#orthopyroxene
Explore tagged Tumblr posts
Note

Clinopyroxene (blue) with orthopyroxene (brown to grey) exsolution lamellae under cross polarized light
5 notes
·
View notes
Text
So, today is my second to last day at work, after 15 years there, and given that a)They Made Me Redundant for stupid Political Reasons, and b)there's almost nothing left to do except turn the lights off, in between finishing up scrapping lab equipment and recording the details of the rock samples that we're not throwing out (5 km of geotechnical and scientific drill core is going in the hardfill skips tho).
So I paused this afternoon and cut a block of peridotite, and a block of rodingite, with the core drill, starting with a 20 mm core barrel, going partway through, then overdrilling with a 60 mm core barrel, all the way through.
Then to the diamond saw, I cut through the overdrilled section, to remove the outside, leaving a 20mm core, attached to a wider 60mm cylinder as a handle, then slab cut the sides of the cylinder off, so I now have a 60 mm by 50 by 20 mm rectangular block on the end of a ~120 mm long cylinder, then cut two triangular sections off the leading long edge of the block, making a triangular wedge section.
Thence to the diamond lap, to shape the working edges, and then smooth the handles.
I now have two more Best Tools: hand cut stone sailor's seam flatteners, made in peridotite from the mantle, and in rodingite, a grossular garnet and omphacite orthopyroxene eclogite relative found only in Aotearoa New/Zealand:

Rodingite one to the top, Peridotite to the bottom.

The rodingite one is best, I think. It's so very hard and dense, having been right down there at the base of the crust before being obducted, and it's taken a lovely polish.

The Peridotite one is a little less smooth, being of course a softer material than grossular garnet. I'm also a little worried that it's got enough incipient fractures that it may break. Time will tell.
But both will be very useful, and I'm of a mind to keep one, and give the other to a friend who also needs a Best Tool.

Every so often, I bestow upon an item the use name 'Best tool'. Here are the three current bearers: A flint chopper and scraper I made when I was ~18, A boxwood seam flattener/plumber's sheet metal folder out of Grandad Alan's tool chest, and the strap cutter Bjorn gave me last month.
#Best Tool#Stone tools#they left me unattended in the diamond saw prep room#Rodingite#Eclogite#Peridotite#Olivine#Grossular Garnet#Omphacite#Orthopyroxenes#chromite
24 notes
·
View notes
Text
Basalt, XPL, 5x magnification
Here's some of the basalt that I've been finding all those lovely orthopyroxenes in. Really a spectacular sample!

288 notes
·
View notes
Text

Solid solution of orthopyroxene within clinopyroxene aka the tiger rock that was a bitch in our prac for a number of reasons.
109 notes
·
View notes
Text

Today's Specimen: Peridotite
Peridotite, not to be confused with Peridot, it's gemstone variety, is a rock rich with Pyroxene and Olivine, which is the material Peridot comes from (the gem form of Olivine). Peridotite is ultramafic (containing high magnesium and iron, but is low in silica) and coarse, and makes up a significant part of the Earth's mantle. This funky rock is an essential source of iron, nickel, talc, chromium, cobalt, peridot, garnets, and even diamonds! It has a hardness of 5.5-6.0 on the Mohs hardness scale, depending on type. Types of Peridotite include: Harzburgite (contains orthopyroxene), Lherzolite (contains orthopyroxene and clinopyroxene), Wherlite (contains clinopyroxene), and Dunite (contains over 90% olivine).
Stay tuned for another rock talk!
11 notes
·
View notes
Text



