 2017, researchers from the University of Hawaii demonstrated for the first time that metformin decreased the percentage of CD4+ T cells expressing the immune checkpoint receptors PD-1, TIGIT, and TIM-3 in HIV-1 patients, receptors that are positively associated with T cells that harbor latent HIV-1. Metformin also destabilized the latent viral reservoir in chronically-infected HIV-1 patients, indicating that metformin may indeed contribute to HIV-1 eradication by inducing an AMPK-mediated reactivation of latent HIV-1, as I initially proposed in 2015 and 2016 [5,6,27-31].
Also, stress-induced AMPK activation likely links latent HIV-1 reactivation with alleviation of accelerated aging defects in cells derived from children with the genetic disorder Hutchinson-Gilford progeria syndrome (HGPS). Studies have shown that efficient reactivation of latent HIV-1 involves a reduction in the splicing of the HIV-1 genome by the gene splicing factor SRSF1 [32-34]. Accelerated cellular aging-like phenotypes in HGPS are primarily linked to aberrant splicing of the LMNA gene, leading to the over production of a toxic protein called progerin [4]. Evidence has also shown that inhibition of the splicing factor SRSF1 leads to a reduction in progerin at both the mRNA and protein levels [5,35].
A recent study published online in the Journal npj Aging and Mechanisms of Disease in November of 2016 provided startling evidence that metformin decreased the expression of progerin and SRSF1 and alleviated pathological defects in cells derived from HGPS patients [36]. Another study published online in the Journal Experimental Dermatology in February of 2017 confirmed that metformin alleviated nuclear defects and premature aging phenotypes and activated AMPK in fibroblasts derived from HGPS patients, substantiating my original hypotheses from 2014 and 2015 proposing that AMPK activators including metformin would improve accelerated aging defects in HGPS cells by inhibiting SRSF1 and activating AMPK [4,5,37]. Temsirolimus, an analog of the macrolide rapamycin (which activates AMPK in vivo), also partially rescued the HGPS cellular phenotype but significantly increased the levels of ROS and superoxide within the first hour of treatment, providing further indication that the induction of cellular stress and subsequent AMPK activation links virus and pathogen elimination with alleviation of accelerated cellular aging defects in HGPS [38,39].
Furthermore, ROS and calcium are well-studied mediators of cellular stress-induced differentiation of embryonic and adult stem cells, AMPK has recently been shown to be essential for mouse embryonic stem cell differentiation, and metformin targets and promotes differentiation and/or apoptosis of cancer stem cells in the deadliest of cancers in an AMPK-dependent manner, including glioblastoma and pancreatic cancer [7]. Such evidence strongly suggests that cellular stress-induced AMPK activation by compounds including metformin links pathogen and virus elimination with HGPS and cancer stem cell differentiation and/or apoptosis, a hypothesis that I first proposed in 2017 [7].
Lastly, AMPK activation also promotes oocyte meiotic induction and maturation (processes that are critical for efficient oocyte activation) and AMPK has recently been found localized across the entire acrosome in human spermatozoa [6,40,41]. The induction of cellular stress (e.g. increases in ROS, intracellular calcium, and/or AMP/ATP ratio increase), which activates AMPK, also promotes oocyte meiotic induction/maturation, oocyte activation, and the acrosome reaction in human sperm, processes critical for the creation of all human life [40,42,43]. Indeed, oocyte activation is indispensable for the creation of all human life and the bacteria-derived calcium ionophore ionomycin, which activates AMPK, is commonly used to promote latent HIV-1 reactivation and is extensively used to activate human oocytes, creating normal healthy children (i.e. the “shock and live” approach) [43-47]. Such evidence indicates and further substantiates the novel and provocative assertion that AMPK activation links L. pneumophila inhibition and pathogen elimination with the amelioration of accelerated aging defects in HGPS cells, HIV-1 latency and replication, adult and cancer stem cells, and the creation of all human life [1,4-7].
https://www.linkedin.com/pulse/metformin-shown-first-time-inhibit-legionella-infection-finley/

References:
Kajiwara C, Kusaka Y, Kimura S, et al. Metformin Mediates Protection against Legionella Pneumonia through Activation of AMPK and Mitochondrial Reactive Oxygen Species. J Immunol. 2017 Dec 15. pii: ji1700474. doi: 10.4049/jimmunol.1700474. [Epub ahead of print].
https://www.cdc.gov/legionella/about/causes-transmission.html
https://www.cdc.gov/legionella/index.html
Finley J. Alteration of splice site selection in the LMNA gene and inhibition of progerin production via AMPK activation. Med Hypotheses. 2014 Nov;83(5):580-7.
Finley J. Reactivation of latently infected HIV-1 viral reservoirs and correction of aberrant alternative splicing in the LMNA gene via AMPK activation: Common mechanism of action linking HIV-1 latency and Hutchinson-Gilford progeria syndrome. Med Hypotheses. 2015 Sep;85(3):320-32.
Finley J. Oocyte activation and latent HIV-1 reactivation: AMPK as a common mechanism of action linking the beginnings of life and the potential eradication of HIV-1. Med Hypotheses. 2016 Aug;93:34-47.
Finley J. Elimination of cancer stem cells and reactivation of latent HIV-1 via AMPK activation: Common mechanism of action linking inhibition of tumorigenesis and the potential eradication of HIV-1. Med Hypotheses. 2017 Jul;104:133-146.
Cheng F, Ramos da Silva S, Huang IC, Jung JU, Gao SJ. Suppression of Zika virus infection and replication in endothelial cells and astrocytes by PKA inhibitor PKI 14-22. J Virol. 2017 Dec 6. pii: JVI.02019-17. doi: 10.1128/JVI.02019-17. [Epub ahead of print].
Moser TS, Schieffer D, Cherry S. AMP-activated kinase restricts Rift Valley fever virus infection by inhibiting fatty acid synthesis. PLoS Pathog. 2012;8(4):e1002661.
Soto-Acosta R, Bautista-Carbajal P, Cervantes-Salazar M, Angel-Ambrocio AH, Del Angel RM. DENV up-regulates the HMG-CoA reductase activity through the impairment of AMPK phosphorylation: A potential antiviral target. PLoS Pathog. 2017 Apr 6;13(4):e1006257.
http://www.reuters.com/article/us-health-dengue-mosquitoes-idUSKCN12Q1PE
https://www.bloomberg.com/news/articles/2016-10-06/alphabet-s-verily-joins-zika-fight-with-sterile-mosquito-lab
Pan X, Zhou G, Wu J, et al. Wolbachia induces reactive oxygen species (ROS)-dependent activation of the Toll pathway to control dengue virus in the mosquito Aedes aegypti. Proc Natl Acad Sci U S A. 2012 Jan 3;109(1):E23-31.
Nunes RD, Ventura-Martins G, Moretti DM, et al. Polyphenol-Rich Diets Exacerbate AMPK-Mediated Autophagy, Decreasing Proliferation of Mosquito Midgut Microbiota, and Extending Vector Lifespan. PLoS Negl Trop Dis. 2016 Oct 12;10(10):e0005034.
Ruivo MT, Vera IM, Sales-Dias J, et al. Host AMPK Is a Modulator of Plasmodium Liver Infection. Cell Rep. 2016 Sep 6;16(10):2539-45.
Mancio-Silva L, Slavic K, Grilo Ruivo MT, et al. Nutrient sensing modulates malaria parasite virulence. Nature. 2017 Jul 13;547(7662):213-216.
Zhang HS, Wu TC, Sang WW, Ruan Z. EGCG inhibits Tat-induced LTR transactivation: role of Nrf2, AKT, AMPK signaling pathway. Life Sci. 2012 May 22;90(19-20):747-54.
Zhang HS, Ruan Z, Sang WW. HDAC1/NFκB pathway is involved in curcumin inhibiting of Tat-mediated long terminal repeat transactivation. J Cell Physiol. 2011 Dec;226(12):3385-91.
Zhang HS, Chen XY, Wu TC, Zhang FJ. Tanshinone II A inhibits tat-induced HIV-1 transactivation through redox-regulated AMPK/Nampt pathway. J Cell Physiol. 2014 Sep;229(9):1193-201.
Mehla R, Bivalkar-Mehla S, Zhang R, et al. Bryostatin modulates latent HIV-1 infection via PKC and AMPK signaling but inhibits acute infection in a receptor independent manner. PLoS One. 2010 Jun 16;5(6):e11160.
Zhang HS, Wu MR. SIRT1 regulates Tat-induced HIV-1 transactivation through activating AMP-activated protein kinase. Virus Res. 2009 Dec;146(1-2):51-7.
Pearce EL, Walsh MC, Cejas PJ, et al. Enhancing CD8 T-cell memory by modulating fatty acid metabolism. Nature. 2009 Jul 2;460(7251):103-7.
Blagih J, Coulombe F, Vincent EE, et al. The energy sensor AMPK regulates T cell metabolic adaptation and effector responses in vivo. Immunity. 2015 Jan 20;42(1):41-54.
Gómez-Gonzalo M, Carretero M, Rullas J et al. The hepatitis B virus X protein induces HIV-1 replication and transcription in synergy with T-cell activation signals: functional roles of NF-kappaB/NF-AT and SP1-binding sites in the HIV-1 long terminal repeat promoter. J Biol Chem. 2001 Sep 21;276(38):35435-43.
Tamás P, Hawley SA, Clarke RG, et al. Regulation of the energy sensor AMP-activated protein kinase by antigen receptor and Ca2+ in T lymphocytes. J Exp Med. 2006 Jul 10;203(7):1665-70.
Fogarty S, Hawley SA, Green KA, Saner N, Mustard KJ, Hardie DG. Calmodulin-dependent protein kinase kinase-beta activates AMPK without forming a stable complex: synergistic effects of Ca2+ and AMP. Biochem J. 2010 Jan 27;426(1):109-18.
G.M. Chew, D.C. Chow, S.A. Souza, et al. Impact of adjunctive metformin therapy on T cell exhaustion and viral persistence in a clinical trial of HIV-infected adults on suppressive ART. Journal of Virus Eradication 2017; 3 (Supplement 1): 6–19.
http://viruseradication.com/supplement-details/Abstracts_of_the_IAS_HIV_Cure_and_Cancer_Forum_2017/
http://www.iasociety.org/HIV-Programmes/Towards-an-HIV-Cure/Events/HIV-Cure-Cancer-Forum
Fromentin R, Bakeman W, Lawani MB, et al. CD4+ T Cells Expressing PD-1, TIGIT and LAG-3 Contribute to HIV Persistence during ART. PLoS Pathog. 2016 Jul 14;12(7):e1005761.
Chew GM, Fujita T, Webb GM, et al. TIGIT Marks Exhausted T Cells, Correlates with Disease Progression, and Serves as a Target for Immune Restoration in HIV and SIV Infection. PLoS Pathog. 2016 Jan 7;12(1):e1005349.
Berro R, Kehn K, de la Fuente C, et al. Acetylated Tat regulates human immunodeficiency virus type 1 splicing through its interaction with the splicing regulator p32. J Virol. 2006 Apr;80(7):3189-204.
Spina CA, Anderson J, Archin NM, et al. An in-depth comparison of latent HIV-1 reactivation in multiple cell model systems and resting CD4+ T cells from aviremic patients. PLoS Pathog. 2013;9(12):e1003834.
Hu M, Crawford SA, Henstridge DC, et al. p32 protein levels are integral to mitochondrial and endoplasmic reticulum morphology, cell metabolism and survival. Biochem J. 2013 Aug 1;453(3):381-91.
Lopez-Mejia IC, Vautrot V, De Toledo M, et al. A conserved splicing mechanism of the LMNA gene controls premature aging. Hum Mol Genet. 2011 Dec 1;20(23):4540-55.
Egesipe AL, Blondel S, Cicero AL, et al. Metformin decreases progerin expression and alleviates pathological defects of Hutchinson-Gilford progeria syndrome cells. NPJ Aging Mech Dis. 2016 Nov 10;2:16026.
Park SK, Shin OS. Metformin Alleviates Ageing Cellular Phenotypes in Hutchinson-Gilford Progeria Syndrome Dermal Fibroblasts. Exp Dermatol. 2017 Oct;26(10):889-895.
Gabriel D, Gordon LB, Djabali K. Temsirolimus Partially Rescues the Hutchinson-Gilford Progeria Cellular Phenotype. PLoS One. 2016 Dec 29;11(12):e0168988.
Chiao YA, Kolwicz SC, Basisty N, et al. Rapamycin transiently induces mitochondrial remodeling to reprogram energy metabolism in old hearts. Aging (Albany NY). 2016 Feb;8(2):314-27.
LaRosa C, Downs SM. Stress stimulates AMP-activated protein kinase and meiotic resumption in mouse oocytes. Biol Reprod. 2006 Mar;74(3):585-92.
Calle-Guisado V, de Llera AH, Martin-Hidalgo D, et al. AMP-activated kinase in human spermatozoa: identification, intracellular localization, and key function in the regulation of sperm motility. Asian J Androl. 2016 Sep 27. doi: 10.4103/1008-682X.185848. [Epub ahead of print].
de Lamirande E, Tsai C, Harakat A, Gagnon C. Involvement of reactive oxygen species in human sperm arcosome reaction induced by A23187, lysophosphatidylcholine, and biological fluid ultrafiltrates. J Androl. 1998 Sep-Oct;19(5):585-94.
Tesarik J, Sousa M, Testart J. Human oocyte activation after intracytoplasmic sperm injection. Hum Reprod. 1994 Mar;9(3):511-8.
Deemeh MR, Tavalaee M, Nasr-Esfahani MH. Health of children born through artificial oocyte activation: a pilot study. Reprod Sci. 2015 Mar;22(3):322-8.
Tamás P, Hawley SA, Clarke RG, et al. Regulation of the energy sensor AMP-activated protein kinase by antigen receptor and Ca2+ in T lymphocytes. J Exp Med. 2006 Jul 10;203(7):1665-70.
Spina CA, Anderson J, Archin NM, et al. An in-depth comparison of latent HIV-1 reactivation in multiple cell model systems and resting CD4+ T cells from aviremic patients. PLoS Pathog 2013;9(12):e1003834.
Liu WC, Slusarchyk DS, Astle G, Trejo WH, Brown WE, Meyers E. Ionomycin, a new polyether antibiotic. J Antibiot (Tokyo). 1978 Sep;31(9):815-9.
0 notes
Text
Metformin shown for the first time to inhibit Zika virus in human umbilical cells: AMPK links virus destruction with Progeria, HIV & Cancer stem cells

