#m7G modification
Explore tagged Tumblr posts
Text
youtube
#Machine learning#colorectal cancer#prognostic signature#epitranscriptomics#m6A modification#m1A modification#m5C modification#m7G modification#bioinformatics#survival prediction#cancer biomarkers#precision oncology#AI in healthcare#omics analysis#feature selection#deep learning#big data#genomics#risk stratification#personalized medicine.#Youtube
0 notes
Text
Amazon MSK Express Brokers For Fast Scaling And Throughput
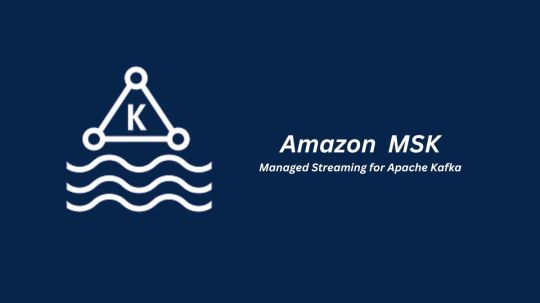
Express brokers for Amazon MSK are now available to provide your Kafka clusters with high throughput and quicker scaling.
Express brokers, a new broker type for Amazon Managed Streaming for Apache Kafka (Amazon MSK), are now generally available. Compared to standard brokers running Apache Kafka, it is intended to provide up to three times the throughput per broker, scale up to 20 times faster, and cut recovery time by 90%. Express brokers support Kafka APIs, are preconfigured with Kafka best practices by default, and offer the same low latency performance that Amazon MSK clients are accustomed to, allowing them to keep using their current client applications without any modifications.
When utilizing Amazon MSK provided clusters, express brokers offer enhanced compute and storage elasticity for Kafka applications. Scalable and highly available Apache Kafka-based systems may be developed and run more easily with the help of Amazon MSK, a fully managed AWS service.
Let’s examine some of the main characteristics and advantages of Express brokers in more detail:
Easy operations and hands-free storage management: By offering limitless storage without preprovisioning, express brokers eliminate disk-related bottlenecks. With just ingress and egress throughput divided by the suggested per-broker throughput, cluster sizing is easier. By removing a possible source of failure, this improves resilience and streamlines cluster administration by doing away with the requirement for proactive disk capacity monitoring and scaling.
Reduced brokers with throughputs of up to three times each Smaller clusters can handle the same demand thanks to higher throughput per broker. While m7g.16xl standard brokers may comfortably handle 154 MBps ingress, their throughput must account for client traffic and background processes. During cluster events, m7g.16xl size instances may securely handle up to 500 MBps ingress without sacrificing availability or performance because to express brokers’ opinionated settings and resource isolation.
20 times faster scaling and higher utilization: By reducing data transfer, Express brokers may scale up to 20 times faster than Standard brokers. This makes cluster resizing faster and more dependable. Over-provisioning in anticipation of traffic surges is no longer necessary because you can add brokers in a matter of minutes and keep an eye on each broker’s ingress throughput capacity.
Improved resilience and 90% quicker recovery: Express brokers are made for mission-critical applications that need to be highly resilient. Best-practice defaults, such as 3-way replication (RF=3), are preconfigured in them to minimize misconfiguration-related failures. In addition, compared to regular Apache Kafka brokers, express brokers recover fromtemporary failures 90% faster. Capacity planning is made easier by express brokers’ recovery and rebalancing, which consume relatively little cluster resources. When right-sizing clusters, this removes the possibility of higher resource consumption and the requirement for ongoing monitoring.
Express brokers are the ideal option for all Kafka workloads because they reduce expenses, offer greater resilience, and have fewer operational overheads. Amazon MSK Serverless is an option if you would rather use Kafka without having any control over its configuration, capacity, or scalability. This gives you a completely abstracted Apache Kafka experience that scales automatically, does not require infrastructure administration, and charges you on a pay-per-use basis without requiring you to minimize resource usage.
