#AFM imaging
Explore tagged Tumblr posts
Text
youtube
#Atomic Force Microscopy#nanomechanical tool#cancer liquid biopsy#cancer diagnostics#extracellular vesicles#circulating tumor cells#nanotechnology#cell elasticity#non-invasive biopsy#cancer biomarkers#early cancer detection#precision oncology#AFM imaging#tumor analysis#biomechanics#personalized cancer treatment#cancer research#molecular profiling#cellular adhesion#liquid biopsy techniques.#Youtube
0 notes
Text
Seeing antibiotics in action
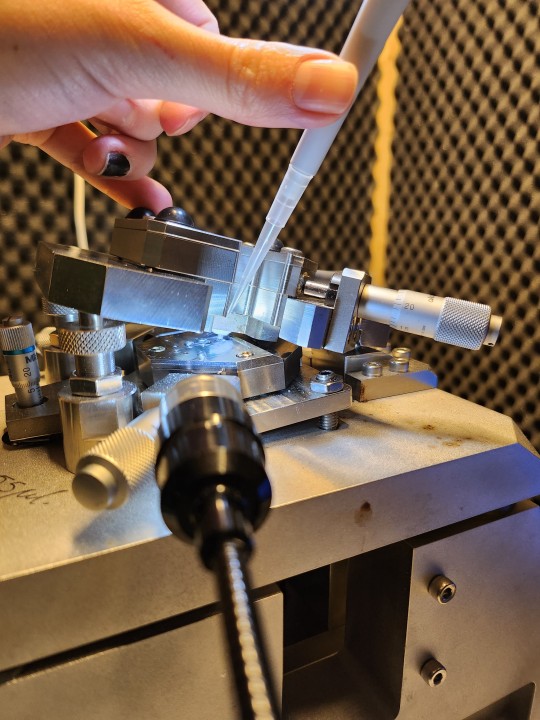
Unveiling the activity of some antibiotics in development using high-speed atomic force microscope. With this machine, I observe interactions between individual molecules in real time.
#biophysics#physics#biology#biochemistry#stem#antimicrobial#antibiotics#research#science#molecules#bacteria#atomic force microscopy#afm#high speed imaging#microscopy#women in science#postdoc#original content
53 notes
·
View notes
Text
A typical AFM image is shown in figure 1.6.

"Chemistry" 2e - Blackman, A., Bottle, S., Schmid, S., Mocerino, M., Wille, U.
1 note
·
View note
Text
X-Men 2 fans: First Look at Nightcrawler and Stryker as brothers in Glenrothan
Brian Cox‘s feature directorial debut “Glenrothan” — billed as his “love letter to Scotland” — has wrapped production, with a first look revealed showing the “Succession” star alongside Alan Cumming in a room full of whisky barrels.
Alongside revealing a first look from the feature, Protagonist Pictures has come aboard for worldwide sales and is set to screen exclusive footage for buyers at the upcoming American Film Market.
Lionsgate, which co-developed the project with London based studio Nevision, has taken U.K. rights on the family drama, which shot in Scotland.
In “Glenrothan,” Donal (Cumming) reluctantly returns to the Scottish Highlands after 35 years in Chicago to make amends with his estranged older brother, Sandy (Cox). Sandy needs Donal to take over the family’s whisky distillery or he will be forced to sell and give up on the family’s legacy. But their reunion forces the brothers to confront the past and the real reason Donal left Glenrothan. Shirley Henderson (“Harry Potter and the Goblet of Fire,” “See How They Run”) and Alexandra Shipp (“Barbie,” “Anyone But You”) also star in the film.
“Glenrothan” is produced by Cox, Neil Zeiger, Crystine Zhang (“Prisoner’s Daughter,” “Lee”), Phin Glynn (“Argentina 1985,” “Kill The Jockey”), Nic Crum (“Book of Love”), James Cabourne and Vladimir Zemtsov (“Club Zero”). The film is co-produced by Nevision and Blazing Griffin Pictures in association with Screen Scotland, Oval-5, Gold Rush Pictures, Head Gear, Infinity Hill and Lionsgate.