By Jim Gathany [Public domain], via Wikimedia Commons; CC-BY-SA-3.0 http://creativecommons.org/licenses/by-sa/3.0/)], via Wikimedia Commons
A study published in the Journal of Virology in December of 2017 by researchers from the University of Southern California showed for the first time that AMPK activators including the anti-diabetic drug metformin caused an approximately 60% to 80% decrease in virus production from human umbilical vein endothelial cells (HUVECs) infected with Zika virus (ZIKV) [1]. ZIKV has been causally linked to microcephaly (head circumference smaller than normal due to abnormal brain development) and has been shown to efficiently infect HUVECs, which directly contact the fetal blood stream [2]. Metformin-induced inhibition of ZIKV replication in HUVECs may thus represent a powerful, safe, and economically viable option to treat and/or prevent conditions associated with ZIKV infection. Interestingly, as further explained below, metformin and AMPK have recently been shown to exert antiviral and anti-parasitic effects against dengue virus (which is transmitted by the same mosquito vector as ZIKV) and different Plasmodium species (the etiological agent of malaria), respectively.
Additionally, metformin activates AMPK and alleviates accelerating aging defects in cells from children with the genetic disorder Hutchinson-Gilford progeria syndrome (HGPS), promotes the differentiation and/or apoptosis of cancer stem cells in an AMPK-dependent manner, and destabilizes the latent HIV-1 reservoir in chronically-infected HIV-1 patients (facilitating virus elimination and potentially contributing to an HIV-1 cure). Also, because AMPK is critical for oocyte maturation and bacteria-derived antibiotics (e.g. ionomycin, A23187) that activate AMPK are used extensively to activate human oocytes to create normal, healthy babies, it is likely that stress-induced AMPK activation (e.g. via reactive oxygen species, intracellular calcium, and/or AMP/ATP ratio increase, etc.) represents a common mechanism linking pathogen elimination with HGPS, caner stem cell elimination, HIV-1 eradication, and the creation of all human life, as I originally proposed in several recent publications (see below) [3-6].
As noted above, the AMPK activators metformin and AICAR potently inhibited ZIKV replication in HUVECs [1]. Although the authors unexplainably found that compound C (an AMPK inhibitor) also inhibited ZIKV replication, AMPK activation has recently been found to exert significant antiviral effects against Rift Valley Fever virus as well as multiple arbovirus family members including the Flavivirus Kunjin virus, the Togavirus Sindbis virus, and the Rhabdovirus Vesicular stomatitis virus [1,7]. Indeed, several AMPK-activating compounds have also recently demonstrated antiviral effects against ZIKV infection and replication. For example, EGCG (found in green tea) inhibited ZIKV entry in Vero E6 cells, curcumin (derived from the plant Curcuma longa) inhibited ZIKV replication in HeLa cells, NDGA (derived from the plant Larrea tridentate) reduced viral yield in Vero cells infected with a ZIKV strain isolated from a human patient, sophoraflavenone G (isolated from the plant Sophora Flavecens) inhibits ZIKV replication in A549 cells, hemin (an iron-containing porphyrin) significantly inhibited ZIKV replication in primary human monocyte-derived macrophages, quercetin (found in a variety of plants) exerted antiviral activity against ZIKV in both tissue culture and knockout mice, and chloroquine (an anti-malarial compound) inhibited ZIKV infection in vitro and protected fetal mice from ZIKV-induced microcephaly [8-14]. Similar to metformin, each of the aforementioned compounds or the plant extracts from which they are derived activates AMPK in vivo and/or in vitro [15-19].
Interestingly, as both ZIKV and dengue virus (DENV) are transmitted by the same mosquito vector, a study recently published in the journal PLoS Pathogens in April of 2017 demonstrated for the first time that metformin exerted significant antiviral effects in DENV-infected human liver cells that was dependent on activation of the master metabolic regulator AMPK [20]. The authors showed that an increase in HMG-CoA reductase (HMGCR) activity, a target of AMPK, was associated with DENV-infected cells, AMPK activation was reduced in DENV-infected cells at 12 and 24 hours post infection (hpi), and metformin significantly decreased the number of infected cells, viral yield, and viral genome copies, leading the authors to conclude that metformin-induced AMPK activation generates a strong antiviral effect against DENV [20]. Recent efforts funded by the U.S. and British governments, the Bill & Melinda Gates Foundation, and the Google health spin-off Verily have sought to decrease the spread of dengue and Zika viruses through the coordinated release of female and/or male mosquitoes (called Aedes aegypti) that were purposely infected with a bacterium that inhibits the mosquito’s ability to transmit the two viruses to humans [21,22]. Studies have shown that this bacterium, called Wolbachia, enhances the mosquito’s immune response by increasing the levels of reactive oxygen species (ROS), thus enhancing inhibition of DENV replication [23]. Because AMPK is activated by cellular stress (e.g. ROS increase, intracellular calcium [Ca2+] increase, AMP/ATP ratio increase, etc.), has been found in Aedes aegypti (Ae. aegypti), and AMPK activation by stress-inducing compounds (e.g. resveratrol) increased average life span and enhanced the immune response in Ae. aegypti in an AMPK-dependent manner, the recent finding that metformin also inhibits DENV replication in human cells in an AMPK-dependent manner provides compelling evidence that the anti-viral and antimicrobial effects of AMPK activation likely crosses species boundaries [24].
Moreover, a study published in the journal Cell Reports in September of 2016 by researchers from the Massachusetts Institute of Technology (MIT) and the University of Lisbon also showed for the first time that metformin and other AMPK activators significantly reduced parasite load in human liver cells of different species of Plasmodium, a protozoan parasite that is the etiological agent of malaria [25]. Importantly, the authors also showed that AMPK activation inhibits growth and replication of different Plasmodium spp. (species) and AMPK activators as well as dietary restriction, which activates AMPK, reduces Plasmodium berghei (malaria-causing species in rodents often used as a model for the study of human malaria) infection in mice [25]. The AMPK-activating compounds salicylate and A769662 also reduced P. berghei and P. falciparum (malaria parasite that infects humans) merozoite formation (infectious parasites generated through replication in erythrocytes) in vitro while salicylate decreased parasitaemia in mice in vivo [26].
Cellular stress-induced AMPK activation has also been shown to exert antiviral effects against HIV-1. AMPK activation and several AMPK-activating compounds, including EGCG, curcumin, tanshinone II A (derived from the plant Salvia miltiorrhiza), byrostatin-1 (isolated from the marine organism Bugula neritina), and resveratrol (found in grapes and in the plant Polygonum cuspidatum) have been shown to exhibit antiviral activity in vitro against HIV-1 [27-31].
An active area among HIV-1 cure researchers, known as the “shock and kill” approach, involves reactivating (i.e. “shock”) a T cell (or another immune cell) that harbors dormant HIV-1, hence reactivating the virus itself and thus inducing destruction of the T cell along with the virus or enhancing recognition and destruction of the virus-infected T cell by the immune system (i.e. “kill”) [4]. Strikingly, AMPK is also critical for the activation of T cells and the mounting of an effective immune response to eliminate viruses, bacteria, and cancer cells [5,32,33]. A recent study demonstrated that metformin, when combined with the protein kinase C modulator bryostatin, induced reactivation of latent HIV-1 in a monocytic cell line in an AMPK-dependent manner. Bryostatin was also shown to induce phosphorylation and activation of AMPK in that study, implying that bryostatin is an indirect AMPK activator as well [30]. Furthermore, the calcium ionophores ionomycin and A23187, both of which activate AMPK and induce human oocyte activation, are often combined with phorbol 12-myristate 13-acetate (PMA) and are extremely efficient in promoting T cell activation-induced latent HIV-1 reactivation [34-36].
Perhaps most convincingly, at the International AIDS Society’s (IAS) HIV Cure and Cancer Forum held in Paris, France in July of 2017, researchers from the University of Hawaii demonstrated for the first time that metformin decreased the percentage of CD4+ T cells expressing the immune checkpoint receptors PD-1, TIGIT, and TIM-3 in HIV-1 patients, receptors that are positively associated with T cells that harbor latent HIV-1. Metformin also destabilized the latent viral reservoir in chronically-infected HIV-1 patients, indicating that metformin may indeed contribute to HIV-1 eradication by inducing an AMPK-mediated reactivation of latent HIV-1, as I initially proposed in 2015 and 2016 [4,5, 37-41].
Also, stress-induced AMPK activation likely also links latent HIV-1 reactivation with alleviation of accelerated aging defects in cells derived from children with the genetic disorder Hutchinson-Gilford progeria syndrome (HGPS). Studies have shown that efficient reactivation of latent HIV-1 involves a reduction in the splicing of the HIV-1 genome by the gene splicing factor SRSF1 [42-44]. Accelerated cellular aging-like phenotypes in HGPS are primarily linked to aberrant splicing of the LMNA gene, leading to the over production of a toxic protein called progerin [3]. Evidence has also shown that inhibition of the splicing factor SRSF1 leads to a reduction in progerin at both the mRNA and protein levels [4,45].
A recent study published online in the Journal npj Aging and Mechanisms of Disease in November of 2016 provided startling evidence that metformin decreased the expression of progerin and SRSF1 and alleviated pathological defects in cells derived from HGPS patients [46]. Another study published online in the Journal Experimental Dermatology in February of 2017 confirmed that metformin alleviated nuclear defects and premature aging phenotypes and activated AMPK in fibroblasts derived from HGPS patients, substantiating my original hypotheses from 2014 and 2015 proposing that AMPK activators including metformin would improve accelerated aging defects in HGPS cells by inhibiting SRSF1 and activating AMPK [3,4,47]. Temsirolimus, an analog of the macrolide rapamycin (which activates AMPK in vivo), also partially rescued the HGPS cellular phenotype but significantly increased the levels of ROS and superoxide within the first hour of treatment, providing further indication that the induction of cellular stress and subsequent AMPK activation links virus and pathogen elimination with alleviation of accelerated cellular aging defects in HGPS [48,49].
Furthermore, ROS and calcium are well-studied mediators of cellular stress-induced differentiation of embryonic and adult stem cells, AMPK has recently been shown to be essential for mouse embryonic stem cell differentiation, and metformin targets and promotes differentiation and/or apoptosis of cancer stem cells in the deadliest of cancers in an AMPK-dependent manner, including glioblastoma and pancreatic cancer [6]. Such evidence strongly suggests that cellular stress-induced AMPK activation by compounds including metformin links pathogen and virus elimination with HGPS and cancer stem cell differentiation and/or apoptosis, a hypothesis that I first proposed in 2017 [6].
Lastly, AMPK activation also promotes oocyte meiotic induction and maturation (processes that are critical for efficient oocyte activation) and AMPK has recently been found localized across the entire acrosome in human spermatozoa [5,50,51]. The induction of cellular stress (e.g. increases in ROS, intracellular calcium, and/or AMP/ATP ratio increase), which activates AMPK, also promotes oocyte meiotic induction/maturation, oocyte activation, and the acrosome reaction in human sperm, processes critical for the creation of all human life [50,52,53]. Indeed, oocyte activation is indispensable for the creation of all human life and the bacteria-derived calcium ionophore ionomycin, which activates AMPK, is commonly used to promote latent HIV-1 reactivation and is extensively used to activate human oocytes, creating normal healthy children (i.e. the “shock and live” approach) [53-57]. Such evidence indicates and further substantiates the novel and provocative assertion that AMPK activation links Zika virus inhibition and pathogen elimination with the amelioration of accelerated aging defects in HGPS cells, HIV-1 latency and replication, adult and cancer stem cells, and the creation of all human life [1,3-6].
https://www.linkedin.com/pulse/metformin-shown-first-time-inhibit-zika-virus-human-umbilical-finley/
References
Cheng F, Ramos da Silva S, Huang IC, Jung JU, Gao SJ. Suppression of Zika virus infection and replication in endothelial cells and astrocytes by PKA inhibitor PKI 14-22. J Virol. 2017 Dec 6. pii: JVI.02019-17. doi: 10.1128/JVI.02019-17. [Epub ahead of print].
Richard AS, Shim BS, Kwon YC, et al. AXL-dependent infection of human fetal endothelial cells distinguishes Zika virus from other pathogenic flaviviruses. Proc Natl Acad Sci U S A. 2017 Feb 21;114(8):2024-2029.
Finley J. Alteration of splice site selection in the LMNA gene and inhibition of progerin production via AMPK activation. Med Hypotheses. 2014 Nov;83(5):580-7.
Finley J. Reactivation of latently infected HIV-1 viral reservoirs and correction of aberrant alternative splicing in the LMNA gene via AMPK activation: Common mechanism of action linking HIV-1 latency and Hutchinson-Gilford progeria syndrome. Med Hypotheses. 2015 Sep;85(3):320-32.
Finley J. Oocyte activation and latent HIV-1 reactivation: AMPK as a common mechanism of action linking the beginnings of life and the potential eradication of HIV-1. Med Hypotheses. 2016 Aug;93:34-47.
Finley J. Elimination of cancer stem cells and reactivation of latent HIV-1 via AMPK activation: Common mechanism of action linking inhibition of tumorigenesis and the potential eradication of HIV-1. Med Hypotheses. 2017 Jul;104:133-146.
Moser TS, Schieffer D, Cherry S. AMP-activated kinase restricts Rift Valley fever virus infection by inhibiting fatty acid synthesis. PLoS Pathog. 2012;8(4):e1002661.
Carneiro BM, Batista MN, Braga AC, Nogueira ML, Rahal P. The green tea molecule EGCG inhibits Zika virus entry. Virology. 2016 Sep;496:215-8.
Mounce BC, Cesaro T, Carrau L, Vallet T, Vignuzzi M. Curcumin inhibits Zika and chikungunya virus infection by inhibiting cell binding. Antiviral Res. 2017 Jun;142:148-157.
Merino-Ramos T, Jiménez de Oya N, Saiz JC, Martín-Acebes MA. Antiviral activity of nordihydroguaiaretic acid and its derivative tetra-O-methyl nordihydroguaiaretic acid against West Nile virus and Zika virus. Antimicrob Agents Chemother. 2017 May 15. pii: AAC.00376-17.
Sze A, Olagnier D, Hadj SB, et al. Sophoraflavenone G Restricts Dengue and Zika Virus Infection via RNA Polymerase Interference. Viruses. 2017 Oct 3;9(10). pii: E287.
Huang H, Falgout B, Takeda K, Yamada KM, Dhawan S. Nrf2-dependent induction of innate host defense via heme oxygenase-1 inhibits Zika virus replication. Virology. 2017 Mar;503:1-5.
Wong G, He S, Siragam V, et al. Antiviral activity of quercetin-3-β-O-D-glucoside against Zika virus infection. Virol Sin. 2017 Sep 5. doi: 10.1007/s12250-017-4057-9. [Epub ahead of print].
Li C, Zhu X, Ji X, et al. Chloroquine, a FDA-approved Drug, Prevents Zika Virus Infection and its Associated Congenital Microcephaly in Mice. EBioMedicine. 2017 Oct;24:189-194.
Kim I, He YY. Targeting the AMP-Activated Protein Kinase for Cancer Prevention and Therapy. Front Oncol. 2013 Jul 15;3:175.
Zhang H, Li Y, Hu J, et al. Effect of Creosote Bush-Derived NDGA on Expression of Genes Involved in Lipid Metabolism in Liver of High-Fructose Fed Rats: Relevance to NDGA Amelioration of Hypertriglyceridemia and Hepatic Steatosis. PLoS One. 2015 Sep 22;10(9):e0138203.
Yang X, Yang J, Xu C, et al. Antidiabetic effects of flavonoids from Sophora flavescens EtOAc extract in type 2 diabetic KK-ay mice. J Ethnopharmacol. 2015 Aug 2;171:161-70.
Park EJ, Kim YM, Chang KC. Hemin Reduces HMGB1 Release by UVB in an AMPK/HO-1-dependent Pathway in Human Keratinocytes HaCaT Cells. Arch Med Res. 2017 Jul;48(5):423-431.
Spears LD, Tran AV, Qin CY, et al. Chloroquine increases phosphorylation of AMPK and Akt in myotubes. Heliyon. 2016 Mar;2(3):e00083.
Soto-Acosta R, Bautista-Carbajal P, Cervantes-Salazar M, Angel-Ambrocio AH, Del Angel RM. DENV up-regulates the HMG-CoA reductase activity through the impairment of AMPK phosphorylation: A potential antiviral target. PLoS Pathog. 2017 Apr 6;13(4):e1006257.
http://www.reuters.com/article/us-health-dengue-mosquitoes-idUSKCN12Q1PE
https://www.bloomberg.com/news/articles/2016-10-06/alphabet-s-verily-joins-zika-fight-with-sterile-mosquito-lab
Pan X, Zhou G, Wu J, et al. Wolbachia induces reactive oxygen species (ROS)-dependent activation of the Toll pathway to control dengue virus in the mosquito Aedes aegypti. Proc Natl Acad Sci U S A. 2012 Jan 3;109(1):E23-31.
Nunes RD, Ventura-Martins G, Moretti DM, et al. Polyphenol-Rich Diets Exacerbate AMPK-Mediated Autophagy, Decreasing Proliferation of Mosquito Midgut Microbiota, and Extending Vector Lifespan. PLoS Negl Trop Dis. 2016 Oct 12;10(10):e0005034.
Ruivo MT, Vera IM, Sales-Dias J, et al. Host AMPK Is a Modulator of Plasmodium Liver Infection. Cell Rep. 2016 Sep 6;16(10):2539-45.
Mancio-Silva L, Slavic K, Grilo Ruivo MT, et al. Nutrient sensing modulates malaria parasite virulence. Nature. 2017 Jul 13;547(7662):213-216.
Zhang HS, Wu TC, Sang WW, Ruan Z. EGCG inhibits Tat-induced LTR transactivation: role of Nrf2, AKT, AMPK signaling pathway. Life Sci. 2012 May 22;90(19-20):747-54.
Zhang HS, Ruan Z, Sang WW. HDAC1/NFκB pathway is involved in curcumin inhibiting of Tat-mediated long terminal repeat transactivation. J Cell Physiol. 2011 Dec;226(12):3385-91.
Zhang HS, Chen XY, Wu TC, Zhang FJ. Tanshinone II A inhibits tat-induced HIV-1 transactivation through redox-regulated AMPK/Nampt pathway. J Cell Physiol. 2014 Sep;229(9):1193-201.
Mehla R, Bivalkar-Mehla S, Zhang R, et al. Bryostatin modulates latent HIV-1 infection via PKC and AMPK signaling but inhibits acute infection in a receptor independent manner. PLoS One. 2010 Jun 16;5(6):e11160.
Zhang HS, Wu MR. SIRT1 regulates Tat-induced HIV-1 transactivation through activating AMP-activated protein kinase. Virus Res. 2009 Dec;146(1-2):51-7.
Pearce EL, Walsh MC, Cejas PJ, et al. Enhancing CD8 T-cell memory by modulating fatty acid metabolism. Nature. 2009 Jul 2;460(7251):103-7.
Blagih J, Coulombe F, Vincent EE, et al. The energy sensor AMPK regulates T cell metabolic adaptation and effector responses in vivo. Immunity. 2015 Jan 20;42(1):41-54.
Gómez-Gonzalo M, Carretero M, Rullas J et al. The hepatitis B virus X protein induces HIV-1 replication and transcription in synergy with T-cell activation signals: functional roles of NF-kappaB/NF-AT and SP1-binding sites in the HIV-1 long terminal repeat promoter. J Biol Chem. 2001 Sep 21;276(38):35435-43.
Tamás P, Hawley SA, Clarke RG, et al. Regulation of the energy sensor AMP-activated protein kinase by antigen receptor and Ca2+ in T lymphocytes. J Exp Med. 2006 Jul 10;203(7):1665-70.
Fogarty S, Hawley SA, Green KA, Saner N, Mustard KJ, Hardie DG. Calmodulin-dependent protein kinase kinase-beta activates AMPK without forming a stable complex: synergistic effects of Ca2+ and AMP. Biochem J. 2010 Jan 27;426(1):109-18.
G.M. Chew, D.C. Chow, S.A. Souza, et al. Impact of adjunctive metformin therapy on T cell exhaustion and viral persistence in a clinical trial of HIV-infected adults on suppressive ART. Journal of Virus Eradication 2017; 3 (Supplement 1): 6–19.
http://viruseradication.com/supplement-details/Abstracts_of_the_IAS_HIV_Cure_and_Cancer_Forum_2017/
http://www.iasociety.org/HIV-Programmes/Towards-an-HIV-Cure/Events/HIV-Cure-Cancer-Forum
Fromentin R, Bakeman W, Lawani MB, et al. CD4+ T Cells Expressing PD-1, TIGIT and LAG-3 Contribute to HIV Persistence during ART. PLoS Pathog. 2016 Jul 14;12(7):e1005761.
Chew GM, Fujita T, Webb GM, et al. TIGIT Marks Exhausted T Cells, Correlates with Disease Progression, and Serves as a Target for Immune Restoration in HIV and SIV Infection. PLoS Pathog. 2016 Jan 7;12(1):e1005349.
Berro R, Kehn K, de la Fuente C, et al. Acetylated Tat regulates human immunodeficiency virus type 1 splicing through its interaction with the splicing regulator p32. J Virol. 2006 Apr;80(7):3189-204.
Spina CA, Anderson J, Archin NM, et al. An in-depth comparison of latent HIV-1 reactivation in multiple cell model systems and resting CD4+ T cells from aviremic patients. PLoS Pathog. 2013;9(12):e1003834.
Hu M, Crawford SA, Henstridge DC, et al. p32 protein levels are integral to mitochondrial and endoplasmic reticulum morphology, cell metabolism and survival. Biochem J. 2013 Aug 1;453(3):381-91.
Lopez-Mejia IC, Vautrot V, De Toledo M, et al. A conserved splicing mechanism of the LMNA gene controls premature aging. Hum Mol Genet. 2011 Dec 1;20(23):4540-55.
Egesipe AL, Blondel S, Cicero AL, et al. Metformin decreases progerin expression and alleviates pathological defects of Hutchinson-Gilford progeria syndrome cells. NPJ Aging Mech Dis. 2016 Nov 10;2:16026.
Park SK, Shin OS. Metformin Alleviates Ageing Cellular Phenotypes in Hutchinson-Gilford Progeria Syndrome Dermal Fibroblasts. Exp Dermatol. 2017 Oct;26(10):889-895.
Gabriel D, Gordon LB, Djabali K. Temsirolimus Partially Rescues the Hutchinson-Gilford Progeria Cellular Phenotype. PLoS One. 2016 Dec 29;11(12):e0168988.
Chiao YA, Kolwicz SC, Basisty N, et al. Rapamycin transiently induces mitochondrial remodeling to reprogram energy metabolism in old hearts. Aging (Albany NY). 2016 Feb;8(2):314-27.
LaRosa C, Downs SM. Stress stimulates AMP-activated protein kinase and meiotic resumption in mouse oocytes. Biol Reprod. 2006 Mar;74(3):585-92.
Calle-Guisado V, de Llera AH, Martin-Hidalgo D, et al. AMP-activated kinase in human spermatozoa: identification, intracellular localization, and key function in the regulation of sperm motility. Asian J Androl. 2016 Sep 27. doi: 10.4103/1008-682X.185848. [Epub ahead of print].
de Lamirande E, Tsai C, Harakat A, Gagnon C. Involvement of reactive oxygen species in human sperm arcosome reaction induced by A23187, lysophosphatidylcholine, and biological fluid ultrafiltrates. J Androl. 1998 Sep-Oct;19(5):585-94.
Tesarik J, Sousa M, Testart J. Human oocyte activation after intracytoplasmic sperm injection. Hum Reprod. 1994 Mar;9(3):511-8.
Deemeh MR, Tavalaee M, Nasr-Esfahani MH. Health of children born through artificial oocyte activation: a pilot study. Reprod Sci. 2015 Mar;22(3):322-8.
Tamás P, Hawley SA, Clarke RG, et al. Regulation of the energy sensor AMP-activated protein kinase by antigen receptor and Ca2+ in T lymphocytes. J Exp Med. 2006 Jul 10;203(7):1665-70.
Spina CA, Anderson J, Archin NM, et al. An in-depth comparison of latent HIV-1 reactivation in multiple cell model systems and resting CD4+ T cells from aviremic patients. PLoS Pathog 2013;9(12):e1003834.
Liu WC, Slusarchyk DS, Astle G, Trejo WH, Brown WE, Meyers E. Ionomycin, a new polyether antibiotic. J Antibiot (Tokyo). 1978 Sep;31(9):815-9
0 notes
Text
Antibiotics produced by Bacteria activate Human Oocytes, creating Healthy Babies: AMPK links the Creation of Human Life with HIV, Progeria, & Cancer
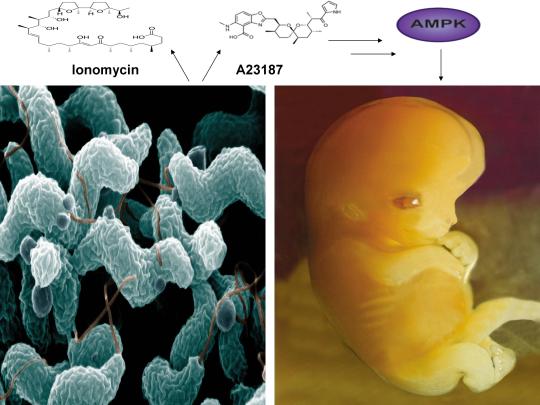
CC-BY-SA-3.0 (http://creativecommons.org/licenses/by-sa/3.0/)], via Wikimedia Commons;By De Wood, Pooley, USDA, ARS, EMU. [Public domain], via Wikimedia Commons
A recent study published online in the journal Fertility and Sterility in September of 2017 systematically reviewed for the first time evidence for the effect of two compounds, ionomycin and A23187 (also known as calcimycin), on fertilization rates and pregnancy outcomes in infertile couples undergoing an in vitro fertilization procedure known as intracytoplasmic sperm injection (ICSI) [1]. ICSI involves the direct deposition of sperm into the oocyte cytoplasm, which typically leads to high rates of fertilization. However, fertilization failure despite repeated ICSI is likely caused by a failure of the oocyte to activate [1]. Physiological oocyte activation is accomplished by the delivery of a sperm-borne oocyte activating factor called phospholipase C zeta (PLCζ). PLCζ activates human oocytes by inducing an intracellular signaling cascade that ultimately results in increased calcium (Ca2+) oscillations in the oocyte, which drives oocyte activation to completion [1]. As oocyte activation is an indispensable prerequisite for the creation of all human life, every human being alive today and any human being that has ever lived began their existence as an activated oocyte [2]. Ionomycin and A23187 increase the levels of intracellular Ca2+ and are thus commonly known as Ca2+ ionophores [1]. The authors of the Fertility and Sterility study showed that over a total of 1,521 ICSI cycles, calcium ionophores including ionomycin and A23187 led to a statistically significant improvement in fertilization, cleavage, blastulation, implantation rates, overall pregnancy, and live-birth rates [1]. Ionomycin and A23187 have also been shown in several independent studies to effectively induce human oocyte activation, leading to the birth of normal, healthy children [3,4].
Strikingly, as described further below, both ionomycin and A23187 are antibiotics that are naturally produced by certain species within the bacterial genus Streptomyces [5,6]. Other structurally distinct compounds and methods have also been shown to induce human oocyte activation, including ethanol, puromycin (an antibiotic and protein synthesis inhibitor produced by Streptomyces alboniger), as well as mechanical manipulation and electrical stimulation, both of with have been reported to result in the creation of normal children [7-11]. Interestingly, as mouse oocytes are considered models for human oocytes, ionomycin, A23187, anisomycin (an antibiotic and protein synthesis inhibitor produced by Streptomyces griseolus), mycophenolic acid (an immunosuppressant produced by the fungus Penicillium brevicompactum), cycloheximide (a protein synthesis inhibitor produced by Streptomyces griseus), carvacrol (a secondary plant metabolite produced by Origanum vulgare{oregano}), and phorbol 12-myristate 13-acetate (PMA, a secondary plant metabolite produced by Croton tiglium) each induce activation of mouse oocytes [12-22]. Ionomycin, A23187, PMA, and reactive oxygen species (ROS) also induce the acrosome reaction in human sperm, a process characterized by the release of hydrolytic enzymes from the head of sperm which is necessary for oocyte penetration and thus indispensable for the creation of all human life outside of a clinical setting (ICSI bypasses the need for oocyte penetration) [23,24]. Additionally, although an over-production of ROS, similar to Ca2+, may lead to deleterious effects including cell death/apoptosis, low levels of ROS have been shown to act as signaling molecules and ROS is significantly increased on or immediately following mouse oocyte activation [25,26].
Furthermore, the master metabolic regulator AMPK is critical for oocyte meiotic resumption and maturation (a process that precedes and is essential for oocyte activation), is found located across the entire acrosome in the head of human sperm, and is activated by increases in ROS and Ca2+ [27-29]. Ionomycin, A23187, ethanol, puromycin, mechanical force, electrical stimulation, anisomycin, mycophenolic acid, carvacrol, and PMA also induce AMPK activation, indicating that a common mechanism of action links chemically distinct compounds with the creation of human life [30-39]. This common mechanism of action likely centers on the induction of cellular stress, mediated by indirect increases in intracellular Ca2+, ROS, and/or the AMP(ADP)/ATP ratio, etc. as I originally proposed in 2016 [40]. Because the bacterial-derived antibiotics ionomycin and A23187 induce both the acrosome reaction in human sperm and human oocyte activation, producing normal, healthy children, it can be said that “non-human organisms have the power to create human life or the power to end life.” As explained below, the beneficial effects of cellular stress induction (i.e. a “shock”) crosses species boundaries and may indeed play a role in facilitating natural selection, a process that underlies and drives evolution.
A number of bacterial species residing within the genus Streptomyces have proven to be extremely important and medicinally valuable as approximately 70% of clinically useful antibiotics are derived from Streptomyces [41]. The antibiotics ionomycin and A23187 are naturally produced by Streptomyces conglobatus and Streptomyces chartreusensis, respectively [5,6]. Other important examples include the antibiotic tetracycline (produced by Streptomyces aureofaciens), the immunosuppressant rapamycin (produced by Streptomyces hygroscopicus), and the anti-helminthic avermectins (produced by Streptomyces avermitilis) [42]. Many soil and aquatic-dwelling species of Streptomyces can be found in harsh environments and are characterized by a unique life cycle, including spore germination followed by vegetative mycelium production, aerial hyphae formation, sporulation (i.e. spore formation), and antibiotic production [43,44]. Curiously, just as cellular stress induction leads to the creation of human life and other beneficial effects in human cells (see below), stress induction also promotes the induction of aerial hyphae formation, sporulation, and antibiotic production in many Streptomyces species (spp.). Indeed, a decrease in the levels of ATP and bacterial growth is associated with sporulation, aerial hyphae formation, and antibiotic production [42,45]. A reduction in glucose/nutritional deprivation, the preferred sugar/carbon source for many Streptomyces spp., also significantly increases antibiotic production [46]. An increase in intracellular ROS and Ca2+ is associated with spore germination, aerial hyphae formation, and antibiotic production [47-49]. Other cellular stressors, including heat shock and ethanol, also significantly increase antibiotic production, provocatively indicating that the effects of cellular stress crosses species boundaries, enhancing bacterial survival and facilitating the creation of human life [50,51].
The beneficial effects of low-level cellular stress induction also extends to plants, as many plants produce secondary metabolites partly for the purpose of self-defense, analogous to antibiotics. Similar to the harsh, stressful environments often inhabited by Streptomyces spp., the Great Basin Bristlecone Pine (Pinus Longaeva), considered the oldest living non-clonal organism on the planet ( >5000 years old), thrives in an exceptionally harsh environment, characterized by increased elevations and exposure to UV radiation, nutritionally-deprived soils, harsh temperatures, and mechanical stress due to wind variances, leading early researchers to conclude that it’s longevity is intimately associated with adversity [52-54]. Conversely, Pinus Longaeva species that are located in less stressful environments (i.e. lower elevations) are strongly associated with younger age classes (<875 years) [55]. Similarly, the Creosote bush (Larrea tridentate), considered one of the oldest living clonal organisms on the planet (>11,000 years old), also thrives in harsh environments including the Mohave Desert [56]. AMPK, which increases lifespan and healthspan in several model organisms, is the primary sensor of cellular stress in eukaryotic organisms (e.g. plants and humans) and the plant AMPK orthologue SnRK1 as well as Ca2+ and ROS are critical for seed germination, fertilization, root gravitropism, and secondary metabolite production [57-64]. The secondary plant metabolites PMA (which activates mouse oocytes and promotes the acrosome reaction in human sperm) and artemisinin (an anti-malarial drug) both activate AMPK and the antibiotic A23187 also increases production of the secondary metabolite resveratrol in grape cell cultures, again indicating that exposure to low-level stressors may promote extension of lifespan and initiate the creation of human life [17,23,39,65,66].
Organismal exposure to beneficial levels of stress may also play a critical role in evolution. As first noted by Charles Darwin, evolution is driven by natural selection, a process characterized by environmentally-induced phenotypic changes that may lead to inheritable survival and reproductive advantages [67]. From “On the Origin of Species by Means of Natural Selection, or the Preservation of Favoured Races in the Struggle for Life”, Darwin explained that “if there be, owing to the high geometrical powers of increase of each species, at some age, season, or year, a severe struggle for life, and this certainly cannot be disputed;……But if variations useful to any organic being do occur, assuredly individuals thus characterised will have the best chance of being preserved in the struggle for life;” [67]. This “struggle for life” Darwin spoke of is embodied by selective pressures which may be abiotic (i.e. light, wind, temperature, etc.) or biotic (predation, disease, competition, etc.) [68,69]. As alluded to above, such selective pressures are indeed sources of cellular stress, sensed by both prokaryotes and eukaryotes, that induce beneficial responses (at appropriate levels), leading to the acquisition of phenotypes conducive for continued survival. Both biotic (e.g. infection) and abiotic (e.g. heat) stressors/selective pressures activate AMPK (which is evolutionarily conserved among eukaryotes) in human cells [70,71]. A phenomenon often cited as an example of natural selection on a readily observable timescale is the development of bacterial resistance to antibiotics, resulting in problematic mutant strains that may be life-threatening for some individuals (i.e. the elderly and immunocompromised) [72]. Intriguingly, lethal levels of bactericidal antibiotics have been shown to kill microorganisms via the induction of ROS, sub-lethal levels of bactericidal antibiotics however increase mutagenesis and bacterial resistance via induction of lower levels of ROS, and heat as well as nutritional stress increase bacterial resistance to antibiotics, providing compelling evidence that continuous exposure to low levels of stress likely plays a significant role in natural selection and evolution [73-75].
Moreover, gravity itself likely functions as a cellular stressor/selective pressure that has influenced the development of organisms on Earth since the emergence of the very first lifeform. Gravity exerts its effects on living organisms via the application of force, which is experienced by human cells in the form of mechanical loading or stress [76]. The application of force or a mechanical load has recently been shown to activate AMPK and simulated microgravity (i.e. hind limb unloading in mice) significantly decreases AMPK activation [77,78]. Spaceflight also inhibits the activation of T cells (immune cells essential for adaptive immunity), whereas the application of force and AMPK activation promote T cell activation [79-81]. Interestingly, spaceflight has recently been shown to decrease the levels of the master antioxidant transcription factor Nrf2 and the heat shock-inducible protein HSP90a but increase the levels of the growth-promoting kinase mTOR in mice [82]. AMPK however inhibits mTOR but increases the phosphorylation, nuclear retention, and transcriptional activity of Nrf2 [57,83,84]. Also, HSP90 interacts with and maintains AMPK activity and HSP90 is necessary for progesterone-induced human sperm acrosome reaction [85,86]. Interestingly, rapamycin, an immunosuppressant produced by Streptomyces hygroscopicus, extends lifespan in genetically heterogeneous mice, activates AMPK in vivo in normal aged mice, and increases human sperm motility [42,87,88]. Simulated microgravity via the use of NASA-designed rotating wall vessels (RWVs) however drastically reduces rapamycin production (~90%) whereas the antibiotic gentamycin increases rapamycin production by Streptomyces hygroscopicus, providing further evidence that cellular stress, in the form of mechanical loading induced by gravity, is essential for development, function, and survival of Earth-bound organisms [89,90].
The induction of cellular stress also links seemingly dissimilar physiological and pathological states with the activation of AMPK. As discussed above, both ionomycin and ROS activate AMPK and promote oocyte meiotic resumption, a process that is AMPK-dependent and is essential for efficient oocyte activation [27,30,91]. ROS is also critical for ovulation, PMA and ionomycin both activate mouse oocytes, and ionomycin is extensively used during ICSI procedures, creating normal healthy children, suggesting that cellular stress-induced AMPK activation is also essential for oocyte activation [3,4,12,17,92]. The activation of oocytes and T cells share strikingly similar intracellular signaling mechanisms (e.g. PLC-PIP2-DAG-PKC-IP3-Ca2+) and ionomycin combined with PMA are extremely effective in activating T cells and are often used as positive controls in HIV-1 latency reversal studies [93-95]. Reactivating latent/dormant HIV-1 in CD4+ T cells, potentially facilitating immune system detection and virus destruction, is currently being pursued as a method for the potential eradication of HIV-1 (called the “shock and kill” approach) [96]. Similar to oocyte activation, both Ca2+ and ROS are critical for T cell activation (and hence latent HIV-1 reactivation) and other cellular stress-inducing compounds, including NDGA derived from the Creosote bush, butyrate derived from bacteria, as well as ROS and HSP90 have been shown to reactivate latent HIV-1 [26,93,94,97-101]. Interestingly, AMPK inhibition leads to cell death on T cell activation, knockdown of AMPK significantly decreases HIV-1 replication, and metformin (a well-studied AMPK activator derived from the French Lilac plant) increases butyrate production in human diabetic patients [81,102,103]. Perhaps most convincingly, early preliminary data showed that metformin significantly reduced several markers preferentially associated with cells latently infected with HIV-1 (e.g. PD-1, TIGIT, TIM-3) and also destabilized the latent HIV-1 reservoir in chronically-infected HIV patients, indicating that cellular-stress induced AMPK activation likely links the creation of human life with the potential eradication of HIV-1, as I originally proposed in 2016 [40,104,105].
AMPK activation may also link the disparate disease states of HIV-1 latency and Hutchinson-Gilford progeria syndrome (HGPS). HGPS is a genetic disorder caused by aberrant alternative splicing of the LMNA gene, generating a toxic protein called progerin that induces an accelerated aging phenotype and premature death at approximately 14 years of age [106]. Excessive activity of the gene splicing factor SRSF1 has been shown to prevent reactivation of latent HIV-1 and contribute to aberrant splicing of the LMNA gene in HGPS [107-109]. Metformin however has recently been shown to ameliorate the accelerated aging phenotype in cells derived from children with HGPS by reducing the levels of both SRSF1 and progerin and activating AMPK, as I first proposed in 2014 [110-112]. Interestingly, both Ca2+ and ROS induce autophagy (a process of disposing of damaged/toxic proteins and organelles) and rapamycin, which activates AMPK in vivo and increases intracellular Ca2+ levels, improves accelerated aging in progeria cells by inducing autophagic degradation of progerin [87,113-116]. Temsirolimus, an analog of rapamycin, also alleviated accelerated aging defects in progeria cells but also increased the levels of ROS and superoxide within the first hour of treatment [117]. Such evidence strongly suggests that cellular stress-induced AMPK activation links the reversal of HIV-1 latency and alleviation of accelerated cellular aging defects in HGPS.
Cellular stress-induced AMPK activation also links the potential elimination of cancer stem cells (CSCs) with HIV-1 latency reversal and viral eradication. CSCs, which are largely resistant to chemoradiation therapy, are a subpopulation of cancer cells that exhibit characteristics similar to embryonic stem cells (ESCs), including self-renewal, multi-lineage differentiation, & the ability to initiate tumorigenesis [118,119]. Mechanisms that sustain quiescence & promote self-renewal in adult stem cells (ASCs) & CSCs likely also function to maintain latency of HIV-1 in CD4+ memory T cells. Indeed, HIV-1 has been found to establish long-lasting latency in a recently discovered subset of CD4+ T cells that exhibit stem cell-like properties known as T memory stem (TSCM) cells and increases in Ca2+, ROS, and AMPK activation have been shown to promote T cell activation and ESC, ASC, and CSC differentiation [119,120]. Additionally, A23187 and PMA have been shown to promote CSC differentiation (causing CSCs to become more susceptible to chemoradiation) and metformin induces CSC differentiation and/or apoptosis in an AMPK-dependent manner in the deadliest of cancers, including glioblastoma and pancreatic cancer, providing support for my publication in 2017 in which I first proposed that CSC differentiation and/or apoptosis and HIV-1 latency reversal/viral eradication may be linked by cellular stress-induced AMPK activation [119,121-124].
In conclusion, the ability of non-human organisms including certain Streptomyces spp. to initiate the creation of human life is predicated on the induction of cellular stress, mediated by increases in intracellular ROS, Ca2+, AMP(ADP)/ATP ratio increase, etc. The beneficial effects of transient cellular stress induction, which may be likened to selective pressures, crosses species boundaries and may indeed play a role in facilitating natural selection, a process that underlies and drives evolution, as evidenced by stress-induced increases in antibiotic production by Streptomyces spp. and stress-induced mutagenesis and antibiotic resistance in various bacterial strains. Because AMPK, a primary sensor of cellular stress in eukaryotic cells that increases lifespan and healthspan, plays a critical role in oocyte meiotic resumption/maturation, T cell activation, and stem cell differentiation, the creation of human life, the potential eradication of HIV-1, amelioration of accelerated aging in HGPS cells, and CSC differentiation/apoptosis are likely linked by a “Shock to Live”, or a “Shock to Kill”.
https://www.linkedin.com/pulse/antibiotics-produced-bacteria-activate-human-oocytes-creating-finley/
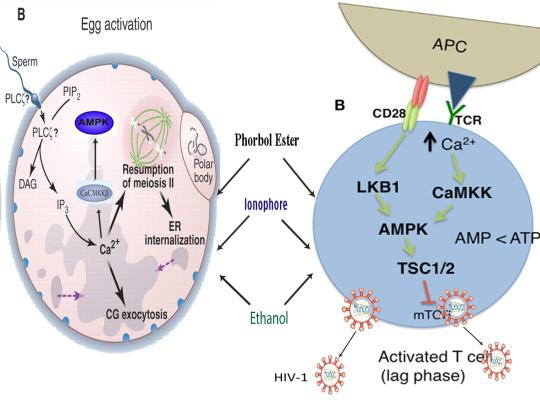
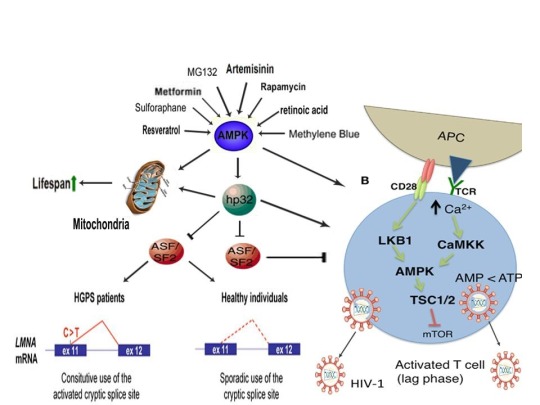
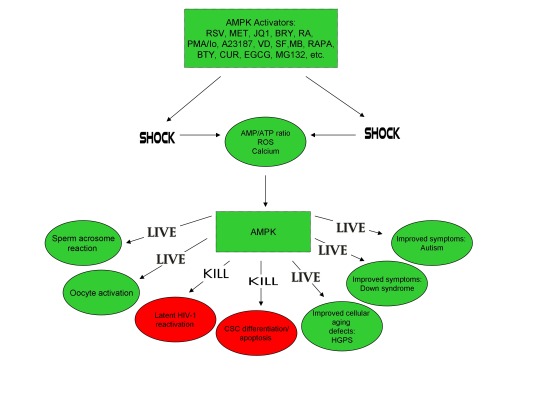
References:
Murugesu S, Saso S, Jones BP, et al. Does the use of calcium ionophore during artificial oocyte activation demonstrate an effect on pregnancy rate? A meta-analysis. Fertil Steril. 2017 Sep;108(3):468-482.e3.
Tesarik J, Sousa M, Testart J. Human oocyte activation after intracytoplasmic sperm injection. Hum Reprod. 1994 Mar;9(3):511-8.
Deemeh MR, Tavalaee M, Nasr-Esfahani MH. Health of children born through artificial oocyte activation: a pilot study. Reprod Sci. 2015 Mar;22(3):322-8.
Eftekhar M, Janati S, Rahsepar M, Aflatoonian A. Effect of oocyte activation with calcium ionophore on ICSI outcomes in teratospermia: A randomized clinical trial. Iran J Reprod Med. 2013 Nov;11(11):875-82.
Liu WC, Slusarchyk DS, Astle G, Trejo WH, Brown WE, Meyers E. Ionomycin, a new polyether antibiotic. J Antibiot (Tokyo). 1978 Sep;31(9):815-9.
Reed PW, Lardy HA. A23187: a divalent cation ionophore. J Biol Chem. 1972 Nov 10;247(21):6970-7.
Zhang Z, Wang T, Hao Y, et al. Effects of trehalose vitrification and artificial oocyte activation on the development competence of human immature oocytes. Cryobiology. 2017 Feb;74:43-49.
De Sutter P, Dozortsev D, Cieslak J, Wolf G, Verlinsky Y, Dyban A. Parthenogenetic activation of human oocytes by puromycin. J Assist Reprod Genet. 1992 Aug;9(4):328-37.
Sankaran L, Pogell BM. Biosynthesis of puromycin in Streptomyces alboniger: regulation and properties of O-demethylpuromycin O-methyltransferase. Antimicrob Agents Chemother. 1975 Dec;8(6):721-32.
Tesarik J, Rienzi L, Ubaldi F, Mendoza C, Greco E. Use of a modified intracytoplasmic sperm injection technique to overcome sperm-borne and oocyte-borne oocyte activation failures. Fertil Steril. 2002 Sep;78(3):619-24.
Mansour R, Fahmy I, Tawab NA, et al. Electrical activation of oocytes after intracytoplasmic sperm injection: a controlled randomized study. Fertil Steril. 2009 Jan;91(1):133-9.
Nikiforaki D, Vanden Meerschaut F, de Roo C, et al. Effect of two assisted oocyte activation protocols used to overcome fertilization failure on the activation potential and calcium releasing pattern. Fertil Steril. 2016 Mar;105(3):798-806.e2.
Liu H1, Zhang J, Krey LC, Grifo JA. In-vitro development of mouse zygotes following reconstruction by sequential transfer of germinal vesicles and haploid pronuclei. Hum Reprod. 2000 Sep;15(9):1997-2002.
Downs SM. Stimulation of parthenogenesis in mouse ovarian follicles by inhibitors of inosine monophosphate dehydrogenase. Biol Reprod. 1990 Sep;43(3):427-36.
Siracusa G, Whittingham DG, Molinaro M, Vivarelli E. Parthenogenetic activation of mouse oocytes induced by inhibitors of protein synthesis. J Embryol Exp Morphol. 1978 Feb;43:157-66.
Carvacho I, Lee HC, Fissore RA, Clapham DE. TRPV3 channels mediate strontium-induced mouse-egg activation. Cell Rep. 2013 Dec 12;5(5):1375-86.
Colonna R, Tatone C, Malgaroli A, Eusebi F, Mangia F. Effects of protein kinase C stimulation and free Ca2+ rise in mammalian egg activation. Gamete Res. 1989 Oct;24(2):171-83.
Tang Z, Xing F, Chen D, et al. In vivo toxicological evaluation of Anisomycin. Toxicol Lett. 2012 Jan 5;208(1):1-11.
Regueira TB, Kildegaard KR, Hansen BG, Mortensen UH, Hertweck C, Nielsen J. Molecular basis for mycophenolic acid biosynthesis in Penicillium brevicompactum. Appl Environ Microbiol. 2011 May;77(9):3035-43.
K'ominek LA. Cycloheximide production by Streptomyces griseus: control mechanisms of cycloheximide biosynthesis. Antimicrob Agents Chemother. 1975 Jun;7(6):856-6.
Ultee A, Slump RA, Steging G, Smid EJ. Antimicrobial activity of carvacrol toward Bacillus cereus on rice. J Food Prot. 2000 May;63(5):620-4.
Pal PK, Nandi MK, Singh NK. Detoxification of Croton tiglium L. seeds by Ayurvedic process of Śodhana. Anc Sci Life. 2014 Jan;33(3):157-61.
Rotem R, Paz GF, Homonnai ZT, et al. Ca(2+)-independent induction of acrosome reaction by protein kinase C in human sperm. Endocrinology. 1992 Nov;131(5):2235-43.
de Lamirande E, Tsai C, Harakat A, Gagnon C. Involvement of reactive oxygen species in human sperm arcosome reaction induced by A23187, lysophosphatidylcholine, and biological fluid ultrafiltrates. J Androl. 1998 Sep-Oct;19(5):585-94.
Görlach A, Bertram K, Hudecova S, Krizanova O. Calcium and ROS: A mutual interplay. Redox Biol. 2015 Dec;6:260-71.
Nasr-Esfahani MM, Johnson MH. The origin of reactive oxygen species in mouse embryos cultured in vitro. Development. 1991 Oct;113(2):551-60.
LaRosa C, Downs SM. Stress stimulates AMP-activated protein kinase and meiotic resumption in mouse oocytes. Biol Reprod. 2006 Mar;74(3):585-92.
Calle-Guisado V, de Llera AH, Martin-Hidalgo D, et al. AMP-activated kinase in human spermatozoa: identification, intracellular localization, and key function in the regulation of sperm motility. Asian J Androl. 2016 Sep 27.
Mungai PT, Waypa GB, Jairaman A, et al. Hypoxia triggers AMPK activation through reactive oxygen species-mediated activation of calcium release-activated calcium channels. Mol Cell Biol. 2011 Sep;31(17):3531-45.
Tamas P, Hawley SA, Clarke RG, et al. Regulation of the energy sensor AMP-activated protein kinase by antigen receptor and Ca2+ in T lymphocytes. J Exp Med 2006;203(7):1665–70.
Hawley SA, Pan DA, Mustard KJ, et al. Calmodulin-dependent protein kinase kinase-beta is an alternative upstream kinase for AMP-activated protein kinase. Cell Metab. 2005 Jul;2(1):9-19.
Nammi S, Roufogalis BD. Light-to-moderate ethanol feeding augments AMPK-α phosphorylation and attenuates SREBP-1 expression in the liver of rats. J Pharm Pharm Sci. 2013;16(2):342-51.
Koh W, Jeong SJ, Lee HJ, et al. Melatonin promotes puromycin-induced apoptosis with activation of caspase-3 and 5'-adenosine monophosphate-activated kinase-alpha in human leukemia HL-60 cells. J Pineal Res. 2011 May;50(4):367-73.
Bays JL, Campbell HK, Heidema C, Sebbagh M, DeMali KA. Linking E-cadherin mechanotransduction to cell metabolism through force-mediated activation of AMPK. Nat Cell Biol. 2017 Jun;19(6):724-731.
Hutber CA, Hardie DG, Winder WW. Electrical stimulation inactivates muscle acetyl-CoA carboxylase and increases AMP-activated protein kinase. Am J Physiol. 1997 Feb;272(2 Pt 1):E262-6.
Ohsaka Y, Nishino H, Nomura Y. Induction of phospho-Thr-172 AMPK in 3T3-L1 adipocytes exposed to cold or treated with anisomycin, mithramycin A, and ionic compounds. Cryo Letters. 2010 May-Jun;31(3):218-29.
Fernández-Ramos AA, Marchetti-Laurent C, Poindessous V, et al. A comprehensive characterization of the impact of mycophenolic acid on the metabolism of Jurkat T cells. Sci Rep. 2017 Sep 5;7(1):10550.
Kim E, Choi Y, Jang J, Park T. Carvacrol Protects against Hepatic Steatosis in Mice Fed a High-Fat Diet by Enhancing SIRT1-AMPK Signaling. Evid Based Complement Alternat Med. 2013;2013:290104.
Zogovic N, Tovilovic-Kovacevic G, Misirkic-Marjanovic M, et al. Coordinated activation of AMP-activated protein kinase, extracellular signal-regulated kinase, and autophagy regulates phorbol myristate acetate-induced differentiation of SH-SY5Y neuroblastoma cells. J Neurochem. 2015 Apr;133(2):223-32.
Finley J. Oocyte activation and latent HIV-1 reactivation: AMPK as a common mechanism of action linking the beginnings of life and the potential eradication of HIV-1. Med Hypotheses. 2016 Aug;93:34-47.
Kitani S1, Miyamoto KT, Takamatsu S, et al. Avenolide, a Streptomyces hormone controlling antibiotic production in Streptomyces avermitilis. Proc Natl Acad Sci U S A. 2011 Sep 27;108(39):16410-5.
Challis GL, Hopwood DA. Synergy and contingency as driving forces for the evolution of multiple secondary metabolite production by Streptomyces species. Proc Natl Acad Sci U S A. 2003 Nov 25;100 Suppl 2:14555-61.
Yagüe P, López-García MT, Rioseras B, Sánchez J, Manteca A. Pre-sporulation stages of Streptomyces differentiation: state-of-the-art and future perspectives. FEMS Microbiol Lett. 2013 May;342(2):79-88.
Seipke RF, Kaltenpoth M, Hutchings MI. Streptomyces as symbionts: an emerging and widespread theme? FEMS Microbiol Rev. 2012 Jul;36(4):862-76.
Meng L, Li M, Yang SH, Kim TJ, Suh JW. Intracellular ATP levels affect secondary metabolite production in Streptomyces spp. Biosci Biotechnol Biochem. 2011;75(8):1576-81.
Sánchez S, Chávez A, Forero A, et al. Carbon source regulation of antibiotic production. J Antibiot (Tokyo). 2010 Aug;63(8):442-59.
Wang SL, Fan KQ, Yang X, Lin ZX, Xu XP, Yang KQ. CabC, an EF-hand calcium-binding protein, is involved in Ca2+-mediated regulation of spore germination and aerial hypha formation in Streptomyces coelicolor. J Bacteriol. 2008 Jun;190(11):4061-8.
Wang D, Wei L, Zhang Y, Zhang M, Gu S. Physicochemical and microbial responses of Streptomyces natalensis HW-2 to fungal elicitor. Appl Microbiol Biotechnol. 2017 Jul 28. doi: 10.1007/s00253-017-8440-0. [Epub ahead of print].
Wei ZH, Bai L, Deng Z, Zhong JJ. Enhanced production of validamycin A by H2O2-induced reactive oxygen species in fermentation of Streptomyces hygroscopicus 5008. Bioresour Technol. 2011 Jan;102(2):1783-7.
Doull JL, Ayer SW, Singh AK, Thibault P. Production of a novel polyketide antibiotic, jadomycin B, by Streptomyces venezuelae following heat shock. J Antibiot (Tokyo). 1993 May;46(5):869-71.
Zhou WW1, Ma B, Tang YJ, Zhong JJ, Zheng X. Enhancement of validamycin A production by addition of ethanol in fermentation of Streptomyces hygroscopicus 5008. Bioresour Technol. 2012 Jun;114:616-21.
Flanary BE, Kletetschka G. Analysis of telomere length and telomerase activity in tree species of various lifespans, and with age in the bristlecone pine Pinus longaeva. Rejuvenation Res. 2006 Spring;9(1):61-3.
R. S. BeasleyJ. O. Klemmedson. Recognizing site adversity and drought-sensitive trees in stands of bristlecone pine (Pinus longaeva). January 1973, Volume 27, Issue 1, pp 141–146. doi: 10.1007/BF02862228.
Schulman E. Longevity under Adversity in Conifers. Science. 1954 Mar 26;119(3091):396-9.
Hiebert, R. D.; Hamrick, J. L. 1984. An ecological study of bristlecone pine (Pinus longaeva) in Utah and eastern Nevada. The Great Basin Naturalist. 44(3): 487-494.
Jorquera MA, Shaharoona B, Nadeem SM, de la Luz Mora M, Crowley DE. Plant growth-promoting rhizobacteria associated with ancient clones of creosote bush (Larrea tridentata). Microb Ecol. 2012 Nov;64(4):1008-17.
Salminen A, Kaarniranta K. AMP-activated protein kinase (AMPK) controls the aging process via an integrated signaling network. Ageing Res Rev. 2012 Apr;11(2):230-41.
Leymarie J, Vitkauskaité G, Hoang HH, et al. Role of reactive oxygen species in the regulation of Arabidopsis seed dormancy. Plant Cell Physiol. 2012 Jan;53(1):96-106.
Pang X, Halaly T, Crane O, et al. Involvement of calcium signalling in dormancy release of grape buds. J Exp Bot. 2007;58(12):3249-62.
Duan Q, Kita D, Johnson EA, et al. Reactive oxygen species mediate pollen tube rupture to release sperm for fertilization in Arabidopsis. Nat Commun. 2014;5:3129.
Gao XQ, Liu CZ, Li DD, et al. The Arabidopsis KINβγ Subunit of the SnRK1 Complex Regulates Pollen Hydration on the Stigma by Mediating the Level of Reactive Oxygen Species in Pollen. PLoS Genet. 2016 Jul 29;12(7):e1006228.
Joo JH, Bae YS, Lee JS. Role of auxin-induced reactive oxygen species in root gravitropism. Plant Physiol. 2001 Jul;126(3):1055-60.
Lee JS, Mulkey TJ, Evans ML. Reversible loss of gravitropic sensitivity in maize roots after tip application of calcium chelators. Science. 1983 Jun 24;220(4604):1375-6.
Zhao J, Davis LC, Verpoorte R. Elicitor signal transduction leading to production of plant secondary metabolites. Biotechnol Adv. 2005 Jun;23(4):283-333.
Wang H, Sharma L, Lu J, Finch P, Fletcher S, Prochownik EV. Structurally diverse c-Myc inhibitors share a common mechanism of action involving ATP depletion. Oncotarget. 2015 Jun 30;6(18):15857-70.
K.V. Kiselev, O.A. Shumakova, A.Y. Manyakhin, A.N. Mazeika. Influence of calcium influx induced by the calcium ionophore, A23187, on resveratrol content and the expression of CDPK and STS genes in the cell cultures of Vitis amurensis. Plant Growth Regulation. December 2012, Volume 68, Issue 3, pp 371–381. DOI: 10.1007/s10725-012-9725-z.
Darwin, Charles (1859). On the origin of species by means of natural selection, or the preservation of favoured races in the struggle for life. London: Murray. [1st ed.].
Sobral M, Veiga T, Domínguez P, Guitián JA, Guitián P, Guitián JM. Selective Pressures Explain Differences in Flower Color among Gentiana lutea Populations. PLoS One. 2015 Jul 14;10(7):e0132522.
Lefebvre V, Kiani SP, Durand-Tardif M. A focus on natural variation for abiotic constraints response in the model species Arabidopsis thaliana. Int J Mol Sci. 2009 Aug 13;10(8):3547-82.
Moser TS, Schieffer D, Cherry S. AMP-activated kinase restricts Rift Valley fever virus infection by inhibiting fatty acid synthesis. PLoS Pathog. 2012;8(4):e1002661.
Goto A, Egawa T, Sakon I, et al. Heat stress acutely activates insulin-independent glucose transport and 5'-AMP-activated protein kinase prior to an increase in HSP72 protein in rat skeletal muscle. Physiol Rep. 2015 Nov;3(11). pii: e12601.
Maclean RC, Hall AR, Perron GG, Buckling A. The evolution of antibiotic resistance: insight into the roles of molecular mechanisms of resistance and treatment context. Discov Med. 2010 Aug;10(51):112-8.
Dwyer DJ, Belenky PA, Yang JH, et al. Antibiotics induce redox-related physiological alterations as part of their lethality. Proc Natl Acad Sci U S A. 2014 May 20;111(20):E2100-9.
Kohanski MA, DePristo MA, Collins JJ. Sublethal antibiotic treatment leads to multidrug resistance via radical-induced mutagenesis. Mol Cell. 2010 Feb 12;37(3):311-20.
Poole K. Bacterial stress responses as determinants of antimicrobial resistance. J Antimicrob Chemother. 2012 Sep;67(9):2069-89.
Ito M, Arakawa T, Okayama M, Shitara A, Mizoguchi I, Takuma T. Gravity loading induces adenosine triphosphate release and phosphorylation of extracellular signal-regulated kinases in human periodontal ligament cells. J Investig Clin Dent. 2014 Nov;5(4):266-74.
Bays JL, Campbell HK, Heidema C, Sebbagh M, DeMali KA. Linking E-cadherin mechanotransduction to cell metabolism through force-mediated activation of AMPK. Nat Cell Biol. 2017 Jun;19(6):724-731.
Zhong G, Li Y, Li H, et al. Simulated Microgravity and Recovery-Induced Remodeling of the Left and Right Ventricle. Front Physiol. 2016 Jun 29;7:274.
Chang TT, Walther I, Li CF, et al. The Rel/NF-jB pathway and transcription of immediate early genes in T cell activation are inhibited by microgravity. J Leukoc Biol 2012;92(6):1133–45.
Li YC, Chen BM, Wu PC, et al. Cutting Edge: mechanical forces acting on T cells immobilized via the TCR complex can trigger TCR signaling. J Immunol 2010;184(11):5959–63.
Ouyang Z, Wang X, Meng Q, et al. Suppression of adenosine monophosphate-activated protein kinase selectively triggers apoptosis in activated T cells and ameliorates immune diseases. Biochem Biophys Res Commun. 2017 May 27;487(2):223-229.
Blaber EA, Pecaut MJ, Jonscher KR. Spaceflight Activates Autophagy Programs and the Proteasome in Mouse Liver. Int J Mol Sci. 2017 Sep 27;18(10). pii: E2062.
Mo C, Wang L, Zhang J, et al. The crosstalk between Nrf2 and AMPK signal pathways is important for the anti-inflammatory effect of berberine in LPS-stimulated macrophages and endotoxin-shocked mice. Antioxid Redox Signal. 2014 Feb 1;20(4):574-88.
Joo MS, Kim WD, Lee KY, Kim JH, Koo JH, Kim SG. AMPK facilitates nuclear accumulation of Nrf2 by phosphorylating at serine 550. Mol Cell Biol. 2016 Jun 29;36(14):1931-42.
Zhang L, Yi Y, Guo Q, et al. Hsp90 interacts with AMPK and mediates acetyl-CoA carboxylase phosphorylation. Cell Signal. 2012 Apr;24(4):859-65.
Sagare-Patil V, Bhilawadikar R, Galvankar M, Zaveri K, Hinduja I, Modi D. Progesterone requires heat shock protein 90 (HSP90) in human sperm to regulate motility and acrosome reaction. J Assist Reprod Genet. 2017 Apr;34(4):495-503.
Lesniewski LA, Seals DR4, Walker AE, et al. Dietary rapamycin supplementation reverses age-related vascular dysfunction and oxidative stress, while modulating nutrient-sensing, cell cycle, and senescence pathways. Aging Cell. 2017 Feb;16(1):17-26.
Aparicio IM, Espino J, Bejarano I, et al. Autophagy-related proteins are functionally active in human spermatozoa and may be involved in the regulation of cell survival and motility. Sci Rep. 2016 Sep 16;6:33647.
Fang A, Pierson DL, Mishra SK, Demain AL. Growth of Steptomyces hygroscopicus in rotating-wall bioreactor under simulated microgravity inhibits rapamycin production. Appl Microbiol Biotechnol. 2000 Jul;54(1):33-6.
Cheng YR, Huang J, Qiang H, Lin WL, Demain AL. Mutagenesis of the rapamycin producer Streptomyces hygroscopicus FC904. J Antibiot (Tokyo). 2001 Nov;54(11):967-72.
Heindryckx B, Lierman S, Combelles CM, Cuvelier CA, Gerris J, De Sutter P. Aberrant spindle structures responsible for recurrent human metaphase I oocyte arrest with attempts to induce meiosis artificially. Hum Reprod. 2011 Apr;26(4):791-800.
Shkolnik K, Tadmor A, Ben-Dor S, Nevo N, Galiani D, Dekel N. Reactive oxygen species are indispensable in ovulation. Proc Natl Acad Sci U S A. 2011 Jan 25;108(4):1462-7.
Amdani SN, Jones C, Coward K. Phospholipase C zeta (PLC ζ): oocyte activation and clinical links to male factor infertility. Adv Biol Regul 2013;53(3):292–308.
Smith-Garvin JE, Koretzky GA, Jordan MS. T cell activation. Annu Rev Immunol 2009;27:591–619.
Spina CA, Anderson J, Archin NM, et al. An in-depth comparison of latent HIV-1 reactivation in multiple cell model systems and resting CD4+ T cells from aviremic patients. PLoS Pathog 2013;9(12):e1003834.
Marsden MD, Zack JA. HIV/AIDS eradication. Bioorg Med Chem Lett 2013;23(14):4003–10.
Sena LA, Li S, Jairaman A, et al. Mitochondria are required for antigen-specific T cell activation through reactive oxygen species signaling. Immunity. 2013 Feb 21;38(2):225-36.
Barquero AA, Dávola ME, Riva DA, Mersich SE, Alché LE. Naturally occurring compounds elicit HIV-1 replication in chronically infected promonocytic cells. Biomed Res Int. 2014;2014:989101.
Imai K, Ochiai K, Okamoto T. Reactivation of latent HIV-1 infection by the periodontopathic bacterium Porphyromonas gingivalis involves histone modification. J Immunol. 2009 Mar 15;182(6):3688-95.
Piette J, Legrand-Poels S. HIV-1 reactivation after an oxidative stress mediated by different reactive oxygen species. Chem Biol Interact. 1994 Jun;91(2-3):79-89.
Anderson I, Low JS, Weston S, et al. Heat shock protein 90 controls HIV-1 reactivation from latency. Proc Natl Acad Sci U S A. 2014 Apr 15;111(15):E1528-37.
Zhou H, Xu M, Huang Q, et al. Genome-scale RNAi screen for host factors required for HIV replication. Cell Host Microbe. 2008 Nov 13;4(5):495-504.
Wu H, Esteve E, Tremaroli V, et al. Metformin alters the gut microbiome of individuals with treatment-naive type 2 diabetes, contributing to the therapeutic effects of the drug. Nat Med. 2017 Jul;23(7):850-858.
G.M. Chew, D.C. Chow, S.A. Souza, et al. Impact of adjunctive metformin therapy on T cell exhaustion and viral persistence in a clinical trial of HIV-infected adults on suppressive ART. Journal of Virus Eradication 2017; 3 (Supplement 1): 6–19.
http://viruseradication.com/supplement-details/Abstracts_of_the_IAS_HIV_Cure_and_Cancer_Forum_2017/
Ullrich NJ, Gordon LB. Hutchinson-Gilford progeria syndrome. Handb Clin Neurol. 2015;132:249-64.
Berro R, Kehn K, de la Fuente C, et al. Acetylated Tat regulates human immunodeficiency virus type 1 splicing through its interaction with the splicing regulator p32. J Virol 2006;80(7):3189–204.
Paz S, Lu ML, Takata H, Trautmann L, Caputi M. SRSF1 RNA Recognition Motifs Are Strong Inhibitors of HIV-1 Replication. J Virol. 2015 Jun;89(12):6275-86.
Lopez-Mejia IC, Vautrot V, De Toledo M, et al. A conserved splicing mechanism of the LMNA gene controls premature aging. Hum Mol Genet. 2011 Dec 1;20(23):4540-55.
Park SK, Shin OS. Metformin alleviates ageing cellular phenotypes in Hutchinson-Gilford progeria syndrome dermal fibroblasts. Exp Dermatol. 2017 Oct;26(10):889-895.
Egesipe AL, Blondel S, Cicero AL, et al. Metformin decreases progerin expression and alleviates pathological defects of Hutchinson-Gilford progeria syndrome cells. NPJ Aging Mech Dis. 2016 Nov 10;2:16026.
Finley J. Alteration of splice site selection in the LMNA gene and inhibition of progerin production via AMPK activation. Med Hypotheses. 2014 Nov;83(5):580-7.
Decuypere JP, Welkenhuyzen K, Luyten T, et al. Ins(1,4,5)P3 receptor-mediated Ca2+ signaling and autophagy induction are interrelated. Autophagy. 2011 Dec;7(12):1472-89.
Cao Y, Fang Y, Cai J, et al. ROS functions as an upstream trigger for autophagy to drive hematopoietic stem cell differentiation. Hematology. 2016 Dec;21(10):613-618.
Decuypere JP, Kindt D, Luyten T, et al. mTOR-Controlled Autophagy Requires Intracellular Ca(2+) Signaling. PLoS One. 2013;8(4):e61020.
Cao K, Graziotto JJ, Blair CD, et al. Rapamycin reverses cellular phenotypes and enhances mutant protein clearance in Hutchinson-Gilford progeria syndrome cells. Sci Transl Med. 2011 Jun 29;3(89):89ra58.
Gabriel D, Gordon LB, Djabali K. Temsirolimus Partially Rescues the Hutchinson-Gilford Progeria Cellular Phenotype. PLoS One. 2016 Dec 29;11(12):e0168988.
Moncharmont C, Levy A, Gilormini M, et al. Targeting a cornerstone of radiation resistance: cancer stem cell. Cancer Lett 2012;322(2):139–47.
Finley J. Elimination of cancer stem cells and reactivation of latent HIV-1 via AMPK activation: Common mechanism of action linking inhibition of tumorigenesis and the potential eradication of HIV-1. Med Hypotheses. 2017 Jul;104:133-146.
Buzon MJ, Sun H, Li C, et al. HIV-1 persistence in CD4+ T cells with stem cell-like properties. Nat Med 2014;20(2):139–42.
Wee S, Niklasson M, Marinescu VD, et al. Selective calcium sensitivity in immature glioma cancer stem cells. PLoS One. 2014 Dec 22;9(12):e115698.
Hayun M, Okun E, Hayun R, Gafter U, et al. Synergistic effect of AS101 and Bryostatin-1 on myeloid leukemia cell differentiation in vitro and in an animal model. Leukemia 2007;21(7):1504–13.
Sato A, Sunayama J, Okada M, et al. Glioma-initiating cell elimination by metformin activation of FOXO3 via AMPK. Stem Cells Transl Med 2012;1(11):811–24.
Fasih A, Elbaz HA, Hüttemann M, Konski AA, Zielske SP. Radiosensitization of pancreatic cancer cells by metformin through the AMPK pathway. Radiat Res 2014;182(1):50–9.
#ampk#gut bacteria#metformin#oocyte#HIV/AIDS#progeria#cancer stem cell#evolution#charles darwin#sperm#acrosome reaction#fertilization#reproduction
1 note
·
View note
Text
AMPK activator Metformin shown for the first time to destabilize latent HIV-1 reservoir in chronic HIV-1 patients & lower immune checkpoint PD-1
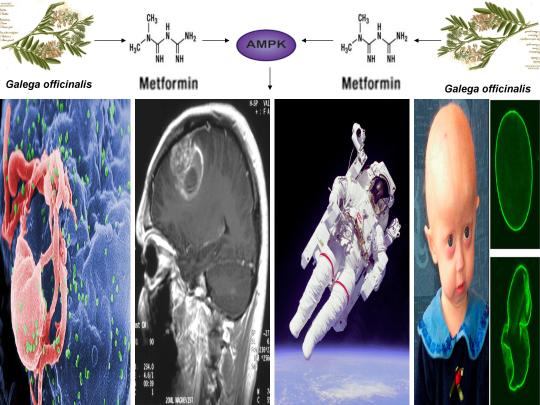
Goldsmith Content Providers: CDC/ C. Goldsmith, P. Feorino, E. L. Palmer, W. R. McManus [Public domain], via Wikimedia Commons; CC BY 2.5 (http://creativecommons.org/licenses/by/2.5)], via Wikimedia Commons; By NASA [Public domain], via Wikimedia
At the International AIDS Society’s (IAS) HIV Cure and Cancer Forum held in Paris, France in July of 2017, researchers from the University of Hawaii demonstrated for the first time that the anti-diabetic drug and AMPK activator metformin not only decreased the percentage of CD4+ T cells expressing the immune checkpoint receptors PD-1, TIGIT, and TIM-3 but also destabilized the viral reservoir in chronically-infected HIV-1 patients, indicating that metformin may indeed contribute to HIV-1 eradication by reactivating CD4+ T cells latently-infected with HIV-1 [1-3]. Current antiretroviral drugs selectively targets only replicating viruses capable of inducing viral gene expression in activated CD4+ T cells [4]. However, a method known as the “shock and kill” approach in HIV-1 cure research involves the reactivation of a small subset of primarily CD4+ memory T cells latently infected with HIV-1 (dormant) with various agents/drugs (i.e. “shock”) and is thought to facilitate virus-induced cell death or immune recognition and destruction of the virus (i.e. “kill”) [4]. Immune checkpoint receptors including PD-1 are considered markers of T cell exhaustion and have also previously been found to be positively associated with T cells that harbor latent HIV-1 [5,6].
As the inhibition of PD-1/PD-L1 significantly enhances CD8+ T cell-mediated immunological responses to both viruses and cancer cells, the finding that metformin decreases PD-1 and destabilizes the latent viral reservoir in chronically-infected HIV-1 patients provides additional evidence and supports several recent publications in which I proposed for the first time that AMPK, which is critical for T cell activation, links the reactivation of latent HIV-1 with the differentiation and/or apoptosis of cancer stem cells and the amelioration of accelerated cellular aging defects in Hutchinson-Gilford progeria syndrome (HGPS) [4,7-10]. Because metformin has recently been shown to improve accelerated aging defects in cells derived from HGPS patients, decrease PD-1 expression on T cells, and potently induce cancer stem cell differentiation and/or apoptosis (e.g. glioblastoma), AMPK activation may indeed lead to the inhibition of tumorigenesis, viral eradication, and mitigation of accelerated aging [1,11-13].
At the IAS HIV Cure and Cancer Forum, Chew et al. presented data from an open label, 24 week clinical trial in which metformin (500 mg increasing to 1000 mg at week 4) was administered to 12 HIV-1 positive patients who were on antiretroviral therapy for greater than a year with plasma HIV RNA less than 50 copies/ml [1]. A non-metformin treated observational arm was also included. Compared to the observational arm, metformin-treated patients exhibited a significant 24 week decease in the change of single positive PD-1+, dual expressing TIGIT+PD-1+, and triple expressing TIGIT+PD-1+TIM-3+ CD4 T cells [1]. Strikingly, although integrated HIV DNA (an indicator of HIV-1 latency) in T cells remained remarkably stable in the 3 subjects in the observation arm, a variance in integrated HIV DNA was observed in metformin-treated patients, indicating that the viral reservoir had been destabilized. Interestingly, the authors also noted a statistically significant decrease in CD32a in metformin-treated patients [1]. CD32a is a surface receptor protein in immune cells that has recently been demonstrated as a reliable marker of CD4+ T cell reservoirs in HIV-1 infected patients harboring replication-competent proviruses, providing compelling evidence that metformin-induced AMPK activation may promote viral eradication by inducing reactivation of latent HIV-1, as evidenced by reductions in PD-1, TIGIT, TIM-3, and CD32a, all markers of CD4+ T cell latent HIV-1 reservoirs [1,14].
In addition to inducing latent HIV-1 reactivation, metformin-induced AMPK activation may also mitigate T cell exhaustion and enhance the immunological response of cytotoxic CD8+ T cells to viruses and cancer cells. Indeed, PD-L1, a ligand that binds to the PD-1 receptor on T cells, has been found on breast and colon cancer stem cells and metformin, AICAR (an AMPK activator), and rapamycin (a macrolide that activates AMPK in vivo) have each been shown to decrease PD-L1 expression on human lung cancer cells [15-18]. Intriguingly, metformin has also been shown to improve intratumoral T cell function and tumor clearance in mice by potentiating PD-1 blockade and significantly increasing the number of activated CD8+ T cells [19].
Moreover, as AMPK is critical for T cell activation and AMPK inhibition during T cell activation leads to T cell death, recent studies indicating that AMPK activators decrease PD-1 expression on T cells and enhance CD8+ T cell-mediated immunological responses strongly suggests that AMPK represents a central node linking latent HIV-1 reactivation with T cell-mediated virus and cancer cell elimination (i.e. immunotherapy) [20]. Eikawa et al. for example showed that metformin enabled normal mice (but not T cell-deficient mice) to reject solid tumors by increasing the number of CD8+ tumor-infiltrating lymphocytes (TILs) and protecting them from exhaustion in an AMPK-dependent manner [21]. Perhaps most convincingly, Chamoto et al. demonstrated in a model using mouse colon cancer MC38 cells that PD-1 blockade (using a PD-L1 inhibitor) led to activation of mitochondria in tumor-reactive cytotoxic CD8+ T lymphocytes, evidenced by increased mitochondrial ROS (i.e. superoxide), larger mitochondrial mass, and higher mitochondrial membrane potential [22].
As mitochondria-derived ROS have previously been shown to be critical, if not indispensable for T cell activation, the combination of a PD-L1 inhibitor/mAb with Luperox (a ROS precursor) greatly enhanced the antitumor activity and survival of tumor-bearing mice [22,23]. Interestingly, the mitochondrial uncouplers FCCP and DNP also extended the survival time of PD-L1 mAb-treated animals, which was mitigated by the ROS scavenger MnTBAP [22]. As ROS have been independently shown to induce AMPK activation and AMPK is essential for T cell activation, the PD-L1 mAb alone induced AMPK activation and the combination of mitochondrial uncouplers with the PD-L1 mAb also further enhanced AMPK activation in CD8+ T cells, indicating that AMPK is essential for enhanced CD8+ T cell activation by PD-1 blockade, ROS generators, and mitochondrial uncouplers [4,22]. Indeed, the authors also showed that the AMPK activator A769662 further enhanced the antitumor activity by PD-L1 mAb treatment and improved animal survival. Additionally, oltipraz and bezafibrate, two compounds that also activate AMPK, strongly enhanced tumor-growth suppression and animal-survival by anti–PD-L1 treatment [22,24,25].
Such data, combined with the recent findings from the IAS HIV Cure and Cancer forum showing that metformin decreases the percentage of CD4+ T cells expressing PD-1, TIGIT, and TIM-3 and destabilizes the viral reservoir in chronically-infected HIV-1 patients, indicates that AMPK activation likely links the reactivation of latent HIV-1 with the facilitation of CD8+ T cell-mediated virus and cancer cell killing, potentially leading to HIV-1 eradication and cancer stem cell elimination, a hypothesis I initially proposed in May of 2017 [4].
Furthermore, AMPK activation also promotes oocyte meiotic induction and maturation (processes that are critical for efficient oocyte activation) and AMPK has recently been found localized across the entire acrosome in human spermatozoa [10,26,27]. The induction of cellular stress (e.g. increases in ROS, intracellular Ca2+, and/or AMP(ADP)/ATP ratio increase), which activates AMPK, also promotes oocyte meiotic induction/maturation, oocyte activation, and the acrosome reaction in human sperm, processes critical for the creation of all human life [26,28,29]. Indeed, the calcium ionophore ionomycin, which activates AMPK, is commonly used to promote latent HIV-1 reactivation and is extensively used to activate human oocytes, creating normal healthy children [29-31]. Such evidence further substantiates the novel and provocative assertion that AMPK activation links the amelioration of pathological cellular defects in Hutchinson-Gilford progeria syndrome with HIV-1 latency, adult and cancer stem cells, learning and memory, and the creation of all human life [4,8-10,32].
https://www.linkedin.com/pulse/ampk-activator-metformin-shown-first-time-destabilize-finley