How to begin using Amazon MSK Express brokers
You can use Amazon MSK’s Sizing and Pricing worksheet to get started with Express brokers. This worksheet provides you with an approximate monthly cost estimate and assists you in determining the cluster size required to support your workload.
The main determinant of your cluster’s size is the throughput demands of your workload. To determine the size and number of brokers your cluster will require, you should also take into account other variables like the amount of connections and the partition. For instance, you can use three express.m7g.large brokers to meet your throughput requirements if your streaming application requires 30 MBps of data ingress (write) and 80 MBps of data egress (read) capacity (assuming the partition count for your workload is within the maximum number of partitions that Amazon MSK recommends for a m7g.large instance).
The following table shows the maximum entrance, egress, and partition counts per instance size that are recommended for secure and long-term operations. The Amazon MSK Developer Guide’s Best Practices section has more information on these suggestions.
Instance size Ingress (MBps) Egress (MBps)
express.m7g.large 15.6 31.2
express.m7g.4xlarge 124.9 249.8
express.m7g.16xlarge 500.0 1000.0
Create an Amazon MSK provisioned cluster using the CreateCluster API or the AWS Management Console after determining the quantity and size of Express brokers required for your workload.
Choose Express brokers under Broker type when creating a new cluster in the Amazon MSK panel. Next, choose the amount of computational capacity you wish to allocate to the broker. As shown in the screenshot, Graviton-based systems running Apache Kafka 3.6.0 can be used for Express brokers. Express brokers do not require storage to be pre-provisioned.
Some of these configurations can also be altered to further optimize your clusters’ performance to your own tastes.
The AWS CLI create-cluster command creates an MSK cluster.
brokernodegroupinfo.JSON. You specify three subnets for Amazon MSK to distribute broker nodes in JSON.
Once the cluster has been created, you may use the bootstrap connection string to connect your clients to the cluster endpoints.
With Express brokers, you may scale vertically (by changing the instance size) or horizontally (by adding brokers). Vertical scaling doubles throughput without requiring partition redistribution. Horizontal scalability lets you add brokers in groups of three and establish extra partitions, but new brokers handling traffic require partition reassignment.
One of Express Brokers’ key advantages is its ability to add brokers and rebalance partitions in a matter of minutes. Rebalancing partitions after adding Standard brokers may take hours. The graph below shows how long it takes to rebalance partitions when three Express brokers are added to a cluster and 2000 partitions are redistributed to each of the extra brokers.
As shown, reassigning partitions and using the new brokers’ capacity takes about 10 minutes. It performed the same experiment on a similar cluster composed of Standard brokers, and partition reassignment took place over a 24-hour period.
Things to consider
There are a few things you should know about Express brokers:
Data migration: Amazon MSK Replicator moves data from your Kafka or MSK cluster to an Express broker cluster. and metadata from your cluster to a new cluster.
Monitoring: Use Amazon CloudWatch metrics to monitor your Express broker cluster at the broker level and within. Additionally, Prometheus may be used to disclose metrics and enable open monitoring with the JMX Exporter and Node Exporter.
Security: By integrating with AWS Key Management Service (AWS KMS), Amazon MSK provides transparent server-side encryption for the storage in Express brokers, just like other broker types. You can give Amazon MSK the AWS KMS key to encrypt your data when you set up an MSK cluster with Express brokers. it’s at rest. If you do not provide a KMS key, Amazon MSK creates an AWS managed key and uses it on your behalf.
Now available
The Express broker type is currently offered by the following regions: Europe (Frankfurt), Europe (Ireland), Europe (Stockholm), Asia Pacific (Singapore), Asia Pacific (Sydney), Asia Pacific (Tokyo), US East (Ohio), US East (N. Virginia), and US West (Oregon).
The size and resolution of Apache Kafka broker instances (invoiced at one-second resolution) for Express brokers determine the hourly charge. The number of active brokers in your MSK clusters also determines the hourly charge. Additionally, data written to an Express broker incurs a fee per gigabyte (GB).