“’Glenrothan’ is a poignant, funny and powerful story about the universal themes of family and heritage, set against the stunning backdrops of Scotland and steeped in the world of whisky,” said Protagonist CEO Dave Bishop. “Brian is an undeniable talent, both behind and in front of the camera, and is joined by the exceptional Alan Cumming — as such we are delighted to introduce buyers to this special film when we debut exclusive footage at the upcoming AFM.”
Neil Zeiger, lead producer added: “I have thoroughly enjoyed helping bring ‘Glenrothan,’ Brian’s ‘love letter to Scotland’, to the screen. Working with our incredible cast led by Alan, Shirley and Alexandra, Brian directed the film brilliantly. A universal story, wonderful performances and stunning images define the film. I am confident Glenrothan will compel and captivate audiences wherever in the world they are.”
Protagonist’s current slate includes the Venice, TIFF and San Sebastian selection “Kill the Jockey,” directed by Luis Ortega and recently selected as Argentina’s feature film contender for the 2025 Academy Awards; Samir Oliveros’ TIFF title, “The Luckiest Man in America,” starring Emmy and Golden Globe winner Paul Walter Hauser; the genre-bending comedy “Sister Midnight,” which recently screened at the London Film Festival and was nominated for Cannes’ Camera D’Or; and from directors Guy Maddin, Evan Johnson, and Galen Johnson, the political horror-comedy “Rumours,” which debuted at Cannes before going on to screen at Toronto, San Sebastian, New York and London Film Festivals.
11 notes
·
View notes
Text

Researchers explain the imaging mechanisms of atomic force microscopy in 3D
Researchers at Nano Life Science Institute (WPI-NanoLSI), Kanazawa University report the 3D imaging of a suspended nanostructure. The technique used is an extension of atomic force microscopy and is a promising approach for visualizing various 3D biological systems. Atomic force microscopy (AFM) was originally invented for visualizing surfaces with nanoscale resolution. Its basic working principle is to move an ultrathin tip over a sample's surface. During this xy-scanning motion, the tip's position in the direction perpendicular to the xy-plane follows the sample's height profile, resulting in a height map of the surface.
Read more.
#Materials Science#Science#Atomic force microscopy#Materials characterization#Nanotechnology#Kanazawa University
16 notes
·
View notes
Text
Atomic force microscopy, or AFM, is a widely used technique that can quantitatively map material surfaces in three dimensions, but its accuracy is limited by the size of the microscope's probe. A new AI technique overcomes this limitation and allows microscopes to resolve material features smaller than the probe's tip. The deep learning algorithm developed by researchers at the University of Illinois Urbana-Champaign is trained to remove the effects of the probe's width from AFM microscope images. As reported in the journal Nano Letters, the algorithm surpasses other methods in giving the first true three-dimensional surface profiles at resolutions below the width of the microscope probe tip.
Continue Reading.
47 notes
·
View notes
Text
I will not comment on the French beyond saying that some of their 1930's-40's developments in chemical protection come off as almost Baroque in nature. This is a variant of the GEP (Gaz Et Protection) with an added speech diaphragm. The large metal cone in the 3rd image is to direct the user's voice into said cone. Placement on the side of the mask like this aids in the use of a standard telephone handset.
GEPs were one of many, many, many private market civilian respirators manufactured in Europe during the decades surrounding World War 2. Both the carrying case and speech diaphragm on this example appear very visually similar to the respective components of the AFM-34 kit. Not entirely sure if this is because GEP made AFM-34s, or because the patterns and shapes were readily available from a common source and simply purchased by GEP as stock for assembling their masks.
15 notes
·
View notes
Text

SUBJECT
The superior physicochemical ambiance provided by Chitin/Carboxylated Chitosan for the formation of hydroxyapatite film
DEPARTMENT
Department of Chemical Engineering
PROFESSOR AND STUDENT
Professor:Ten-Chin Wen
Student:Wei-Cheng Li
ABSTRACT
Chitosan (CS) and chitin (CH) are two natural polysaccharides. Chitosan with different carboxylation degrees rendering specific zwitterionic properties. In this study, carboxylated chitosan (CCS) and CH polymeric matrix was mineralized to form an hydroxyapatite film.