Seismic clues from Marsquakes suggest liquid water and life potential beneath the surface
Are subterranean lifeforms viable on Mars? A new interpretation of Martian seismic data by scientists Ikuo Katayama of Hiroshima University and Yuya Akamatsu of Research Institute for Marine Geodynamics suggests the presence of water below the surface of Mars. "If liquid water exists on Mars," Katayama says, "the presence of microbial activity" is possible.
This analysis is based on seismic data from SEIS (Seismic Experiment for the Interior Structure), deployed from NASA's InSight lander that landed on Mars in 2018. This robotic lander is unique because it was able to use its robotic arm to place a seismometer on the surface of Mars. The SEIS instrument, which contains the seismometer, uses the seismic waves naturally generated on Mars from Marsquakes or meteorite impacts to scan the planet's interior.
When a Marsquake or meteorite impact occurs on Mars, SEIS can read the energy emitted as P-waves, S-waves, and surface waves to create an image of the planet's interior. Scientists can use P-waves and S-waves to determine a lot about the rocks that make up Mars, including the density of the rocks or potential composition changes within the rocks.
For example, S-waves cannot travel through water and move at a slower speed than P-waves. Therefore, the presence, absence, and arrival time of S-waves can determine what the subsurface looks like. Moreover, P-waves can travel faster through higher-density material and slower through less dense material, so their velocity can help determine the density of the material the wave is traveling through, as well as if there are any changes in density along its path. The seismic data collected with SEIS shows a boundary at 10 km depth and 20 km depth from measured discrepancies in seismic velocity.
This boundary has previously been interpreted as sharp transitions in the porosity (the percentage of open space in a rock) or chemical composition of the Martian interior. However, Katayama and Akamatsu have interpreted these cracks as potential evidence for water within the Martian subsurface. The seismic data indicate a boundary between dry cracks and water-filled cracks in the Martian subsurface. To test their hypothesis, they measured the seismic velocity passing through rocks with the same structures and composition of a typical Martian crustal rock under wet, dry, and frozen conditions.
A typical Martian rock is similar to the diabase rocks from Rydaholm, Sweden, due to their evenly sized plagioclase and orthopyroxene grains. In the lab, Katayama and Akamatsu measured P-wave and S-wave velocity using a piezoelectric transducer, which uses "electrical energy . . . as a wave source" that "monitor[s] seismic wave energy" on dry, wet, and frozen diabase samples. Experimentation revealed that the seismic velocities of the dry, wet, and frozen samples are significantly different, which supports the interpretation that the boundary at 10 km and 20 km could be from a change from dry rock to wet rock.
These laboratory experiments back up Katayama and Yuya's hypothesis that the boundary measured by seismic data indicates a transition from dry rock to wet rock rather than a change in porosity or chemical composition. The findings, therefore, provide compelling evidence for the existence of liquid water beneath the surface of Mars. "Many studies suggest the presence of water on ancient Mars billions of years ago," Katayama explains, "but our model indicates the presence of liquid water on present-day Mars."
TOP IMAGE: NASA's InSight lander is shown above with all of its different devices that have been used for scientific discovery. The SEIS (Seismic Experiment for the Interior Structure) seismometer is shown to the bottom left of the lander. Credit: Ikuo Katayama
CENTRE IMAGE: A diagram showing how different seismic waves travel across Mars. Credit: Ikuo Katayama
LOWER IMAGE: A panel of figures showing how S-wave and P-wave velocities, the ratio of P-wave and S-wave velocity, and porosity change throughout the Martian subsurface. The diagram on the far right shows what these differences mean for each rock layer. Credit: Ikuo Katayama
7 notes
·
View notes
Text
Rock Swag Tournament Round 1: Igneous Rocks Part 8
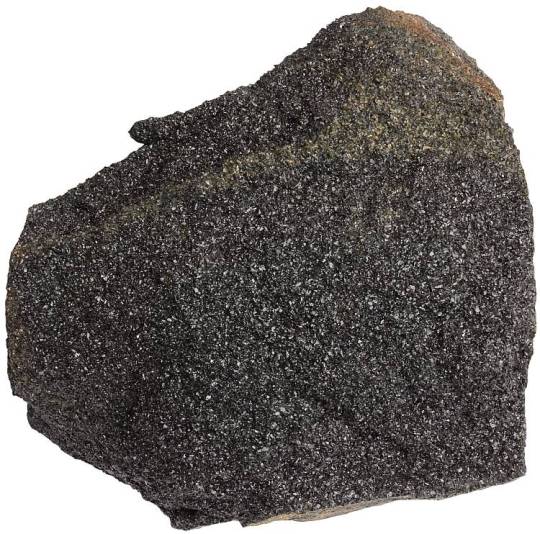

Once again we have some rocks named after minerals! This time, they're both ultramafic. Also this time, we also have a fun diagram to look at.