References
G.M. Chew, D.C. Chow, S.A. Souza, et al. Impact of adjunctive metformin therapy on T cell exhaustion and viral persistence in a clinical trial of HIV-infected adults on suppressive ART. Journal of Virus Eradication 2017; 3 (Supplement 1): 6–19.
http://viruseradication.com/supplement-details/Abstracts_of_the_IAS_HIV_Cure_and_Cancer_Forum_2017/
http://www.iasociety.org/HIV-Programmes/Towards-an-HIV-Cure/Events/HIV-Cure-Cancer-Forum
Finley J. Elimination of cancer stem cells and reactivation of latent HIV-1 via AMPK activation: Common mechanism of action linking inhibition of tumorigenesis and the potential eradication of HIV-1. Med Hypotheses. 2017 Jul;104:133-146.
Fromentin R, Bakeman W, Lawani MB, et al. CD4+ T Cells Expressing PD-1, TIGIT and LAG-3 Contribute to HIV Persistence during ART. PLoS Pathog. 2016 Jul 14;12(7):e1005761.
Chew GM, Fujita T, Webb GM, et al. TIGIT Marks Exhausted T Cells, Correlates with Disease Progression, and Serves as a Target for Immune Restoration in HIV and SIV Infection. PLoS Pathog. 2016 Jan 7;12(1):e1005349.
Sakthivel P, Gereke M, Bruder D. Therapeutic intervention in cancer and chronic viral infections: antibody mediated manipulation of PD-1/PD-L1 interaction. Rev Recent Clin Trials. 2012 Feb;7(1):10-23.
Finley J. Alteration of splice site selection in the LMNA gene and inhibition of progerin production via AMPK activation. Med Hypotheses. 2014 Nov;83(5):580-7.
Finley J. Reactivation of latently infected HIV-1 viral reservoirs and correction of aberrant alternative splicing in the LMNA gene via AMPK activation: Common mechanism of action linking HIV-1 latency and Hutchinson-Gilford progeria syndrome. Med Hypotheses. 2015 Sep;85(3):320-32.
Finley J. Oocyte activation and latent HIV-1 reactivation: AMPK as a common mechanism of action linking the beginnings of life and the potential eradication of HIV-1. Med Hypotheses. 2016 Aug;93:34-47.
Park SK, Shin OS. Metformin alleviates ageing cellular phenotypes in Hutchinson-Gilford progeria syndrome dermal fibroblasts. Exp Dermatol. 2017 Feb 13. doi: 10.1111/exd.13323. [Epub ahead of print].
Egesipe AL, Blondel S, Cicero AL, et al. Metformin decreases progerin expression and alleviates pathological defects of Hutchinson-Gilford progeria syndrome cells. NPJ Aging Mech Dis. 2016 Nov 10;2:16026.
Sato A, Sunayama J, Okada M, et al. Glioma-initiating cell elimination by metformin activation of FOXO3 via AMPK. Stem Cells Transl Med 2012;1(11):811–24.
Descours B, Petitjean G, López-Zaragoza JL, et al. CD32a is a marker of a CD4 T-cell HIV reservoir harbouring replication-competent proviruses. Nature. 2017 Mar 23;543(7646):564-567.
Wu Y, Chen M, Wu P, Chen C, Xu ZP, Gu W. Increased PD-L1 expression in breast and colon cancer stem cells. Clin Exp Pharmacol Physiol. 2017 May;44(5):602-604.
Kurimoto R, Iwasawa S, Ebata T, et al. Drug resistance originating from a TGF-β/FGF-2-driven epithelial-to-mesenchymal transition and its reversion in human lung adenocarcinoma cell lines harboring an EGFR mutation. Int J Oncol. 2016 May;48(5):1825-36.
Lastwika KJ, Wilson W 3rd, Li QK, et al. Control of PD-L1 Expression by Oncogenic Activation of the AKT-mTOR Pathway in Non-Small Cell Lung Cancer. Cancer Res. 2016 Jan 15;76(2):227-38.
Chiao YA, Kolwicz SC, Basisty N, et al. Rapamycin transiently induces mitochondrial remodeling to reprogram energy metabolism in old hearts. Aging (Albany NY). 2016 Feb;8(2):314-27.
Scharping NE, Menk AV, Whetstone RD, Zeng X, Delgoffe GM. Efficacy of PD-1 Blockade Is Potentiated by Metformin-Induced Reduction of Tumor Hypoxia. Cancer Immunol Res. 2017 Jan;5(1):9-16.
Rao E, Zhang Y, Zhu G, et al. Deficiency of AMPK in CD8+ T cells suppresses their anti-tumor function by inducing protein phosphatase-mediated cell death. Oncotarget 2015;6(10):7944–58.
Eikawa S, Nishida M, Mizukami S, Yamazaki C, Nakayama E, Udono H. Immune-mediated antitumor effect by type 2 diabetes drug, metformin. Proc Natl Acad Sci U S A. 2015 Feb 10;112(6):1809-14.
Chamoto K, Chowdhury PS, Kumar A, et al. Mitochondrial activation chemicals synergize with surface receptor PD-1 blockade for T cell-dependent antitumor activity. Proc Natl Acad Sci U S A. 2017 Jan 31;114(5):E761-E770.
Sena LA, Li S, Jairaman A, et al. Mitochondria are required for antigen-specific T cell activation through reactive oxygen species signaling. Immunity. 2013 Feb 21;38(2):225-36.
Zhong X, Xiu LL, Wei GH, et al. Bezafibrate enhances proliferation and differentiation of osteoblastic MC3T3-E1 cells via AMPK and eNOS activation. Acta Pharmacol Sin. 2011 May;32(5):591-600.
Kim TH, Eom JS, Lee CG, Yang YM, Lee YS, Kim SG. An active metabolite of oltipraz (M2) increases mitochondrial fuel oxidation and inhibits lipogenesis in the liver by dually activating AMPK. Br J Pharmacol. 2013 Apr;168(7):1647-61.
LaRosa C, Downs SM. Stress stimulates AMP-activated protein kinase and meiotic resumption in mouse oocytes. Biol Reprod. 2006 Mar;74(3):585-92.
Calle-Guisado V, de Llera AH, Martin-Hidalgo D, et al. AMP-activated kinase in human spermatozoa: identification, intracellular localization, and key function in the regulation of sperm motility. Asian J Androl. 2016 Sep 27. doi: 10.4103/1008-682X.185848. [Epub ahead of print].
de Lamirande E, Tsai C, Harakat A, Gagnon C. Involvement of reactive oxygen species in human sperm arcosome reaction induced by A23187, lysophosphatidylcholine, and biological fluid ultrafiltrates. J Androl. 1998 Sep-Oct;19(5):585-94.
Deemeh MR, Tavalaee M, Nasr-Esfahani MH. Health of children born through artificial oocyte activation: a pilot study. Reprod Sci. 2015 Mar;22(3):322-8.
Tamás P, Hawley SA, Clarke RG, et al. Regulation of the energy sensor AMP-activated protein kinase by antigen receptor and Ca2+ in T lymphocytes. J Exp Med. 2006 Jul 10;203(7):1665-70.
Spina CA, Anderson J, Archin NM, et al. An in-depth comparison of latent HIV-1 reactivation in multiple cell model systems and resting CD4+ T cells from aviremic patients. PLoS Pathog 2013;9(12):e1003834.
Finley J. Facilitation of hippocampal long-term potentiation and reactivation of latent HIV-1 via AMPK activation: Common mechanism of action linking learning, memory, and the potential eradication of HIV-1. Med Hypotheses. Manuscript submitted.
0 notes
Text
AMPK activators Metformin & CHIR99021 improve gut bacteria in humans, Fragile X, & promote inner ear, dental pulp, & cancer stem cell differentiation