CS grafted carboxylated group at pH 6, 8, and 10 for products denoted as CCS6, CCS8, CCS10. It was the better degrees of carboxylated, the higher ion conductivity. CCS with zwitterion helped ions movement. Based on thermogravimetric analysis, thermal cracking temperature of the amide group on chitin increased after mineralization. In the TEM image, the PILP behavior was found, and formed hexagonal hydroxyapatite beside chitin. The Young's modulus of hydroxyapatite evaluated by AFM was 5.19±0.06GPa. Meanwhile, Ca/P ratio by EDX analysis was 1.65, similar to bones.
Original URL: 15-Student:Department of Chemical Engineering【Wei-Cheng Li】-Undergraduate Research , NCKU https://en.ur.ncku.edu.tw/book/15-Student:Department+of+Chemical+Engineering%E3%80%90Wei-Cheng+Li%E3%80%91/
The copyright belongs to the author. For commercial reprints, please contact the author for authorization, and for non-commercial reprints, please indicate the source.
2 notes
·
View notes
Note
So on the whole nano vehicles thing!
This is something people are researching in the field of nanotechnology! It's a field that combines chemistry, biology, physics (and maths/computer science in some ways but that makes it even more complicated) on the nano scale! Now first what even is nano? Nano refers to the scale of something of 10^-9 in this case it's about sizes but nano is a prefix so eh.
Regardless nanotech is a relatively new field (if you're interested in it there's a lecture called "there's plenty of room at the bottom" by Richard P. Feynman who is often considered the parent of nanotechnology) having only been brought up by the previously mentioned, R. Feynman in 1959 and only really sparking the research field to start in the 1980s.
Anyways so yay small scale science! So nanovehicles: nanovehicles are often bottom up (see: bottom-up = using bricks to build a house vs. top down = carving a house out of rock) produced small "vehicles". They came about because we're looking for ways to transport cargos in a more controlled method than we've been able to mimicking certain biological functions. But the way we've being going about it is by making tiny little car shaped molecules. As you can see in the image below just car shaped with Buckminster fullerene (not actually buckminster fullerene but spherical carbon structures) type wheels.
So basically just like cars work, movement is perpendicular to the axle(usually), however you cannot change the positioning of the axles so this thing can only move forwards and backwards.
Oh quick little side note to even analyse the motion of these things, we have to put them on a surface and depending on the type of motor that can lead to either a caterpillar like motion or just increasingly long tails. But what we can also do is use the tips AFM (atomic force microscopy)or SPM (scanning probe microscopy) and move them with that however that's incredibly expensive Sheesh. We have to do this on a surface because of Brownian motion because these things are so small they'll just move around and about randomly in solution.
Okay back to vehicles. So before I showed a 2 directional transverse moving nanocar. But in the previous ask I said there's nanovehicles that can only spin around themselves. And that's because the Axel's are thusly oriented that any transverse motion it wants to make would be obstructed by another axle. So then it can only do the spinny thing.
See below:
a) shows translational motion such as a nanocar can make.
b) shows rotational motion, such as the nanovehicle I was so excited about.
As well as these images having microscopy which shows how they have moved and where the vehicles are and it's very cool.
And below you can see different types of nanovehicles, 22 & 23 can do translational motion forwards and backwards. 24 can move in a slight circle around a middle point that is not its own center point.
And then you have 25, my beloved, it can only spin in circles <3 its sole purpose is to just spin around and around and around and I think that's somehow so very poetic, the fact you've been made in such a way that your sole purpose cannot be fulfilled but you did prove that yes we can move nanovehicles in more than just the two directions. Also that molecule is a blorbo to me.
i forgot all about this and i have still never been so interested in something i understood next to nothing about thank you for infodumping lulu i heart you
2 notes
·
View notes
Text
The Revolutionary History of Nanotechnology

Nanotechnology, a groundbreaking field that has revolutionized numerous industries, continues to shape the world as we know it. In this article, we delve into the rich history of nanotechnology, exploring its origins, major milestones, and transformative applications. Join us on this captivating journey through the nano realm and discover how this remarkable technology has reshaped various sectors, from healthcare and electronics to energy and materials science.