Read more on govindhtech.com
#AmazonMSK#MSKExpressBrokers#MSK#AWS#MSKcluster#AWSservice#Cloudcomputing#Expressbrokers#News#Technews#Technology#Technologynews#Technologytrends#govindhtech
0 notes
Text
Correction: M7G modification of FTH1 and pri-miR-26a regulates ferroptosis and chemotherapy resistance in osteosarcoma
http://dlvr.it/T3PKVz
0 notes
Text
The Bacillus subtilis ywbD gene encodes RlmQ, the 23S rRNA methyltransferase forming m7G2574 in the A-site of the peptidyl transferase center. [Letter to the Editor]
Ribosomal RNA contains many posttranscriptionally modified nucleosides, particularly in the functional parts of the ribosome. The distribution of these modifications varies from one organism to another. In Bacillus subtilis , the model organism for Gram-positive bacteria, mass spectrometry experiments revealed the presence of 7-methylguanosine (m7G) at position 2574 of the 23S rRNA, which lies in the A-site of the peptidyl transferase center of the large ribosomal subunit. Testing several m7G methyltransferase candidates allowed to identify the RlmQ enzyme, encoded by the ywbD open reading frame, as the MTase responsible for this modification. The enzyme methylates free RNA and not ribosomal 50S or 70S particles, suggesting that modification occurs in the early steps of ribosome biogenesis http://rnajournal.cshlp.org/cgi/content/short/rna.079853.123v1?rss=1&utm_source=dlvr.it&utm_medium=tumblr
0 notes
Link
mRNA vaccine development for COVID-19
Optimization of mRNA vaccine design
Typical vaccine development using live-attenuated or inactivated virus or a pseudovirus system involves tedious and time-consuming steps and has become a bottleneck for responding to an epidemic or pandemic caused by newly emerging viruses. As described above, mRNA vaccines possess distinctive advantages of rapid development and versatility as exemplified by the swift development of multiple COVID-19 mRNA vaccines. More importantly, recent preliminary data from clinical trials have shown that two licensed mRNA vaccines, mRNA-1273 and BNT162b, have higher protective efficacy than ChAdOx1 vaccine developed using a chimpanzee adenovirus (~95% vs. ~70%) 4, 41.
However, some intrinsic features of mRNA molecules demand special strategies to guarantee the stability, efficacy and safety of mRNA vaccines. First, mRNA are intrinsically unstable and prone to degradation due to the omnipresence of RNases in the serum and plasma 42. Second, the cellular machinery recognizes exogenous RNA molecules as immunological mimic of viral infection, which results in an immediate immune response 43. Thus, it is a prerequisite for the design of mRNA vaccines to maximize the stability of RNA and translation efficiency and avoid the innate immune response by host cells 44, 45. Below we will discuss the major strategies used in designing mRNA vaccines, including 5'-capping, nucleoside modification, codon optimization, and efficient delivery of mRNA molecules with nanoparticles (Table (Table22).
5'-capping of mRNA vaccines
Endogenous mRNA molecules undergo post-transcriptional modifications, including 5'-capping and polyadenylation, for the stability of mRNA and efficient translation. Naturally, 7-methylguanosine cap (m7G) is added to the first nucleotide of a mRNA chain via 5' to 5' linkage. The 2'-OH of the ribose of the first nucleotide is further methylated to form m7GpppNm, also known as cap1. 5'-capping is critical for protecting mRNA from exonuclease activity, facilitating pre-mRNA splicing, and serving as the binding site for eIF4F, the heterodimeric translation initiation complex 46-49. Recent studies have indicated the 5'-cap structure as a major determinant by which the host can discriminate between self vs. non-self mRNA molecules 50-53. A m7GpppNm cap was added to the 5'-end of the majority of the mRNA vaccines reported thus far during their IVT 54-57.
