#bacterial conjugation
Explore tagged Tumblr posts
Text

TSRNOSS, p 588.
#high altitude populations#bacterial conjugation#oligotrophic lakes#detergents#deoxycholic acid#deoxyribose#disinfectants#potassium permanganate#oxidation of ferrous iron#electroconvulsive shock#ozone#xenobiotics#aromatic ring#biodegradation#trophic level#mercury#volcanoes#brain#metabolic rate#gas equation#gas bladder#carbon monoxide#spores#oligotrophs#ultraviolet light#high altitude#mutation
0 notes
Text

PANDEMICS- Hostile Power Takeover? Learnings on Urban & Domestic Warfare, “Disease: Bacteria Part 1, Fundamental Considerations”:
Let’s say the hostile power is more technologically advanced & half robot/half machine or full machine, so seemingly unkillable. Organic beings are very vulnerable to having disease used as a weapon against them.
Disease can be a major benefit to this type of hostile power & it would be an incredibly powerful weapon. This allows the destruction of an organic-based domestic population & it can also allow the harvesting of resources to build new bodies and/or reuse of the entire body depending on the disease process.
There are many insidious ways diseases develop & spread. This process DOES NOT just occur in a laboratory. Remember that there are many different “groupings” of entities we refer to as pathogens or things with the ability to cause disease.
Bacteria are an important one. Bacteria & other pathogens can reproduce by multiple means. Here I’m going to speak about bacteria with the capacity to do Bacterial conjugation. This involves passing characteristic between two different bacteria similar to how sexual reproduction can pass on characteristics. This is overall an important conversation because a lot of the most complex & common life forms in our daily lives also spread these characteristics through similar principles through sexual reproduction.
> A lot of bacteria to our awareness are able to pass on characteristics. Bacterial DNA contains the “instructions”/“resources” for bacteria to either have or not have characteristics.
-Bacterial conjugation for example allows one bacteria to attach to a second bacteria & send resources to the second bacteria. After this process, the second bacteria is able to transform and display the characteristics transferred to it. Example: Bacteria A can change colors like a chameleon. Bacteria B cannot change color. Once Bacteria A attaches to Bacteria B and they are compatible, Bacteria A passes on resources to Bacteria B. Bacteria B then acquires the ability to change color. Bacteria B now can change color & has attainted the same advantage as originally only bacteria A had. Now Bacteria A and Bacteria B can change color like a chameleon.
- The other way characteristics form & occur in a bacterial population is through mutation. If a bacteria’s DNA is altered or mutates then it can produce a bacteria with new traits & characteristics. Radiation for example, like from X-rays, often causes mutations. Sometimes mutations do “nothing” we can really perceive with our eyes. But overtime, they will eventually create large changes and can produce huge benefits for bacteria. For example: A bacteria could have always have been wiped out from nuclear weapons then overtime from mutations it can acquire the ability to survive living inside an area with nuclear radiation.
-This is a very important concept to fully understand so that you can become cognizant of how insidious this process is when discussing what bioterrorism in the modern world can look like. Pandemics are not caused just from mysterious lab leaks. The practices we do everyday are still contributing to the next pandemic occurring.
-This also gives everyone a better understanding of how MRSA or an antibiotic resistant bacteria really was “made” inside our hospitals.
> Bacteria can possibly have random or genetically engineered characteristics.
-If there are 100 random bacteria on an isolated surface that formed there naturally, some will have favorable characteristics to cause severe disease. But, some bacteria will not have those characteristics to cause severe disease. The bacteria lacking these deadly characteristics, but are still part of the same family of bacteria, would be considered weaker pathogens (weaker pathogen meaning they would cause less severe disease in organic beings).
-**But it is important to remember, If someone purposefully put bacteria down on a surface there is a chance it will not be a random distribution in strength of bacteria & they will mostly all be bacteria with strong characteristics. That group would probably be closer to 100 out of 100 of the bacteria carrying the deadly characteristic.**
>There are 2 main basic premises (which can be further subdivided and added onto when discussing what makes pathogens strong, but for now I’m discussing a more fundamentals explanation) we consider when determining bacterial pathogen strength: number of bacteria & the amount of deadly/harmful characteristics each bacteria possess.
-Reducing the overall number of bacteria in a group of random bacteria does not always mean you make a pathogen less strong. (Example: Purposefully killing 50 bacteria out of 100 and now there are only 50 bacteria in the group.)
If you destroy many of the weaker bacteria & only leave strong bacteria to reproduce, pathogens overtime can get stronger & more deadly. So, by destroying only the weaker bacteria in a group of bacteria, you slowly make pathogens stronger through this natural process & it doesn’t have to occur inside of a laboratory. To make a bacterial pathogen less strong by focusing on decreasing the overall number of those bacteria that exist in our world, you would also have to consider how many of each strength you eliminate. This is because we currently we do not use practices that wipe out groups of bacteria 100%, so we must consider these two elements together instead of separate when evaluating pathogen strength. Example: Lets say there are 100 bacteria and you wipe out 90. Bacteria A can cause humans to be paralyzed. Bacteria B cannot paralyze humans. Out of the 10 bacteria still alive, if all 10 are Bacteria A then you have eliminated the chance people would be infected with the less severe version of the disease, with Bacteria B. In the long term Bacteria A now has a strong chance to reproduce & when Bacteria A infects people it would then cause paralysis in everyone & the population could collapse. In another scenario, consider if you wiped out 90 bacteria out of 100, but you did it purposefully. Out of the 10 bacteria left, 9 were Bacteria that were Bacteria B & couldn’t cause paralysis. The last 1 out of the 10 left was Bacteria A. Then when those 10 bacteria reproduced it effectively helps “dilute” this negative characteristic in this bacterial family. Based off randomness & probability, when there this group reproduces to the size of 20 bacteria only approximately 2 of them may carry Bacteria A’s paralytic characteristic & 18 will carry bacteria B’s characteristic that does not cause paralysis. So, even though we can’t stop the bacteria number from growing, since we mindfully intervened we can still divert the trajectory of the pathogen from becoming a pathogen with the ability to become “pandemic level” and/or very very harmful.