Ternary plots, very useful but sometimes difficult to plot on (at least for me. I always confuse myself, even now). But we're not plotting anything, we're just looking at trends!
If you look at the three corners of this triangle, you will see they are labelled Ol for olivine), Opx for orthopyroxene, and Cpx for clinopyroxene. These corners tell us what three things we are comparing the proportions of. In this case, we are comparing what percent of olivine, orthopyroxene, and clinopyroxene are in a rock (and what we call that rock based on the relative amounts of these minerals).
Ternary diagrams can have any three things in the corners, but you'll often see QFL (Quartz, Feldspar, Lithics) diagrams to classify sedimentary rocks, or sand-silt-clay diagrams for soil texture. You can also stick two triangles together to make a diamond for something like a QAPF diagram where the four points are quartz, alkali feldspar, plagioclase, and feldspathoids. QAPF diagrams are also used to classify igneous rocks (you can plot syenite and monzonite on them, as well as your granites).
The closer you get to the top corner of the triangle, the more olivine you have. The closer you get to the right corner of the triangle, the more clinopyroxene you have, and the closer you get to the left corner of the triangle, the more orthopyroxene you have.
If your rock sample plots in the top half (ish) of the triangle (at least 40% olivine), your rock is a peridotite. Now, depending on the relative amounts of pyroxene minerals, you may have different types of peridotite like lherzolite, wherlite, harzburgite, or dunite.
In the picture of peridotite above, the peridotite is actually the xenolith (the green rock embedded in the black rock) and it would be considered dunite because it is more than 90% olivine. The reason a rock full of olivine is called peridotite is because peridot is another name for olivine (specifically gemstone-quality olivine).
As for pyroxenites, if you have less than 40% olivine, the rock would be a pyroxenite. If it's almost all pyroxene (no more than ~5% olivine, at the bottom of the pyramid), it would be called websterite.
As for other facts about the rocks, both peridotite and pyroxenite are typically intrusive, phaneritic rocks derived from the mantle. Peridotite is going to be some shade of green because of the olivine, and pyroxenite is usually black or at least dark in color.
I'm also thinking of making an informational post about volcanoes, partial melting (and magma composition) and how these rocks/magmas get from the mantle to the surface, so look out for that at some point (I know myself better than to name a specific timeline).
125 notes
·
View notes
Text
Inspired by the soil poll, let's make one of my FAVORITE ROCK COMPOSITIONS
37 notes
·
View notes
Note
1/?
The Moon is a very slightly scalene ellipsoid due to tidal stretching, with its long axis displaced 30° from facing the Earth, due to gravitational anomalies from impact basins. Its shape is more elongated than current tidal forces can account for. This 'fossil bulge' indicates that the Moon solidified when it orbited at half its current distance to the Earth, and that it is now too cold for its shape to restore hydrostatic equilibrium at its current orbital distance.
Size and mass
Further information: List of natural satellites
Size comparison of the main moons of the Solar System with Earth to scale. Nineteen moons are large enough to be round, several having subsurface oceans and one, Titan, having a considerable atmosphere.
The Moon is by size and mass the fifth largest natural satellite of the Solar System, categorizable as one of its planetary-mass moons, making it a satellite planet under the geophysical definitions of the term. It is smaller than Mercury and considerably larger than the largest dwarf planet of the Solar System, Pluto. The Moon is the largest natural satellite in the Solar System relative to its primary planet.
The Moon's diameter is about 3,500 km, more than one-quarter of Earth's, with the face of the Moon comparable to the width of either mainland Australia, Europe or the contiguous United States. The whole surface area of the Moon is about 38 million square kilometers, comparable to that of the Americas.
The Moon's mass is 1⁄81 of Earth's, being the second densest among the planetary moons, and having the second highest surface gravity, after Io, at 0.1654 g and an escape velocity of 2.38 km/s (8600 km/h; 5300 mph).
Structure
Main articles: Internal structure of the Moon and Geology of the Moon
Moon's internal structure: solid inner core (iron-metallic), molten outer core, hardened mantle and crust. The crust on the Moon's near side permanently facing Earth is thinner, featuring larger areas flooded by material of the once molten mantle forming today's lunar mare.
The Moon is a differentiated body that was initially in hydrostatic equilibrium but has since departed from this condition. It has a geochemically distinct crust, mantle, and core. The Moon has a solid iron-rich inner core with a radius possibly as small as 240 kilometres (150 mi) and a fluid outer core primarily made of liquid iron with a radius of roughly 300 kilometres (190 mi). Around the core is a partially molten boundary layer with a radius of about 500 kilometres (310 mi). This structure is thought to have developed through the fractional crystallization of a global magma ocean shortly after the Moon's formation 4.5 billion years ago.
Crystallization of this magma ocean would have created a mafic mantle from the precipitation and sinking of the minerals olivine, clinopyroxene, and orthopyroxene; after about three-quarters of the magma ocean had crystallized, lower-density plagioclase minerals could form and float into a crust atop. The final liquids to crystallize would have been initially sandwiched between the crust and mantle, with a high abundance of incompatible and heat-producing elements. Consistent with this perspective, geochemical mapping made from orbit suggests a crust of mostly anorthosite. The Moon rock samples of the flood lavas that erupted onto the surface from partial melting in the mantle confirm the mafic mantle composition, which is more iron-rich than that of Earth. The crust is on average about 50 kilometres (31 mi) thick.
The Moon is the second-densest satellite in the Solar System, after Io.[78] However, the inner core of the Moon is small, with a radius of about 350 kilometres (220 mi) or less, around 20% of the radius of the Moon. Its composition is not well understood but is probably metallic iron alloyed with a small amount of sulfur and nickel analyses of the Moon's time-variable rotation suggest that it is at least partly molten. The pressure at the lunar core is estimated to be 5 GPa (49,000 atm).
Gravitational field
Astronaut John Young jumping on the Moon, illustrating that the gravitational pull of the Moon is approximately 1/6 of Earth's. The jumping height is limited by the EVA space suit's weight on the Moon of about 13.6 kg (30 lb) and by the suit's pressurization resisting the bending of the suit, as needed for jumping.
On average the Moon's surface gravity is 1.62 m/s2[4] (0.1654 g; 5.318 ft/s2), about half of the surface gravity of Mars and about a sixth of Earth's.
The Moon's gravitational field is not uniform. The details of the gravitational field have been measured through tracking the Doppler shift of radio signals emitted by orbiting spacecraft. The main lunar gravity features are mascons, large positive gravitational anomalies associated with some of the giant impact basins, partly caused by the dense mare basaltic lava flows that fill those basins. The anomalies greatly influence the orbit of spacecraft about the Moon. There are some puzzles: lava flows by themselves cannot explain all of the gravitational signature, and some mascons exist that are not linked to mare volcanism.
Magnetic field
The Moon has an external magnetic field of less than 0.2 nanoteslas, or less than one hundred thousandth that of Earth. The Moon does not have a global dipolar magnetic field and only has crustal magnetization likely acquired early in its history when a dynamo was still operating. Early in its history, 4 billion years ago, its magnetic field strength was likely close to that of Earth today. This early dynamo field apparently expired by about one billion years ago, after the lunar core had crystallized. Theoretically, some of the remnant magnetization may originate from transient magnetic fields generated during large impacts through the expansion of plasma clouds. These clouds are generated during large impacts in an ambient magnetic field. This is supported by the location of the largest crustal magnetizations situated near the antipodes of the giant impact basins.
Atmosphere
Main article: Atmosphere of the Moon
The thin lunar atmosphere is visible on the Moon's surface at sunrise and sunset with the lunar horizon glow and lunar twilight rays, like Earth's crepuscular rays. This Apollo 17 sketch depicts the glow and rays among the general zodiacal light.
The Moon has an atmosphere consisting of only an exosphere, which is so tenuous as to be nearly vacuum, with a total mass of less than 10 tonnes (9.8 long tons; 11 short tons). The surface pressure of this small mass is around 3 × 10−15 atm (0.3 nPa); it varies with the lunar day. Its sources include outgassing and sputtering, a product of the bombardment of lunar soil by solar wind ions. Elements that have been detected include sodium and potassium, produced by sputtering (also found in the atmospheres of Mercury and Io); helium-4 and neon from the solar wind; and argon-40, radon-222, and polonium-210, outgassed after their creation by radioactive decay within the crust and mantle. The absence of such neutral species (atoms or molecules) as oxygen, nitrogen, carbon, hydrogen and magnesium, which are present in the regolith, is not understood. Water vapor has been detected by Chandrayaan-1 and found to vary with latitude, with a maximum at ~60–70 degrees; it is possibly generated from the sublimation of water ice in the regolith. These gases either return into the regolith because of the Moon's gravity or are lost to space, either through solar radiation pressure or, if they are ionized, by being swept away by the solar wind's magnetic field.