CC BY 2.5 (http://creativecommons.org/licenses/by/2.5)], via Wikimedia Commons; By Peter Saxon (Own work) [CC BY-SA 4.0 (http://creativecommons.org/licenses/by-sa/4.0)], via Wikimedia Commons; Rocky Mountain Laboratories, NIAID, NIH [Public domain]
A recent study published online in the journal Nature Medicine in May of 2017 presented startling evidence that the AMPK activator metformin, which has also recently been shown to alleviate accelerated aging defects in cells derived from Hutchinson-Gilford progeria (HGPS) patients, exerted a significant beneficial effect on the gut microbiome in humans in a randomized double-blind placebo controlled study [1-4]. In treatment-naïve patients with Type 2 diabetes (placebo: n = 18 or 1,700 mg/d of metformin: n = 22), a significant decrease in hemoglobin A1c (HbA1c) levels and fasting blood glucose was observed only in metformin-treated patients during the 4-month study period [1]. HbA1c levels and fasting blood glucose were also significantly reduced in a subset of placebo-treated patients that were switched to metformin after 6 months of treatment. Interestingly, whole-genome shotgun sequencing of fecal samples indicated that metformin treatment for 2 and 4 months significantly altered the relative abundance of 81 and 86 bacterial strains, respectively, with an increase in Bifidobacterium and Akkermansia muciniphila [1]. Metformin also directly promoted the growth of Bifidobacterium adolescentis and A. muciniphila in vitro, both of which have been associated with improved metabolic features in mice [1].
Importantly, fecal samples from humans that had been treated with metformin for 4 months and transferred to germ-free mice (via oral gavage) fed a high-fat diet improved glucose tolerance in mice compared to mice that received fecal samples from humans before treatment with metformin [1]. Intriguingly, metformin treatment was also linked to gene enrichment for bacterial environmental responses, including metabolism of the short-chain fatty acids and AMPK activators butyrate and propionate [1,5]. Fecal propionate and butyrate concentrations were significantly increased after 4 months of metformin treatment in men compared to the placebo group, indicating that metformin also beneficially modulates bacterial secondary metabolite production by inducing a bacterial stress response [1]. Moreover, several environmental stressors, including heat shock/stress, which activates AMPK in human cells, also promotes the production of various bacterial secondary metabolites, indicating that the mechanism of action by which metformin promotes an increase in beneficial human gut bacteria and release of bacterial secondary metabolites is via the induction of a stress response in bacteria [6,7].
Furthermore, the beneficial effects of the induction of a cellular stress response likely crosses species boundaries, as increases in calcium (Ca2+) and reactivate oxygen species (ROS) (mediators of cellular stress induction) also promotes seed germination, root gravitropism, and fertilization in plants [8-13]. Additionally, an increase in the AMP(ADP)/ATP ratio, intracellular Ca2+ increases, or an increase in the levels of ROS have been shown to activate the master metabolic regulator AMPK and promote the differentiation of embryonic, adult, and cancer stem cells [14-18]. Metformin and butyrate have also been shown to synergistically activate AMPK and decrease the cancer stem cell-like population in breast cancer cells, butyrate has been shown to induce pancreatic cancer stem cell differentiation, and metformin induces glioma stem cell differentiation and elimination in an AMPK-dependent manner, indicating that cellular stress-induced AMPK activation is a critical mediator linking cancer, embryonic, and adult stem cell differentiation, as proposed in my recent publication linking cancer stem cell differentiation and/or apoptosis with latent HIV-1 reactivation [19-21].
Indeed, a recent study published in the journal Cell Reports by researchers from Harvard Medical School and MIT showed that the glycogen synthase kinase 3β (GSK3β) inhibitor CHIR99021 (CHIR) and the histone deacetylase (HDAC) inhibitor valproic acid (VPA), both of which activate AMPK, significantly expanded cochlear supporting cells (i.e. “inner ear stem cells”) that expressed and maintained the epithelial stem cell marker Lgr5 [22-24]. Treatment with CHIR and VPA also led to the differentiation of Lgr5-expressing cells into hair cells in high yield, providing additional evidence that AMPK activation promotes differentiation of adult stem cells including inner ear stem cells, possibly leading to treatments for hearing loss [24]. Interestingly, the authors demonstrated in a previous study that CHIR and VPA also promoted the multilineage differentiation of Lgr5+ intestinal stem cells into mature enterocytes, goblet cells and Paneth cells [25]. AMPK activation has also been shown to improve gut epithelial differentiation and metformin increases goblet and Paneth cell differentiation from intestinal epithelial cells, further indicating that AMPK activation likely represents a common mechanism of action linking structurally dissimilar compounds that enhance inner ear and intestinal stem cell maintenance and differentiation [26,27].
Moreover, a recently published study in the journal Scientific Reports in January of 2017 demonstrated that topical administration of GSK3β inhibitors including the AMPK activator CHIR led to the mobilization of resident mesenchymal stem cells in the tooth pulp that had been exposed via the drilling of holes in mice molars [28]. GSK3β inhibitor-induced stem cell mobilization promoted a natural process of reparative dentin (also spelled dentine) formation that completely restored dentin, leading the authors to conclude that stimulation of mesenchymal stem cell mobilization and differentiation into odontoblast-like cells may represent a novel approach to clinical tooth restoration [28]. AMPK activation has previously been shown to promote osteogenic (i.e. bone forming) differentiation of human adipose tissue-derived mesenchymal stem cells and metformin induces osteoblastic differentiation of human induced pluripotent stem cell-derived mesenchymal stem cells in an AMPK-dependent manner, providing further evidence that structurally diverse compounds including metformin and CHIR that promote adult stem cell differentiation likely do so via a common mechanism of AMPK activation [29,30].
The induction of cellular stress and AMPK activation may also link beneficial modulation of the gut microbiome in humans not only with adult stem cell maintenance and differentiation, but also with the amelioration of pathologies associated with neurological disorders. A study recently published in the journal Clinical Genetics in April of 2017 demonstrated for the first time that metformin consistently improved behavior in several patients diagnosed with Fragile X Syndrome (FXS), a genetic disorder characterized by intellectual disability and significant deficits in neurological function and cognitive development [31]. An improvement in behavior was documented in the Aberrant Behavior Checklist (ABC) for all cases, as evidenced by consistent improvements (i.e. lower scores compared to pre-metformin treatment) in social avoidance, irritability, hyperactivity, and social unresponsiveness as well as improvements in language and conversational skills reported by familial caretakers [31].
Also, metformin has been shown to rescue and restore memory deficits in a Drosophila model of FXS and a recently published study (2017) demonstrated that metformin corrected social novelty impairment, reduced testicular weight, decreased repetitive grooming, rescued excessive long-term depression and dendritic spine abnormalities, restored excitatory synaptic transmission, and acutely activated AMPK in hippocampal pyramidal neurons in an FXS mouse model [32,33]. Interestingly, GSK3β inhibitors including the AMPK activator CHIR have been shown to rescue deficits in long-term potentiation at medial perforant path-dentate granule cells synapses in an FXS mouse model, indicating that cellular stress-induced AMPK activation by metformin and CHIR links the beneficial effects of those compounds in phenomena as disparate as stem cell differentiation, FXS, and long-term potentiation, hypotheses that I initially proposed in 2017 [34-36].
Lastly, as butyrate has been shown to reactivate latent HIV-1, facilitating immune system detection and virus destruction, and metformin when combined with bryostatin-1 (which also activates AMPK) promotes latent HIV-1 reactivation, cellular stress-induced AMPK activation likely also links beneficial modulation of human gut bacteria with latent HIV-1 reactivation [37-39].
AMPK activation also promotes oocyte meiotic induction and maturation (processes that are critical for efficient oocyte activation) and AMPK has recently been found localized across the entire acrosome in human spermatozoa [40-42]. The induction of cellular stress (e.g. increases in ROS, intracellular Ca2+, and/or AMP(ADP)/ATP ratio increase), which activates AMPK, also promotes oocyte meiotic induction/maturation, oocyte activation, and the acrosome reaction in human sperm, processes critical for the creation of all human life [41,43,44]. Indeed, the calcium ionophore ionomycin, which activates AMPK, is commonly used to promote latent HIV-1 reactivation and is extensively used to activate human oocytes, creating normal healthy children [44-46]. Such evidence indicates and further substantiates the novel and provocative assertion that AMPK activation links the amelioration of pathological cellular defects in FXS and Hutchinson-Gilford progeria syndrome with the gut microbiota, HIV-1 latency, adult and cancer stem cells, learning and memory, and the creation of all human life [4,35,36,39,40,47].

https://www.linkedin.com/pulse/ampk-activators-metformin-chir99021-improve-gut-bacteria-finley
References
Wu H, Esteve E, Tremaroli V, et al. Metformin alters the gut microbiome of individuals with treatment-naive type 2 diabetes, contributing to the therapeutic effects of the drug. Nat Med. 2017 Jul;23(7):850-858.
Egesipe AL, Blondel S, Cicero AL, et al. Metformin decreases progerin expression and alleviates pathological defects of Hutchinson-Gilford progeria syndrome cells. NPJ Aging Mech Dis. 2016 Nov 10;2:16026.
Park SK, Shin OS. Metformin alleviates ageing cellular phenotypes in Hutchinson-Gilford progeria syndrome dermal fibroblasts. Exp Dermatol. 2017 Feb 13. doi: 10.1111/exd.13323. [Epub ahead of print].
Finley J. Alteration of splice site selection in the LMNA gene and inhibition of progerin production via AMPK activation. Med Hypotheses. 2014 Nov;83(5):580-7.
Elamin EE, Masclee AA, Dekker J, Pieters HJ, Jonkers DM. Short-chain fatty acids activate AMP-activated protein kinase and ameliorate ethanol-induced intestinal barrier dysfunction in Caco-2 cell monolayers. J Nutr. 2013 Dec;143(12):1872-81.
Yoon V, Nodwell JR. Activating secondary metabolism with stress and chemicals. J Ind Microbiol Biotechnol. 2014 Feb;41(2):415-24.
Lee H, Park HJ, Park CS, et al. Response of breast cancer cells and cancer stem cells to metformin and hyperthermia alone or combined. PLoS One. 2014 Feb 5;9(2):e87979.
Leymarie J, Vitkauskaité G, Hoang HH, et al. Role of reactive oxygen species in the regulation of Arabidopsis seed dormancy. Plant Cell Physiol. 2012 Jan;53(1):96-106.
Pang X, Halaly T, Crane O, et al. Involvement of calcium signalling in dormancy release of grape buds. J Exp Bot. 2007;58(12):3249-62.
Krieger G, Shkolnik D, Miller G, Fromm H. Reactive Oxygen Species Tune Root Tropic Responses. Plant Physiol. 2016 Oct;172(2):1209-1220.
Plieth C, Trewavas AJ. Reorientation of seedlings in the earth's gravitational field induces cytosolic calcium transients. Plant Physiol. 2002 Jun;129(2):786-96.
Denninger P, Bleckmann A, Lausser A, et al. Male-female communication triggers calcium signatures during fertilization in Arabidopsis. Nat Commun. 2014 Aug 22;5:4645.
Duan Q, Kita D, Johnson EA, et al. Reactive oxygen species mediate pollen tube rupture to release sperm for fertilization in Arabidopsis. Nat Commun. 2014;5:3129.
Hardie DG. AMP-activated protein kinase: an energy sensor that regulates all aspects of cell function. Genes Dev. 2011 Sep 15;25(18):1895-908.
Sook SH, Lee HJ, Kim JH, et al. Reactive oxygen species-mediated activation of AMP-activated protein kinase and c-Jun N-terminal kinase plays a critical role in beta-sitosterol-induced apoptosis in multiple myeloma U266 cells. Phytother Res. 2014 Mar;28(3):387-94.
Ji AR, Ku SY, Cho MS, et al. Reactive oxygen species enhance differentiation of human embryonic stem cells into mesendodermal lineage. Exp Mol Med. 2010 Mar 31;42(3):175-86.
Sun S, Liu Y, Lipsky S, Cho M. Physical manipulation of calcium oscillations facilitates osteodifferentiation of human mesenchymal stem cells. FASEB J. 2007 May;21(7):1472-80.
Wee S, Niklasson M2, Marinescu VD, et al. Selective calcium sensitivity in immature glioma cancer stem cells. PLoS One. 2014 Dec 22;9(12):e115698.
Lee KM, Lee M, Lee J, et al. Enhanced anti-tumor activity and cytotoxic effect on cancer stem cell population of metformin-butyrate compared with metformin HCl in breast cancer. Oncotarget. 2016 Jun 21;7(25):38500-38512.
Lang D, Mascarenhas JB, Powell SK, Halegoua J, Nelson M, Ruggeri BA. PAX6 is expressed in pancreatic adenocarcinoma and is downregulated during induction of terminal differentiation. Mol Carcinog 2008;47(2):148–56.
Sato A, Sunayama J, Okada M, et al. Glioma-initiating cell elimination by metformin activation of FOXO3 via AMPK. Stem Cells Transl Med 2012;1(11):811–24.
Suzuki T, Bridges D, Nakada D, et al. Inhibition of AMPK catabolic action by GSK3. Mol Cell. 2013 May 9;50(3):407-19.
Avery LB, Bumpus NN. Valproic acid is a novel activator of AMP-activated protein kinase and decreases liver mass, hepatic fat accumulation, and serum glucose in obese mice. Mol Pharmacol. 2014 Jan;85(1):1-10.
McLean WJ, Yin X, Lu L, et al. Clonal Expansion of Lgr5-Positive Cells from Mammalian Cochlea and High-Purity Generation of Sensory Hair Cells. Cell Rep. 2017 Feb 21;18(8):1917-1929.
Yin X, Farin HF, van Es JH, et al. Niche-independent high-purity cultures of Lgr5+ intestinal stem cells and their progeny. Nat Methods. 2014 Jan;11(1):106-12.
Sun X, Yang Q, Rogers CJ, Du M, Zhu MJ. AMPK improves gut epithelial differentiation and barrier function via regulating Cdx2 expression. Cell Death Differ. 2017 May;24(5):819-831.
Xue Y, Zhang H, Sun X, Zhu MJ. Metformin Improves Ileal Epithelial Barrier Function in Interleukin-10 Deficient Mice. PLoS One. 2016 Dec 21;11(12):e0168670.
Neves VC, Babb R, Chandrasekaran D, Sharpe PT. Promotion of natural tooth repair by small molecule GSK3 antagonists. Sci Rep. 2017 Jan 9;7:39654.
Kim EK, Lim S, Park JM, et al. Human mesenchymal stem cell differentiation to the osteogenic or adipogenic lineage is regulated by AMP-activated protein kinase. J Cell Physiol. 2012 Apr;227(4):1680-7.
Wang P, Ma T, Guo D, et al. Metformin Induces Osteoblastic Differentiation of Human Induced Pluripotent Stem Cell-derived Mesenchymal Stem Cells. J Tissue Eng Regen Med. 2017 May 11. doi: 10.1002/term.2470. [Epub ahead of print].
Dy ABC, Tassone F, Eldeeb M, Salcedo-Arellano MJ, Tartaglia N, Hagerman R. Metformin as Targeted Treatment in Fragile X Syndrome. Clin Genet. 2017 Apr 24. doi: 10.1111/cge.13039. [Epub ahead of print].
Monyak RE, Emerson D, Schoenfeld BP, et al. Insulin signaling misregulation underlies circadian and cognitive deficits in a Drosophila fragile X model. Mol Psychiatry. 2017 Aug;22(8):1140-1148.
Gantois I, Khoutorsky A, Popic J, et al. Metformin ameliorates core deficits in a Fragile X syndrome mouse model. Nat Med. 2017 Jun;23(6):674-677.
Franklin AV, King MK, Palomo V, Martinez A, McMahon LL, Jope RS. Glycogen synthase kinase-3 inhibitors reverse deficits in long-term potentiation and cognition in fragile X mice. Biol Psychiatry. 2014 Feb 1;75(3):198-206.
Finley J. Elimination of cancer stem cells and reactivation of latent HIV-1 via AMPK activation: Common mechanism of action linking inhibition of tumorigenesis and the potential eradication of HIV-1. Med Hypotheses. 2017 Jul;104:133-146.
Finley J. Facilitation of hippocampal long-term potentiation and reactivation of latent HIV-1 via AMPK activation: Common mechanism of action linking learning, memory, and the potential eradication of HIV-1. Med Hypotheses. Manuscript submitted.
Imai K, Ochiai K, Okamoto T. Reactivation of latent HIV-1 infection by the periodontopathic bacterium Porphyromonas gingivalis involves histone modification. J Immunol 2009;182(6):3688–95.
Mehla R, Bivalkar-Mehla S, Zhang R, et al. Bryostatin modulates latent HIV-1 infection via PKC and AMPK signaling but inhibits acute infection in a receptor independent manner. PLoS ONE 2010;5(6):e11160.
Finley J. Reactivation of latently infected HIV-1 viral reservoirs and correction of aberrant alternative splicing in the LMNA gene via AMPK activation: Common mechanism of action linking HIV-1 latency and Hutchinson-Gilford progeria syndrome. Med Hypotheses. 2015 Sep;85(3):320-32.
Finley J. Oocyte activation and latent HIV-1 reactivation: AMPK as a common mechanism of action linking the beginnings of life and the potential eradication of HIV-1. Med Hypotheses. 2016 Aug;93:34-47.
LaRosa C, Downs SM. Stress stimulates AMP-activated protein kinase and meiotic resumption in mouse oocytes. Biol Reprod. 2006 Mar;74(3):585-92.
Calle-Guisado V, de Llera AH, Martin-Hidalgo D, et al. AMP-activated kinase in human spermatozoa: identification, intracellular localization, and key function in the regulation of sperm motility. Asian J Androl. 2016 Sep 27. doi: 10.4103/1008-682X.185848. [Epub ahead of print].
de Lamirande E, Tsai C, Harakat A, Gagnon C. Involvement of reactive oxygen species in human sperm arcosome reaction induced by A23187, lysophosphatidylcholine, and biological fluid ultrafiltrates. J Androl. 1998 Sep-Oct;19(5):585-94.
Deemeh MR, Tavalaee M, Nasr-Esfahani MH. Health of children born through artificial oocyte activation: a pilot study. Reprod Sci. 2015 Mar;22(3):322-8.
Tamás P, Hawley SA, Clarke RG, et al. Regulation of the energy sensor AMP-activated protein kinase by antigen receptor and Ca2+ in T lymphocytes. J Exp Med. 2006 Jul 10;203(7):1665-70.
Spina CA, Anderson J, Archin NM, et al. An in-depth comparison of latent HIV-1 reactivation in multiple cell model systems and resting CD4+ T cells from aviremic patients. PLoS Pathog 2013;9(12):e1003834.
Finley J. AMPK activation as a common mechanism of action linking the effects of diverse compounds that ameliorate accelerated cellular aging defects in Hutchinson-Gilford progeria syndrome. Med Hypotheses. Manuscript submitted.
0 notes
Text
New study shows AMPK activator MG132 rescues Progeria cells, protects against Microgravity, & inhibits Cancer Stem Cells, HIV, Dengue, & Malaria

Goldsmith Content Providers: CDC/ C. Goldsmith, P. Feorino, E. L. Palmer, W. R. McManus [Public domain], via Wikimedia Commons; CC BY 2.5 (http://creativecommons.org/licenses/by/2.5)], via Wikimedia Commons;Cell Nucleus and Aging: Tantalizing Clues and Hopeful Promises.Scaffidi P, Gordon L, Misteli T. PLoS Biology Vol. 3/11/2005, e395; By NASA [Public domain], via Wikimedia Commons; By Thomas Splettstoesser (www.scistyle.com) [CC BY-SA 3.0 (http://creativecommons.org/licenses/by-sa/3.0)], via Wikimedia Commons.
A recent study published online in the journal EMBO Molecular Medicine in July of 2017 strikingly demonstrated that the proteasome inhibitor and AMPK activator MG132 alleviated accelerated aging defects in cells derived from children with the genetic disorder Hutchinson-Gilford progeria syndrome(HGPS) by inducing autophagic degradation of progerin, the toxic protein responsible for accelerated aging defects in HGPS cells [1]. MG132 also beneficially altered splicing of the LMNA gene (a gene that is aberrantly spliced to produce large amounts of progerin instead of the normal lamin A gene product) by decreasing the gene splicing factor SRSF1 but increasing the splicing factor SRSF5 [1]. Interestingly, progerin was shown to accumulate in structures known as promyelocytic nuclear bodies (PML-NBs) in the nucleus of HGPS cells and local injection of MG132 into a progeria mouse model also led to a reduction in the levels of SRSF1 and progerin [1]. Intriguingly, normal humans also produce progerin via the same aberrant gene splicing method as do children with HGPS, just at much lower levels that increase with age [2].
The recent finding that MG132-induced proteasome inhibition also results in a rapid activation of the master metabolic regulator AMPK (a kinase that increases lifespan and healthspan in several model organisms) and AMPK-dependent autophagy stimulation via the induction of cellular stress (i.e. reactive oxygen species [ROS] generation) further substantiates my hypothesis published in 2014 in which I was first to propose that AMPK activation by structurally diverse compounds (e.g. metformin, resveratrol, etc.) will lead to alleviation of accelerated aging defects in HGPS cells by decreasing the gene splicing factor SRSF1, thus beneficially altering splicing of the LMNA gene, as well as decreasing progerin levels by AMPK-induced autophagy [3,4,33].
Indeed, metformin, which induces cellular stress by mildly inhibiting complex I of the electron transport chain (thus increasing the AMP/ATP ratio) has also recently been shown to reduce the levels of SRSF1 and progerin and activate AMPK in HGPS cells [5-7]. Platelet-derived growth factor BB (PDGF-BB) also increases intracellular calcium (Ca2+) and ROS levels (mediators of cellular stress induction), activates AMPK, and increases SRSF5 in HGPS cells, thus altering splice site selection and beneficially increasing the lamin A/progerin ratio, providing compelling evidence that cellular stress-induced AMPK activation indeed represents a common mechanism of action for gene splicing- and autophagy-induced reductions of progerin in HGPS cells [8-10].
Interestingly, SRSF1 (also known as ASF/SF2) and PML-NBs also inhibit latent HIV-1 reactivation (preventing immune system detection and virus eradication) and SRSF5 (also known as SRp40) increases the abundance and translation of unspliced HIV-1 RNA, which is necessary for latent HIV-1 reactivation [11-13]. As AMPK promotes both latent HIV-1 reactivation and prevents HIV-1 transactivation, MG132 has been shown to reactivate latent HIV-1 and inhibit HIV-1 replication, substantiating my hypothesis published in 2015 in which I first proposed that AMPK activation links correction of aberrant alternative splicing in HGPS cells with reactivation of latent HIV-1 by compounds including MG132, metformin, and resveratrol [14-18].
Additionally, as the induction of cellular stress (e.g. intracellular Ca2+ increase, ROS generation, AMP/ATP ratio increase, etc.) activates AMPK, reactivates latent HIV-1, and leads to the differentiation and/or apoptosis of cancer stem cells in an AMPK-dependent manner, MG132 has also recently been found to induce apoptosis in glioma cancer stem cells, substantiating my most recent publication (2017) in which I propose for the first time that AMPK activation links reactivation of latent HIV-1 with cancer stem cell differentiation and/or apoptosis by diverse compounds that induce cellular stress including metformin and MG132 [5,19-23].
MG132 also induces mouse oocyte meiotic resumption (a process orchestrated by cellular stress-induced AMPK activation and is critical for efficient oocyte activation), delays in vitro oocyte aging, promotes embryonic development from aged oocytes after in vitro fertilization procedures, and alleviates deleterious effects associated with simulated microgravity, further supporting my hypotheses in 2016 and 2017 in which I first proposed that cellular stress-induced AMPK activation links oocyte activation (and hence the beginning of all human life) with latent HIV-1 reactivation and that AMPK activation will improve the activation of T cells in simulated microgravity/spaceflight (which is dependent on intracellular increases in Ca2+ and ROS) [23-28].
As AMPK activators including metformin and MG132 also inhibit dengue virus replication and malaria parasite growth, the aforementioned studies strongly suggests the novel observation that AMPK activation represents a common mechanism of action linking chemically distinct compounds and the effects of those compounds in diseases and phenomena as seemingly disparate as HGPS, HIV-1, microgravity/spaceflight, cancer stem cells, dengue fever, and malaria [29-32].
https://www.linkedin.com/pulse/new-study-shows-ampk-activator-mg132-rescues-progeria-finley?published=t