>Two ways pathogens can get weaker is by lowering the amount of bacteria in the world & by lowering its severe disease characteristics, but this these two categories have an important interplay.
-This is an oversimplified explanation of how disease spreads & evolves, but the fundamental principles are VERY important to the overall understanding of what’s occurring. Imagine a group of bacteria you count has 100 total bacteria. 50 of them carry a gene to cause paralysis in humans & 50 do not carry this gene. When 100 people come in contact with the 50/50 bacteria distribution and get sick only 50 out of 100 of the people get paralyzed. This allows the other 50 people time to work on vaccinations & interventions to stop everyone from eventually being paralyzed.
-But, if you kill the 50 out of the 100 bacteria that do not carry the gene for paralysis then your bacteria group went from 100 to a total of 50 in size. In the short term the spread of the disease is likely to go down, as it is less likely people will randomly spread 50 objects instead of 100. BUT, those 50 bacteria with the gene to cause paralysis will only reproduce with other bacteria that also have that gene. So this bacteria, since you wiped out the 50 that don’t cause paralysis, now ALL cause paralysis & anyone who comes in contact with this bacteria strain will get paralyzed. So eventually with time the group of 50 bacteria will reproduce to 100 & spread at the same rate as they were originally, but now they cause more harm to people.
>When you unknowingly touch a colony of bacteria on an object or life form, you pick up a random sample of random “strength” of bacteria.
>****PLEASE READ: you can ALSO pickup a sample of bacteria that is all “strong bacteria” but this is NOT usually a natural occurrence you will see & is suggestive someone or something altered the bacteria and purposefully put those bacteria there. A group of bacteria that looks like it formed organically vs one that was purposefully placed there can be differentiated with taking samples of surfaces and people & counting how many strong bacteria vs weak bacteria there are, but we as a population do not regularly test for this in this way. Due to this I’m going to speak with the viewpoint of natural bacteria groups that have a gradient of “strengths”. In an ideal world we would identity groups of bacteria that have gradients of strength of bacteria vs groups of all similar strength, as interventions to stop them from becoming strong pathogens work DIFFERENTLY.)
>After you touch those bacteria they attempt to multiply and stay alive on you. Then if you touch other things they can be placed on another surface or thing. Sometimes they are placed on other surfaces in an environment or you touch your body & they are placed closer to an entrance to the inside or your body & then they are able to enter your body.
-This process will cause one of the following to occur: bacteria will stay in the area you touched & colonize it, they will die when attempting to enter the body, the bacteria will give you a disease , or in some cases the bacteria will live symbiotically inside you & help your body. If a bacteria lives symbiotically with you & does not cause harm then we do not refer to that as a pathogen, but rather just as a bacteria.
>Anytime you wipe out a group of bacteria by taking out 100% it causes that pathogen to get weaker overall, but the issue is that we do not do interventions that wipe out 100%.
-Currently anytime you clean an object in the hospital with a sanitizing wipe, you always kill less than 100% of the bacteria. This leaves behind a certain % of bacteria & they will be the strongest of that group of bacteria, because they were able to live even though you applied a cleaning product on them. This means the strongest bacteria left, even though there are less after cleaning, are now reproducing over and over again & getting stronger.
-So, when there is an environment with a large amount of bacteria variability (so all these new patients with new exposures to new bacteria that travel and touch things all the time), with shared equipment, with not 100% effective methods to destroy pathogens, & this long list of variables, we slowly produce very strong & deadly pathogens inside of hospitals.
-IF someone purposefully puts deadly bacteria ontop of a surface inside a hospital and it is a group of 100 strong & identical or cloned bacteria with no difference in genetics then wiping them out through imperfect cleaning will overall reduce pathogen deadliness. This is because there are no “stronger” pathogens vs “weaker” pathogens. They are all the same strength in this example and therefore will always get weaker when you reduce their number because they won’t reproduce to be more deadly.
>People often think when people are trying to cause them harm that would only occur when someone makes a pathogen in a lab & then deceptively goes and places some near you. This is not accurate.
-With knowing this do you see how for a hostile power there is actually LESS incentive to going through with all that work & instead a hostile power can abuse the system to cause harm? If you expect biological warfare to ONLY come out of a lab, this means you would be looking for the wrong patterns of behavior & pathogens will spiral out of control.
A lot of practices we currently use now unfortunately heavily contribute to this process that causes pathogens to get stronger.
#pathogens#doctor#medicine#physician assistant#nursing#nurse#registered nurse#evolution#disease#bacteria#np#nurse practitioner#pa#md#rn#meds#pharmacy
433 notes
·
View notes
Text
Bacterial cells may exchange plasmids via their sex pili, in a process known as bacterial conjugation
this line from my textbook has me giggling icl like they're conjugating w their sex pili !!
13 notes
·
View notes
Text
Ea, Our Second Chance (10c)
10c. Eucytobionta (part 3/3, biotechnology)
(Index) (< 10b. Eucytobionta, unicellular diversity > 11. The early days)
(original link)
« For the future of war: study bacteria. Information is their key. Taking down antibiotic defence systems has involved them in every kind of infiltration, net-communicated adaptivity, cryptographic subtlety, plastic modularization, and synergistic coalition... Bacterial sex is tactical, continuous with making war. » – Nick Land, Meltdown, 1994
« Remember that the so-called laws of Nature are always descriptive, never prescriptive... All that unfolds before us in the natural world, it's up to us to shape it in the form that serves best that which thinks and feels. Down with the natural; up with the good. » – architect Evgeny Kantorovich, The Inhuman Humane
« During cellular division, in both one-celled and multi-celled organisms, it's possible to identify a "mother" and a "daughter" cell by the fact that the latter receives only a copy of the protonucleus. Only once the division is complete does it divide again to generate the new paranuclei. Presumably this is a measure to limit the transmission of dangerous mutations (see Appendix B), which are bound to occur in the mother's paranuclei because of their massive activity of PNA replication, but not in the protonucleus, which bears only one, relatively inert copy of each gene.