Studies of Moon magma samples retrieved by the Apollo missions demonstrate that the Moon had once possessed a relatively thick atmosphere for a period of 70 million years between 3 and 4 billion years ago. This atmosphere, sourced from gases ejected from lunar volcanic eruptions, was twice the thickness of that of present-day Mars. The ancient lunar atmosphere was eventually stripped away by solar winds and dissipated into space.
A permanent Moon dust cloud exists around the Moon, generated by small particles from comets. Estimates are 5 tons of comet particles strike the Moon's surface every 24 hours, resulting in the ejection of dust particles. The dust stays above the Moon approximately 10 minutes, taking 5 minutes to rise, and 5 minutes to fall. On average, 120 kilograms of dust are present above the Moon, rising up to 100 kilometers above the surface. Dust counts made by LADEE's Lunar Dust EXperiment (LDEX) found particle counts peaked during the Geminid, Quadrantid, Northern Taurid, and Omicron Centaurid meteor showers, when the Earth, and Moon pass through comet debris. The lunar dust cloud is asymmetric, being denser near the boundary between the Moon's dayside and nightside.
Surface conditions
Gene Cernan with lunar dust stuck on his suit. Lunar dust is highly abrasive and can cause damage to human lungs and nervous and cardiovascular systems.
Ionizing radiation from cosmic rays, their resulting neutron radiation, and the Sun results in an average radiation level of 1.369 millisieverts per day during lunar daytime,[14] which is about 2.6 times more than the level on the International Space Station, 5–10 times more than the level during a trans-Atlantic flight, and 200 times more than the level on Earth's surface. For further comparison, radiation levels average about 1.84 millisieverts per day on a flight to Mars and about 0.64 millisieverts per day on Mars itself, with some locations on Mars possibly having levels as low as 0.342 millisieverts per day. Solar radiation also electrically charges the highly abrasive lunar dust and makes it levitate. This effect contributes to the easy spread of the sticky, lung- and gear-damaging lunar dust.
The Moon's axial tilt with respect to the ecliptic is only 1.5427°, much less than the 23.44° of Earth. This small axial tilt means that the Moon's solar illumination varies much less with season than Earth's, and it also allows for the existence of some peaks of eternal light at the Moon's north pole, at the rim of the crater Peary.
The lunar surface is exposed to drastic temperature differences ranging from 120 °C to −171 °C depending on the solar irradiance. Because of the lack of atmosphere, temperatures of different areas vary particularly upon whether they are in sunlight or shadow, making topographical details play a decisive role on local surface temperatures. Parts of many craters, particularly the bottoms of many polar craters,are permanently shadowed. These craters of eternal darkness have extremely low temperatures. The Lunar Reconnaissance Orbiter measured the lowest summer temperatures in craters at the southern pole at 35 K (−238 °C; −397 °F) and just 26 K (−247 °C; −413 °F) close to the winter solstice in the north polar crater Hermite. This is the coldest temperature in the Solar System ever measured by a spacecraft, colder even than the surface of Pluto.
Blanketed on top of the Moon's crust is a highly comminuted (broken into ever smaller particles) and impact gardened mostly gray surface layer called regolith, formed by impact processes. The finer regolith, the lunar soil of silicon dioxide glass, has a texture resembling snow and a scent resembling spent gunpowder. The regolith of older surfaces is generally thicker than for younger surfaces: it varies in thickness from 10–15 m (33–49 ft) in the highlands and 4–5 m (13–16 ft) in the maria. Beneath the finely comminuted regolith layer is the megaregolith, a layer of highly fractured bedrock many kilometers thick.
These extreme conditions are considered to make it unlikely for spacecraft to harbor bacterial spores at the Moon for longer than just one lunar orbit.
Surface features
Main articles: Selenography, Lunar terrane, List of lunar features, and List of quadrangles on the Moon
Apollo 17 astronaut Harrison H. Schmitt next to the large Moon boulder nicknamed "Tracy's Rock"
The topography of the Moon has been measured with laser altimetry and stereo image analysis. Its most extensive topographic feature is the giant far-side South Pole–Aitken basin, some 2,240 km (1,390 mi) in diameter, the largest crater on the Moon and the second-largest confirmed impact crater in the Solar System. At 13 km (8.1 mi) deep, its floor is the lowest point on the surface of the Moon. The highest elevations of the Moon's surface are located directly to the northeast, which might have been thickened by the oblique formation impact of the South Pole–Aitken basin. Other large impact basins such as Imbrium, Serenitatis, Crisium, Smythii, and Orientale possess regionally low elevations and elevated rims. The far side of the lunar surface is on average about 1.9 km (1.2 mi) higher than that of the near side.
The discovery of fault scarp cliffs suggest that the Moon has shrunk by about 90 metres (300 ft) within the past billion years. Similar shrinkage features exist on Mercury. Mare Frigoris, a basin near the north pole long assumed to be geologically dead, has cracked and shifted. Since the Moon does not have tectonic plates, its tectonic activity is slow, and cracks develop as it loses heat.
Scientists have confirmed the presence of a cave on the Moon near the Sea of Tranquillity, not far from the 1969 Apollo 11 landing site. The cave, identified as an entry point to a collapsed lava tube, is roughly 45 meters wide and up to 80 m long. This discovery marks the first confirmed entry point to a lunar cave. The analysis was based on photos taken in 2010 by NASA's Lunar Reconnaissance Orbiter. The cave's stable temperature of around 17 °C could provide a hospitable environment for future astronauts, protecting them from extreme temperatures, solar radiation, and micrometeorites. However, challenges include accessibility and risks of avalanches and cave-ins. This discovery offers potential for future lunar bases or emergency shelters.
Volcanic features
Main article: Volcanism on the Moon
The names of the main volcanic features, the maria (blue), and of some craters (brown) of the near side of the Moon
The main features visible from Earth by the naked eye are dark and relatively featureless lunar plains called maria (singular mare; Latin for "seas", as they were once believed to be filled with water) are vast solidified pools of ancient basaltic lava. Although similar to terrestrial basalts, lunar basalts have more iron and no minerals altered by water. The majority of these lava deposits erupted or flowed into the depressions associated with impact basins, though the Moon's largest expanse of basalt flooding, Oceanus Procellarum, does not correspond to an obvious impact basin. Different episodes of lava flow in maria can often be recognized by variations in surface albedo and distinct flow margins.
As the maria formed, cooling and contraction of the basaltic lava created wrinkle ridges in some areas. These low, sinuous ridges can extend for hundreds of kilometers and often outline buried structures within the mare. Another result of maria formation is the creation of concentric depressions along the edges, known as arcuate rilles. These features occur as the mare basalts sink inward under their own weight, causing the edges to fracture and separate.
In addition to the visible maria, the Moon has mare deposits covered by ejecta from impacts. Called cryptomares, these hidden mares are likely older than the exposed ones. Conversely, mare lava has obscured many impact melt sheets and pools. Impact melts are formed when intense shock pressures from collisions vaporize and melt zones around the impact site. Where still exposed, impact melt can be distinguished from mare lava by its distribution, albedo, and texture.
Sinuous rilles, found in and around maria, are likely extinct lava channels or collapsed lava tubes. They typically originate from volcanic vents, meandering and sometimes branching as they progress. The largest examples, such as Schroter's Valley and Rima Hadley, are significantly longer, wider, and deeper than terrestrial lava channels, sometimes featuring bends and sharp turns that again, are uncommon on Earth.
Mare volcanism has altered impact craters in various ways, including filling them to varying degrees, and raising and fracturing their floors from uplift of mare material beneath their interiors. Examples of such craters include Taruntius and Gassendi. Some craters, such as Hyginus, are of wholly volcanic origin, forming as calderas or collapse pits. Such craters are relatively rare and tend to be smaller (typically a few kilometers wide), shallower, and more irregularly shaped than impact craters. They also lack the upturned rims characteristic of impact craters.
Several geologic provinces containing shield volcanoes and volcanic domes are found within the near side maria. There are also some regions of pyroclastic deposits, scoria cones and non-basaltic domes made of particularly high viscosity lava.
Almost all maria are on the near side of the Moon, and cover 31% of the surface of the near side compared with 2% of the far side. This is likely due to a concentration of heat-producing elements under the crust on the near side, which would have caused the underlying mantle to heat up, partially melt, rise to the surface and erupt. Most of the Moon's mare basalts erupted during the Imbrian period, 3.3–3.7 billion years ago, though some being as young as 1.2 billion years and as old as 4.2 billion years.