Optimization of 5'- and 3'-untranslated regions and the length of polyadenylation tail
Regulatory elements in the 5′-untranslated region (UTR) 58 and the length of 3′-UTR 59 increase protein translation. In addition, the polyadenylation (polyA) tail stabilizes mRNA and increases protein translation. Several recent reports have shown that the length of polyA tail is closely associated with the translation efficiency 60. However, the information on 5'- and 3'-UTRs and the nature of polyA signal sequence remains proprietary and undisclosed for the seven reported mRNA vaccines.
Nucleoside modification during IVT
Kariko, et al., demonstrated that RNA recognition by Toll-like receptors (TLRs) is suppressed via modification of the nucleosides in mRNA molecules 61, 62. Incorporating m5C, m6A, m5U, s2U, or pseudouridine into mRNA molecules abrogates the immune response by evading the activation of TLR-3, -7, and -8 61. For all the seven reported vaccines, pseudouridine was incorporated into the mRNA vaccines in the place of uridine. In addition, the substitution with pseudouridine, m6A, and s2U in RNA molecules suppresses the degradation of RNA by RNase L 63. Thus, the nucleoside modifications not only enhance the stability of RNA but also reduce the innate immune response.
Purification of IVT
The contaminating impurities during IVT can massively affect the safety of mRNA vaccines once they are introduced to human cells. Even residual amounts of double-stranded RNA and DNA-RNA hybrid molecules can trigger the innate immune response as they can be recognized by the cellular sensors pattern recognition receptors. Various purification techniques have been used to remove residual impurities from IVT reactions for all the seven mRNA vaccines currently on clinical trials. A previous study indicates that the purification of mRNA reduces the expression of type I interferon and increases the protein translation 64. As summarized in Table Table2,2, various purification techniques such as Oligo dT column, LiCl precipitation, and silicone column have been employed to remove contaminants from in vitro synthesized mRNA 45.
Codon optimization
Several parameters have been considered for the codon optimization, which affects the translation efficiency, protein folding, and mRNA abundance. One example is that the GC content in the sequence. Although GC-rich sequences may be problematic for the secondary structure formation of mRNA, the translation efficiency of a GC-rich sequence can be 100-fold higher than that of a GC-poor sequence 65. The translation elongation rate highly depends on the availability of the cognate tRNA species and the optimization of the codon usage to avoid sequences that match rare tRNA species and incorporate sequences that match more abundant tRNA species 66. Moreover, the codon optimization is essential for the mRNA stability as the codon-dependent translation elongation rate has been implicated as a major determinant of the mRNA stability 67. Mechanistically, reduced translational elongation of mRNA with suboptimal codons results in the recruitment of the DEAD-box RNA helicase, Dhh1p, which triggers mRNA decay 68. Two additional codon optimization methods involve the use of the codons with human bias and the maximum adaptation index 69, 70. Other bioinformatics approaches can be explored to further enhance the stability of mRNA, e.g., via design of the secondary structures and prediction of the expression level based on deep learning 71, 72.
Designing platform and target immunogen for the seven mRNA candidate vaccines
Each of the seven mRNA candidate vaccines was synthesized in vitro from a DNA template encoding either the full-length S protein or RBD of SARS-CoV-2 using bacteriophage T7 RNA polymerase. mRNA-1273, CVnCoV, LUNAR-CoV19, and LNP-nCoVsaRNA mRNA vaccines used the template encoding the full-length S protein with 2P substitutions at K986 and V987 positions to produce the stable pre-fusion form of S protein 73. Pfizer/BioNTech have developed two immunogens, the RBD (BNT162b1) and the full-length S protein (BNT162b2). BNT162b2 has been shown to be safer than BNT162b1, especially in older adults in a preliminary clinical trial, and thus was chosen for a phase 3 clinical trial 74. ARCoV vaccines are based on the RBD of SARS-CoV-2. Whereas the sequences of the 5'- and 3'-UTR of the mRNA templates were not revealed in the literature, the 3'-UTR of BNT162b mRNA vaccine derived empirically by screening naturally occurring 3'-UTRs for the highest RNA stability 75. On the other hand, CVnCoV and LNP-nCoVsaRNA were built on the saRNA platform containing a self-replicating replicon of Trinidad donkey Venezuelan equine encephalitis virus (VEEV). The viral protein-encoding gene of the replicon is replaced with a modified S protein-encoding gene of SARS-CoV-2 with two proline mutations in the S2 subunit, K986P and V987P 54, 55. Consistent with the notion that saRNA vaccines can self-amplify after delivery into host cells, the dose used for vaccination was one to two magnitude lower than conventional mRNA vaccines. As shown in Table Table2,2, the dosage range for CVnCoV and LNP-nCoVsaRNA was 2-12 μg and 0.01-10 μg, respectively. In comparison, the typical dose range for the conventional mRNA vaccines was 30-100 μg.