[...] During paranuclear genesis, not all genes are multiplied equally. The exact amount of copies that are produced of each gene seems to be the most important tool of developmental regulation, especially in multicellular organisms, wherein different cells must be specialized for different tasks (see Appendix D, 23-31). [...] We believe that identifying the genes that regulate paranuclear gene multiplication would allow significant directed alteration to the development of economically-significant cultured organisms, for example by directly increasing the edible storage tissue of a new strand of nopal trees.
[...] It's worth noting that nevertheless a paranucleus contains far fewer mutations than one would expect from its rate of PNA replication (see Appendix D, 37-42), which suggests extremely strong safeguards against replication errors. [...] Given the multi-terabyte capacity of each paranucleus, we suggest that synthetic PNA within a paranuclear environment could be an extremely effective form of micro-storage for digital information.
[...] Sexual recombination uncoupled from reproduction, or conjugation, is common among Ean microbes as it is among Terran ones. Most commonly [...] two partners duplicate their protonucleus by binary fission, and exchange one copy with each other. The diploid nucleus resulting from the fusion of the stationary protonucleus with the one received from the other partner immediately performs meiosis to produce once again haploid protonuclei (see Appendix B ) carrying a mixture of genes from both partners. All such protonuclei but one are dismantled and digested, as are the existing paranuclei. New paranuclei are generated, as described above, by the division of the surviving protonucleus.
Recent studies (see Appendix D, 51-64) suggest that a similar process of quasi-sexual genetic exchange (microconjugation) may occur among the cells of multicellular organisms [...] and that virus- or viroid-like particles may be introduced into the conjugating nuclei (see Appendix D, 65-67). We propose to look into the potential of artificial microconjugation to insert arbitrary PNA sequences into selected target cells. This would allow highly targeted modification of already-developed Ean multicellular organisms, with an extremely broad array of applications: consider splicing metal-sequestering cuprophyte complexes into fast-growing phytoplankton for oceanic mining, or breeding edible Enantiozoa with pre-flipped flesh. »
– internal memo, Penglai-Lifeware, "Three avenues of investigation into cellular xenobiology", circa 160 AL
#speculative biology#speculative evolution#xenobiology#alien life#scifi#my work#ea our second chance
13 notes
·
View notes
Text
This is a computationally designed peptidomimetic that self assembles into a fascinating bilayered capsid-like assembly. Its amazing, and never fails to make an academic sit up and pay attention. Oh and it’s a novel antibiotic too.

What the researchers have basically done is take a short peptide with known anti bacterial properties and engineer it such that it makes a nanocapsule. The really ingenious part of this design is the way the researchers engineered the peptide to have 3-fold symmetry. They used a lysine dipeptide scaffold and then attached copies of the antibacterial peptide as spokes via the lysine amino groups to create this pseudo symmetrical ‘triskelion conjugate’ which is just really cool.

The paper has a lot more info on the design process, but those are the broad strokes. After designing this lil guy the researchers synthesised it and even tested it against some E. coli. The antibacterial action works by rapidly creating a high local concentration of antibacterial peptide on the bacterial cell wall, creating very large hole/pores. Basically because the capsid is composed of an antibiotic, when it bumps into a bacterium it will immediately start ripping the cell wall apart.

Overall, this is just such a cool application of peptide engineering that I can gush about it for ages. The paper is really worth a read and has some beautiful graphics, full MD renders, some lovely electron and atomic force microscopy pics and even a simulated movie of the self-assembly.
References
Kepiro, I. E.; Marzuoli, I.; Hammond, K.; Ba, X.; Lewis, H.; Shaw, M.; Gunnoo, S. B.; De Santis, E.; Łapińska, U.; Pagliara, S.; Holmes, M. A.; Lorenz, C. D.; Hoogenboom, B. W.; Fraternali, F.; Ryadnov, M. G. Engineering Chirally Blind Protein Pseudocapsids into Antibacterial Persisters. ACS Nano 2020, 14 (2), 1609–1622. https://doi.org/10.1021/acsnano.9b06814.
Images adapted from here too
2 notes
·
View notes
Text
In our series of novels, the Ktletaccete originally had pronouns that corresponded to what kind of tail you had. Over the millenia, after several cycles of fascist governments that messed with their genetics with breeding programs and genetic engineering, a group of them developed bimodal sexual dimorphism similar to humans and had similar binary pronouns, but their descendants who populate the generational starship Sunspot undid that and resurrected all the old pronouns but decoupled them from any physical characters and just let people choose them. Everyone aboard the Sunspot is effectively intersex by human standards, naturally hermaphroditic but with sexual maturity suppressed (not a good choice, they’re not perfect).
Anyway, so that's where they're at when they encounter modern day humanity.
It's not as imaginitive as the bacterial colony coded categories that you've suggested, azzandra, but we've kind of realized it's still gender, basically.
Gender doesn't have to be based on sexual traits. It's just any sort of arbitrary category. In language, it's groups of words that all get the same treatment and conjugations of verbs and such. In people, it's broad cultural wide groups of people who feel kinship and familiarity within the group and get stereotypes applied to them from outside the group. And it's gender whether it's based on genitals, tails, bacterial colonies, or personal preference of pronoun.
Whenever I see a post on tumblr suggesting aliens don’t have gender, I always think–‘but what if also the reverse. What if aliens also have some fundamental social construct we don’t’.
Like, they come and meet us and they’re like ‘hey this is an awkward question but what’s your gooblebygark?’
And we’re like what.
‘You know, the… the thing. Your goobledygark. The thing that dictates whether you’re gnarfgnoovles or brubledoopes’
What. What. What the fuck, those words don’t even mean anything??? What are you talking about?