References
Harhouri K, Navarro C, Depetris D, et al. MG132-induced progerin clearance is mediated by autophagy activation and splicing regulation. EMBO Mol Med. 2017 Jul 3. pii: e201607315. doi: 10.15252/emmm.201607315. [Epub ahead of print].
Scaffidi P, Misteli T. Lamin A-dependent nuclear defects in human aging. Science. 2006 May 19;312(5776):1059-63.
Jiang S, Park DW, Gao Y, et al. Participation of proteasome-ubiquitin protein degradation in autophagy and the activation of AMP-activated protein kinase. Cell Signal. 2015 Jun;27(6):1186-97.
Finley J. Alteration of splice site selection in the LMNA gene and inhibition of progerin production via AMPK activation. Med Hypotheses. 2014 Nov;83(5):580-7; https://www.ncbi.nlm.nih.gov/pubmed/25216752
Hardie DG. AMPK: a target for drugs and natural products with effects on both diabetes and cancer. Diabetes. 2013 Jul;62(7):2164-72.
Egesipe, Blondel, Cicero, et al. Metformin decreases progerin expression and alleviates pathological defects of Hutchinson–Gilford progeria syndrome cells. npj Aging and Mechanisms of Disease 2, Article number: 16026 (2016); http://www.nature.com/articles/npjamd201626?WT.feed_name=subjects_drug-discovery
Park SK, Shin OS. Metformin Alleviates Aging Cellular Phenotypes in Hutchinson-Gilford Progeria Syndrome Dermal Fibroblasts. Exp Dermatol. 2017 Feb 13. doi: 10.1111/exd.13323. [Epub ahead of print].
Lange S, Heger J, Euler G, Wartenberg M, Piper HM, Sauer H. Platelet-derived growth factor BB stimulates vasculogenesis of embryonic stem cell-derived endothelial cells by calcium-mediated generation of reactive oxygen species. Cardiovasc Res. 2009 Jan 1;81(1):159-68.
Kato K, Otsuka T, Kondo A, et al. AMP-activated protein kinase regulates PDGF-BB-stimulated interleukin-6 synthesis in osteoblasts: involvement of mitogen-activated protein kinases. Life Sci. 2012 Jan 2;90(1-2):71-6.
Vautrot V, Aigueperse C, Oillo-Blanloeil F, et al. Enhanced SRSF5 Protein Expression Reinforces Lamin A mRNA Production in HeLa Cells and Fibroblasts of Progeria Patients. Hum Mutat. 2016 Mar;37(3):280-91.
Berro R, Kehn K, de la Fuente C, et al. Acetylated Tat regulates human immunodeficiency virus type 1 splicing through its interaction with the splicing regulator p32. J Virol 2006;80(7):3189–204.
Lusic M, Marini B, Ali H, Lucic B, Luzzati R, Giacca M. Proximity to PML nuclear bodies regulates HIV-1 latency in CD4+ T cells. Cell Host Microbe. 2013 Jun 12;13(6):665-77.
Swanson CM, Sherer NM, Malim MH. SRp40 and SRp55 promote the translation of unspliced human immunodeficiency virus type 1 RNA. J Virol. 2010 Jul;84(13):6748-59.
Mehla R, Bivalkar-Mehla S, Zhang R, et al. Bryostatin modulates latent HIV-1 infection via PKC and AMPK signaling but inhibits acute infection in a receptor independent manner. PLoS One. 2010 Jun 16;5(6):e11160.
Zhang HS, Wu MR. SIRT1 regulates Tat-induced HIV-1 transactivation through activating AMP-activated protein kinase. Virus Res. 2009 Dec;146(1-2):51-7.
Miller LK, Kobayashi Y, Chen CC, Russnak TA, Ron Y, Dougherty JP. Proteasome inhibitors act as bifunctional antagonists of human immunodeficiency virus type 1 latency and replication. Retrovirology. 2013 Oct 24;10:120.
Yu L, Mohanram V, Simonson OE, Smith CI, Spetz AL, Mohamed AJ. Proteasome inhibitors block HIV-1 replication by affecting both cellular and viral targets. Biochem Biophys Res Commun. 2009 Jul 17;385(1):100-5.
Finley J. Reactivation of latently infected HIV-1 viral reservoirs and correction of aberrant alternative splicing in the LMNA gene via AMPK activation: Common mechanism of action linking HIV-1 latency and Hutchinson-Gilford progeria syndrome. Med Hypotheses. 2015 Sep;85(3):320-32; https://www.ncbi.nlm.nih.gov/pubmed/26115946
Sook SH, Lee HJ, Kim JH, et al. Reactive oxygen species-mediated activation of AMP-activated protein kinase and c-Jun N-terminal kinase plays a critical role in beta-sitosterol-induced apoptosis in multiple myeloma U266 cells. Phytother Res. 2014 Mar;28(3):387-94.
Piette J, Legrand-Poels S. HIV-1 reactivation after an oxidative stress mediated by different reactive oxygen species. Chem Biol Interact. 1994 Jun;91(2-3):79-89.
Cheng X, Kim JY, Ghafoory S, et al. Methylisoindigo preferentially kills cancer stem cells by interfering cell metabolism via inhibition of LKB1 and activation of AMPK in PDACs. Mol Oncol 2016. pii: S1574-7891(16)00018-1.
Yoo YD, Lee DH, Cha-Molstad H, et al. Glioma-derived cancer stem cells are hypersensitive to proteasomal inhibition. EMBO Rep. 2017 Jan;18(1):150-168.
Finley J. Elimination of cancer stem cells and reactivation of latent HIV-1 via AMPK activation: Common mechanism of action linking inhibition of tumorigenesis and the potential eradication of HIV-1. Med Hypotheses. 2017 Jul;104:133-146; https://www.ncbi.nlm.nih.gov/pubmed/28673572
Huo LJ, Fan HY, Zhong ZS, Chen DY, Schatten H, Sun QY. Ubiquitin-proteasome pathway modulates mouse oocyte meiotic maturation and fertilization via regulation of MAPK cascade and cyclin B1 degradation. Mech Dev. 2004 Oct;121(10):1275-87.
LaRosa C, Downs SM. Stress stimulates AMP-activated protein kinase and meiotic resumption in mouse oocytes. Biol Reprod. 2006 Mar;74(3):585-92.
Ono T, Mizutani E, Li C, Yamagata K, Wakayama T. Offspring from intracytoplasmic sperm injection of aged mouse oocytes treated with caffeine or MG132. Genesis. 2011 Jun;49(6):460-71.
Jamart C, Raymackers JM, Li An G, Deldicque L, Francaux M. Prevention of muscle disuse atrophy by MG132 proteasome inhibitor. Muscle Nerve. 2011 May;43(5):708-16.
Finley J. Oocyte activation and latent HIV-1 reactivation: AMPK as a common mechanism of action linking the beginnings of life and the potential eradication of HIV-1. Med Hypotheses. 2016 Aug;93:34-47; https://www.ncbi.nlm.nih.gov/pubmed/27372854
Soto-Acosta R, Bautista-Carbajal P, Cervantes-Salazar M, Angel-Ambrocio AH, Del Angel RM. DENV up-regulates the HMG-CoA reductase activity through the impairment of AMPK phosphorylation: A potential antiviral target. PLoS Pathog. 2017 Apr 6;13(4):e1006257.
Ruivo MT, Vera IM, Sales-Dias J, et al. Host AMPK Is a Modulator of Plasmodium Liver Infection. Cell Rep. 2016 Sep 6;16(10):2539-45.
Fernandez-Garcia MD, Meertens L, Bonazzi M, Cossart P, Arenzana-Seisdedos F, Amara A. Appraising the roles of CBLL1 and the ubiquitin/proteasome system for flavivirus entry and replication. J Virol. 2011 Mar;85(6):2980-9.
Prasad R, Atul, Kolla VK, et al. Blocking Plasmodium falciparum development via dual inhibition of hemoglobin degradation and the ubiquitin proteasome system by MG132. PLoS One. 2013 Sep 2;8(9):e73530.
Salminen A, Kaarniranta K. AMP-activated protein kinase (AMPK) controls the aging process via an integrated signaling network. Ageing Res Rev. 2012 Apr;11(2):230-41.
#progeria#metformin#ampk#cancer stem cell#HIV/AIDS#microgravity#spaceflight#Dengue Virus#malaria#mg132
0 notes
Text
AMPK & Metformin links T cell aging during Spaceflight with Cancer stem cells, HIV-1 latency, telomeres, oocyte activation, & Progeria

Goldsmith Content Providers: CDC/ C. Goldsmith, P. Feorino, E. L. Palmer, W. R. McManus [Public domain], via Wikimedia Commons; The Cell Nucleus and Aging: Tantalizing Clues and Hopeful Promises.Scaffidi P, Gordon L, Misteli T. PLoS Biology Vol. 3/11/2005, e395; CC BY 2.5 (http://creativecommons.org/licenses/by/2.5)], via Wikimedia Commons; By NASA [Public domain], via Wikimedia Commons
A recent study published in the journal Oncogene in June of 2017 by researchers from The Johns Hopkins University School of Medicine and Emory University School of Medicine demonstrated that a bioactive molecule derived from Magnolia grandiflora significantly inhibited breast cancer stem cells (also known as tumor-initiating cells) in vivo in mice and also inhibited the expression of the stem cell/pluripotency markers Oct4, Nanog, and Sox2 in breast cancer cells in an AMPK-dependent manner [1]. Interestingly, a recent study published in the journal Oncotarget in May of 2017 showed that metformin activated AMPK and inhibited cell proliferation, clonogenic ability, tumorsphere formation (a characteristic of cancer stem cells), and the cancer stem cell population in chemotherapy-resistant colorectal cancer cells [2]. Metformin has also been shown to promote the differentiation and/or apoptosis of cancer stem cells in vitro and in vivo in an AMPK-dependent manner from the deadliest of cancers, including glioblastoma and pancreatic cancer [3]. Cancer stem cells (CSCs) exhibit several stem cell-like qualities that are characteristic of embryonic or adult stem cells, including self-renewal, differentiation, and the ability to initiate tumorigenesis. CSCs, similar to adult stem cells (ASCs), may also undergo quiescence, a state or period of inactivity or dormancy that contributes to the resistance of CSCs to radiation and/or chemotherapy, potentially allowing CSCs to re-seed tumors at a later time [3]. Intriguingly, HIV-1 has been shown to establish durable and long-stating latency, similar to CSC quiescence, in central CD4+ memory T cells as well as in T memory stem cells, T cell subsets that also display stem-cell like qualities including self renewal and differentiation. Latency establishment by HIV-1 in these T cell subsets prevents immune system detection and virus destruction or virus-induced cell death, analogous to CSC quiescence [3]. Because the use of AMPK activators, including metformin, bryostain-1, JQ1, and resveratrol as single agents or in a combinatorial fashion reactivates latent HIV-1 and induces CSC differentiation and/or apoptosis, a novel observation would logically follow that AMPK activation links the reactivation of latent HIV-1 (known as the “shock and kill” approach in HIV-1 cure research) with the “activation” (i.e. cell-cycle reentry), differentiation, and/or apoptosis of CSCs (which may also be labeled as a “shock and kill” approach) [3].
Indeed, a recently published paper in June of 2017 that I authored (and an additional paper currently under review) elucidates a novel link that connects CSCs with HIV-1 latency, microgravity and spaceflight, Hutchinson-Gilford progeria syndrome (HGPS), oocyte activation, the sperm acrosome reaction, Down syndrome, and hippocampal long-term potentiation [3,4]. This connection is predicated on the induction of cellular stress (mediated by increases in intracellular calcium [Ca2+] levels, reactive oxygen species [ROS] generation, and/or AMP/ATP ratio increases, etc), leading to beneficial cellular responses that are mediated by the master metabolic regulator AMPK [3]. As further explained below, AMPK is activated by a variety of stressors (osmotic, electrical stimulation, chemical, heat stress, etc.) as well as stressors that are diminished in microgravity/spaceflight (e.g. shear stress, force). AMPK also increases lifespan and healthspan in several model organisms (e.g. yeast, worms, flies, and mice) and metformin alleviates accelerated aging defects in cells from children with the genetic disorder HGPS. Because AMPK is also critical for T cell activation and the mounting of an effective immune response, promotes embryonic and adult stem cell differentiation, and metformin enhances bone formation, osteoprotegerin synthesis, and telomere integrity, AMPK activation by structurally diverse compounds that have a proven safety record may indeed provide protection for astronauts embarking on long-term space missions [3,5-7].
In May of 2017, I published for the first time that cellular stress-induced AMPK activation links the differentiation and/or apoptosis of cancer stem cells (CSCs) with the reactivation of latent HIV-1 and alleviation of accelerated aging-like cellular defects resulting from microgravity and spaceflight [3]. ROS and Ca2+ are well-studied mediators of cellular stress-induced differentiation of embryonic and adult stem cells, AMPK has recently been shown to be essential for mouse embryonic stem cell differentiation, and metformin targets and promotes differentiation and/or apoptosis of cancer stem cells in the deadliest of cancers in an AMPK-dependent manner, including glioblastoma and pancreatic cancer [3]. Interestingly, AMPK is critical for T cell activation and the mounting of an effective immune response in vivo, as AMPK inhibition during T cell activation leads to T cell death [3]. HIV-1 has been shown to establish latency (thus preventing viral eradication) in long-lived T cell subsets that display longer telomeres, including central CD4+ memory T cells and CD4+ T memory stem cells [3]. However, the phorbol ester PMA combined with the calcium ionophore ionomycin is used as a positive control to reactivate latent HIV-1 and both compounds have been shown in independent studies to activate AMPK. Several structurally diverse compounds that also induce reactivation of latent HIV-1 in CD4+ memory T cells (called the “shock and kill” approach in HIV-1 cure research), including metformin combined with bryostatin-1, butyrate, JQ1, etc. also promote the differentiation and/or apoptosis of CSCs, indicating that the cellular states of CSC quiescence and HIV-1 latency are analogous and the induction of a cellular stress response, mediated by AMPK, may lead to both CSC elimination and viral eradication [3].
Additionally, simulated microgravity has been shown to inhibit AMPK activation, transient levels of hypergravity activate AMPK, and shear stress and the application of force have both been shown to activate AMPK, indicating that gravity itself represents a cellular stressor and is essential for efficient T cell activation and the mounting of an effective immune response [3,8,9]. Indeed, the application of force enhances T cell activation and both embryonic stem cell differentiation and T cell activation are significantly inhibited during actual spaceflight [3]. The lack of cellular stress induction (i.e. mechano-transduction) in microgravity/spaceflight may also explain the recent unusual observance of longer telomeres in the flight-based compared to the ground-based subject in the NASA TWINS Study [10]. Central memory T cells and T memory stem cells (TSCMs) are long-lived T cell subsets with longer telomeres and are capable of self-renewal and differentiation into more differentiated effector T cells [11,12]. However, as noted above, cellular stress and subsequent AMPK activation is essential for T cell activation and the mounting of an effective immune response and microgravity/spaceflight minimizes cellular stress induction, leading to an increase in undifferentiated T cell subsets that possess stem-cell like qualities (e.g. longer telomeres), with a consequent deficiency in the response to viruses, bacteria, and pathogens that return to normal levels after re-introduction of cellular stress (i.e. return to Earth’s gravitational field) [3].
Strikingly, cells that are placed in NASA-developed rotating wall vessel bioreactors (RWVs), devices that are commonly used to mimic microgravity that also reduce shear stress, exhibit stem cell-like behavior (i.e. sphere formation) and reduced differentiation, very much similar to cancer stem cells [13]. A recent study has also shown that multipotent cells treated with strontium chloride, a compound that activates mouse oocytes and has been used to activate human oocytes, producing normal healthy children, induced activation of osteoblastogenesis in RWVs, indicating that cellular stress, mediated by gravity/force (i.e. mechano-transduction), Ca2+, ROS, and/or AMP/ATP ratio increases, etc. links AMPK activation with microgravity/spaceflight, oocyte activation, cancer stem cells, and HGPS (see below) [14-16].
In 2014, I was the first to propose and publish that metformin would alleviate accelerated aging defects in cells derived from patients with the accelerated aging disorder HGPS by promoting cellular stress-induced AMPK activation and beneficially altering the activity of the gene splicing factor SRSF1 (which is dysregulated in HGPS) [17]. This hypothesis was substantiated in 2016 and 2017, with metformin activating AMPK, decreasing the levels of SRSF1, and alleviating accelerated aging defects in HGPS cells [18,19]. In 2015, I was also the first to publish that the gene splicing factor SRSF1 links HGPS and HIV-1 latency, as excessive splicing activity of SRSF1 prevents reactivation of HIV-1, preventing viral cytopathic or cytolytic effects [20]. Because AMPK activation is critical for T cell activation, I also hypothesized that structurally dissimilar compounds that induce latent HIV-1 reactivation actually do so via indirect cellular stress-induced AMPK activation [20]. Intriguingly, a study by researchers at Merck showed that knockdown of AMPK or CaMKK2 (an upstream kinase/activator of AMPK) significantly inhibits HIV-1 replication [21]. Several compounds and methodologies that reactivate latent HIV-1, including heat stress, free-radical generating compounds, resveratrol, and many others have been shown to induce AMPK activation, indicating that cellular stress-induced AMPK activation orchestrates and connects both latent HIV-1 reactivation and HGPS [3,20,25]. Interestingly, temsirolimus, a rapamycin analog, was recently shown to correct accelerated aging defects in HGPS cells but transiently increase the levels of ROS and superoxide anion but decrease VO2 max within the first hour, providing further evidence that cellular stress-induced AMPK activation links HIV-1 latency and HGPS [22]. Intriguingly, rapamycin production by the bacterium Streptomyces hygroscopicus is significantly inhibited (~90%) when the bacterium is exposed to conditions of microgravity (i.e. being placed in a rotating wall vessel bioreactor), indicating that the beneficial effects of cellular stress induction may cross species boundaries, leading to increased production of valuable secondary metabolites by microorganisms (e.g. penicillin, rapamycin, avermectins, etc.) [23].
In 2016, I also first proposed that AMPK activation links oocyte activation, which is a prerequisite for the creation of all human life, with the reactivation of latent HIV-1 reservoirs [24]. The intracellular signaling mechanisms that characterize oocyte activation are incredibly similar to those that characterize latent HIV-1 reactivation in memory CD4+ T cells, and include the PLC-PIP2-IP3-Ca2+ and PLC-PIP2-DAG-PKC pathways. AMPK activation is also critical for oocyte meiotic resumption and maturation, two processes that precede and are critical for efficient oocyte activation [24]. Also, PMA and ionomycin, both of which activate AMPK and are used as positive controls in HIV-1 latency reversal studies, also induce mouse oocyte activation (models for human oocytes) and ionomycin is extensively used during in vitro fertilization procedures to activate human oocytes, producing normal healthy children [24]. Interestingly, MG132 (proteasome inhibitor), methylene blue, menadione (free radical-generating agent), resveratrol analogs, heat stress, and osmotic stress have each been shown to induce mouse oocyte meiotic resumption and activate AMPK [24]. Additionally, AMPK has recently been found localized across the entire acrosome in human spermatozoa and stress-inducing compounds, including ROS, PMA, ionomycin, vitamin D, and capsaicin have each been shown to induce the acrosome reaction in human sperm, an indispensable process for the creation of all human life outside of a clinical setting [3,4,26]. Interestingly, electrical stimulation, puromycin (a protein synthesis inhibitor), and ethanol have also been shown to activate human oocytes and induce AMPK activation, suggesting that cellular stress-induced AMPK activation, mediated by Ca2+, ROS, and AMP/ATP ratio increases, etc. links the creation of all human life (i.e. the “shock and live” approach) with HGPS and latent HIV-1 reactivation (i.e. the “shock and kill” approach) [24].
Also, I have a recently submitted manuscript (2017) currently under review that links cellular stress-induced AMPK activation with hippocampal CA1 long-term potentiation (LTP, a process critical for learning and memory) and HIV-1 latency [4]. Indeed, an increase in intracellular Ca2+ levels is indispensable for the induction of hippocampal CA1 LTP, the inhibition of ROS production or ROS scavengers significantly inhibits hippocampal CA1 LTP, and the primary excitatory neurotransmitter glutamate activates AMPK in neurons and also enhances T cell activation/proliferation [4]. Metformin also activates AMPK in hippocampal CA1 neurons, resveratrol upregulates AMPA receptors in the hippocampus in an AMPK-dependent manner (AMPA receptors are considered critical for the expression of LTP), the phorbol ester PMA enhances hippocampal CA1 LTP, and metformin alleviates Aβ-mediated reductions in hippocampal LTP, indicating that cellular stress-induced AMPK activation links hippocampal CA1 LTP with HIV-1 latency, HGPS, cancer stem cells, oocyte activation, sperm acrosome reaction, and spaceflight [4]. Interestingly, metformin also improves behavioral defects and activates AMPK in a mouse model of Fragile X syndrome, reverses mitochondrial defects in human fibroblasts derived from fetuses with Down syndrome, and inhibition of the Down syndrome gene DYRK1A induces reactivation of latent HIV-1, indicating that cellular stress-induced AMPK activation is also beneficial for and links certain genetic disorders with HIV-1 latency [3,27].
In conclusion, accumulating evidence strongly suggests that the induction of cellular stress, mediated by increases in ROS, intracellular Ca2+, and/or AMP/ATP ratio increases, etc. leads to beneficial cellular responses that are orchestrated by the master metabolic regulator AMPK. As first proposed in my current and forthcoming publications, cellular stress-induced AMPK activation links the therapeutic effects of structurally diverse compounds in seemingly disparate physiological and patho-physiological states, including cancer stem cells, HIV-1 latency, microgravity and spaceflight, Hutchinson-Gilford progeria syndrome, oocyte activation, the sperm acrosome reaction, Down syndrome, Fragile X syndrome, and hippocampal long-term potentiation. As evidence continues to accrue substantiating this novel link, a paradigm shift in the assessment of disease etiology and the practice of medicine is inevitable.
https://www.linkedin.com/pulse/ampk-metformin-links-cell-aging-during-spaceflight-cancer-finley?published=t

References:
Sengupta S, Nagalingam A, Muniraj N, et al. Activation of tumor suppressor LKB1 by honokiol abrogates cancer stem-like phenotype in breast cancer via inhibition of oncogenic Stat3. Oncogene. 2017 Jun 5. doi: 10.1038/onc.2017.164. [Epub ahead of print].
Kim SH, Kim SC, Ku JL. Metformin increases chemo-sensitivity via gene downregulation encoding DNA replication proteins in 5-Fu resistant colorectal cancer cells. Oncotarget. 2017 May 11. doi: 10.18632/oncotarget.17798. [Epub ahead of print].
Finley J. Elimination of cancer stem cells and reactivation of latent HIV-1 via AMPK activation: Common mechanism of action linking inhibition of tumorigenesis and the potential eradication of HIV-1. Med Hypotheses. 2017 May, In Press, Accepted Manuscript; https://authors.elsevier.com/a/1VBXX15pGbnzkq
Finley J. Facilitation of hippocampal long-term potentiation and reactivation of latent HIV-1 via AMPK activation: Common mechanism of action linking learning, memory, and the potential eradication of HIV-1. Med Hypotheses. Manuscript submitted.
Shah M, Kola B, Bataveljic A, et al. AMP-activated protein kinase (AMPK) activation regulates in vitro bone formation and bone mass. Bone. 2010 Aug;47(2):309-19.
Mai QG, Zhang ZM, Xu S, et al. Metformin stimulates osteoprotegerin and reduces RANKL expression in osteoblasts and ovariectomized rats. J Cell Biochem. 2011 Oct;112(10):2902-9.
Diman A, Boros J, Poulain F, et al. Nuclear respiratory factor 1 and endurance exercise promote human telomere transcription. Sci Adv. 2016 Jul 27;2(7):e1600031.
Bays JL, Campbell HK, Heidema C, Sebbagh M, DeMali KA. Linking E-cadherin mechanotransduction to cell metabolism through force-mediated activation of AMPK. Nat Cell Biol. 2017 Jun;19(6):724-731.
Dixit M, Bess E, Fisslthaler B, et al. Shear stress-induced activation of the AMP-activated protein kinase regulates FoxO1a and angiopoietin-2 in endothelial cells. Cardiovasc Res. 2008 Jan;77(1):160-8.
https://www.nasa.gov/feature/symphonizing-the-science-nasa-twins-study-team-begins-integrating-results
Ahmed R, Roger L, Costa Del Amo P, et al. Human Stem Cell-like Memory T Cells Are Maintained in a State of Dynamic Flux. Cell Rep. 2016 Dec 13;17(11):2811-2818.
Graef P, Buchholz VR, Stemberger C, et al. Serial transfer of single-cell-derived immunocompetence reveals stemness of CD8(+) central memory T cells. Immunity. 2014 Jul 17;41(1):116-26.
Ingram M, Techy GB, Saroufeem R, et al. Three-dimensional growth patterns of various human tumor cell lines in simulated microgravity of a NASA bioreactor. In Vitro Cell Dev Biol Anim. 1997 Jun;33(6):459-66.
Louis F, Bouleftour W, Rattner A, et al. RhoGTPase stimulation is associated with strontium chloride treatment to counter simulated microgravity-induced changes in multipotent cell commitment. npj Microgravity 3, Article number: 7 (2017).
Ma SF, Liu XY, Miao DQ, et al. Parthenogenetic activation of mouse oocytes by strontium chloride: a search for the best conditions. Theriogenology. 2005 Sep 15;64(5):1142-57.
Kim JW, Kim SD, Yang SH, Yoon SH, Jung JH, Lim JH. Successful pregnancy after SrCl2 oocyte activation in couples with repeated low fertilization rates following calcium ionophore treatment. Syst Biol Reprod Med. 2014 Jun;60(3):177-82.
Finley J. Alteration of splice site selection in the LMNA gene and inhibition of progerin production via AMPK activation. Med Hypotheses. 2014 Nov;83(5):580-7; https://www.ncbi.nlm.nih.gov/pubmed/25216752
Egesipe, Blondel, Cicero, et al. Metformin decreases progerin expression and alleviates pathological defects of Hutchinson–Gilford progeria syndrome cells. npj Aging and Mechanisms of Disease 2, Article number: 16026 (2016).
Park SK, Shin OS. Metformin Alleviates Aging Cellular Phenotypes in Hutchinson-Gilford Progeria Syndrome Dermal Fibroblasts. Exp Dermatol. 2017 Feb 13. doi: 10.1111/exd.13323. [Epub ahead of print].
Finley J. Reactivation of latently infected HIV-1 viral reservoirs and correction of aberrant alternative splicing in the LMNA gene via AMPK activation: Common mechanism of action linking HIV-1 latency and Hutchinson-Gilford progeria syndrome. Med Hypotheses. 2015 Sep;85(3):320-32; https://www.ncbi.nlm.nih.gov/pubmed/26115946
Zhou H, Xu M, Huang Q, et al. Genome-scale RNAi screen for host factors required for HIV replication. Cell Host Microbe. 2008 Nov 13;4(5):495-504.
Gabriel D, Gordon LB, Djabali K. Temsirolimus Partially Rescues the Hutchinson-Gilford Progeria Cellular Phenotype. PLoS One. 2016 Dec 29;11(12):e0168988.
Fang A, Pierson DL, Mishra SK, Demain AL. Growth of Steptomyces hygroscopicus in rotating-wall bioreactor under simulated microgravity inhibits rapamycin production. Appl Microbiol Biotechnol. 2000 Jul;54(1):33-6.
Finley J. Oocyte activation and latent HIV-1 reactivation: AMPK as a common mechanism of action linking the beginnings of life and the potential eradication of HIV-1. Med Hypotheses. 2016 Aug;93:34-47; https://www.ncbi.nlm.nih.gov/pubmed/27372854
Finley J. AMPK activation as a common mechanism of action linking the effects of diverse compounds that ameliorate accelerated cellular aging defects in Hutchinson-Gilford progeria syndrome. Med Hypotheses. Manuscript submitted.
De Toni L, Garolla A, Menegazzo M, et al. Heat Sensing Receptor TRPV1 Is a Mediator of Thermotaxis in Human Spermatozoa. PLoS One. 2016 Dec 16;11(12):e0167622.
Gantois I, Khoutorsky A, Popic J, et al. Metformin ameliorates core deficits in a Fragile X syndrome mouse model. Nat Med. 2017 May 15. doi: 10.1038/nm.4335. [Epub ahead of print].
0 notes
Text
Metformin shown for first time to inhibit malaria parasite in human liver cells: AMPK links pathogen destruction, Down syndrome, Fragile X, & Progeria