Old hardened lava flows of Mare Imbrium forming wrinkle ridges
In 2006, a study of Ina, a tiny depression in Lacus Felicitatis, found jagged, relatively dust-free features that, because of the lack of erosion by infalling debris, appeared to be only 2 million years old. Moonquakes and releases of gas indicate continued lunar activity. Evidence of recent lunar volcanism has been identified at 70 irregular mare patches, some less than 50 million years old. This raises the possibility of a much warmer lunar mantle than previously believed, at least on the near side where the deep crust is substantially warmer because of the greater concentration of radioactive elements. Evidence has been found for 2–10 million years old basaltic volcanism within the crater Lowell, inside the Orientale basin. Some combination of an initially hotter mantle and local enrichment of heat-producing elements in the mantle could be responsible for prolonged activities on the far side in the Orientale basin.
The lighter-colored regions of the Moon are called terrae, or more commonly highlands, because they are higher than most maria. They have been radiometrically dated to having formed 4.4 billion years ago and may represent plagioclase cumulates of the lunar magma ocean. In contrast to Earth, no major lunar mountains are believed to have formed as a result of tectonic events.
The concentration of maria on the near side likely reflects the substantially thicker crust of the highlands of the Far Side, which may have formed in a slow-velocity impact of a second moon of Earth a few tens of millions of years after the Moon's formation. Alternatively, it may be a consequence of asymmetrical tidal heating when the Moon was much closer to the Earth.
Impact craters
Further information: List of craters on the Moon
A gray, many-ridged surface from high above. The largest feature is a circular ringed structure with high walled sides and a lower central peak: the entire surface out to the horizon is filled with similar structures
oh wow
Rotation
Main article: Solar rotation
The Sun rotates faster at its equator than at its poles. This differential rotation is caused by convective motion due to heat transport and the Coriolis force due to the Sun's rotation. In a frame of reference defined by the stars, the rotational period is approximately 25.6 days at the equator and 33.5 days at the poles. Viewed from Earth as it orbits the Sun, the apparent rotational period of the Sun at its equator is about 28 days.[47] Viewed from a vantage point above its north pole, the Sun rotates counterclockwise around its axis of spin.[d][48]
A survey of solar analogs suggest the early Sun was rotating up to ten times faster than it does today. This would have made the surface much more active, with greater X-ray and UV emission. Sun spots would have covered 5–30% of the surface.[49] The rotation rate was gradually slowed by magnetic braking, as the Sun's magnetic field interacted with the outflowing solar wind.[50] A vestige of this rapid primordial rotation still survives at the Sun's core, which has been found to be rotating at a rate of once per week; four times the mean surface rotation rate.[51][52]
Composition
See also: Molecules in stars
The Sun consists mainly of the elements hydrogen and helium. At this time in the Sun's life, they account for 74.9% and 23.8%, respectively, of the mass of the Sun in the photosphere.[53] All heavier elements, called metals in astronomy, account for less than 2% of the mass, with oxygen (roughly 1% of the Sun's mass), carbon (0.3%), neon (0.2%), and iron (0.2%) being the most abundant.[54]
The Sun's original chemical composition was inherited from the interstellar medium out of which it formed. Originally it would have been about 71.1% hydrogen, 27.4% helium, and 1.5% heavier elements.[53] The hydrogen and most of the helium in the Sun would have been produced by Big Bang nucleosynthesis in the first 20 minutes of the universe, and the heavier elements were produced by previous generations of stars before the Sun was formed, and spread into the interstellar medium during the final stages of stellar life and by events such as supernovae.[55]
Since the Sun formed, the main fusion process has involved fusing hydrogen into helium. Over the past 4.6 billion years, the amount of helium and its location within the Sun has gradually changed. The proportion of helium within the core has increased from about 24% to about 60% due to fusion, and some of the helium and heavy elements have settled from the photosphere toward the center of the Sun because of gravity. The proportions of heavier elements are unchanged. Heat is transferred outward from the Sun's core by radiation rather than by convection (see Radiative zone below), so the fusion products are not lifted outward by heat; they remain in the core,[56] and gradually an inner core of helium has begun to form that cannot be fused because presently the Sun's core is not hot or dense enough to fuse helium. In the current photosphere, the helium fraction is reduced, and the metallicity is only 84% of what it was in the protostellar phase (before nuclear fusion in the core started). In the future, helium will continue to accumulate in the core, and in about 5 billion years this gradual build-up will eventually cause the Sun to exit the main sequence and become a red giant.[57]
The chemical composition of the photosphere is normally considered representative of the composition of the primordial Solar System.[58] Typically, the solar heavy-element abundances described above are measured both by using spectroscopy of the Sun's photosphere and by measuring abundances in meteorites that have never been heated to melting temperatures. These meteorites are thought to retain the composition of the protostellar Sun and are thus not affected by the settling of heavy elements. The two methods generally agree well.[59]
Structure and fusion
Main article: Standard solar modelIllustration of the Sun's structure, in false color for contrast
Core
Main article: Solar core
The core of the Sun extends from the center to about 20–25% of the solar radius.[60] It has a density of up to 150 g/cm3[61][62] (about 150 times the density of water) and a temperature of close to 15.7 million kelvin (K).[62] By contrast, the Sun's surface temperature is about 5800 K. Recent analysis of SOHO mission data favors the idea that the core is rotating faster than the radiative zone outside it.[60] Through most of the Sun's life, energy has been produced by nuclear fusion in the core region through the proton–proton chain; this process converts hydrogen into helium.[63] Currently, 0.8% of the energy generated in the Sun comes from another sequence of fusion reactions called the CNO cycle; the proportion coming from the CNO cycle is expected to increase as the Sun becomes older and more luminous.[64][65]
The core is the only region of the Sun that produces an appreciable amount of thermal energy through fusion; 99% of the Sun's power is generated in the innermost 24% of its radius, and almost no fusion occurs beyond 30% of the radius. The rest of the Sun is heated by this energy as it is transferred outward through many successive layers, finally to the solar photosphere where it escapes into space through radiation (photons) or advection (massive particles).[66][67]Illustration of a proton-proton reaction chain, from hydrogen forming deuterium, helium-3, and regular helium-4
The proton–proton chain occurs around 9.2×1037 times each second in the core, converting about 3.7×1038 protons into alpha particles (helium nuclei) every second (out of a total of ~8.9×1056 free protons in the Sun), or about 6.2×1011 kg/s. However, each proton (on average) takes around 9 billion years to fuse with another using the PP chain.[66] Fusing four free protons (hydrogen nuclei) into a single alpha particle (helium nucleus) releases around 0.7% of the fused mass as energy,[68] so the Sun releases energy at the mass–energy conversion rate of 4.26 billion kg/s (which requires 600 billion kg of hydrogen[69]), for 384.6 yottawatts (3.846×1026 W),[5] or 9.192×1010 megatons of TNT per second. The large power output of the Sun is mainly due to the huge size and density of its core (compared to Earth and objects on Earth), with only a fairly small amount of power being generated per cubic metre. Theoretical models of the Sun's interior indicate a maximum power density, or energy production, of approximately 276.5 watts per cubic metre at the center of the core,[70] which, according to Karl Kruszelnicki, is about the same power density inside a compost pile.[71]
The fusion rate in the core is in a self-correcting equilibrium: a slightly higher rate of fusion would cause the core to heat up more and expand slightly against the weight of the outer layers, reducing the density and hence the fusion rate and correcting the perturbation; and a slightly lower rate would cause the core to cool and shrink slightly, increasing the density and increasing the fusion rate and again reverting it to its present rate.[72][73]
Radiative zone
Main article: Radiative zoneIllustration of different stars' internal structure based on mass. The Sun in the middle has an inner radiating zone and an outer convective zone.
The radiative zone is the thickest layer of the Sun, at 0.45 solar radii. From the core out to about 0.7 solar radii, thermal radiation is the primary means of energy transfer.[74] The temperature drops from approximately 7 million to 2 million kelvins with increasing distance from the core.[62] This temperature gradient is less than the value of the adiabatic lapse rate and hence cannot drive convection, which explains why the transfer of energy through this zone is by radiation instead of thermal convection.[62] Ions of hydrogen and helium emit photons, which travel only a brief distance before being reabsorbed by other ions.[74] The density drops a hundredfold (from 20,000 kg/m3 to 200 kg/m3) between 0.25 solar radii and 0.7 radii, the top of the radiative zone.[74]
1 note
·
View note
Text