‘Look, your ridiculous human languages don’t seem to have the words for these! But they’re totally a thing, they’re like, fundamental aspects of social life for our species, just… just let us lick you so we can know what verb tense to use when we speak to you.’
What does one thing have to do with the other??? That makes no–
‘UGH, nevermind, you’re totally brubledoopes, I can just tell, I don’t even need to taste your bacterial skin colonies.’
And then another alien overhears and is like ‘holy shit, you can’t stereotype like that, that’s SO NOT COOL’
‘yeaH BUT THEY WON’T LET ME LICK THEM’
142K notes
·
View notes
Text
Protein G Agarose Beads: Reliable Choice for IgG Purification

When it comes to immunoglobulin G (IgG) purification, efficiency, reliability, and consistency are key factors. Whether you are working in research, diagnostics, or therapeutic development, having a dependable purification method ensures the highest level of antibody yield and functionality. One of the most trusted tools in this process is Protein G Agarose Beads. These beads offer superior binding specificity, high recovery rates, and excellent stability, making them an ideal choice for IgG purification across various species and subclasses.
Why Protein G Agarose Beads are Essential for IgG Purification
Protein G is a bacterial cell wall protein that binds specifically to the Fc region of immunoglobulins, particularly IgG. When conjugated to agarose beads, this protein creates a highly effective affinity matrix for purifying IgG from complex biological samples such as serum, ascites fluid, or cell culture supernatants. Here’s why Protein G Agarose Beads stand out:
High Affinity and Specificity
Protein G exhibits strong binding to most mammalian IgG subclasses, offering broader compatibility than alternatives like Protein A. This makes it especially valuable when working with species where Protein A has weaker interactions.
Superior Yield and Recovery
With optimized binding conditions, Protein G Agarose Beads can achieve high IgG recovery rates with minimal sample loss. This ensures that even small-volume samples can be processed efficiently.
Minimal Non-Specific Binding
Compared to other affinity matrices, Protein G Agarose Beads reduce the risk of non-specific protein interactions, leading to higher purity of the isolated IgG. This is particularly important for applications requiring downstream functional assays or therapeutic antibody production.
Robust and Reusable
These beads can withstand multiple rounds of purification cycles with proper regeneration protocols, making them a cost-effective solution for laboratories handling large-scale IgG purification.
Applications of Protein G Agarose Beads
Whether you are engaged in immunological research, therapeutic development, or diagnostic assay preparation, Protein G Agarose Beads can play a critical role in your workflow. Some of the most common applications include:
Monoclonal and Polyclonal Antibody Purification: Ideal for obtaining high-purity IgG from hybridoma culture supernatants or serum samples.
Immunoprecipitation (IP): Used to isolate antigen-antibody complexes for protein interaction studies.
ChIP (Chromatin Immunoprecipitation): Helps in studying protein-DNA interactions crucial for epigenetic research.
Therapeutic Antibody Production: Ensures high-yield purification of antibodies for clinical and biopharmaceutical applications.
How to Use Protein G Agarose Beads for IgG Purification
Using Protein G Agarose Beads in an affinity chromatography workflow is straightforward. Here’s a step-by-step overview:
Equilibration: Pre-wash the beads with a binding buffer (commonly phosphate-buffered saline) to remove storage preservatives.
Binding: Incubate your sample with the beads to allow IgG binding to Protein G.
Washing: Remove unbound proteins using an appropriate wash buffer.
Elution: Use an elution buffer (commonly low pH glycine or another suitable buffer) to release the purified IgG.
Neutralization & Storage: Quickly neutralize the eluted IgG and store it under appropriate conditions for downstream applications.
Choosing the Right Protein G Agarose Beads
When selecting Protein G Agarose Beads, consider factors like bead size, binding capacity, and crosslinking stability. High-quality beads ensure minimal leakage of Protein G, preventing contamination of your purified IgG. Additionally, some commercial products come pre-blocked to reduce non-specific binding further.
For those looking to check over here for high-quality Protein G Agarose Beads, ensuring compatibility with your specific application is crucial. Reliable suppliers provide detailed product specifications, including binding capacity and recommended protocols.
Final Thoughts
In antibody purification, consistency and reliability are paramount. Protein G Agarose Beads offer a highly effective solution for purifying IgG with excellent specificity, yield, and reusability. Whether you’re conducting research or manufacturing therapeutic antibodies, these beads provide a robust and efficient method for achieving high-purity IgG.
If you are looking for the best solutions in IgG purification, click this link here now to explore high-quality Protein G Agarose Beads tailored to your needs.
Original Source: https://lyticsolutions.blogspot.com/2025/02/protein-g-agarose-beads-reliable-choice.html
0 notes
Text
Global Strategies for Meningitis Prevention: Trends in the Meningococcal Vaccine Market
The global meningococcal vaccines market size is expected to reach USD 5.28 billion by 2030, registering a CAGR of 6.0% over the forecast period, according to a new report by Grand View Research, Inc. Increasing prevalence of meningitis is one of the major factors attributed to the market growth. For instance, according to ECDC, it was estimated that in 2017, 3,221 confirmed cases were observed in EU member states, wherein 58% cases were found in the U.K., France, Spain, and Germany. Moreover, a high incidence of meningitis disease is found in the “meningitis belt” of sub-Saharan Africa, and approximately 30,000 cases are reported every year in this region.
In addition, rising FDA approval for meningococcal vaccines is expected to boost the growth. For instance, in April 2020, Sanofi received FDA approval for its MenQuadfi Meningococcal Conjugate Vaccine for the prevention of invasive meningococcal disorders in individuals at least two years old. The increase in approval of novel vaccines is expected to improve meningococcal prevention which in turn, is anticipated to drive the market.
Initiatives undertaken by the government to combat the rising incidence of the disease are anticipated to boost the meningococcal vaccine market growth. For instance, from April 2019, Nimenrix was made available for free as a part of the National Immunization Program (NIP) in Australia for the age group 15 to 19 years. Nimenrix is a quadrivalent meningococcal vaccine that targets serogroups A, C, W & Y.