Vanellus Foto (Own work) [GFDL (http://www.gnu.org/copyleft/fdl.html); "Hutchinson-Gilford Progeria Syndrome" by The Cell Nucleus and Aging: Tantalizing Clues and Hopeful Promises. Scaffidi P, Gordon L, Misteli T. PLoS Biology Vol. 3/11/2005, e395; Peter Saxon (Own work) [CC BY-SA 4.0 (http://creativecommons.org/licenses/by-sa/4.0)], via Wikimedia Commons; Jim Gathany [Public domain], via Wikimedia Commons
In line with recent evidence that demonstrated for the first time that metformin exerted significant antiviral effects in dengue virus-infected human liver cells in an AMPK-dependent manner, a study published in the journal Cell Reports in September of 2016 by researchers from the Massachusetts Institute of Technology (MIT) and the University of Lisbon also showed for the first time that metformin and other AMPK activators significantly reduced parasite load in human liver cells of different species of Plasmodium, a protozoan parasite that is the etiological agent of malaria [1,2]. Importantly, the authors also showed that AMPK activation inhibits growth and replication of different Plasmodium spp. (species) and AMPK activators as well as dietary restriction, which activates AMPK, reduces Plasmodium berghei (malaria-causing species in rodents often used as a model for the study of human malaria) infection in mice [1]. Interestingly, metformin combined with another AMPK activator, bryostatin-1, has been shown to reactivate latent HIV-1 (facilitating immune system detection and virus destruction) while AMPK activation inhibits HIV-1 transactivation (a process necessary for HIV-1 replication), indicating that metformin will likely enhance latent HIV-1 reactivation and inhibit productive HIV-1 replication via AMPK activation, a hypothesis that I first proposed in an upcoming publication [3-5]. Additionally, metformin has recently been shown to beneficially alter gene splicing, activate AMPK, and alleviate accelerated aging defects in cells derived from Hutchison-Gilford progeria syndrome (HGPS) patients, reverse mitochondrial dysfunction in human fetal cells with Down syndrome (DS), restore behavioral and morphological defects in a mouse model of Fragile X syndrome (FXS), and improve behavior in humans with FXS (see below). Such evidence strongly suggests that AMPK activation induced by cellular stress (i.e. AMP/ATP ratio increase, reactive oxygen species generation, and/or intracellular calcium increases, etc.) links the amelioration and/or reversal of pathological cellular defects in HGPS, DS, and FXS with HIV-1 latency and replication, adult and cancer stem cells, learning and memory, and the creation all human life (via oocyte activation/sperm acrosome reaction), hypotheses that I first proposed in several publications and pending manuscripts [5-10].
Malaria is mosquito-borne infectious disease caused by several species of Plasmodium and is characterized by fever and flu-like illness that may lead to coma or death in severe cases [1]. Interestingly, although blood stage parasites cause the symptoms of malaria, malaria infection begins with parasitization of liver cells, marked by invasion and replication of sporozoites (infective agents introduced into the host) via schizogony (asexual reproduction by multiple fission) into thousands of merozoites (new parasites) that typically occurs in human and rodent parasites within 2 weeks and 48 hours, respectively [1].
The authors initially showed that phosphorylation/activation of AMPKα is decreased in Huh7 cells (human liver cells) infected with the rodent parasite Plasmodium berghei (P. berghei) compared to non-infected cells at 18 hours (hr) post-infection. RNAi-induced knockdown of both AMPKα subunits (AMPKα1 & AMPKα2) in P. berghei-infected cells also led to a significant increase in mean size distribution of schizont parasite forms (cells that divide by schizogony to form daughter cells) at 48 hr post-infection [1]. However, Huh7 cells expressing a constitutively active form of the AMPKα1 subunit displayed significantly smaller hepatic schizonts compared to control cells, indicating that AMPK restricts growth of P. berghei in liver cells. Exposure of P. berghei-infected Huh7 cells to the AMPK-activating compounds salicylate, metformin, 2-deoxy-D-glucose, and A769662 led to a significant decrease in schizont size and a dose-dependent reduction of total parasite load for each compound tested [1]. Further testing with salicylate (an ester of salicylic acid; aspirin is a derivative of salicylic acid) revealed similar inhibitory effects on different species of Plasmodium in both mouse and human cells, with salicylate inhibiting parasite development in Hepa1-6 cells (mouse hepatoma cell line) infected with P. yoelii (used to infect mice as a model of human malaria), Huh7 cells and mouse primary hepatocytes infected with P. berghei, and in human donor-derived primary hepatocytes infected with P. falciparum (causes malaria in humans) [1].
Additionally, after maturation of P berghei into the final endstage of hepatic development, characterized by fully mature merozoites and merosome release from the substratum, salicylate treatment reduced merosome size and number at 66 hr and reduced total merosome load by 80% up to 74 hr [1]. Cells treated with salicylate also contained smaller MSP1-positive schizonts (MSP1 is a merozoite surface marker essential for merozoite maturation), indicating that salicylate-induced AMPK activation will reduce the total number of merozoites released into the blood to infect erythrocytes [1]. Indeed, salicylate activated AMPKα in vivo in mouse livers, significantly reduced parasite size compared to control mice (after intradermal injection of sporozoites to mimic a mosquito bite), and reduced the number of infected erythrocytes by 57 % 72 hr after infection. Intriguingly, mice placed on a dietary restriction protocol for 2–3 weeks prior to and during liver-stage infection displayed increased liver AMPK activation, a significant reduction of hepatic schizont size, and a 66% decrease in pre-patent blood stage infection, demonstrating that AMPK activation potently inhibits Plasmodium growth during malaria liver-stage infection and reduces subsequent erythrocyte infection [1].
As noted above, in addition to reducing malaria parasite load in human liver cells, metformin has also been shown to inhibit dengue virus replication in human liver cells in an AMPK-dependent [1,2]. The AMPK activator resveratrol also inhibits dengue virus replication in human liver cells, inhibits emtricitabine-resistant HIV-1, and reactivates latent HIV-1 (facilitating immune system detection and virus destruction or viral cytopathic effects) [11-13]. Furthermore, a common metabolite of artemisinin and its analogs, a powerful drug considered the standard first-line treatment for malaria caused by P. falciparum, shares a common mechanism of AMPK activation with both metformin and the BRD4 inhibitor JQ1, a compound that has shown exceptional effects in reactivating latent HIV-1, implicating AMPK activation as common mechanism through which structurally distinct compounds exert anti-viral effects against dengue virus, HIV-1 latency and replication, and malaria [14-16]. Moreover, as discussed below, metformin also activates AMPK and alleviates accelerated aging defects in cells from Hutchinson-Gilford progeria syndrome (HGPS) patients, rescues mitochondrial defects in human fetal cells with Down syndrome (DS), and significantly improves behavioral deficits in humans and in a mouse model with the genetic disorder Fragile X syndrome (FXS), providing compelling evidence that AMPK activation induced by cellular stress (i.e. AMP/ATP ratio increase, reactive oxygen species generation, and/or intracellular calcium increases, etc.) links the amelioration and/or reversal of pathological cellular defects in HGPS, DS, and FXS with HIV-1 latency and replication, adult and cancer stem cells, learning and memory, and the creation of all human life (via oocyte activation/sperm acrosome reaction), hypotheses that I first proposed in several publications and pending manuscripts [5-10].
Dy ABC et al. demonstrated for the first time that metformin consistently improved behavior in several patients diagnosed with Fragile X Syndrome (FXS), a genetic disorder characterized by intellectual disability and significant deficits in neurological function and cognitive development [17]. An improvement in behavior was documented in the Aberrant Behavior Checklist (ABC) for all cases, as evidenced by consistent improvements (i.e. lower scores compared to pre-metformin treatment) in social avoidance, irritability, hyperactivity, and social unresponsiveness as well as improvements in language and conversational skills reported by familial caretakers [17]. Metformin has also recently been shown to rescue and restore memory deficits in a Drosophila model of FXS and correct social novelty impairment, reduce testicular weight, decrease repetitive grooming, rescue excessive long-term depression and dendritic spine abnormalities, and alter excitatory synaptic transmission in an FXS mouse model [18,19].
Down syndrome (DS) is caused by either a partial or full trisomy of chromosome 21 and is associated with impairments in cognition, learning and memory, and disorders of the immune system [20]. Human and animal studies strongly suggest that mitochondrial dysfunction is associated with pathogenic features of DS and mitochondrial abnormalities have been found in all DS cells analyzed in culture to date, including neurons, astrocytes, and lymphocytes [20]. Indeed, in DS human fetal fibroblasts, PGC-1α, a master regulator of mitochondrial biogenesis, is down regulated at both the mRNA and protein levels and AMPK phosphorylation of the PGC-1α protein is essential for PGC-1α-dependent induction of the PGC-1α promoter [20].
Izzo et al. observed that DS human fetal fibroblasts (DS-HFFs) displayed a 40%-50% reduction in PGC-1α mRNA and protein levels compared to non-trisomic counterparts (N-HFFs). DS-HFFs treated with metformin however led to a significant increase in PGC-1α at both the mRNA and protein levels compared to untreated control cells, an increase in the mRNA levels of NRF-1 and TFAM (PGC-1α target genes critical in promoting mitochondrial biogenesis), and an increase in mtDNA content [20]. Additionally, compared to N-HFFs, DS-HFFs were characterized by a decrease in basal oxygen consumption rate (OCR, ~55% inhibition), a decrease in OCR related to ATP production (~58% inhibition), and a reduction in maximal respiratory capacity (~58% inhibition). DS-HFFs treated with metformin increased basal OCR, OCR related to ATP production, ATP concentration, mitochondrial membrane potential, and maximal respiratory capacity, thus restoring mitochondrial respiration in DS-HFFs [20].
Furthermore, compared to N-HFFs, DS-HFFs exhibited extensive mitochondrial damage, as evidenced by swollen cristae (~60%-90% of DS-HFFs vs. ~15% of N-HFFs), mitochondria with intra-oedema (~40% of DS-HFFs vs. ~6% of N-HFFs), and an increase in the number of damaged mitochondria (~90% in DS-HFFs vs. ~25% in N-HFFs). Metformin-treated DS-HFFs, compared to untreated cells, displayed narrower cristae with widths that were comparable to N-HFFs cells, fewer damaged mitochondria (~40%), and fewer mitochondria with intra-oedema (~5%) [20]. Interestingly, as mitochondrial fusion also plays an important role in mitochondrial functionality, the mRNA and protein expression of OPA1 and MFN2 (genes that regulate mitochondrial fusion) were significantly down-regulated in DS-HFFs vs. N-HFFs and correlated with extensive mitochondrial fragmentation in DS-HFFs. Treatment of DS-HFFs with metformin significantly increased the mRNA and protein levels of OPA1 and MFN2 and also reduced fragmentation of the mitochondrial network, indicating that metformin induces correction of mitochondrial phenotype by also restoring mitochondrial fusion machinery [20].
The AMPK activator EGCG also reverses cognitive defects in DS patients, promotes oxidative phosphorylation and mitochondrial biogenesis in lymphoblasts and fibroblasts from DS patients (i.e. increased levels and activity of PGC-1α, NRF-1 and TFAM), and restores oxidative phosphorylation, mitochondrial biogenesis, and improves hippocampal neural progenitor cell proliferation in a DS mouse model (Ts65Dn) in combination with the polyphenol resveratrol (i.e. increase in PGC-1α) in an AMPK-dependent manner [21-23]. Mitochondrial dysfunction is also a prominent feature associated with the accelerated aging disorder Hutchison-Gilford progeria syndrome (HGPS) and metformin has recently been shown to alleviate accelerated aging defects, activate AMPK, and beneficially alter gene splicing in HGPS patient cells, as I first hypothesized and proposed in 2014 and 2015 [6,7].
Egesipe et al. demonstrated, using mesenchymal stem cells (MSCs) derived from HGPS induced pluripotent stem cells (i.e. HGPS MSCs), a significant dose-dependent decrease in SRSF1 mRNA levels after metformin treatment and up to a 40% decrease in SRSF1 protein levels after treatment with 5 mmol/l of metformin (SRSF1 is a gene splicing factor that is upregulated in HGPS cells and causes faulty gene splicing of the LMNA gene leading to increased production of the toxic protein progerin) [24]. A significant decrease was also observed in both lamin A and progerin mRNA expression in HGPS MSCs treated with 5 mmol/l metformin, with progerin mRNA expression and protein levels reduced to levels lower than that of lamin A mRNA expression and protein levels, indicating that metformin-induced inhibition of SRSF1 led to an increase in the lamin A/progerin ratio and thus beneficially altered gene splicing [24].
The results obtained using HGPS MSCs were also replicated in additional in vitro cell models, with 5 mmol/l of metformin decreasing progerin mRNA expression up to 50% in LmnaG609G/G609G mouse primary fibroblasts (HGPS mouse model) and decreasing both lamin A and progerin mRNA expression in primary HGPS fibroblasts [24]. Interestingly, 5 mmol/l of metformin also decreased progerin mRNA expression in wild-type/normal MSCs that had been incubated with a compound that induces progerin expression, indicating that metformin may also prove beneficial in reducing progerin levels in normal humans [24].
Most importantly, however, treatment of HGPS MSCs with 5 mmol/l of metformin reduced the percentage of abnormal nuclei from 60% pre-treatment to less than 40% after treatment (wild-type MSCs presented less than 20% of abnormal nuclei). The metformin-induced reduction in abnormal nuclei was comparable to the reference treatment tipifarnib (1 μmol/1), a farnesyl-transferase inhibitor [24]. Additionally, as HGPS MSCs are characterized by premature osteogenic differentiation (indicated by increased alkaline phosphatase activity compared to wild-type osteogenic progenitor cells), 5 mmol/l of metformin led to a significant rescue of alkaline phosphatase activity in HGPS osteogenic progenitor cells, comparable to levels found in tipifarnib-treated cells [24].
Park et al. also characterized the cellular phenotypes of primary dermal fibroblasts derived from HGPS patients of different ages and showed increased staining of senescence-associated beta-galactosidase (SA-β-gal, an indicator of cellular senescence) and increased levels of mitochondrial superoxide in HG8 cells (from an 8 year old patient) compared to HG3 (3 year old) and HG5 (5 year old) cells [25]. All cells expressed the toxic protein progerin, superoxide dismutase 2 (SOD2, a mitochondrial antioxidant enzyme) was highest in normal fibroblasts and lowest in HG8 cells, and cellular proliferation rate slowed at an earlier time in HGPS cells compared to normal fibroblasts [25].
Utilizing HG8 cells (which demonstrated the highest levels of senescence), the authors also elucidated the effects of metformin (2mM), rapamycin (200nM), or a combination of both drugs on the nuclear phenotype of HGPS cells. Rapamycin significantly decreased the number of nuclei with abnormal morphology and metformin treatment also led to a significant increase in the number of cells with normal nuclei compared to control-treated cells [25]. Metformin also reduced senescence in HGPS cells (i.e. reduction in SA-β-gal staining) and co-treatment with rapamycin and metformin led to an approximately 34.2 % inhibition of senescence, with similar results observed in HG3 and HG8 cells [25]. Metformin, rapamycin, or co-treatment with both compounds led to a significant reduction in the number of cells containing more than 20 γ-H2AX foci (a marker of DNA damage) in HG8, HG3, and HG5 cells, indicating that metformin increases the efficiency of DNA repair in HGPS cells [25].
Metformin also exerted antioxidant effects in HGPS cells, as evidenced by a significant decrease in ROS production and mitochondrial superoxide formation compared to control cells as well as an upregulation of SOD2 mRNA expression in aged BALB/c mice (>18 months old) [25].
Most importantly, metformin treatment at 2 and 20mM reduced progerin protein expression by approximately 20 % and 60 %, respectively, compared to mock-treated cells and increased the presence of normal nuclear phenotypes in HGPS cells [25]. Metformin treatment also significantly increased the phosphorylation and activation of AMPK in HGPS cells. Furthermore, western blot analysis indicated that rapamycin increased AMPK activation as well.
Strikingly, AMPK activation also promotes oocyte meiotic induction and maturation (processes that are critical for efficient oocyte activation) and AMPK has recently been found localized across the entire acrosome in human spermatozoa [8,26,27]. The induction of cellular stress (e.g. increases in reactive oxygen species, intracellular calcium, and/or AMP/ATP ratio increase), which activates AMPK, also promotes oocyte meiotic induction/maturation, oocyte activation, and the acrosome reaction in human sperm, processes critical for the creation of all human life [26,28,29]. Indeed, the calcium ionophore ionomycin, which activates AMPK, is commonly used to promote latent HIV-1 reactivation and is extensively used to activate human oocytes, creating normal healthy children [29-31]. Such evidence indicates and further substantiates the novel and provocative assertion that AMPK activation links the amelioration of pathological cellular defects in FXS, HGPS, and DS with HIV-1 latency and replication, adult and cancer stem cells, learning and memory, and the creation of all human life [5-10].
https://www.linkedin.com/pulse/metformin-shown-first-time-inhibit-malaria-parasite-human-finley

References:
Ruivo MT, Vera IM, Sales-Dias J, et al. Host AMPK Is a Modulator of Plasmodium Liver Infection. Cell Rep. 2016 Sep 6;16(10):2539-45.
Soto-Acosta R, Bautista-Carbajal P, Cervantes-Salazar M, Angel-Ambrocio AH, Del Angel RM. DENV up-regulates the HMG-CoA reductase activity through the impairment of AMPK phosphorylation: A potential antiviral target. PLoS Pathog. 2017 Apr 6;13(4):e1006257.
Mehla R, Bivalkar-Mehla S, Zhang R, et al. Bryostatin modulates latent HIV-1 infection via PKC and AMPK signaling but inhibits acute infection in a receptor independent manner. PLoS One. 2010 Jun 16;5(6):e11160.
Zhang HS, Wu MR. SIRT1 regulates Tat-induced HIV-1 transactivation through activating AMP-activated protein kinase. Virus Res. 2009 Dec;146(1-2):51-7.
Finley J. Elimination of cancer stem cells and reactivation of latent HIV-1 via AMPK activation: Common mechanism of action linking inhibition of tumorigenesis and the potential eradication of HIV-1 (manuscript in press).
Finley J. Alteration of splice site selection in the LMNA gene and inhibition of progerin production via AMPK activation. Med Hypotheses. 2014 Nov;83(5):580-7.
Finley J. Reactivation of latently infected HIV-1 viral reservoirs and correction of aberrant alternative splicing in the LMNA gene via AMPK activation: Common mechanism of action linking HIV-1 latency and Hutchinson-Gilford progeria syndrome. Med Hypotheses. 2015 Sep;85(3):320-32.
Finley J. Oocyte activation and latent HIV-1 reactivation: AMPK as a common mechanism of action linking the beginnings of life and the potential eradication of HIV-1. Med Hypotheses. 2016 Aug;93:34-47.
Finley J. AMPK activation as a common mechanism of action linking the effects of diverse compounds that ameliorate accelerated cellular aging defects in Hutchinson-Gilford progeria syndrome (manuscript submitted).
Finley J. Facilitation of hippocampal long-term potentiation and reactivation of latent HIV-1 via AMPK activation: Common mechanism of action linking learning, memory, and the potential eradication of HIV-1 (manuscript submitted).
Zainal N, Chang CP, Cheng YL, et al. Resveratrol treatment reveals a novel role for HMGB1 in regulation of the type 1 interferon response in dengue virus infection. Sci Rep. 2017 Feb 20;7:42998.
Heredia A, Davis C, Amin MN, et al. Targeting host nucleotide biosynthesis with resveratrol inhibits emtricitabine-resistant HIV-1. AIDS. 2014 Jan 28;28(3):317-23.
Pan XY, Zhao W, Zeng XY, et al. Heat Shock Factor 1 Mediates Latent HIV Reactivation. Sci Rep. 2016 May 18;6:26294.
Wang H, Sharma L, Lu J, Finch P, Fletcher S, Prochownik EV. Structurally diverse c-Myc inhibitors share a common mechanism of action involving ATP depletion. Oncotarget. 2015 Jun 30;6(18):15857-70.
Wang DT, He J, Wu M, Li SM, Gao Q, Zeng QP. Artemisinin mimics calorie restriction to trigger mitochondrial biogenesis and compromise telomere shortening in mice. PeerJ. 2015 Mar 5;3:e822.
Darcis G, Kula A, Bouchat S, et al. An In-Depth Comparison of Latency-Reversing Agent Combinations in Various In Vitro and Ex Vivo HIV-1 Latency Models Identified Bryostatin-1+JQ1 and Ingenol-B+JQ1 to Potently Reactivate Viral Gene Expression. PLoS Pathog. 2015 Jul 30;11(7):e1005063.
Dy ABC, Tassone F, Eldeeb M, Salcedo-Arellano MJ, Tartaglia N, Hagerman R. Metformin as Targeted Treatment in Fragile X Syndrome. Clin Genet. 2017 Apr 24. doi: 10.1111/cge.13039. [Epub ahead of print].
Monyak RE, Emerson D, Schoenfeld BP, et al. Insulin signaling misregulation underlies circadian and cognitive deficits in a Drosophila fragile X model. Mol Psychiatry. 2016 Apr 19. doi: 10.1038/mp.2016.51. [Epub ahead of print].
Gantois I, Khoutorsky A, Popic J, et al. Metformin ameliorates core deficits in a Fragile X syndrome mouse model. Nat Med. 2017 May 15. doi: 10.1038/nm.4335. [Epub ahead of print].
Izzo A, Nitti M, Mollo N, et al. Metformin restores the mitochondrial network and reverses mitochondrial dysfunction in Down syndrome cells. Hum Mol Genet. 2017 Jan 13. pii: ddx016. doi: 10.1093/hmg/ddx016. [Epub ahead of print].
De la Torre R, De Sola S, Pons M, et al. Epigallocatechin-3-gallate, a DYRK1A inhibitor, rescues cognitive deficits in Down syndrome mouse models and in humans. Mol Nutr Food Res. 2014 Feb;58(2):278-88.
Valenti D, de Bari L, de Rasmo D, et al. The polyphenols resveratrol and epigallocatechin-3-gallate restore the severe impairment of mitochondria in hippocampal progenitor cells from a Down syndrome mouse model. Biochim Biophys Acta. 2016 Jun;1862(6):1093-104.
Valenti D, De Rasmo D, Signorile A, et al. Epigallocatechin-3-gallate prevents oxidative phosphorylation deficit and promotes mitochondrial biogenesis in human cells from subjects with Down's syndrome. Biochim Biophys Acta. 2013 Apr;1832(4):542-52.
Egesipe, Blondel, Cicero, et al. Metformin decreases progerin expression and alleviates pathological defects of Hutchinson–Gilford progeria syndrome cells. npj Aging and Mechanisms of Disease 2, Article number: 16026 (2016); http://www.nature.com/articles/npjamd201626?WT.feed_name=subjects_drug-discovery
Park SK, Shin OS. Metformin Alleviates Aging Cellular Phenotypes in Hutchinson-Gilford Progeria Syndrome Dermal Fibroblasts. Exp Dermatol. 2017 Feb 13. doi: 10.1111/exd.13323. [Epub ahead of print].
LaRosa C, Downs SM. Stress stimulates AMP-activated protein kinase and meiotic resumption in mouse oocytes. Biol Reprod. 2006 Mar;74(3):585-92.
Calle-Guisado V, de Llera AH, Martin-Hidalgo D, et al. AMP-activated kinase in human spermatozoa: identification, intracellular localization, and key function in the regulation of sperm motility. Asian J Androl. 2016 Sep 27. doi: 10.4103/1008-682X.185848. [Epub ahead of print].
de Lamirande E, Tsai C, Harakat A, Gagnon C. Involvement of reactive oxygen species in human sperm arcosome reaction induced by A23187, lysophosphatidylcholine, and biological fluid ultrafiltrates. J Androl. 1998 Sep-Oct;19(5):585-94.
Deemeh MR, Tavalaee M, Nasr-Esfahani MH. Health of children born through artificial oocyte activation: a pilot study. Reprod Sci. 2015 Mar;22(3):322-8.
Tamás P, Hawley SA, Clarke RG, et al. Regulation of the energy sensor AMP-activated protein kinase by antigen receptor and Ca2+ in T lymphocytes. J Exp Med. 2006 Jul 10;203(7):1665-70.
Spina CA, Anderson J, Archin NM, et al. An in-depth comparison of latent HIV-1 reactivation in multiple cell model systems and resting CD4+ T cells from aviremic patients. PLoS Pathog 2013;9(12):e1003834.
#malaria#progeria#ampk#metformin#fragile x syndrome#down syndrome#dengue virus#sperm#oocyte#HIV/AIDS
0 notes
Text
Metformin shown for the first time to improve behavior in humans with Fragile X Mental Retardation Syndrome: AMPK links Progeria, FXS, & Down syndrome

By Vanellus Foto (Own work) [GFDL (http://www.gnu.org/copyleft/fdl.html) or CC BY-SA 3.0 (http://creativecommons.org/licenses/by-sa/3.0)], via Wikimedia Commons; "Hutchinson-Gilford Progeria Syndrome" by The Cell Nucleus and Aging: Tantalizing Clues and Hopeful Promises. Scaffidi P, Gordon L, Misteli T; By Peter Saxon (Own work) [CC BY-SA 4.0 (http://creativecommons.org/licenses/by-sa/4.0)], via Wikimedia Commons
A study recently published in the journal Clinical Genetics in April of 2017 by researchers from the University of California, Davis Medical Center and the University of Colorado School of Medicine demonstrated for the first time that metformin consistently improved behavior in several patients diagnosed with Fragile X Syndrome (FXS), a genetic disorder characterized by intellectual disability and significant deficits in neurological function and cognitive development [1]. An improvement in behavior was documented in the Aberrant Behavior Checklist (ABC) for all cases, as evidenced by consistent improvements (i.e. lower scores compared to pre-metformin treatment) in social avoidance, irritability, hyperactivity, and social unresponsiveness (see table below) as well as improvements in language and conversational skills reported by familial caretakers [1]. Interestingly, a study published in 2016 showed that metformin rescued and restored memory deficits in a Drosophila (fly) model of FXS and a soon-to-be published study currently in press also demonstrated that metformin restored behavioral and morphological defects in a mouse model of FXS [2-4]. In 2014 and 2015, I was first to hypothesize and publish that AMPK activation via compounds including metformin would beneficially alter gene splicing and alleviate accelerated aging defects in cells derived from patients with the genetic disorder Hutchinson-Gilford progeria syndrome, which was later substantiated by studies published in 2016 and 2017 (see below). Because AMPK activation has been shown to restore severe mitochondrial impairment in hippocampal progenitor cells from a Down syndrome mouse model and metformin reverses mitochondrial dysfunction in human fetal cells with Down syndrome, the activation of AMPK via the induction of cellular stress (i.e. AMP/ATP ratio increase, reactive oxygen species generation, and/or intracellular calcium increases, etc.) links Fragile X syndrome, Hutchison-Gilford progeria syndrome, and Down syndrome with the therapeutic effects of structurally dissimilar AMPK activators including metformin, resveratrol, and lithium. Indeed, in my recently submitted manuscripts and publications, I initially propose that all three of the aforementioned genetic disorders as well as learning and memory (i.e. hippocampal long-term potentiation), adult and cancer stem cells, and the creation all human life (via oocyte activation/sperm acrosome reaction) are connected to HIV-1 latency via AMPK activation [5-10]. Fragile X syndrome (FXS) is the most common inherited cause of autism spectrum disorder (ASD) and intellectual disability (ID) and results from a trinucelotide expansion-induced hypermethylation and silencing of the Fragile X Mental Retardation 1 gene (FMR1) and resultant silencing of the FMR1 gene product FMR1 protein (FMRP) [1]. Symptomatic behaviors associated with FXS include aggression, self-injurious behaviors, anxiety, and attention deficit hyperactivity disorder (ADHD). In the study, several patients, listed below, were treated clinically with metformin at the Fragile X Treatment and Research Center at the University of California, Davis Medical Center MIND Institute for at least six months along with assessments of medical and behavioral co-morbidities and laboratory studies before and after treatment [1]. Case 1: Case 1 was a 19 year-old male diagnosed with FXS and ASD at age 5 with a history of hyperphagia, characteristic features of FXS including macroorchidism (abnormally large testicles), and behavioral issues including aggression, hand biting, hyperactivity, ADHD, poor eye contact, and skin picking and scratching [1]. After being placed on several mediations (e.g. minocycline, divalproex, etc.), he was started on metformin 500 mg twice a day (bid) and subsequently experienced significant improvements in hyperphagia, irritability, aggression, communication, and behavior. It was also noted that the patient no longer focuses on food (as he did for many years), now enjoys outdoor activities, and has considerably improved self-esteem [1]. Case 2: Case 2 was a 13 year-old boy with FXS who at age 10 had a Leiter IQ of 44 and an Autism Diagnostic Observation Schedule (ADOS) total score of 25 (well into ASD range) [1]. Behavioral symptoms consisted of hyperphagia, severe hyperactivity and impulsivity, significant aggression characterized by kicking and hitting at home and at school, and escalating aggressiveness towards his mother (which led to her becoming depressed), leading to his placement in a residential group home to control his eating and aggression [1]. After starting metformin 500 mg bid at age 12, his mother and care center noted significant improvements in his behavior over the course of a year, including the patient being more calm and patient, negotiating with teachers more, displaying a better understanding when things were explained to him, being more responsive to rewards, and able to follow directions better and work longer with less agitation and aggression [1]. Case 3: Case 3 was another 19-year-old boy diagnosed at age 3 with FXS and had also been diagnosed with ADHD, ASD, and specific phobias [1]. Behavioral symptoms included impulsive episodic outbursts, persistent hyperphagia, skin pricking, enuresis (inability to control urination), and binge eating to the point of vomiting. After starting metformin 500 mg bid, the patient experienced significant behavioral improvements including a decrease in tantrums, improvement in communication ability, and cessation of head-banging and other self-injurious behavior [1]. Case 4 Case 4 was a 60-year-old female diagnosed with FXS and mild ID currently residing in a group home with a history of morbid obesity along with repetitive behavior, memory deficits, agitation, and anxiety that caused concern among her caregivers [1]. After starting on metformin 500 mg bid, the patient is no longer overeating, has lost 41.3 pounds, and her irritability and social responsiveness has improved (see table below) [1]. Case 5 Case 5 was a 31-year-old man diagnosed with FXS and ASD with a full scale IQ of 54 on the WISC III. The patient exhibited macroorchidism and also had problems with anxiety and tantrums [1]. After starting metformin 500 mg bid, the patient’s appetite decreased, more language was noted by his speech and language therapist, and he has improved self-initiative. Also, he “will carry out chores such as cleaning his room or cleaning the house without excessive urging or reminders, helps his mom with gardening and is able to coordinate the rake better, has a lot of trivia knowledge, and asks more inquisitive questions. The mother is able to have a discussion with him even about emotional issues, he is able to talk about his grief and to relate his father's death to his excessive smoking and drinking problems, and he seems to be putting together concepts better and having back-and-forth conversations.” [1]. Case 6 Case 6 was originally seen at 10 years of age and exhibited typical characteristics of FXS on physical examination, including soft skin, flat feet, and poor eye contact [1]. DNA testing confirmed a diagnosis of FXS and the patient demonstrated a full scale IQ of 50. The patient also displayed a dramatic increase in anxiety, aversion to people, and intermittent aggression and headaches due to a traumatic episode wherein the patient had to be tasered by police at a hospital due to his aggression [1]. After starting metformin 500 mg bid at age 24, he has not experienced on outburst, “seems happier and more active, has improvement in language, is able to carry out a two way conversation, has become more outgoing socially with less anxiety, and eats without gorging himself.” [1]. Case 7 Case 7 was a 4 year old boy diagnosed with FXS at 14 months and displayed features typical of FXS, including prominent ears, a broad forehead, and flat feet [1]. He had motor and language delays at an early age and the parents self-treated the child with a cannabinoid tincture at age 2 due to staring spells and a lack of language. The parents noted that the tincture led to a decrease in staring spells, increased verbalization, and improved anxiety [1]. “After 6 months of a dose of metformin 50 mg the family felt he has fewer outbursts or tantrums, better attention, less hyperactivity and improvements in his language. Developmental testing after 4 months of treatment at 4.5 years showed expressive language at the 31 month age equivalent.” [1]. As demonstrated by these case studies, it appears that metformin significantly improves cognitive and behavioral deficits in the genetic disorder FXS. Additionally, as noted above, metformin has recently been shown to rescue and restore memory deficits in a Drosophila model of FXS and a study in press has shown that metformin corrected social novelty impairment, reduced testicular weight, decreased repetitive grooming, rescued excessive long-term depression and dendritic spine abnormalities, and altered excitatory synaptic transmission in an FXS mouse model, indicating that metformin likely exerts significant beneficial effects in disparate genetic disorders [2-4]. Indeed, metformin has also been shown to correct alternative splicing defects in primary myoblasts derived from patients with myotonic dystrophy type I (DM1) as well as in derivatives of embryonic stem cells that carry the DM1 mutation via activation of AMPK. DM1 is a genetic disorder characterized by muscle wasting that is also caused by the expansion of a trinucleotide repeat, similar to FXS [11]. As further explained below, because metformin activates AMPK and alleviates accelerated aging defects in cells from Hutchinson-Gilford progeria syndrome (HGPS) patients and because metformin also rescues mitochondrial defects in human fetal cells with Down syndrome (DS), AMPK activation induced by cellular stress (i.e. AMP/ATP ratio increase, reactive oxygen species generation, and/or intracellular calcium increases, etc.) links the amelioration and/or reversal of pathological cellular defects in FXS, HGPS, DM1, and DS with HIV-1 latency, adult and cancer stem cells, learning and memory, and the creation all human life (via oocyte activation/sperm acrosome reaction), hypotheses that I first proposed in several publications and pending manuscripts [5-10]. Down syndrome (DS) is caused by either a partial or full trisomy of chromosome 21 and is associated with impairments in cognition, learning and memory, and disorders of the immune system [12]. Human and animal studies strongly suggest that mitochondrial dysfunction is associated with pathogenic features of DS and mitochondrial abnormalities have been found in all DS cells analyzed in culture to date, including neurons, astrocytes, and lymphocytes [12]. Indeed, in DS human fetal fibroblasts, PGC-1α, a master regulator of mitochondrial biogenesis, is down regulated at both the mRNA and protein levels and AMPK phosphorylation of the PGC-1α protein is essential for PGC-1α-dependent induction of the PGC-1α promoter [12]. Izzo et al. initially observed that DS human fetal fibroblasts (DS-HFFs) displayed a 40%-50% reduction in PGC-1α mRNA and protein levels compared to non-trisomic counterparts (N-HFFs). DS-HFFs treated with metformin however led to a significant increase in PGC-1α at both the mRNA and protein levels compared to untreated control cells, an increase in the mRNA levels of NRF-1 and TFAM (PGC-1α target genes critical in promoting mitochondrial biogenesis), and an increase in mtDNA content [12]. Additionally, compared to N-HFFs, DS-HFFs were characterized by a decrease in basal oxygen consumption rate (OCR, ~55% inhibition), a decrease in OCR related to ATP production (~58% inhibition), and a reduction in maximal respiratory capacity (~58% inhibition). DS-HFFs treated with metformin increased basal OCR, OCR related to ATP production, ATP concentration, mitochondrial membrane potential, and maximal respiratory capacity, thus restoring mitochondrial respiration in DS-HFFs [12]. Furthermore, compared to N-HFFs, DS-HFFs exhibited extensive mitochondrial damage, as evidenced by swollen cristae (~60%-90% of DS-HFFs vs. ~15% of N-HFFs), mitochondria with intra-oedema (~40% of DS-HFFs vs. ~6% of N-HFFs), and an increase in the number of damaged mitochondria (~90% in DS-HFFs vs. ~25% in N-HFFs). Metformin-treated DS-HFFs, compared to untreated cells, displayed narrower cristae with widths that were comparable to N-HFFs cells, fewer damaged mitochondria (~40%), and fewer mitochondria with intra-oedema (~5%) [12]. Interestingly, as mitochondrial fusion also plays an important role in mitochondrial functionality, the mRNA and protein expression of OPA1 and MFN2 (genes that regulate mitochondrial fusion) were significantly down-regulated in DS-HFFs vs. N-HFFs and correlated with extensive mitochondrial fragmentation in DS-HFFs. Treatment of DS-HFFs with metformin significantly increased the mRNA and protein levels of OPA1 and MFN2 and also reduced fragmentation of the mitochondrial network, indicating that metformin induces correction of mitochondrial phenotype by also restoring mitochondrial fusion machinery [12]. The AMPK activator EGCG also reverses cognitive defects in DS patients, promotes oxidative phosphorylation and mitochondrial biogenesis in lymphoblasts and fibroblasts from DS patients (i.e. increased levels and activity of PGC-1α, NRF-1 and TFAM), and restores oxidative phosphorylation, mitochondrial biogenesis, and improves hippocampal neural progenitor cell proliferation in a DS mouse model (Ts65Dn) in combination with the polyphenol resveratrol (i.e. increase in PGC-1α) in an AMPK-dependent manner [13-15]. Mitochondrial dysfunction is also a prominent feature associated with the accelerated aging disorder Hutchison-Gilford progeria syndrome (HGPS) and metformin has recently been shown to alleviate accelerated aging defects, activate AMPK, and beneficially alter gene splicing in HGPS patient cells. Egesipe et al. initially demonstrated, using mesenchymal stem cells (MSCs) derived from HGPS induced pluripotent stem cells (i.e. HGPS MSCs), a significant dose-dependent decrease in SRSF1 mRNA levels after metformin treatment and up to a 40% decrease in SRSF1 protein levels after treatment with 5 mmol/l of metformin (SRSF1 is a gene splicing factor that is upregulated in HGPS cells and causes faulty gene splicing of the LMNA gene leading to increased production of the toxic protein progerin) [16]. A significant decrease was also observed in both lamin A and progerin mRNA expression in HGPS MSCs treated with 5 mmol/l metformin, with progerin mRNA expression and protein levels reduced to levels lower than that of lamin A mRNA expression and protein levels, indicating that metformin-induced inhibition of SRSF1 led to an increase in the lamin A/progerin ratio and thus beneficially altered gene splicing [16]. The results obtained using HGPS MSCs were also replicated in additional in vitro cell models, with 5 mmol/l of metformin decreasing progerin mRNA expression up to 50% in LmnaG609G/G609G mouse primary fibroblasts (HGPS mouse model) and decreasing both lamin A and progerin mRNA expression in primary HGPS fibroblasts [16]. Interestingly, 5 mmol/l of metformin also decreased progerin mRNA expression in wild-type/normal MSCs that had been incubated with a compound that induces progerin expression, indicating that metformin may also prove beneficial in reducing progerin levels in normal humans [16]. Most importantly, however, treatment of HGPS MSCs with 5 mmol/l of metformin reduced the percentage of abnormal nuclei from 60% pre-treatment to less than 40% after treatment (wild-type MSCs presented less than 20% of abnormal nuclei). The metformin-induced reduction in abnormal nuclei was comparable to the reference treatment tipifarnib (1 μmol/1), a farnesyl-transferase inhibitor [16]. Additionally, as HGPS MSCs are characterized by premature osteogenic differentiation (indicated by increased alkaline phosphatase activity compared to wild-type osteogenic progenitor cells), 5 mmol/l of metformin led to a significant rescue of alkaline phosphatase activity in HGPS osteogenic progenitor cells, comparable to levels found in tipifarnib-treated cells [16]. Park et al. also characterized the cellular phenotypes of primary dermal fibroblasts derived from HGPS patients of different ages and showed increased staining of senescence-associated beta-galactosidase (SA-β-gal, an indicator of cellular senescence) and increased levels of mitochondrial superoxide in HG8 cells (from an 8 year old patient) compared to HG3 (3 year old) and HG5 (5 year old) cells [17]. All cells expressed the toxic protein progerin, superoxide dismutase 2 (SOD2, a mitochondrial antioxidant enzyme) was highest in normal fibroblasts and lowest in HG8 cells, and cellular proliferation rate slowed at an earlier time in HGPS cells compared to normal fibroblasts [17]. Utilizing HG8 cells (which demonstrated the highest levels of senescence), the authors also elucidated the effects of metformin (2mM), rapamycin (200nM), or a combination of both drugs on the nuclear phenotype of HGPS cells. Rapamycin significantly decreased the number of nuclei with abnormal morphology and metformin treatment also led to a significant increase in the number of cells with normal nuclei compared to control-treated cells [17]. Metformin also reduced senescence in HGPS cells (i.e. reduction in SA-β-gal staining) and co-treatment with rapamycin and metformin led to an approximately 34.2 % inhibition of senescence, with similar results observed in HG3 and HG8 cells [3]. Metformin, rapamycin, or co-treatment with both compounds led to a significant reduction in the number of cells containing more than 20 γ-H2AX foci (a marker of DNA damage) in HG8, HG3, and HG5 cells, indicating that metformin increases the efficiency of DNA repair in HGPS cells [17]. Metformin also exerted antioxidant effects in HGPS cells, as evidenced by a significant decrease in ROS production and mitochondrial superoxide formation compared to control cells as well as an upregulation of SOD2 mRNA expression in aged BALB/c mice (>18 months old) [17]. Most importantly, metformin treatment at 2 and 20mM reduced progerin protein expression by approximately 20 % and 60 %, respectively, compared to mock-treated cells and increased the presence of normal nuclear phenotypes in HGPS cells [17]. Metformin treatment also significantly increased the phosphorylation and activation of AMPK in HGPS cells. Furthermore, western blot analysis indicated that rapamycin increased AMPK activation as well. Strikingly, metformin has recently been shown to inhibit dengue virus replication in human liver cells in an AMPK-dependent manner, resveratrol (an AMPK activator) has recently been shown to reactivate latent HIV-1, and HIV-1 replication is significantly inhibited via knockdown of AMPK, indicating that AMPK activation leads to both the reactivation of latent viruses (facilitating immune system detection and destruction) as well as inhibition of productive viral replication [18-20]. AMPK activation also promotes oocyte meiotic induction and maturation (processes that are critical for efficient oocyte activation) and AMPK has recently been found localized across the entire acrosome in human spermatozoa [7,21,22]. The induction of cellular stress (e.g. increases in ROS, intracellular calcium, and/or AMP/ATP ratio increase), which activates AMPK, also promotes oocyte meiotic induction/maturation, oocyte activation, and the acrosome reaction in human sperm, processes critical for the creation of all human life [21,23,24]. Indeed, the calcium ionophore ionomycin, which activates AMPK, is commonly used to promote latent HIV-1 reactivation and is extensively used to activate human oocytes, creating normal healthy children [24-26]. Such evidence indicates and further substantiates the novel and provocative assertion that AMPK activation links the amelioration of pathological cellular defects in FXS, HGPS, DS, and DM1 with HIV-1 latency, adult and cancer stem cells, learning and memory, and the creation of all human life [5-10]. https://www.linkedin.com/pulse/metformin-shown-first-time-improve-behavior-humans-fragile-finley

*Table 2 adapted from: Dy ABC, Tassone F, Eldeeb M, Salcedo-Arellano MJ, Tartaglia N, Hagerman R. Metformin as Targeted Treatment in Fragile X Syndrome. Clin Genet. 2017 Apr 24. doi: 10.1111/cge.13039. [Epub ahead of print].