NWA 7811
Classification : achondrite HED (eucrite, polymicte)
NWA 7811 est une fraîche météorite classée eucrite polymict breccia de seulement 95 grammes.
Elle a été découverte en 2011 et classifiée par Anthony Irving en 2012.
Les eucrites proviennent de l’Astéroïde Vesta.
Petrography: (A. Irving and S. Kuehner, UWS) Fresh fragmental breccia composed mainly of crystal debris from several different basaltic eucrite and gabbroic eucrite protoliths (with some polymineralic clasts of same), plus sparse clasts of diogenite and diogenitic orthopyroxene. Minerals include exsolved pigeonite, orthopyroxene, clinopyroxene, calcic plagioclase, silica polymorph, ilmenite, troilite, chromite, and fayalite.
Geochemistry: Diogenitic orthopyroxene (Fs24.1Wo1.5; FeO/MnO = 34), orthopyroxene (Fs49.9Wo2.5; FeO/MnO = 31), clinopyroxene (Fs20.8-25.8Wo45.1-44.6; FeO/MnO = 32-35), host orthopyroxene (Fs61.6Wo1.8; FeO/MnO = 34), clinopyroxene exsolution lamellae (Fs21.6Wo43.8; FeO/MnO = 34), olivine (Fa92.7).
0 notes
Photo