According to WHO, as of 2021, 24 of the 26 nations in the meningitis belt have introduced mass preventive campaigns targeting the age of 1 to 29 years. Moreover, around 13 nations have undertaken the vaccine in their national routine immunization programs. Such initiatives undertaken by countries having high disease burdens are expected to boost the market growth.
Non-profit organizations entering into strategic initiatives such as partnering, alliances, and others to improve access to new vaccines for vulnerable children are anticipated to drive the market. For instance, in September 2021, WHO and its partners announced the first-ever global strategy to defeat meningitis. This initiative aims to reduce epidemics of bacterial meningitis. Under this strategy, WHO goals to save more than 200,000 lives annually.
The availability of vaccines at lower prices in countries with an increasing prevalence of the meningococcal disease is anticipated to fuel the revenue of the manufacturers and drive the meningococcal vaccine market. For instance, under Meningitis Vaccine Project, MenAfriVac costs less than USD 0.5 per dose.
Meningococcal Vaccines Market Report Highlights
Quadrivalent vaccines dominated for the largest revenue share of 51.1% in 2022 in type segment owing to their ability to target four serogroups and the strong presence of vaccines such as Menveo, Menactra, and Nimenrix
The infants (0-2 years) segment is expected to be the fastest-growing segment over the forecast period. The increasing prevalence of meningitis in the age group 9 months to 11 years contributed to the segment growth
Asia Pacific region is expected to witness the highest CAGR over the forecast period. High unmet needs, rising healthcare expenditure, and a large population base in this region are likely to bolster the market
Meningococcal Vaccines Market Segmentation
Grand View Research has segmented the global meningococcal vaccines market on the basis of type, brand, serotype, age group, sales channel, and region:
Meningococcal Vaccines Type Outlook (Revenue, USD Million, 2018 - 2030)
Bivalent
Quadrivalent
Others
Meningococcal Vaccines Brand Outlook (Revenue, USD Million, 2018 - 2030) (Volume, Number of Doses (In Thousands))
Menactra
Menveo
Nimenrix
Trumenba
Bexsero
Others
Meningococcal Vaccines Age Group Outlook (Revenue, USD Million, 2018 - 2030)
Infants (0 to 2 years)
Children And Adults (2 years & above)
Meningococcal Vaccines Serotype Outlook (Revenue, USD Million, 2018 - 2030) (Volume, Number of Doses (In Thousands))
Serotype A
Serotype B
Serotype C
Serotype W-135
Serotype Y
Meningococcal Vaccines Sales Channel Outlook (Revenue, USD Million, 2018 - 2030)
Private
Public
Meningococcal Vaccines Regional Outlook (Revenue, USD Million, 2018 - 2030)
North America
US
Canada
Europe
Germany
UK.
France
Italy
Spain
Russia
Belarus
Georgia
Moldova
Denmark
Sweden
Norway
Asia Pacific
Japan
China
India
Australia
Singapore
South Korea
Thailand
Vietnam
Malaysia
Indonesia
Kazakhstan
Kyrgyzstan
Armenia
Azerbaijan
Tajikistan
Turkmenistan
Uzbekistan
Latin America
Brazil
Mexico
Argentina
Chile
Cuba
Ecuador
Peru
Colombia
Venezuela
Middle East & Africa
Saudi Arabia
South Africa
UAE
Algeria
Egypt
Tunisia
Iran
Turkey
Iraq
Morocco
Kuwait
List of Key Players in Meningococcal Vaccines Market
Pfizer Inc.
Sanofi
Serum Institute of India Ltd.
GlaxoSmithKline plc.
Merck & co., Inc.
Walvax Biotechnology Co., Ltd.
Order a free sample PDF of the Meningococcal Vaccines Market Intelligence Study, published by Grand View Research.
0 notes
Text
Conjugation and Meiosis divisions both send genetic material to another cell, but conjugation create unique bacterial cells by exchange genes use plasmid tubes, whereas meiosis divisions create completely unique copies of genetic information use sexual reproduction #geneticteacher
0 notes
Text

The Science Research Folios of S. Sunkavally, p 587.
#alkalinity#excitability of neurones#diabetes#leukocytes#Cambrian explosion#Rubner's law#thermal conductivity of the plasma membrane#heat dissipation from the cell#photosynthesis#singlet oxygen#alpha tocopherol#ethanol#China#lactic acid#origin of metazoans#meteorite#atmospheric oxygen#bacterial conjugation
0 notes
Text
Combating Antibiotic Resistance: A Global Health Threat

Antibiotic resistance is one of the most pressing health challenges of our time. This phenomenon occurs when bacteria evolve and develop the ability to defeat the drugs designed to kill them. As a result, common infections and minor injuries that have been treatable for decades can once again become deadly. The rise of antibiotic resistance threatens to undermine the significant medical advances made over the last century.
In this blog, we’ll get to know the causes and consequences of antibiotic resistance,
Understanding Antibiotic Resistance
Definition
Antibiotic resistance happens when bacteria change in response to the use of these medicines. These resistant bacteria are often referred to as “superbugs.” They survive exposure to antibiotics and continue to multiply, causing more harm. The resistance can occur naturally, but misuse and overuse of antibiotics in humans and animals accelerate the process.
How is it developed?
Natural Selection: Bacteria naturally evolve resistance through mutations. When exposed to antibiotics, susceptible bacteria die, while resistant ones survive and reproduce. Over time, the resistant bacteria become dominant.
Horizontal Gene Transfer: Bacteria can acquire resistance genes from other bacteria through mechanisms like conjugation, transformation, or transduction. This transfer can occur across different bacterial species, spreading resistance rapidly.
Misuse of Antibiotics: Inappropriate prescribing, not completing prescribed antibiotic courses, and using antibiotics for viral infections (like colds and flu) contribute significantly to resistance.