References
Dy ABC, Tassone F, Eldeeb M, Salcedo-Arellano MJ, Tartaglia N, Hagerman R. Metformin as Targeted Treatment in Fragile X Syndrome. Clin Genet. 2017 Apr 24. doi: 10.1111/cge.13039. [Epub ahead of print].
Monyak RE, Emerson D, Schoenfeld BP, et al. Insulin signaling misregulation underlies circadian and cognitive deficits in a Drosophila fragile X model. Mol Psychiatry. 2016 Apr 19. doi: 10.1038/mp.2016.51. [Epub ahead of print].
Gantois I, Khoutorsky A, Popic J, et al. Metformin ameliorates core deficits in a Fragile X syndrome mouse model. Nat Med. 2017; In press.
Gantois I, Khoutorsky A, Popic J, et al. Chronic administration of metformin restores behavioral and morphological abnormalities in the Fragile X Syndrome mouse model. Program No. 392.06/C23. 2016 Neuroscience Meeting Planner. San Diego, CA: Society for Neuroscience, 2016. Online.
Finley J. Alteration of splice site selection in the LMNA gene and inhibition of progerin production via AMPK activation. Med Hypotheses. 2014 Nov;83(5):580-7.
Finley J. Reactivation of latently infected HIV-1 viral reservoirs and correction of aberrant alternative splicing in the LMNA gene via AMPK activation: Common mechanism of action linking HIV-1 latency and Hutchinson-Gilford progeria syndrome. Med Hypotheses. 2015 Sep;85(3):320-32.
Finley J. Oocyte activation and latent HIV-1 reactivation: AMPK as a common mechanism of action linking the beginnings of life and the potential eradication of HIV-1. Med Hypotheses. 2016 Aug;93:34-47.
Finley J. Elimination of cancer stem cells and reactivation of latent HIV-1 via AMPK activation: Common mechanism of action linking inhibition of tumorigenesis and the potential eradication of HIV-1 (manuscript in press).
Finley J. AMPK activation as a common mechanism of action linking the effects of diverse compounds that ameliorate accelerated cellular aging defects in Hutchinson-Gilford progeria syndrome (manuscript submitted).
Finley J. Facilitation of hippocampal long-term potentiation and reactivation of latent HIV-1 via AMPK activation: Common mechanism of action linking learning, memory, and the potential eradication of HIV-1 (manuscript submitted).
Laustriat D, Gide J, Barrault L, et al. In Vitro and In Vivo Modulation of Alternative Splicing by the Biguanide Metformin. Mol Ther Nucleic Acids. 2015 Nov 3;4:e262.
Izzo A, Nitti M, Mollo N, et al. Metformin restores the mitochondrial network and reverses mitochondrial dysfunction in Down syndrome cells. Hum Mol Genet. 2017 Jan 13. pii: ddx016. doi: 10.1093/hmg/ddx016. [Epub ahead of print].
De la Torre R, De Sola S, Pons M, et al. Epigallocatechin-3-gallate, a DYRK1A inhibitor, rescues cognitive deficits in Down syndrome mouse models and in humans. Mol Nutr Food Res. 2014 Feb;58(2):278-88.
Valenti D, de Bari L, de Rasmo D, et al. The polyphenols resveratrol and epigallocatechin-3-gallate restore the severe impairment of mitochondria in hippocampal progenitor cells from a Down syndrome mouse model. Biochim Biophys Acta. 2016 Jun;1862(6):1093-104.
Valenti D, De Rasmo D, Signorile A, et al. Epigallocatechin-3-gallate prevents oxidative phosphorylation deficit and promotes mitochondrial biogenesis in human cells from subjects with Down's syndrome. Biochim Biophys Acta. 2013 Apr;1832(4):542-52.
Egesipe, Blondel, Cicero, et al. Metformin decreases progerin expression and alleviates pathological defects of Hutchinson–Gilford progeria syndrome cells. npj Aging and Mechanisms of Disease 2, Article number: 16026 (2016); http://www.nature.com/articles/npjamd201626?WT.feed_name=subjects_drug-discovery
Park SK, Shin OS. Metformin Alleviates Aging Cellular Phenotypes in Hutchinson-Gilford Progeria Syndrome Dermal Fibroblasts. Exp Dermatol. 2017 Feb 13. doi: 10.1111/exd.13323. [Epub ahead of print].
Soto-Acosta R, Bautista-Carbajal P, Cervantes-Salazar M, Angel-Ambrocio AH, Del Angel RM. DENV up-regulates the HMG-CoA reductase activity through the impairment of AMPK phosphorylation: A potential antiviral target. PLoS Pathog. 2017 Apr 6;13(4):e1006257.
Zeng X, Pan X, Xu X, et al. Resveratrol Reactivates Latent HIV through Increasing Histone Acetylation and Activating Heat Shock Factor 1. J Agric Food Chem. 2017 May 4. doi: 10.1021/acs.jafc.7b00418.
Zhou H, Xu M, Huang Q, et al. Genome-scale RNAi screen for host factors required for HIV replication. Cell Host Microbe. 2008 Nov 13;4(5):495-504.
LaRosa C, Downs SM. Stress stimulates AMP-activated protein kinase and meiotic resumption in mouse oocytes. Biol Reprod. 2006 Mar;74(3):585-92.
Calle-Guisado V, de Llera AH, Martin-Hidalgo D, et al. AMP-activated kinase in human spermatozoa: identification, intracellular localization, and key function in the regulation of sperm motility. Asian J Androl. 2016 Sep 27. doi: 10.4103/1008-682X.185848. [Epub ahead of print].
de Lamirande E, Tsai C, Harakat A, Gagnon C. Involvement of reactive oxygen species in human sperm arcosome reaction induced by A23187, lysophosphatidylcholine, and biological fluid ultrafiltrates. J Androl. 1998 Sep-Oct;19(5):585-94.
Deemeh MR, Tavalaee M, Nasr-Esfahani MH. Health of children born through artificial oocyte activation: a pilot study. Reprod Sci. 2015 Mar;22(3):322-8.
Tamás P, Hawley SA, Clarke RG, et al. Regulation of the energy sensor AMP-activated protein kinase by antigen receptor and Ca2+ in T lymphocytes. J Exp Med. 2006 Jul 10;203(7):1665-70.
Spina CA, Anderson J, Archin NM, et al. An in-depth comparison of latent HIV-1 reactivation in multiple cell model systems and resting CD4+ T cells from aviremic patients. PLoS Pathog 2013;9(12):e1003834.
0 notes
Text
Metformin shown for the first time to restore mitochondria in human fetal cells with Down syndrome: AMPK links Progeria, Down syndrome, & Dengue virus

By Vanellus Foto (Own work) [GFDL (http://www.gnu.org/copyleft/fdl.html) or CC BY-SA 3.0 (http://creativecommons.org/licenses/by-sa/3.0)], via Wikimedia Commons; "Hutchinson-Gilford Progeria Syndrome" by The Cell Nucleus and Aging: Tantalizing Clues and Hopeful Promises. Scaffidi P, Gordon L, Misteli T
In line with recent evidence demonstrating for the first time that metformin improved accelerated aging defects, activated AMPK, and beneficially altered gene splicing in cells derived from Hutchinson-Gilford progeria syndrome (HGPS) patients and exerted significant antiviral effects in dengue virus-infected human liver cells in an AMPK-dependent manner, a study published in the journal Human Molecular Genetics in January of 2017 showed for the first time that metformin corrected mitochondrial dysfunction in human fetal cells taken from aborted fetuses that had been diagnosed with Down syndrome (DS) [1-4]. Metformin induced the expression and activity of PGC-1α (a master regulator of mitochondrial biogenesis) and the PGC-1α target genes NRF-1 and TFAM, enhanced oxygen consumption and ATP production, reversed mitochondrial fragmentation, and increased the expression of the mitochondrial fusion genes OPA1 and MFN2, leading to enhanced mitochondrial activity and strongly promoting mitochondrial biogenesis. Recent studies have also shown that the polyphenols resveratrol and epigallocatechin-3-gallate (EGCG) restored mitochondrial functionality and improved hippocampal neural progenitor cell proliferation in a mouse model of DS in an AMPK-dependent manner, while EGCG significantly reversed cognitive deficits in human DS patients [5,6].
Interestingly, as metformin inhibits dengue virus replication in human liver cells in an AMPK-dependent manner, both EGCG and resveratrol have been shown to increase the lifespan of the mosquito Aedes aegypti (a vector for dengue and Zika viruses), with resveratrol also boosting the immune response and activating AMPK in Aedes aegypti [7]. Recent evidence also demonstrated that resveratrol inhibits dengue virus replication in human liver cells, inhibits emtricitabine-resistant HIV-1, and reactivates latent HIV-1, facilitating immune system detection and destruction [8-10]. EGCG has also shown anti-HIV-1 activity [11]. Although structurally dissimilar, metformin, EGCG, and resveratrol each activate AMPK and AMPK activation links reversal of accelerated aging defects in HGPS with rescue of mitochondrial function in Down syndrome, inhibition of dengue virus and HIV-1 replication, and reactivation of latent HIV-1. Such an interconnectedness, as further explained below, provides substantial support for my initial proposal and publications that AMPK activation links HGPS, latent HIV-1 reactivation, Down syndrome (manuscript in press) and the beginnings of all human life (via oocyte activation and the acrosome reaction) [12-14].
Down syndrome (DS) is caused by either a partial or full trisomy of chromosome 21 and is associated with impairments in cognition, learning and memory, and disorders of the immune system [1]. Human and animal studies strongly suggest that mitochondrial dysfunction is associated with pathogenic features of DS and mitochondrial abnormalities have been found in all DS cells analyzed in culture to date, including neurons, astrocytes, and lymphocytes [1]. Indeed, in DS human fetal fibroblasts, PGC-1α, a master regulator of mitochondrial biogenesis, is down regulated at both the mRNA and protein levels and AMPK phosphorylation of the PGC-1α protein is essential for PGC-1α-dependent induction of the PGC-1α promoter [1].
In the Human Molecular Genetics study, Izzo et al. initially observed that DS human fetal fibroblasts (DS-HFFs) displayed a 40%-50% reduction in PGC-1α mRNA and protein levels compared to non-trisomic counterparts (N-HFFs). DS-HFFs treated with metformin however led to a significant increase in PGC-1α at both the mRNA and protein levels compared to untreated control cells, an increase in the mRNA levels of NRF-1 and TFAM (PGC-1α target genes critical in promoting mitochondrial biogenesis), and an increase in mtDNA content [1]. Additionally, compared to N-HFFs, DS-HFFs were characterized by a decrease in basal oxygen consumption rate (OCR, ~55% inhibition), a decrease in OCR related to ATP production (~58% inhibition), and a reduction in maximal respiratory capacity (~58% inhibition). DS-HFFs treated with metformin increased basal OCR, OCR related to ATP production, ATP concentration, mitochondrial membrane potential, and maximal respiratory capacity, thus restoring mitochondrial respiration in DS-HFFs [1].
Furthermore, compared to N-HFFs, DS-HFFs exhibited extensive mitochondrial damage, as evidenced by swollen cristae (~60%-90% of DS-HFFs vs. ~15% of N-HFFs), mitochondria with intra-oedema (~40% of DS-HFFs vs. ~6% of N-HFFs), and an increase in the number of damaged mitochondria (~90% in DS-HFFs vs. ~25% in N-HFFs). Metformin-treated DS-HFFs, compared to untreated cells, displayed narrower cristae with widths that were comparable to N-HFFs cells, fewer damaged mitochondria (~40%), and fewer mitochondria with intra-oedema (~5%) [1]. Interestingly, as mitochondrial fusion also plays an important role in mitochondrial functionality, the mRNA and protein expression of OPA1 and MFN2 (genes that regulate mitochondrial fusion) were significantly down-regulated in DS-HFFs vs. N-HFFs and correlated with extensive mitochondrial fragmentation in DS-HFFs. Treatment of DS-HFFs with metformin significantly increased the mRNA and protein levels of OPA1 and MFN2 and also reduced fragmentation of the mitochondrial network, indicating that metformin induces correction of mitochondrial phenotype by also restoring mitochondrial fusion machinery [1].
As noted above, the AMPK activator EGCG reverses cognitive defects in DS patients, promotes oxidative phosphorylation and mitochondrial biogenesis in lymphoblasts and fibroblasts from DS patients (i.e. increased levels and activity of PGC-1α, NRF-1 and TFAM), and restores oxidative phosphorylation, mitochondrial biogenesis, and improves hippocampal neural progenitor cell proliferation in a DS mouse model (Ts65Dn) in combination with the polyphenol resveratrol (i.e. increase in PGC-1α) in an AMPK-dependent manner [5,6,15]. Mitochondrial dysfunction is also a prominent feature associated with the accelerated aging disorder Hutchison-Gilford progeria syndrome (HGPS) and metformin has recently been shown to alleviate accelerated aging defects, activate AMPK, and beneficially alter gene splicing in HGPS patient cells, as I first hypothesized and published in 2014 and 2015 [12,13].
Egesipe et al. initially demonstrated, using mesenchymal stem cells (MSCs) derived from HGPS induced pluripotent stem cells (i.e. HGPS MSCs), a significant dose-dependent decrease in SRSF1 mRNA levels after metformin treatment and up to a 40% decrease in SRSF1 protein levels after treatment with 5 mmol/l of metformin (SRSF1 is a gene splicing factor that is upregulated in HGPS cells and causes faulty gene splicing of the LMNA gene leading to increased production of the toxic protein progerin) [2]. A significant decrease was also observed in both lamin A and progerin mRNA expression in HGPS MSCs treated with 5 mmol/l metformin, with progerin mRNA expression and protein levels reduced to levels lower than that of lamin A mRNA expression and protein levels, indicating that metformin-induced inhibition of SRSF1 led to an increase in the lamin A/progerin ratio and thus beneficially altered gene splicing [2].
The results obtained using HGPS MSCs were also replicated in additional in vitro cell models, with 5 mmol/l of metformin decreasing progerin mRNA expression up to 50% in LmnaG609G/G609G mouse primary fibroblasts (HGPS mouse model) and decreasing both lamin A and progerin mRNA expression in primary HGPS fibroblasts [2]. Interestingly, 5 mmol/l of metformin also decreased progerin mRNA expression in wild-type/normal MSCs that had been incubated with a compound that induces progerin expression, indicating that metformin may also prove beneficial in reducing progerin levels in normal humans [2].
Most importantly, however, treatment of HGPS MSCs with 5 mmol/l of metformin reduced the percentage of abnormal nuclei from 60% pre-treatment to less than 40% after treatment (wild-type MSCs presented less than 20% of abnormal nuclei). The metformin-induced reduction in abnormal nuclei was comparable to the reference treatment tipifarnib (1 μmol/1), a farnesyl-transferase inhibitor [2]. Additionally, as HGPS MSCs are characterized by premature osteogenic differentiation (indicated by increased alkaline phosphatase activity compared to wild-type osteogenic progenitor cells), 5 mmol/l of metformin led to a significant rescue of alkaline phosphatase activity in HGPS osteogenic progenitor cells, comparable to levels found in tipifarnib-treated cells [2].
Park et al. also characterized the cellular phenotypes of primary dermal fibroblasts derived from HGPS patients of different ages and showed increased staining of senescence-associated beta-galactosidase (SA-β-gal, an indicator of cellular senescence) and increased levels of mitochondrial superoxide in HG8 cells (from an 8 year old patient) compared to HG3 (3 year old) and HG5 (5 year old) cells [3]. All cells expressed the toxic protein progerin, superoxide dismutase 2 (SOD2, a mitochondrial antioxidant enzyme) was highest in normal fibroblasts and lowest in HG8 cells, and cellular proliferation rate slowed at an earlier time in HGPS cells compared to normal fibroblasts [3].
Utilizing HG8 cells (which demonstrated the highest levels of senescence), the authors also elucidated the effects of metformin (2mM), rapamycin (200nM), or a combination of both drugs on the nuclear phenotype of HGPS cells. Rapamycin significantly decreased the number of nuclei with abnormal morphology and metformin treatment also led to a significant increase in the number of cells with normal nuclei compared to control-treated cells [3]. Metformin also reduced senescence in HGPS cells (i.e. reduction in SA-β-gal staining) and co-treatment with rapamycin and metformin led to an approximately 34.2 % inhibition of senescence, with similar results observed in HG3 and HG8 cells [3]. Metformin, rapamycin, or co-treatment with both compounds led to a significant reduction in the number of cells containing more than 20 γ-H2AX foci (a marker of DNA damage) in HG8, HG3, and HG5 cells, indicating that metformin increases the efficiency of DNA repair in HGPS cells [3].
Metformin also exerted antioxidant effects in HGPS cells, as evidenced by a significant decrease in ROS production and mitochondrial superoxide formation compared to control cells as well as an upregulation of SOD2 mRNA expression in aged BALB/c mice (>18 months old) [3]. Most importantly, metformin treatment at 2 and 20mM reduced progerin protein expression by approximately 20 % and 60 %, respectively, compared to mock-treated cells and increased the presence of normal nuclear phenotypes in HGPS cells [3]. Metformin treatment also significantly increased the phosphorylation and activation of AMPK in HGPS cells. Furthermore, western blot analysis indicated that rapamycin increased AMPK activation as well.
As noted previously, metformin-induced AMPK activation also exerts potent antiviral effects. With respect to dengue virus replication, Soto-Acosta et al. first showed that AMPK activation was reduced in DENV-infected Huh7 (human liver) cells (serotype 2 and 4, 2/4) at 12 and 24 hpi compared to mock-treated cells [4]. Importantly, metformin enhanced AMPK activation in DENV 2/4-infected cells compared to mock/vehicle-treated cells, reduced NS3 levels compared to vehicle-treated cells, and reduced the levels of the viral structural proteins E and prM. DENV 2/4 infection also increased the activity of HMGCR (a rate-controlling enzyme of the cholesterol biosynthetic pathway) in vehicle-treated cells compared to mock-infected cells, whereas metformin disturbed the co-localization between HMGCR and NS4A or NS3, disrupted replicative complex integrity, and decreased the levels of the viral proteins NS4A and NS3. Furthermore, metformin also led to a reduction in the levels of DENV dsRNA, indicating that metformin exerted a strong antiviral effect against DENV [4].
Indeed, 24 hour treatment of DENV2/4-infected Huh7 cells with metformin led to a reduction in the amount of infected cells, decreased the viral yield up to one logarithm, and reduced NS1 secretion up to 90%. Metformin also led to a dose-dependent reduction in viral genome copies (up to 0.7 logarithm for DENV2 and 1.5 logarithm for DENV4) compared to non-treated cells [4]. Additionally, treatment of DENV-infected cells with the AMPK inhibitor compound C (CC) increased viral infection compared to non-treated cells and CC-induced AMPK inhibition increased viral genome copies up to a half logarithm in DENV 2- and up to 0.7 logarithm in DENV 4-infected cells, indicating that metformin’s potent antiviral effects against DENV infection and replication is dependent on AMPK activation [4].
In conclusion, data from recent evidence strongly suggests a compelling and novel assertion that the activation of AMPK via the induction of cellular stress (e.g. intracellular calcium increase, increased reactive oxygen species [ROS] production, and/or AMP/ATP ratio increase, etc.) represents a central node linking the therapeutic effects of structurally diverse compounds (e.g. metformin) with physiological and patho-physiological states, including Down syndrome, HGPS, HIV-1 latency and replication, dengue virus replication, oocyte activation, and sperm acrosome reaction induction. Indeed, AMPK activation is critical for oocyte meiotic resumption and maturation and AMPK has recently been found localized in human spermatozoa across the entire acrosome, indicating that induction of the acrosome reaction likely involves AMPK activation. Because oocyte activation is indispensable for the creation of all human life and AMPK activators including the calcium ionophores ionomycin and A23187 are extensively used to activate human oocytes, creating normal healthy children, AMPK likely connects the amelioration of normal and pathological aging defects with potential viral eradication, Down syndrome, and the creation of all human life.
https://www.linkedin.com/pulse/metformin-shown-first-time-restore-mitochondria-human-finley

References:
Izzo A, Nitti M, Mollo N, et al. Metformin restores the mitochondrial network and reverses mitochondrial dysfunction in Down syndrome cells. Hum Mol Genet. 2017 Jan 13. pii: ddx016. doi: 10.1093/hmg/ddx016. [Epub ahead of print].
Egesipe, Blondel, Cicero, et al. Metformin decreases progerin expression and alleviates pathological defects of Hutchinson–Gilford progeria syndrome cells. npj Aging and Mechanisms of Disease 2, Article number: 16026 (2016); http://www.nature.com/articles/npjamd201626?WT.feed_name=subjects_drug-discovery
Park SK, Shin OS. Metformin Alleviates Aging Cellular Phenotypes in Hutchinson-Gilford Progeria Syndrome Dermal Fibroblasts. Exp Dermatol. 2017 Feb 13. doi: 10.1111/exd.13323. [Epub ahead of print].
Soto-Acosta R, Bautista-Carbajal P, Cervantes-Salazar M, Angel-Ambrocio AH, Del Angel RM. DENV up-regulates the HMG-CoA reductase activity through the impairment of AMPK phosphorylation: A potential antiviral target. PLoS Pathog. 2017 Apr 6;13(4):e1006257.
De la Torre R, De Sola S, Pons M, et al. Epigallocatechin-3-gallate, a DYRK1A inhibitor, rescues cognitive deficits in Down syndrome mouse models and in humans. Mol Nutr Food Res. 2014 Feb;58(2):278-88.
Valenti D, de Bari L, de Rasmo D, et al. The polyphenols resveratrol and epigallocatechin-3-gallate restore the severe impairment of mitochondria in hippocampal progenitor cells from a Down syndrome mouse model. Biochim Biophys Acta. 2016 Jun;1862(6):1093-104.
Nunes RD, Ventura-Martins G, Moretti DM, et al. Polyphenol-Rich Diets Exacerbate AMPK-Mediated Autophagy, Decreasing Proliferation of Mosquito Midgut Microbiota, and Extending Vector Lifespan. PLoS Negl Trop Dis. 2016 Oct 12;10(10):e0005034.
Zainal N, Chang CP, Cheng YL, et al. Resveratrol treatment reveals a novel role for HMGB1 in regulation of the type 1 interferon response in dengue virus infection. Sci Rep. 2017 Feb 20;7:42998.
Heredia A, Davis C, Amin MN, et al. Targeting host nucleotide biosynthesis with resveratrol inhibits emtricitabine-resistant HIV-1. AIDS. 2014 Jan 28;28(3):317-23.
Pan XY, Zhao W, Zeng XY, et al. Heat Shock Factor 1 Mediates Latent HIV Reactivation. Sci Rep. 2016 May 18;6:26294.
Zhang HS, Wu TC, Sang WW, Ruan Z. EGCG inhibits Tat-induced LTR transactivation: role of Nrf2, AKT, AMPK signaling pathway. Life Sci. 2012 May 22;90(19-20):747-54.
Finley J. Alteration of splice site selection in the LMNA gene and inhibition of progerin production via AMPK activation. Med Hypotheses. 2014 Nov;83(5):580-7.
Finley J. Reactivation of latently infected HIV-1 viral reservoirs and correction of aberrant alternative splicing in the LMNA gene via AMPK activation: Common mechanism of action linking HIV-1 latency and Hutchinson-Gilford progeria syndrome. Med Hypotheses. 2015 Sep;85(3):320-32.
Finley J. Oocyte activation and latent HIV-1 reactivation: AMPK as a common mechanism of action linking the beginnings of life and the potential eradication of HIV-1. Med Hypotheses. 2016 Aug;93:34-47.
Valenti D, De Rasmo D, Signorile A, et al. Epigallocatechin-3-gallate prevents oxidative phosphorylation deficit and promotes mitochondrial biogenesis in human cells from subjects with Down's syndrome. Biochim Biophys Acta. 2013 Apr;1832(4):542-52.
0 notes
Text
Metformin shown for the first time to inhibit Dengue virus in human liver cells via AMPK: AMPK links Viral Eradication with Oocytes, Sperm, & Progeria