Packaging mRNA vaccines with lipid nanoparticle (LNPs)
An early study has shown that the transfection efficiency of naked mRNAs is nearly two orders of magnitude lower than that of mRNA bound to lipofectin formulation 27. The lipofectin-based carriers effectively help mRNA delivery into target cells and protect mRNA from RNase 36, 76. The formulation of liposome-based transfection reagents containing cationic lipids has remarkably been improved in recent years 77. In particular, LNPs, composed of proprietary components including positively charged lipids, cationic polypeptides, polymers, micelles or dendrimers, have been widely used for in vivo RNA delivery 78, 79. LNPs encapsulate mRNA and assemble it into the stable lipid bilayers, which are then ingested by cells through a variety of endocytosis pathways. Below is the information for packaging of mRNA vaccines with various LNPs.
1. mRNA-1273: It was loaded into two proprietary cationic LNPs, WO2017070626 and WO2018115527. Although the exact formulation is not known, the composition of the LNPs was described as follows, SM-102, polyethylene glycol-2000-dimyristoyl glycerol (PEG2000-DMG), cholesterol, and 1,2-distearoyl-sn-glycero-3-phosphocholine (DSPC) 80.
2. BNT162b mRNA: It was encapsulated by patented LNPs with improved efficiency of the mRNA delivery according to its clinical trial report (#NCT04368728) 81, 82. The LNPs are composed of ionizable amino lipid, phospholipid, cholesterol and a PEGylated lipid prepared at a ratio of 50:10:38.5:1.5 mol/mol 82, 83. It is interesting to note that BTN162b and mRNA-1273 vaccines are suggested to be shipped and stored at -80˚C and -20°C, respectively 80, 82.
3. CVnCoV: It was formulated with a proprietary LNP, referred to as RNActive® technology platform. The LNP consists of four lipid components: cholesterol, DSPC, PEGylated lipid, and a cationic lipid, however the detailed formulation information was not disclosed. CVnCoV remains stable for at least three months when it is stored at 5℃ as suggested by its manufacturer. Moreover, CVnCoV can be stored at room temperature as a ready-to-use the vaccine for up to 24 hours 84, 85.
4. ARCoV: It was encapsulated in LNPs of a proprietary composition using a preformed vesicle method and found thermostable at different temperatures, including 4°C, 25°C, and 37°C for up to one week 86.
5. ARCT-021: Currently undergoing phase 1/2 clinical trials, it combines two technologies, i.e., saRNA STARR™ and LUNAR® lipid-mediated delivery method. It was designed to enhance and extend antigen expression, enabling vaccination at lower doses 87. In addition, LUNAR® lipids are pH-sensitive and biodegradable, causing minimal lipid accumulation in cells after multiple dosing 87
6. LNP-nCoVsaRNA: Developed by Imperial College London using cationic liposome as the carrier, it has just entered phase 1 clinical trial 55.
4. ARCoV: It was encapsulated in LNPs of a proprietary composition using a preformed vesicle method and found thermostable at different temperatures, including 4°C, 25°C, and 37°C for up to one week 86.