Origins of Nanotechnology
Unveiling the Nanoscale
Nanotechnology finds its roots in the exploration of the minuscule world at the nanoscale. The concept of nanoscale was first introduced by physicist Richard Feynman in his visionary lecture in 1959, where he discussed the potential for manipulating matter at the atomic and molecular levels. This groundbreaking concept laid the foundation for the birth of nanotechnology.
The Birth of Nanotechnology
In 1981, the term "nanotechnology" was officially coined by engineer K. Eric Drexler in his influential book, "Engines of Creation." Drexler envisioned a future where nanomachines could manipulate matter at the atomic scale, leading to remarkable advancements in various fields. His work served as a catalyst for the rapid development of nanotechnology research and applications.
Major Milestones in Nanotechnology
Scanning Probe Microscopy
In the early 1980s, the invention of scanning probe microscopy revolutionized nanotechnology research. The scanning tunneling microscope (STM) and atomic force microscope (AFM) allowed scientists to visualize and manipulate individual atoms and molecules with unprecedented precision. These breakthroughs opened up new possibilities for studying nanoscale phenomena and laid the groundwork for further advancements in the field.
Fullerenes and Nanotubes
In 1985, a significant discovery shook the scientific community—the identification of fullerenes. Researchers Robert Curl, Harold Kroto, and Richard Smalley stumbled upon these unique carbon molecules, marking the birth of a new class of nanomaterials. Fullerenes paved the way for the development of carbon nanotubes, cylindrical structures with remarkable strength and conductivity. These nanotubes would go on to become key building blocks in various nanotechnology applications.
Nanotechnology in Medicine
Nanotechnology's potential to revolutionize healthcare became evident with the advent of targeted drug delivery systems. Nanoparticles, such as liposomes and polymeric nanoparticles, can be designed to encapsulate drugs and deliver them precisely to targeted cells or tissues. This approach minimizes side effects and maximizes therapeutic efficacy. Additionally, nanotechnology plays a vital role in imaging techniques, enabling highly sensitive and precise detection of diseases at the molecular level.
Nanoelectronics and Quantum Computing
The relentless pursuit of smaller, faster, and more energy-efficient electronics led to the emergence of nanoelectronics. By utilizing nanoscale materials and devices, researchers have pushed the boundaries of traditional silicon-based technology. Nanoscale transistors, quantum dots, and nanowires have paved the way for advancements in computing power, memory storage, and energy efficiency. Furthermore, the field of quantum computing, which harnesses quantum phenomena at the nanoscale, holds the promise of solving complex problems that are currently beyond the capabilities of classical computers.
Nanomaterials and Energy
Nanotechnology has also played a significant role in addressing global energy challenges. By developing advanced nanomaterials, scientists have made strides in enhancing solar cell efficiency, enabling the production of clean and renewable energy. Nanomaterials have also been employed in energy storage devices, such as batteries and supercapacitors, to improve their performance and longevity. Additionally, nanotechnology has opened up avenues for energy harvesting and energy conversion, contributing to a more sustainable future.
Transformative Applications of Nanotechnology
Nanomedicine and Disease Treatment
Nanotechnology has revolutionized medicine, offering innovative solutions for disease diagnosis, treatment, and prevention. Targeted drug delivery systems, nanoscale imaging techniques, and nanobiosensors have transformed the landscape of healthcare, enabling personalized and precise interventions. From cancer therapy to regenerative medicine, nanotechnology has the potential to revolutionize patient care and improve outcomes.
Nanoelectronics and Wearable Technology
The marriage of nanotechnology and electronics has given rise to the era of wearable technology. Nanoscale sensors, flexible displays, and energy-efficient components have paved the way for smartwatches, fitness trackers, and augmented reality devices. These advancements in nanoelectronics have made it possible to integrate technology seamlessly into our everyday lives, enhancing convenience and connectivity.
Nanomaterials and Advanced Manufacturing
Nanotechnology has propelled advancements in materials science and manufacturing. Nanomaterials with tailored properties and enhanced performance characteristics have found applications in aerospace, automotive, and construction industries. From lightweight and high-strength composites to self-cleaning surfaces and energy-efficient coatings, nanomaterials have revolutionized product design, durability, and sustainability.