HiPOD 22 Sept 2021: A Colorful Landslide in Eos Chasma
This image was requested in the very first month of MRO’s Primary Science Phase, November 2006. Due to many competing targets in the Valles Marineris canyon system, it took nearly 15 years to acquire. But it was worth the wait!
A massive landslide has transported diverse rocks from the canyon’s wall layers down onto its floor, jumbling them up in the process. Lower-resolution infrared data had previously revealed an unusual concentration of the igneous mineral orthopyroxene at this location. The range of colors visible to HiRISE implies that many other minerals are present here as well.
Enhanced color images is less than 1 km across.
ID: ESP_070264_1675 date: 23 July 2021 altitude: 265 km (165 mi)
NASA/JPL/UArizona
71 notes
·
View notes
Text

Orthopyroxene in basalt, XPL, 10X magnification.
#*wipes tear away*#isnt he the most perfect little hexagon?#geology#in which cube is a geologist#thin section#basalt
109 notes
·
View notes
Photo










Summer Sky, Tyresö (No. 8)
Crystallization of this magma ocean would have created a mafic mantle from the precipitation and sinking of the minerals olivine, clinopyroxene, and orthopyroxene; after about three-quarters of the magma ocean had crystallised, lower-density plagioclase minerals could form and float into a crust atop. The final liquids to crystallise would have been initially sandwiched between the crust and mantle, with a high abundance of incompatible and heat-producing elements. Consistent with this perspective, geochemical mapping made from orbit suggests a crust of mostly anorthosite. The Moon rock samples of the flood lavas that erupted onto the surface from partial melting in the mantle confirm the mafic mantle composition, which is more iron-rich than that of Earth. The crust is on average about 50 kilometres (31 mi) thick.
The Moon is the second-densest satellite in the Solar System, after Io. However, the inner core of the Moon is small, with a radius of about 350 kilometres (220 mi) or less, around 20% of the radius of the Moon. Its composition is not well understood, but is probably metallic iron alloyed with a small amount of sulfur and nickel; analyses of the Moon's time-variable rotation suggest that it is at least partly molten. The pressure at the lunar core is estimated to be 5 GPa (49,000 atm).
Source: Wikipedia
#Tyresö#Stockholm County#moon rise#moon rising#moon#Sweden#Sverige#Scandinavia#Northern Europe#silhouette#original photography#tourism#nature#flora#pastel#tree#bush#fir#pine#evening light#evening sky#natural satellite#summer 2020#travel#vacation#blue hour#dusk#astronomy#astronomical photography#close up
16 notes
·
View notes
Photo