5. ARCT-021: Currently undergoing phase 1/2 clinical trials, it combines two technologies, i.e., saRNA STARR™ and LUNAR® lipid-mediated delivery method. It was designed to enhance and extend antigen expression, enabling vaccination at lower doses 87. In addition, LUNAR® lipids are pH-sensitive and biodegradable, causing minimal lipid accumulation in cells after multiple dosing 87
6. LNP-nCoVsaRNA: Developed by Imperial College London using cationic liposome as the carrier, it has just entered phase 1 clinical trial 55.
#covid-19#covid-19 vaccine#mRNA vaccine#epigenetics#genetics#family medicine#RNA#RNA binding proteins#prion-like domains#pseudouridine#print this off later#GC-rich sequences#RNA-binding protein#RNA-binding proteins
29 notes
·
View notes
Text
AlkAniline-Seq: profiling of m7G and m3C RNA modifications at single nucleotide resolution.
Pubmed: http://dlvr.it/QpyYyf
0 notes
Text
Identification of m7G regulator-mediated #RNA methylation modification patterns and related immune microenvironment regulation characteristics in heart failure
CONCLUSIONS: Our study discovered for the first time that m⁷G #RNA modification and immune microenvironment are closely correlated in HF development. A five-gene m⁷G regulator diagnostic signature for HF (NUDT16, NUDT4, CYFIP1, LARP1, and DCP2) and three m⁷G methylation-associated hub genes (UQCRC1, NDUFB6, and NDUFA13) were identified, providing new insights into the underlying mechanisms and effective treatments of HF. https://pubmed.ncbi.nlm.nih.gov/36782329/?utm_source=dlvr.it&utm_medium=tumblr&utm_campaign=None&utm_content=1RYYbE7j9SUSBe_aHniaI_J1MQIFIBbfLuFxoWdLNMNDzVVIWF&fc=None&ff=20230216100559&v=2.17.9.post6%2086293ac
0 notes
Text
THRONE – a new approach for accurate prediction of human #RNA N7-methylguanosine sites
N7-methylguanosine (m7G) is an essential, ubiquitous, and positively charged modification at the 5′ cap of eukaryotic mRNA, modulating its export, translation, and splicing processes. Although several machine learning (ML)-based computational predictors for m7G have been developed, all utilized specific computational framework. A team led by researchers at Mahidol University explored four different computational frameworks and ... https://www.rna-seqblog.com/throne-a-new-approach-for-accurate-prediction-of-human-rna-n7-methylguanosine-sites/?utm_source=dlvr.it&utm_medium=tumblr
0 notes
Text
Ontogenic #mRNA expression of #RNA modification writers, erasers, and readers in mouse liver
by Liming Chen, Pei Wang, Raman Bahal, José E. Manautou, Xiao-bo Zhong #RNA modifications are recently emerged epigenetic modifications. These diverse #RNA modifications have been shown to regulate multiple biological processes, including development. #RNA modifications are dynamically controlled by the “writers, erasers, and readers”, where #RNA modifying proteins are able to add, remove, and recognize specific chemical modification groups on #RNAs. However, little is known about the ontogenic expression of these #RNA modifying proteins in various organs, such as liver. In the present study, the hepatic #mRNA expression of selected #RNA modifying proteins involve in m6A, m1A, m5C, hm5C, m7G, and Ψ modifications was analyzed using the #RNA-seq technique. Liver samples were collected from male C57BL/6 mice at several ages from prenatal through neonatal, infant, child to young adult. Results showed that most of the #RNA modifying proteins were highly expressed in prenatal mouse liver with a dramatic drop at birth. After birth, most of the #RNA modifying proteins showed a downregulation trend during liver maturation. Moreover, the #RNA modifying proteins that belong to the same enzyme family were expressed at different abundances at the same ages in mouse liver. In conclusion, this study unveils that the #mRNA expression of #RNA modifying proteins follows specific ontogenic expression patterns in mice liver during maturation. These data indicated that the changes in expression of #RNA modifying proteins might have a potential role to regulate gene expression in liver through alteration of #RNA modification status. http://bit.ly/2ZF24C5
0 notes