In Conclusion
Nanotechnology's journey from its conceptualization to its present-day applications has been nothing short of extraordinary. The field's remarkable achievements in diverse domains, including medicine, electronics, and energy, continue to drive innovation and shape the future. As we delve deeper into the nanoscale world, the possibilities seem boundless. With ongoing research and collaboration, nanotechnology will undoubtedly unlock new frontiers, leading to breakthroughs that will reshape industries and improve lives across the globe.
#history of nanotechnology#richard feynman#chemestry#green chemistry#nanotechnology#science#nanomaterials#nanocoating#nanomedicine#probe microscopy#revolutionary
3 notes
·
View notes
Text
Powerhouse of Precision: Exploring the Key Features of Piezo Stacks
Piezo stacks are innovative devices that utilize the piezoelectric effect to achieve precise and controlled movements. These stacks consist of multiple layers of piezoelectric ceramic materials stacked together, enabling them to generate high forces and displacements with nanoscale precision. In this infographic, we explore the key features, working principle, and applications of piezo stacks.
For More Information Please visit, pzt stacks
Key Features of Piezo Stacks:
Nanoscale Precision: Piezo stacks offer exceptional precision, allowing for nanometer-level movements and control. This precision is crucial in applications such as microscopy, semiconductor manufacturing, and optical alignment.
High Force Output: Despite their compact size, piezo stacks can generate significant amounts of force. The stacked design allows for the amplification of force, enabling precise control even in applications that require high force requirements.
Rapid Response: Piezo stacks can respond to electrical signals in microseconds, enabling fast and dynamic adjustments. Their rapid response is vital in applications that require quick positioning or movement changes.
Direct Drive and Non-Magnetic Operation: Piezo stacks operate without the need for mechanical gears or magnetic components. This direct drive mechanism eliminates backlash and allows for precise control, even in non-magnetic environments.
Working Principle of Piezo Stacks:
Piezoelectric Effect: Piezo stacks utilize the piezoelectric effect, which refers to the ability of certain materials to generate an electric charge when subjected to mechanical stress. This effect allows the stack to convert electrical signals into precise mechanical movements.
Layered Structure: Piezo stacks consist of multiple thin layers of piezoelectric ceramics. Each layer is carefully engineered to maximize the piezoelectric effect.
Voltage Application: When a voltage is applied to the stack, an electric field is created within the piezoelectric layers. This field causes the layers to expand or contract, resulting in precise mechanical displacements.
Applications of Piezo Stacks:
Precision Positioning: Piezo stacks are extensively used in positioning systems for microscopy, atomic force microscopy (AFM), lithography, and optical alignment. Their precise control allows for accurate positioning and scanning.
Micro-dispensing and Jetting: Piezo stacks find application in micro-dispensing and jetting systems, enabling precise control of fluid flow. They are utilized in industries such as inkjet printing, pharmaceuticals, and biotechnology.

Active Damping and Vibration Control: Piezo stacks are employed in active damping and vibration control systems to minimize unwanted vibrations. They find use in precision manufacturing equipment, semiconductor fabrication, and optical instruments.
Adaptive Optics: In astronomy and laser applications, piezo stacks are used in adaptive optics systems to compensate for atmospheric distortions. They allow for real-time adjustments of deformable mirrors, improving image quality and resolution.
Conclusion:
Piezo stacks provide an effective solution for achieving precision and control in various industries. Their nanoscale precision, high force output, rapid response, and direct drive operation make them invaluable in applications requiring accurate positioning, micro-dispensing, vibration control, and adaptive optics. With ongoing advancements in technology, piezo stacks are poised to continue empowering innovation and driving progress in precision engineering and control systems.
2 notes
·
View notes
Text

Here is the Playlist of the first Show this Year!
Hope you liked it?