Agricultural Practices: The use of antibiotics in livestock to promote growth and prevent disease in healthy animals is a major factor. These practices lead to the development of resistant bacteria that can transfer to humans through food, water, and direct contact.
Global Impact

It poses a significant threat to global health, food security, and development. Its impact is felt across various sectors:
Healthcare: Infections caused by resistant bacteria are more difficult to treat, requiring longer hospital stays, more intensive care, and more expensive and toxic medications. This leads to increased morbidity and mortality.
Economy: The economic burden of antibiotic resistance is substantial. Increased healthcare costs, loss of productivity due to prolonged illness, and the need for more expensive treatments put a strain on economies worldwide.
Food Security: Antibiotic resistance affects food production and safety. Resistant bacteria can spread from animals to humans through the food chain, making it harder to treat infections from foodborne pathogens.
Global Health: The spread of resistant bacteria does not recognize borders. International travel and trade facilitate the global dissemination of superbugs, making antibiotic resistance a truly global issue that requires coordinated international action.
Strategies
Addressing antibiotic resistance requires a multifaceted approach involving individuals, healthcare providers, policymakers, and industries. Here are key strategies to combat this global health threat:
1. Rational Use of Antibiotics
Education and Awareness: Educating the public about the importance of using antibiotics only when prescribed by a healthcare professional is crucial. Awareness campaigns can help reduce the misuse of antibiotics.
Stewardship Programs: Healthcare facilities should implement antibiotic stewardship programs to ensure that antibiotics are prescribed only when necessary and that the right
antibiotic is chosen for the right duration. These programs can help optimize antibiotic use and reduce the emergence of resistant bacteria.
2. Improved Infection Prevention and Control
Hygiene and Sanitation: Good hygiene practices, such as regular handwashing, can prevent the spread of infections in both healthcare settings and the community. Improved sanitation and access to clean water are also critical in reducing infection rates.
Vaccination: Vaccines can reduce the need for antibiotics by preventing bacterial infections. Expanding vaccination coverage, especially in low- and middle-income countries, can play a significant role.
Infection Control Measures: Hospitals and healthcare facilities should implement strict infection control measures, including the use of personal protective equipment (PPE), sterilization of medical instruments, and isolation of patients with resistant infections.
3. Surveillance and Research
Monitoring and Reporting: Establishing robust surveillance systems to monitor antibiotic use and resistance patterns is essential. This data can inform public health strategies and guide the development of targeted interventions.
Research and Development: Investment in research to develop new antibiotics, alternative treatments, and rapid diagnostic tests is critical. Encouraging innovation and supporting scientific research can help stay ahead of evolving bacterial resistance.
4. Policy and Regulation

Regulatory Measures: Governments should implement and enforce regulations to control the sale and use of antibiotics. This includes restricting over-the-counter sales and ensuring that antibiotics are prescribed only by qualified healthcare professionals.
Agricultural Practices: Policies should promote the responsible use of antibiotics in agriculture. This involves banning the use of antibiotics for growth promotion and implementing guidelines for their therapeutic use in animals.
International Cooperation:This is a global issue that requires international collaboration. Countries should work together to share data, resources, and best practices. Global initiatives, such as the World Health Organization’s Global Action Plan on Antimicrobial Resistance, provide a framework for coordinated action.
The Role of Individuals
Every individual has a role to play in the fight against antibiotic resistance. Here are some steps you can take:
Use Antibiotics Wisely: Only take antibiotics when prescribed by a healthcare professional. Follow the prescribed dosage and complete the entire course, even if you feel better.
Practice Good Hygiene: Regular handwashing, proper food handling, and maintaining cleanliness can help prevent infections and reduce the need for antibiotics.
Stay Vaccinated: Keep up-to-date with recommended vaccinations to protect yourself from infections and reduce the need for antibiotics.
Educate Others: Spread awareness about the dangers of antibiotic resistance and the importance of responsible antibiotic use within your community.
Success Stories
While antibiotic resistance remains a significant challenge, there have been notable successes in combating it:
1. Sweden’s Antibiotic Stewardship Program
Sweden has one of the lowest rates of antibiotic use and resistance in Europe. The country implemented a comprehensive antibiotic stewardship program that includes strict regulations on antibiotic prescriptions, extensive public awareness campaigns, and robust surveillance systems. This multifaceted approach has led to a significant reduction in antibiotic use and a decrease in resistant infections.
2. The Netherlands’ Agricultural Reforms

The Netherlands has made significant strides in reducing antibiotic use in agriculture. The country implemented strict regulations and guidelines for antibiotic use in livestock, including banning antibiotics for growth promotion and limiting their therapeutic use. As a result, antibiotic use in Dutch livestock has decreased by more than 60%, leading to a significant drop in resistant bacteria in both animals and humans.
3. The Fleming Fund
The Fleming Fund, named after Alexander Fleming, the discoverer of penicillin, is a UK aid program that supports low- and middle-income countries in tackling antibiotic resistance. The fund provides financial and technical assistance to improve laboratory capacity, enhance surveillance systems, and strengthen national action plans. This initiative has helped many countries build the infrastructure needed to combat antibiotic resistance effectively.
Conclusion
Antibiotic resistance is a formidable global health threat that requires urgent and coordinated action. By understanding the causes , implementing effective strategies, and fostering international collaboration, we can combat this challenge and protect public health. We can preserve the effectiveness of antibiotics for future generations and ensure a healthier, more resilient world.
In this blog, we have explored the rise of antibiotic resistance, its global impact, and the strategies needed to combat it. By embracing responsible antibiotic use, improving infection prevention and control, investing in research and surveillance, and implementing robust policies, we can address this critical issue. Let us work together to combat antibiotic resistance and safeguard the health of people worldwide.