Rock from inside of the Earth
Top and bottom image is of the same feature but using different light filters in the microscope.
Inside of our planet known as mantle is made of rather fewer rock types than what we get on the surface, and likewise for the minerals that constitute them. Here, is a microscope image of one of those rocks called harzburgite which is made of just olivine also known as peridot in gem community (green-blue-pink) and orthopyroxene (orange).
Under the microscope this very thin slice of rock refracts the light what gives these minerals spectacular colours when the light is polarized to help with mineral identification (top image). Whereas, when the light is not polarized, the minerals are left looking plain, which is helpful in identifying the texture of the rock (bottom image).
Image taken with the petrographic microscope.
#geology#olivine#peridot#pyroxene#harzburgite#thin section#minerals#microscope#microscopic#gem#earth#colorful#mantle#world#petrography#art#abstract#studyblr#student#university#earth science#science#geoscience#original photography#photography#lensblr#drrock
40 notes
·
View notes
Photo



Websterite from Norway.
This one is composed of Pyrope rich Garnet, green Clinopyroxe Diopside, and the Orthopyroxene. I’ve been looking for one of these for the last 12 years. I saw a sample back in my geology classes and since then I’ve been looking. This one is missing the purple garnets that the other specimen had along side the red, but it’s more colors than the other’s I’ve seen!
249 notes
·
View notes
Text


This is a rock called a xenolith. The term xenolith is applied to any rock that does not match the composition of the rock it was found in (called the "country rock" in this situation). Specifically, this sample is a dunite xenolith encased in Hawaiian tholeiitic lava. I collected it at a site on the 1800-1801 flow of Hualālai.
All of the green you see in this rock is the mineral olivine. The black specs may be a number of things - unfortunately, they are too small for me to accurately identify myself. However, I recently studied the mineralogy of another dunite xenolith I collected at the same site.

Beautiful to look at, yes? Aside from the small section in the upper left and some amphibole found while using specialized equipment to search for mineral elements, nearly everything in this sample is olivine. The black spots are an oxide mineral called magnesiochromite, which is opaque.
That upper left section there is part of the tholeiitic crust I mentioned earlier. It looks like this when you look more closely (though this photo is not phenominal)

The colored spots are olivine, while the long monochromatic streaks are a mineral called plagioclase feldspar.
Dunites are part of the peridotite group of rocks. These are named depending on the ratio of the minerals olivine, clinopyroxene, and orthopyroxene. Unusually, I found no pyroxenes at all in my rock sample. Having less than 10% pyroxenes between the three minerals classifies this rock as a dunite.
Although these particular samples likely formed from minerals settling to the bottom of a magma chamber, peridotite is what the mantle is made of! That's right, the mantle is green. Your textbooks lied to you.
#from the desk of anachron#any questions?#i'm in a geology infodumping mood today#geology#mineralogy
17 notes
·
View notes