Stormwitch-Rondo ala Turca-eye of the Storm-1989-(Hot Blood Records) Danger Danger-Monkey Business-Screw it!-1991-(Epic Records) Casanova-Burning Love-Casanova-1991-(Warner Records) Firehouse-All She wrote-Firehouse-1990-(CBS Records) Gorky Park-Peace in our Time-Gorky Park-1989-(PolyGram Records) Chris Rosander-Northern Lights-The Monster Inside-17.02.2023-(Pride&Joy Music) Gotthard-What I like-Human Zoo-2003-(BMG Records) Ronnie Atkins-Unsung Heroes-Make it Count-2022-(Frontiers Records) Miss Behaviour-Runaway Man-Heart of Midwinter-17.02.2023-(Pride&Joy Music) Nestor-On the Run-Kids in a Ghost Town-2021-(Nestor Prestor Music Group) Sebastian Bach-Kicking and Screaming- Kicking and Screaming-2011-(Frontiers Records) Shakra-Crazy-Back on Track-2011-(AFM Records) Sargant Fury-Wrong Place/Wrong Time-Little Fish-1993-(WEA Records) Ghost-Call me little Sunshine-Impera-2022-(Loma Vista Recordings) Katatonia-Birds-Sky Void of Stars-20.01.2023-(Napalm Records) Dream Theater-Pull me under-Images and Words-1992-(ATCO Records) Metallica-One-…and Justice for all-1988-(Phonogram Records) Saxon-The Pilgrimage-Carpe Diem-2022-(Silver Lining Music) Iron Maiden-Fear of the Dark-Fear of the Dark-1992-(EMI Records) Nightwish-Wish I had an Angel-Once-2004-(Nuclear Blast Records) Stratovarius-Season of Change-Episode-1996-(Modern Music Records) Accept-Heaven is Hell-Russian Roulette-1986-(RCA Records) Armored Saint-After me,The Flood-Revelation-2000-(Metal Blade Records) Blind Guardian-Mr. Sandman (Single)-1996-(Virgin Records)
#hard rock party#youtube#hard rock#rockchicks radio#rock#rock of ages#rock music#rock n roll#rock party#classic rock#glam rock#rock and roll#heavy metal maniac#heavy metal#metal music#speed metal#80s metal#thrash metal#80s music
3 notes
·
View notes
Text
My artistic side is not lost in the world of science!
My art featuring antibiotics that slow down or dissolve domains in bacteria membranes was selected as the cover of the latest Nano Letters issue! The cover art combines hand-drawn bacteria and 3D rendered atomic force microscopy images of bacteria membranes.
My process of drawing and shading the coccus bacteria:

And the final cover:

I had a great time thinking about the concept of this cover, drawing the bacteria, making the 3D bacteria membrane views from my AFM data, and putting everything together. It was a great creative work, although it was under a stressful deadline for the manuscript resubmission including the cover art suggestion.
The Nano Letters issue and the article here: https://pubs.acs.org/toc/nalefd/24/38
#science#art#postdoc#research#bacteria#antibiotics#original content#cover art#journal cover#Nano Letters#publication#scientific illustration#scientific publication
27 notes
·
View notes
Text
#RussellCrowe volverá a enfrentar al Imperio Romano en #TheLastDruid √
El ganador del Oscar Russell Crowe regresará para luchar contra el Imperio Romano en la película de acción The Last Druid, proyecto fílmico que recientemente tuvo un gran éxito de ventas para AGC en el AFM. Russell Crowe / Imagen cortesía Pool Insabato Rovaris/Mondadori Portfolio/Getty Images 25 años después de que se vengó de Roma en Gladiator, Crowe está dispuesto a despertar una vez más al…
0 notes
Text
0 notes
Text

Imaging advance creates clearer picture of organic solar cells' molecular structure
Research on organic solar cells has been conducted for a long time. Recent advancements in understanding their molecular structures are now paving the way for the development of highly efficient solar cells. "By using atomic force microscopy-infrared spectroscopy, AFM-IR, we've been able to create clearer images of the morphology or structure of the material," says Ishita Jalan, postdoc in physical chemistry and main author of a recently published article in ACS Applied Polymer Materials. This structure and how it can be controlled determines the effect of the solar cell.
Read more.
#Materials Science#Science#Solar power#Materials characterization#Structures#Atomic force microscopy#Polymers#Karlstad University
4 notes
·
View notes