Did you find this article helpful? Visit more of our blogs! Business Wolf Magazine
0 notes
Text
The TyphiNET data visualisation dashboard: Unlocking Salmonella Typhi genomics data to support public health
Background: Salmonella enterica subspecies enterica serovar Typhi (abbreviated as Typhi) is the bacterial agent of typhoid fever. Effective antimicrobial therapy reduces complications and mortality; however, antimicrobial resistance (AMR) is a major problem in many endemic countries. Prevention through vaccination is possible through recently-licensed Gavi-supported typhoid conjugate vaccines (TCVs), and national immunisation programs are currently being considered or deployed in several countries where AMR prevalence is known to be high. Pathogen whole genome sequence data are a rich source of information on Typhi variants (genotypes or lineages), AMR prevalence, and mechanisms. However, this information is currently not readily accessible to non-genomics experts, including those driving vaccine implementation or empirical therapy guidance. Results: We developed TyphiNET (https://www.typhi.net), an interactive online dashboard for exploring Typhi genotype and AMR distributions derived from publicly available pathogen genome sequences. TyphiNET allows users to explore country-level summaries such as the frequency of pathogen lineages, temporal trends in resistance to clinically relevant antimicrobials, and the specific variants and mechanisms underlying emergent AMR trends. User-driven plots and session reports can be downloaded for ease of sharing. Importantly, TyphiNET is populated by high-quality genome data curated by the Global Typhoid Pathogen Genomics Consortium, analysed using the Pathogenwatch platform, and identified as coming from non-targeted sampling frames that are suitable for estimating AMR prevalence amongst Typhi infections (no personal data is included in the platform). As of February 2024, data from a total of n=11,836 genomes from 101 countries are available in TyphiNET. We outline case studies illustrating how the dashboard can be used to explore these data and gain insights of relevance to both researchers and public health policy-makers. Conclusions: The TyphiNET dashboard provides an interactive platform for accessing genome-derived data on pathogen variant frequencies to inform typhoid control and intervention strategies. The platform is extensible in terms of both data and features, and provides a model for making complex bacterial genome-derived data accessible to a wide audience. http://dlvr.it/T7pkzx
0 notes
Text
Exploring Peptide Discovery: Unveiling Nature’s Molecular Gems
Introduction: Peptides, as nature’s molecular artisans, offer a treasure trove of therapeutic potential, from combating diseases to advancing biotechnological applications. The pursuit of discovering novel peptides is a captivating journey into the intricate world of molecular design and function. This article delves into the strategies and advancements in peptide discovery, highlighting its pivotal role in shaping modern medicine and biotechnology.
Understanding Peptide Discovery: Peptide discovery encompasses a multifaceted approach that integrates computational modeling, combinatorial chemistry, and innovative screening techniques. Computational tools play a crucial role in predicting peptide structures, interactions, and bioactivities, guiding researchers in designing custom peptides with desired properties. Combinatorial chemistry techniques enable the synthesis of vast peptide libraries, providing a diverse pool for screening.
Screening and Selection: High-throughput screening methods accelerate the identification of bioactive peptides from large libraries. Techniques such as phage display, yeast two-hybrid systems, and bacterial display enable the rapid screening of peptide candidates based on their binding affinity, specificity, and functional activity. Moreover, advances in microfluidics and automation have streamlined the screening process, enhancing efficiency and throughput.
Targeted Applications: Peptides exhibit remarkable versatility in therapeutic applications, ranging from antimicrobial agents and anticancer drugs to peptide-based vaccines and drug delivery systems. By targeting specific molecular pathways or cellular receptors, therapeutic peptides offer precise and tailored treatment options with reduced off-target effects. Additionally, peptide-based biomaterials hold promise for tissue engineering, regenerative medicine, and diagnostics, opening new frontiers in healthcare innovation.
Emerging Trends: The field of peptide discovery continues to evolve with emerging trends such as de novo peptide design, peptide-drug conjugates, and peptide-based therapeutics for personalized medicine. De novo design approaches leverage machine learning algorithms and structural biology insights to engineer peptides with enhanced stability, specificity, and bioactivity. Peptide-drug conjugates combine the targeting ability of peptides with the therapeutic payload of small molecules, enabling targeted drug delivery and improved efficacy.
Challenges and Future Directions: Despite significant progress, peptide discovery faces challenges such as peptide stability, bioavailability, and immunogenicity. Addressing these hurdles requires innovative strategies in peptide engineering, formulation, and delivery. Future directions in peptide discovery involve harnessing synthetic biology techniques, exploring peptide mimetics, and leveraging interdisciplinary collaborations to accelerate translation from bench to bedside.
Conclusion: Peptide discovery epitomizes the synergy between scientific inquiry and technological innovation, offering a rich tapestry of molecular solutions to address unmet medical needs and societal challenges. By unraveling nature’s molecular gems, researchers continue to unlock the therapeutic potential of peptides, shaping the landscape of modern medicine and biotechnology.
In essence, peptide discovery transcends the boundaries of traditional drug development, heralding a new era of precision medicine and molecular therapeutics.
0 notes
Text
Bioengineered Protein Drugs Market: Trends Fuel Advanced Remedies
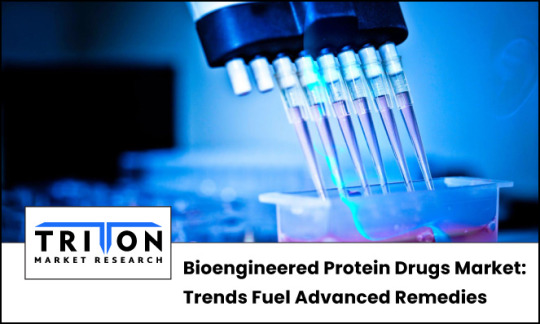
As per IDF Diabetes Atlas 10th edition, around 537 million adults are diagnosed with diabetes globally; this number is anticipated to surge to 643 million by 2030. Type 1 diabetes is among the most common autoimmune diseases, followed by lupus, rheumatoid arthritis, and psoriasis. According to the National Stem Cell Foundation, nearly 4% of the world’s population is distressed by at least 1 out of 80+ diseases. Such high prevalence has increased the adoption of bioengineered protein medications due to high affinity and low toxicity. Based on our estimates, the global bioengineered protein drugs market is predicted to witness progress with a CAGR of 7.7% during the forecast period 2023-2030.