A study recently published in the journal PLoS Pathogens in April of 2017 provided startling evidence demonstrating for the first time that the commonly-prescribed anti-diabetic drug metformin exerted significant antiviral effects in dengue virus-infected human liver cells that was dependent on activation of the master metabolic regulator AMPK [59]. The authors showed that an in increase HMG-CoA reductase (HMGCR) activity, a target of AMPK, was associated with dengue virus (DENV)-infected cells, AMPK activation was reduced in DENV-infected cells at 12 and 24 hours post infection (hpi), and metformin significantly decreased the number of infected cells, viral yield, and viral genome copies, leading the authors to conclude that metformin-induced AMPK activation generates a strong antiviral effect against DENV [59]. Interestingly, as discussed below, recent efforts funded by the U.S. and British governments, the Bill & Melinda Gates Foundation, and the Google health spin-off Verily have sought to decrease the spread of dengue and Zika viruses through the coordinated release of female and/or male mosquitoes (called Aedes aegypti) that were purposely infected with a bacterium that inhibits the mosquito’s ability to transmit the two viruses to humans [1]. Studies have shown that this bacterium, called Wolbachia, enhances the mosquito’s immune response by increasing the levels of reactive oxygen species (ROS), thus enhancing inhibition of dengue virus replication [30]. Because AMPK is activated by cellular stress (e.g. ROS increase, intracellular calcium [Ca2+] increase, AMP/ATP ratio increase, etc.), has been found in Aedes aegypti (Ae. aegypti), and AMPK activation by stress-inducing compounds (e.g. resveratrol) increased average life span and enhanced the immune response in Ae. aegypti in an AMPK-dependent manner, the recent finding that metformin also inhibits DENV replication in human cells in an AMPK-dependent manner provides compelling evidence that the anti-viral effects of AMPK activation likely crosses species boundaries [31]. Additionally, metformin has recently been shown to beneficially alter gene splicing, activate AMPK, and ameliorate accelerated aging defects in cells derived from Hutchison-Gilford progeria syndrome patients (HGPS), as I first hypothesized and published in 2014 [60-62]. AMPK activation also promotes oocyte meiotic induction and maturation (in preparation for oocyte activation) and AMPK has recently been found localized across the entire acrosome in human spermatozoa [53,55]. The induction of cellular stress (e.g. ROS, intracellular Ca2+, and/or AMP/ATP ratio increase) also promotes oocyte meiotic induction/maturation, oocyte activation, and the acrosome reaction in human sperm, processes critical for the creation of all human life [53,56,63]. As further discussed below, such interconnectedness implicates AMPK as a central mediator in the promotion of lifespan and healthspan, amelioration of pathological aging, the mounting of an effective immune response, and the creation of all human life. Transmission of DENV by mosquito vectors including Ae. aegypti may lead to febrile illness (known as dengue fever) in humans characterized by fluid retention, respiratory distress, and/or organ impairment [59]. There are four DENV serotypes (DENV 1-4) and several structural (capsid (C), membrane (M), and envelope (E)) and nonstructural proteins (NS1, NS2A, NS2B, NS3, NS4A, NS4B, and NS5) are encoded by the DENV genome. Interestingly, lipids and the formation of replication complexes (i.e. membranous compartments) have been shown to play important roles in the DENV replication cycle whereas the viral protein NS4A is directly associated with DENV-associated membrane rearrangement [59]. The authors first showed that AMPK activation was reduced in DENV-infected Huh7 cells (serotype 2 and 4, 2/4) at 12 and 24 hpi compared to mock-treated cells. Importantly, metformin enhanced AMPK activation in DENV 2/4-infected cells compared to mock/vehicle-treated cells, reduced NS3 levels compared to vehicle-treated cells, and reduced the levels of the viral structural proteins E and prM. DENV 2/4 infection also increased the activity of HMGCR (a rate-controlling enzyme of the cholesterol biosynthetic pathway) in vehicle-treated cells compared to mock-infected cells, whereas metformin disturbed the co-localization between HMGCR and NS4A or NS3, disrupted replicative complex integrity, and decreased the levels of the viral proteins NS4A and NS3. Furthermore, metformin also led to a reduction in the levels of DENV dsRNA, indicating that metformin exerted a strong antiviral effect against DENV [59]. Indeed, 24 hour treatment of DENV2/4-infected Huh7 cells with metformin led to a reduction in the amount of infected cells, decreased the viral yield up to one logarithm, and reduced NS1 secretion up to 90%. Metformin also led to a dose-dependent reduction in viral genome copies (up to 0.7 logarithm for DENV2 and 1.5 logarithm for DENV4) compared to non-treated cells [59]. Additionally, treatment of DENV-infected cells with the AMPK inhibitor compound C (CC) increased viral infection compared to non-treated cells and CC-induced AMPK inhibition increased viral genome copies up to a half logarithm in DENV 2- and up to 0.7 logarithm in DENV 4-infected cells, indicating that metformin’s potent antiviral effects against DENV infection and replication is dependent on AMPK activation [59]. Metformin’s AMPK-dependent antiviral effects against DENV in human liver cells likely represent a common mechanism that crosses species boundaries to effectuate viral eradication. As noted above, current efforts by organizations including the Bill and Melinda Gates foundation and the Google health spin-off Verily has focused on reducing transmission of dengue and Zika viruses by infecting the mosquito Ae. aegypti with the bacterium Wolbachia, leading to inhibition of viral replication. As explained below, inhibition of DENV replication in both mosquitoes and in human cells is likely orchestrated by stress-induced AMPK activation. Although the method through which Wolbachia is transmitted to mosquito offspring has been well studied, how Wolbachia infection leads to a decrease in dengue and Zika virus replication in, and transmission by, mosquitoes is heavily debated. Interestingly, although Wolbachia does not naturally occur in Ae. aegypti, Wolbachia appears to impart beneficial immunomodulatory effects in these mosquitoes not unlike that of certain beneficial bacteria that normally colonize the human gut. Such bacteria, also known as “probiotics”, are typified by the genera Lactobacillus and Bifidobacterium and have been shown to significantly enhance natural and acquired immunity, induce reactive oxygen species (ROS) generation and the upregulation of Nrf2-dependent cytoprotective genes, produce short chain fatty acids (SCFAs) that promote T cell differentiation, and produce antimicrobial peptides that exhibit bactericidal activity [4-7]. Interestingly, the induction of cellular stress via ROS generation has been shown to activate the master metabolic regulator AMPK and AMPK activation has been shown to be critical for T cell activation and the mounting of an effective immune response in vivo to viral and bacterial infections [8-10]. Indeed, acetate, propionate, and butyrate, three SCFAs abundantly produced by probiotic bacteria, each activate AMPK, indicating that the immunomodulatory effects of probiotic bacteria are dependant on cellular stress-induced activation of AMPK [11]. Furthermore, AMPK has recently been discovered in Ae. aegypti and activation of AMPK in Ae. aegypti by compounds that induce cellular stress leads to an enhanced immune response and increased longevity in Ae. aegypti (see below), implicating the provocative assertion that inhibition of dengue and Zika virus replication via Wolbachia infection occurs as a result of cellular stress-induced activation of AMPK [12]. Wolbachia, which are found in reproductive tissues of arthropods, often engage in a mutualistic relationship with its host. Indeed, Wolbachia has been shown to induce resistance to RNA viral infections in the model organism Drosophila melanogaster [13]. D. melanogaster (also known as the fruit fly) has been studied extensively in biological research and activation of AMPK has been shown to extend lifespan as well as slow aging in D. melanogaster through autophagy induction via upregulation of Atg1 (ULK1 in mammals), reduction of insulin-like peptide levels in the brain, and an increase in 4E-BP [14,15]. Interestingly, insulin-like peptides and hyperphosphorylation of 4E-BP (i.e. inactivation) is positively associated with target of rapamycin (TOR) activation and egg development in Ae. aegypti [16]. Mammalian target of rapamycin (mTORC1/TORC1) is a protein kinase that plays a critical role in promoting mRNA translation and is the primary target of rapamycin, a macrolide drug that extends lifespan in several model organisms including normal genetically heterogeneous mice and D. melanogaster [17,18]. Rapamycin was shown to delay yolk deposition in control Ae. aegypti eggs and inhibit insulin-mediated 4E-BP (a negative regulator of translation) phosphorylation. Silencing of 4E-BP also led to a reduction in lifespan of adult female mosquitoes, mirroring results obtained via AMPK activation in D. melanogaster [15,16,19]. Strikingly, in addition to delaying egg maturation in Ae. aegypti, rapamycin has also been shown to dramatically increase the number of Wolbachia in D. melanogaster oocytes via TORC1 inhibition, whereas somatic hyperactivation of TORC1 decreases Wolbachia titer in oocytes [20]. Interestingly, ablation of insulin-producing cells in the D. melanogaster brain abolished the yeast-induced reduction of Wolbachia in oocytes, mirroring previous results showing that AMPK-induced lifespan extension in D. melanogaster is associated with a decrease in insulin-like peptides in the brain [15,20]. Because rapamycin, similar to AMPK activation by diverse compounds, induces autophagy, inhibits mTOR/TORC1, promotes mitochondrial biogenesis, and extends lifespan in several model organisms, it would expected that rapamycin would also induce activation of AMPK, likely via the induction of cellular stress (i.e. increase in the levels of ROS, intracellular Ca2+ increase, increase in the AMP:ADP/ATP ratio, etc). Indeed, two recent publications clearly demonstrated that rapamycin potently induced the activation of AMPK in vivo in normal elderly mice, along with an upregulation of ULK1 (mammalian orthologue of Atg1 critical for the induction of autophagy) and PGC-1a (a transcription factor essential for promoting mitochondrial biogenesis) [21,22]. As rapamycin has been shown to increase the number of Wolbachia in D. melanogaster oocytes, delay egg maturation and inhibit insulin-mediated 4E-BP phosphorylation in Ae. aegypti, and increase lifespan in D. melanogaster, the recent finding that activation of AMPK in Ae. aegypti also increases lifespan and improves the immune response (see below) further bolsters the notion that activation of AMPK via the induction of cellular stress likely promotes lifespan extension, immune system enhancement, and Wolbachia propagation in oocytes in both D. melanogaster and Ae. aegypti. Moreover, because certain bacteria (e.g. Lactobacillus) that colonize the human gut induces beneficial immune responses by inducing cellular stress (e.g. increased ROS levels) and also produce compounds that activate AMPK, the inhibition of dengue and Zika virus replication in, and transmission by, Ae. aegypti is likely the result of a Wolbachia-induced cellular stress response, leading to the up regulation of anti-viral mechanisms that are likely modulated by AMPK. Indeed, a recent study by Wong et al. demonstrated that ROS/oxidative stress is positively correlated with Wolbachia-mediated antiviral protection in D. melanogaster [23]. The authors of the study observed that H2O2 (hydrogen peroxide) was increased 1.25- to 2- fold in flies that harbored protective strains of Wolbachia (e.g. wMelCS, wRi, wAu) compared to Wolbachia-free controls. Interestingly, flies with a null mutation in Cu/Zn SOD (an antioxidant enzyme) exhibited elevated endogenous levels of oxidative stress that mimicked Wolbachia-induced oxidative stress. In these Wolbachia-free Cu/Zn SOD mutant flies, a 70% survival rate was observed at 4 days post-infection after Drosophila C virus infection compared to a less than 10% survival rate for non-mutant flies, indicating that elevated levels of oxidative stress induced by protective Wolbachia strains confers a survival advantage by decreasing susceptibility to viral infection via activation of signaling pathways that potentiate anti-viral immune responses [23]. As noted above, ROS/oxidative stress has been shown to activate AMPK and AMPK activation in both D. melanogaster and Ae. Aegypti leads to an increase in lifespan [8,12,15]. Interestingly, Sykiotis et al. showed that oxidants/oxidative stress promote lifespan extension in D. melanogaster males by activating the Nrf2 (CncC) pathway, a master antioxidant transcription factor that induces the expression of several antioxidant genes including NAD(P)H quinone oxidoreductase 1 (NQO1) and heme oxygenase-1 (HO-1) [24]. Curiously, Sykiotis et al. also observed that the synthetic dithiolthione oltipraz, which has been shown to activate AMPK, inhibit HIV-1 replication, and reverse accelerating aging defects in Hutchinson-Gilford progeria syndrome (see below), also induced Nrf2 signaling in flies [24-27]. AMPK has also been shown to increase the transcriptional activity of Nrf2 and increase Nrf2 nuclear retention via phosphorylation, suggesting that AMPK may represent a central node in ROS/oxidative stress-induced immune and anti-viral responses [28,29]. A recent study by Pan et al. showed that infection of Ae. Aegypti with Wolbachia (wAlbB strain) led to a ROS/oxidative stress-induced upregulation of genes associated with immunity and reduction-oxidation, thus enhancing inhibition of dengue virus replication [30]. Several gene transcripts related to the immunity and redox/stress/mitochondrion group were upregulated in Wolbachia-infected Ae. aegypti females before blood feeding and after infection with DENV serotype 2, including the antimicrobial peptides cecropin D (CECD) and defensin C (DEFC) as well as the antioxidants glutathione peroxidase, CuZnSOD, MnSOD, and glutathione peroxidase [30]. A significant increase in the levels of H2O2 and in the transcript abundance of both NADPH oxidase M (NOXM) and dual oxidase 2 (DUOX2), two enzymes that generate ROS, were also observed in Wolbachia-infected mosquitoes compared to control mosquitoes. Interestingly, the expression of several Toll pathway (a pathway that mediates the production of antioxidants and antimicrobial peptides) marker genes as well as the antimicrobial peptides CECD and DEFC were increased by the addition of H2O2 in a sugar solution given to female control/uninfected mosquitoes [30]. This effect was also mirrored in Wolbachia-infected female mosquitoes, wherein silencing of NOXM and DUOX2 deactivated the Toll pathway and suppressed the expression of CECD and DEFC, indicating that Wolbachia-induced ROS is required for activation of the Toll pathway and induction of antimicrobial peptides. Importantly, individual or double RNAi-induced knockdown of DEFC and CECD significantly increased viral titers of DENV serotype 2 in Wolbachia-infected mosquitoes compared to controls, providing further evidence that Wolbachia-induced ROS leads to a beneficial cellular response characterized by antimicrobial-mediated inhibition of viral replication [30]. Because Wolbachia-induced ROS/oxidative stress leads to beneficial anti-viral cellular responses in both D. melanogaster and Ae. aegypti and because AMPK activation, which increases Nrf2 transcriptional activity, leads to increased lifespan in D. melanogaster, it would be expected that cellular stress-induced AMPK activation in Ae. aegypti would also lead to an increase in lifespan as well as an enhanced immune response. Indeed, Nunes et al. showed that autophagy, immune system activation, and the average lifespan of Ae. Aegypti (a vector for dengue as well as chikungunya and Zika virus) is increased by feeding mosquitoes several plant-based polyphenols [31]. Interestingly, administration of resveratrol (derived from grapes), quercetin (found in many fruits and vegetables), epigallocathechin-3-gallate (EGCG, found in green tea), and genistein (found in soybeans) each increased average lifespan for male and female mosquitoes compared to controls. Insects fed resveratrol also displayed higher levels of AMPK phosphorylation/activation compared to controls and compound C, a pharmacological inhibitor of AMPK, blocked the suppression of triglyceride (TG) content induced by resveratrol [31]. Resveratrol treatment also led to immunomodulatory effects, with reduced bacterial populations for female mosquitoes compared to controls, an effect that was mimicked by AICAR (a prototypical AMPK activator) but inhibited by compound C. Interestingly, resveratrol only slightly decreased bacterial populations in vitro, indicating that the effects of resveratrol are indirect and likely associated with activation of the immune response in mosquitoes. Autophagy was also stimulated in resveratrol- and AICAR-fed mosquitoes which was abolished by silencing of AMPK [31]. Interestingly, each of the polyphenols used in that study (resveratrol, quercetin, epigallocathechin-3-gallate, and genistein) have each been shown to induce cellular stress (e.g. ROS generation), similar to Wolbachia-induced ROS generation, in a number of mammalian cells in addition to activating AMPK [32-35]. As AMPK is activated by a number of different compounds (polyphenols, bacterial metabolites, metformin etc.) and methodologies (e.g. electrical stimulation) that induce cellular stress, it is likely that Wolbachia-induced inhibition of dengue and Zika virus replication in and transmission by Ae. Aegypti is also modulated by AMPK, a master metabolic regulator that increases lifespan and improves immune and antioxidant responses in another Wolbachia-infected insect, D. melanogaster [36,37]. Although Nunes et al. did not determine if the polyphenols tested inhibited dengue virus replication in or transmission by Ae. Aegypti, recent studies have shown that in mammalian cells quercetin and analogs of resveratrol exhibit significant inhibitory activities against dengue virus type 2 [38,39]. EGCG has also recently been shown to inhibit Zika virus entry in Vero E6 cells (albeit at higher concentrations), indicating that cellular stress-induced activation of AMPK may represent a common mechanism of action that spans species boundaries to prevent the replication and/or transmission of dengue and Zika viruses [40]. Additionally, salidroside (derived from the plant Rhodiola rosea) and curcumin (derived from the plant Curcuma longa) have both been shown to exhibit anti-dengue virus activity in vitro and induce AMPK activation in vivo, providing further evidence that many structurally diverse compounds likely share a common mechanism of stress-induced AMPK activation to effectuate antiviral responses [41-44]. Remarkably, cellular stress-induced activation of AMPK (i.e. “Shock”) appears to link the antiviral effects mediated by a Wolbachia-induced increase in ROS production in A. aegypti with the amelioration of accelerated cellular aging defects in the genetic disorder Hutchinson-Gilford progeria syndrome (HGPS), the reactivation of latent HIV-1 in infected T cells (to facilitate immune system detection and virus destruction), oocyte meiotic resumption, and the acrosome reaction in sperm (i.e. “Live”). Indeed, the proteasome inhibitor MG132 has recently been shown to inhibit progerin (the toxic protein that causes accelerated cellular aging defects) in fibroblasts derived from HGPS patients, inhibit dengue, West Nile, and Yellow fever viruses, and activate AMPK [45-47]. 1α,25-dihydroxyvitamin D3, the most potent metabolite of vitamin D, has also been shown to delay premature senescence and significantly improve accelerated aging in patient-derived HGPS cells, reduce dengue virus infection in human myelomonocyte and hepatic cell lines, and activate AMPK [48-50]. Also, as discussed above, metformin has recently been shown to beneficially alter gene splicing, activate AMPK, and ameliorate accelerated aging defects in cells derived from Hutchison-Gilford progeria syndrome patients (HGPS) and significantly inhibit dengue virus infection and replication in human liver cells [59,60-62]. Interestingly, nuclear aging defects in HGPS patient-derived cells were also reversed via activation of the antioxidant transcription factor Nrf2 by oltipraz. Oltipraz has also been shown to activate AMPK, inhibit HIV-1 replication, and induce Nrf2 signaling in D. melanogaster [24-27]. Furthermore, just as Wolbachia-induced ROS/hydrogen peroxide (H2O2) generation (i.e. “Shock”) leads to a compensatory upregulation of antimicrobial peptides and an enhanced antiviral immune response in Ae. Aegypti (i.e. “Live”), the exposure of HIV-1 latently infected monocyte or lymphocyte cell lines to H2O2 has also been shown to reactivate HIV-1 [51]. Additionally, ROS induces activation of AMPK and AMPK plays a critical role in T cell activation (and thus reactivation of latent HIV-1 that resides in T cell cells) [8,52,64]. AMPK activation has also been shown to play a critical role in the initiation of oocyte meiotic resumption and maturation (in preparation for oocyte activation) and the free radical-generating agent menadione (i.e. “Shock”) has been shown to induce AMPK-dependent meiotic resumption in cumulus-enclosed and denuded mouse oocytes (i.e. “Live”) via promotion of oxidative stress [53]. Lastly, ROS also plays a critical role in the induction of the acrosome reaction in sperm, a process that facilitates oocyte penetration through release of hydrolytic enzymes and is indispensable, along with oocyte activation, for the creation of all human life outside of a clinical setting [54]. Strikingly, AMPK has recently been found for the first time to be localized across the entire acrosome in human sperm and both H2O2/ROS and vitamin D (i.e. “Shock) have been shown to induce the acrosome reaction in human sperm (i.e. “Live”), providing compelling evidence that cellular stress-induced AMPK activation is critical for the induction of the acrosome reaction in human sperm and the creation of human life [55-57]. The antiviral effects mediated by Wolbachia-induced ROS generation paints a clear yet provocative portrait that cellular-stress mediated activation of AMPK represents a common mechanism of action that spans species boundaries, beneficially modulating cellular processes that are involved in the immune response, fertilization, and aging itself. Indeed, structurally distinct compounds including metformin, MG132, and vitamin D have been shown to exert potent antiviral effects against dengue virus and significantly improve accelerated aging defects in HGPS. More tellingly, H2O2/ROS/oxidative stress has been shown to reactivate latent HIV-1, promote oocyte meiotic resumption, and induce the acrosome reaction in human sperm. Because AMPK is critical for oocyte meiotic resumption (and hence oocyte activation) and AMPK has recently been found for the first time to be localized across the entire acrosome in human sperm, AMPK activation may indeed be critical for the creation of all human life, as originally proposed in my recent publication [58]. Perhaps beyond perplexing on first glance, Wolbachia, mosquitoes, and the creation of all human life are likely connected. https://www.linkedin.com/pulse/metformin-shown-first-time-inhibit-dengue-virus-human-finley

References
http://www.reuters.com/article/us-health-dengue-mosquitoes-idUSKCN12Q1PE
https://www.bloomberg.com/news/articles/2016-10-06/alphabet-s-verily-joins-zika-fight-with-sterile-mosquito-lab
Werren JH. Biology of Wolbachia. Annu Rev Entomol. 1997;42:587-609.
Castillo NA, Perdigón G, de Moreno de Leblanc A. Oral administration of a probiotic Lactobacillus modulates cytokine production and TLR expression improving the immune response against Salmonella enterica serovar Typhimurium infection in mice. BMC Microbiol. 2011 Aug 3;11:177.
Gill HS, Rutherfurd KJ, Prasad J, Gopal PK. Enhancement of natural and acquired immunity by Lactobacillus rhamnosus (HN001), Lactobacillus acidophilus (HN017) and Bifidobacterium lactis (HN019). Br J Nutr. 2000 Feb;83(2):167-76.
Jones RM, Desai C, Darby TM, et al. Lactobacilli Modulate Epithelial Cytoprotection through the Nrf2 Pathway. Cell Rep. 2015 Aug 25;12(8):1217-25.
Park J, Kim M, Kang SG, et al. Short-chain fatty acids induce both effector and regulatory T cells by suppression of histone deacetylases and regulation of the mTOR-S6K pathway. Mucosal Immunol. 2015 Jan;8(1):80-93.
Mungai PT, Waypa GB, Jairaman A, et al. Hypoxia triggers AMPK activation through reactive oxygen species-mediated activation of calcium release-activated calcium channels. Mol Cell Biol. 2011 Sep;31(17):3531-45.
Tamás P, Hawley SA, Clarke RG, et al. Regulation of the energy sensor AMP-activated protein kinase by antigen receptor and Ca2+ in T lymphocytes. J Exp Med 2006;203(7):1665–70.
Blagih J, Coulombe F, Vincent EE, et al. The energy sensor AMPK regulates T cell metabolic adaptation and effector responses in vivo. Immunity. 2015 Jan 20;42(1):41-54.
Elamin EE, Masclee AA, Dekker J, Pieters HJ, Jonkers DM. Short-chain fatty acids activate AMP-activated protein kinase and ameliorate ethanol-induced intestinal barrier dysfunction in Caco-2 cell monolayers. J Nutr. 2013 Dec;143(12):1872-81.
Nunes RD, Ventura-Martins G, Moretti DM, et al. Polyphenol-Rich Diets Exacerbate AMPK-Mediated Autophagy, Decreasing Proliferation of Mosquito Midgut Microbiota, and Extending Vector Lifespan. PLoS Negl Trop Dis. 2016 Oct 12;10(10):e0005034.
Teixeira L, Ferreira A, Ashburner M. The bacterial symbiont Wolbachia induces resistance to RNA viral infections in Drosophila melanogaster. PLoS Biol. 2008 Dec 23;6(12):e2.
Yang S, Long LH, Li D, et al. β-Guanidinopropionic acid extends the lifespan of Drosophila melanogaster via an AMP-activated protein kinase-dependent increase in autophagy. Aging Cell. 2015 Dec;14(6):1024-33.
Ulgherait M, Rana A, Rera M, Graniel J, Walker DW. AMPK modulates tissue and organismal aging in a non-cell-autonomous manner. Cell Rep. 2014 Sep 25;8(6):1767-80.
Gulia-Nuss M, Robertson AE, Brown MR, Strand MR. Insulin-like peptides and the target of rapamycin pathway coordinately regulate blood digestion and egg maturation in the mosquito Aedes aegypti. PLoS One. 2011;6(5):e20401.
Harrison DE, Strong R, Sharp ZD, et al. Rapamycin fed late in life extends lifespan in genetically heterogeneous mice. Nature. 2009 Jul 16;460(7253):392-5.
Sun X, Wheeler CT, Yolitz J, et al. A mitochondrial ATP synthase subunit interacts with TOR signaling to modulate protein homeostasis and lifespan in Drosophila. Cell Rep. 2014 Sep 25;8(6):1781-92.
Roy SG, Raikhel AS. Nutritional and hormonal regulation of the TOR effector 4E-binding protein (4E-BP) in the mosquito Aedes aegypti. FASEB J. 2012 Mar;26(3):1334-42.
Serbus LR, White PM, Silva JP, et al. The impact of host diet on Wolbachia titer in Drosophila. PLoS Pathog. 2015 Mar 31;11(3):e1004777.
Chiao YA, Kolwicz SC, Basisty N, et al. Rapamycin transiently induces mitochondrial remodeling to reprogram energy metabolism in old hearts. Aging (Albany NY). 2016 Feb;8(2):314-27.
Lesniewski LA, Seals DR, Walker AE, et al. Dietary rapamycin supplementation reverses age-related vascular dysfunction and oxidative stress, while modulating nutrient-sensing, cell cycle, and senescence pathways. Aging Cell. 2016 Sep 22. doi: 10.1111/acel.12524. [Epub ahead of print].
Wong ZS, Brownlie JC, Johnson KN. Oxidative stress correlates with Wolbachia-mediated antiviral protection in Wolbachia-Drosophila associations. Appl Environ Microbiol. 2015 May 1;81(9):3001-5.
Sykiotis GP, Bohmann D. Keap1/Nrf2 signaling regulates oxidative stress tolerance and lifespan in Drosophila. Dev Cell. 2008 Jan;14(1):76-85.
Prochaska HJ, Fernandes CL, Pantoja RM, Chavan SJ. Inhibition of human immunodeficiency virus type 1 long terminal repeat-driven transcription by an in vivo metabolite of oltipraz: implications for antiretroviral therapy. Biochem Biophys Res Commun. 1996 Apr 25;221(3):548-53.
Kubben N, Zhang W2, Wang L, et al. Repression of the Antioxidant NRF2 Pathway in Premature Aging. Cell. 2016 Jun 2;165(6):1361-74.
Kim TH, Eom JS, Lee CG, Yang YM, Lee YS, Kim SG. An active metabolite of oltipraz (M2) increases mitochondrial fuel oxidation and inhibits lipogenesis in the liver by dually activating AMPK. Br J Pharmacol. 2013 Apr;168(7):1647-61. doi: 10.1111/bph.12057.
Mo C, Wang L, Zhang J, et al. The crosstalk between Nrf2 and AMPK signal pathways is important for the anti-inflammatory effect of berberine in LPS-stimulated macrophages and endotoxin-shocked mice. Antioxid Redox Signal. 2014 Feb 1;20(4):574-88.
Joo MS, Kim WD, Lee KY, Kim JH, Koo JH, Kim SG. AMPK Facilitates Nuclear Accumulation of Nrf2 by Phosphorylating at Serine 550. Mol Cell Biol. 2016 Jun 29;36(14):1931-42.
Pan X, Zhou G, Wu J, et al. Wolbachia induces reactive oxygen species (ROS)-dependent activation of the Toll pathway to control dengue virus in the mosquito Aedes aegypti. Proc Natl Acad Sci U S A. 2012 Jan 3;109(1):E23-31.
Nunes RD, Ventura-Martins G, Moretti DM, et al. Polyphenol-Rich Diets Exacerbate AMPK-Mediated Autophagy, Decreasing Proliferation of Mosquito Midgut Microbiota, and Extending Vector Lifespan. PLoS Negl Trop Dis. 2016 Oct 12;10(10):e0005034.
Li GX, Chen YK, Hou Z, et al. Pro-oxidative activities and dose-response relationship of (-)-epigallocatechin-3-gallate in the inhibition of lung cancer cell growth: a comparative study in vivo and in vitro. Carcinogenesis. 2010 May;31(5):902-10.
Chang YF, Chi CW, Wang JJ. Reactive oxygen species production is involved in quercetin-induced apoptosis in human hepatoma cells. Nutr Cancer. 2006;55(2):201-9.
Miki H, Uehara N, Kimura A, et al. Resveratrol induces apoptosis via ROS-triggered autophagy in human colon cancer cells. Int J Oncol. 2012 Apr;40(4):1020-8.
Ullah MF, Ahmad A, Zubair H, et al. Soy isoflavone genistein induces cell death in breast cancer cells through mobilization of endogenous copper ions and generation of reactive oxygen species. Mol Nutr Food Res. 2011 Apr;55(4):553-9.
Matsuyama S, Moriuchi M1, Suico MA, et al. Mild electrical stimulation increases stress resistance and suppresses fat accumulation via activation of LKB1-AMPK signaling pathway in C. elegans. PLoS One. 2014 Dec 9;9(12):e114690.
Dutra HL, Rocha MN, Dias FB, Mansur SB, Caragata EP, Moreira LA. Wolbachia Blocks Currently Circulating Zika Virus Isolates in Brazilian Aedes aegypti Mosquitoes. Cell Host Microbe. 2016 Jun 8;19(6):771-4.
Zandi K, Teoh BT, Sam SS, Wong PF, Mustafa MR, Abubakar S. Antiviral activity of four types of bioflavonoid against dengue virus type-2. Virol J. 2011 Dec 28;8:560.
Han YS, Penthala NR2, Oliveira M, et al. Identification of resveratrol analogs as potent anti-dengue agents using a cell-based assay. J Med Virol. 2016 Aug 10. doi: 10.1002/jmv.24660. [Epub ahead of print].
Carneiro BM, Batista MN, Braga AC, Nogueira ML, Rahal P. The green tea molecule EGCG inhibits Zika virus entry. Virology. 2016 Sep;496:215-8.
Padilla-S L, Rodríguez A, Gonzales MM, Gallego-G JC, Castaño-O JC. Inhibitory effects of curcumin on dengue virus type 2-infected cells in vitro. Arch Virol. 2014 Mar;159(3):573-9.
Sharma N, Mishra KP, Ganju L. Salidroside exhibits anti-dengue virus activity by upregulating host innate immune factors. Arch Virol. 2016 Dec;161(12):3331-3344.
Chang X, Zhang K, Zhou R, et al. Cardioprotective effects of salidroside on myocardial ischemia-reperfusion injury in coronary artery occlusion-induced rats and Langendorff-perfused rat hearts. Int J Cardiol. 2016 Jul 15;215:532-44.
Ejaz A, Wu D, Kwan P, Meydani M. Curcumin inhibits adipogenesis in 3T3-L1 adipocytes and angiogenesis and obesity in C57/BL mice.
Pellegrini C, Columbaro M, Capanni C, et al. All-trans retinoic acid and rapamycin normalize Hutchinson Gilford progeria fibroblast phenotype. Oncotarget. 2015 Oct 6;6(30):29914-28.
Fernandez-Garcia MD, Meertens L, Bonazzi M, Cossart P, Arenzana-Seisdedos F, Amara A. Appraising the roles of CBLL1 and the ubiquitin/proteasome system for flavivirus entry and replication. J Virol. 2011 Mar;85(6):2980-9.
Jiang S, Park DW, Gao Y, et al. Participation of proteasome-ubiquitin protein degradation in autophagy and the activation of AMP-activated protein kinase. Cell Signal. 2015 Jun;27(6):1186-97.
Kreienkamp R, Croke M, Neumann MA, et al. Vitamin D receptor signaling improves Hutchinson-Gilford progeria syndrome cellular phenotypes. Oncotarget. 2016 Apr 27. doi: 10.18632/oncotarget.9065.
Puerta-Guardo H, Medina F, De la Cruz Hernández SI, Rosales VH, Ludert JE, del Angel RM. The 1α,25-dihydroxy-vitamin D3 reduces dengue virus infection in human myelomonocyte (U937) and hepatic (Huh-7) cell lines and cytokine production in the infected monocytes. Antiviral Res. 2012 Apr;94(1):57-61.
Swami S, Krishnan AV, Williams J, et al.. Vitamin D mitigates the adverse effects of obesity on breast cancer in mice. Endocr Relat Cancer. 2016 Apr;23(4):251-64.
Piette J, Legrand-Poels S. HIV-1 reactivation after an oxidative stress mediated by different reactive oxygen species. Chem Biol Interact. 1994 Jun;91(2-3):79-89.
Tamás P, Hawley SA, Clarke RG, et al. Regulation of the energy sensor AMP-activated protein kinase by antigen receptor and Ca2+ in T lymphocytes. J Exp Med. 2006 Jul 10;203(7):1665-70.
LaRosa C, Downs SM. Stress stimulates AMP-activated protein kinase and meiotic resumption in mouse oocytes. Biol Reprod. 2006 Mar;74(3):585-92.
Patrat C, Serres C, Jouannet P. The acrosome reaction in human spermatozoa. Biol Cell. 2000 Jul;92(3-4):255-66.
Calle-Guisado V, de Llera AH, Martin-Hidalgo D, et al. AMP-activated kinase in human spermatozoa: identification, intracellular localization, and key function in the regulation of sperm motility. Asian J Androl. 2016 Sep 27. doi: 10.4103/1008-682X.185848. [Epub ahead of print].
de Lamirande E, Tsai C, Harakat A, Gagnon C. Involvement of reactive oxygen species in human sperm arcosome reaction induced by A23187, lysophosphatidylcholine, and biological fluid ultrafiltrates. J Androl. 1998 Sep-Oct;19(5):585-94.
Blomberg Jensen M, Bjerrum PJ, Jessen TE, et al. Vitamin D is positively associated with sperm motility and increases intracellular calcium in human spermatozoa. Hum Reprod. 2011 Jun;26(6):1307-17.
Finley J. Oocyte activation and latent HIV-1 reactivation: AMPK as a common mechanism of action linking the beginnings of life and the potential eradication of HIV-1. Med Hypotheses. 2016 Aug;93:34-47.
Soto-Acosta R, Bautista-Carbajal P, Cervantes-Salazar M, Angel-Ambrocio AH, Del Angel RM. DENV up-regulates the HMG-CoA reductase activity through the impairment of AMPK phosphorylation: A potential antiviral target. PLoS Pathog. 2017 Apr 6;13(4):e1006257.
Park SK, Shin OS. Metformin Alleviates Aging Cellular Phenotypes in Hutchinson-Gilford Progeria Syndrome Dermal Fibroblasts. Exp Dermatol. 2017 Feb 13. doi: 10.1111/exd.13323. [Epub ahead of print].
Egesipe, Blondel, Cicero, et al. Metformin decreases progerin expression and alleviates pathological defects of Hutchinson–Gilford progeria syndrome cells. npj Aging and Mechanisms of Disease 2, Article number: 16026 (2016); http://www.nature.com/articles/npjamd201626?WT.feed_name=subjects_drug-discovery
Finley J. Alteration of splice site selection in the LMNA gene and inhibition of progerin production via AMPK activation. Med Hypotheses. 2014 Nov;83(5):580-7.
Deemeh MR, Tavalaee M, Nasr-Esfahani MH. Health of children born through artificial oocyte activation: a pilot study. Reprod Sci. 2015 Mar;22(3):322-8.
Finley J. Reactivation of latently infected HIV-1 viral reservoirs and correction of aberrant alternative splicing in the LMNA gene via AMPK activation: Common mechanism of action linking HIV-1 latency and Hutchinson-Gilford progeria syndrome. Med Hypotheses. 2015 Sep;85(3):320-32.
1 note
·
View note