The burden of diseases due to the rising aging population has elevated the demand for advanced medications. As per the World Population Prospects 2019, 1 in 6 people will be over 65 globally by 2050. This has prompted advancements in biotechnology and protein engineering, resulting in the availability of various drugs, including monoclonal antibodies, peptide hormones, vaccines, and fusion proteins.
Let’s look deeper into the key trends prompting developments in drug delivery systems, leading to the bioengineered protein drugs market’s expansion.
· Rising Chronic Cases Amplifies Recombinant Proteins’ Application
Over the past decade, the incidence rate of chronic diseases has soared due to unhealthy lifestyles. According to WHO, more than 15 million people between 30-69 years decease from chronic conditions annually. Cardiovascular disease accounts for most non-communicable disease mortality, followed by cancers, diabetes, etc. This has led to a significant shift in the pharmaceutical industry from chemical drugs to protein drug development. For instance, between 2021 and 2022, the US FDA approved 19 mAbs, 6 peptide hormones, 1 fusion protein, 4 therapeutic enzymes, etc.
Protein drugs using recombinant DNA technology have gained significant traction recently, leading to various launches. For instance, in February 2023, Researchers at the Indian Institute of Technology (IIT) Guwahati developed a ‘Recombinant Protein Toolbox’ consisting of six proteins that convert skin cells into heart cells, especially cardiomyocytes. This toolbox regenerates damaged heart tissues using DNA technology.
· Manufacturing Advancements Widen Drug Scope
Earlier, protein drugs were obtained from humans or other resources. For example, vaccines were formulated using egg cultures. However, recombinant DNA technology has enabled manufacturers to produce bioengineered protein drugs like vaccines, monoclonal antibodies, and blood factors using genetically modified organisms. To elucidate, blood products can be manufactured using DNA technology in bacterial expression or mammalian cell culture systems. The prominence of protein engineering platform technologies is mainly owing to their ability to enhance medications’ functionality, purity, and circulating half-life. Protein conjugation and derivatization are among the major approaches that elevate circulating half-life.
Advancements in protein engineering have led to further R&D investments in protein-based drug manufacturing. For instance, in March 2023, Eli Lilly and Company invested $1.5 billion to expand its manufacturing footprint with new facilities in North Carolina, Indiana, and Ireland. Its facility in Ireland is set to be the most technically advanced manufacturing site for existing monoclonal antibodies, producing existing and pipeline clinical products by 2026. Hence, drug manufacturing and delivery advancements create opportunities for the bioengineered protein drugs market.
Growth Projections: R&D Expands Treatment Possibilities
Considering the rising demand for effective therapies and high unmet needs, healthcare companies are surpassing research boundaries to improve treatments. This has led the pharmaceutical industry to move towards novel molecular formats to deliver breakthrough therapies. Moreover, rare diseases with few or no treatment drugs have influenced players to expand research capabilities and launch new drugs. Since numerous factors drive many complex conditions, constraining one target may not prosper in achieving efficiency. In such a scenario, developing multi-specific antibody formats treats multi-layered diseases by engaging two or more targets with one molecule. Therefore, rising research and development activities are expected to boost the bioengineered protein drugs market.
FAQs:
Q1) Which region holds the highest share in the bioengineered protein drugs market?
North America holds the highest share of the global market.
Q2) Which are the major bioengineered protein drug types?
Monoclonal antibodies, peptide hormones, vaccines, blood factors & peptide antibiotics, fusion proteins, cytokines, and therapeutic enzymes are major drug types.
0 notes
Text
The Power of Antibody Reagents in Biomedical Applications
In the realm of biomedical research and clinical diagnostics, the significance of antibody reagents cannot be overstated. Antibodies are versatile molecules that play crucial roles in various applications, ranging from basic research to the development of diagnostic tests and targeted therapies. This article explores the profound impact of antibody reagents in advancing biomedical science and healthcare.
Understanding Antibody Reagents
Antibodies, also known as immunoglobulins, are specialized proteins produced by the immune system in response to foreign substances known as antigens. These proteins are highly specific and can recognize and bind to unique epitopes on antigens, initiating a series of immune responses. In laboratory settings, antibodies are harnessed as reagents for their ability to selectively target and bind to specific molecules of interest.
Antibodies in Basic Research
One of the primary applications of antibody reagents is in basic research. Scientists use antibodies to identify and localize proteins within cells and tissues, facilitating the study of biological processes and disease mechanisms. Techniques such as immunohistochemistry (IHC) and immunofluorescence (IF) rely on antibodies to visualize specific molecules under a microscope. By labeling antibodies with fluorescent or enzymatic markers, researchers can gain valuable insights into cellular structures and functions.
Moreover, antibodies are indispensable tools in techniques like Western blotting and enzyme-linked immunosorbent assay (ELISA), enabling the detection and quantification of proteins in complex biological samples. These assays are fundamental for characterizing biomarkers, studying protein-protein interactions, and assessing changes in gene expression.
Diagnostic Applications of Antibodies
Antibody-based diagnostics are widely employed in clinical settings for disease detection and monitoring. For instance, rapid diagnostic tests for infectious diseases often utilize antibodies to detect pathogen-specific antigens in patient samples. ELISA-based tests can identify antibodies produced by the immune system in response to infections, aiding in the diagnosis of viral or bacterial diseases.
Antibodies are also pivotal in medical imaging techniques like positron emission tomography (PET) and single-photon emission computed tomography (SPECT). In these methods, radiolabeled antibodies selectively bind to tumor cells or other diseased tissues, allowing for non-invasive visualization and localization of pathological sites within the body.
Therapeutic Potential of Antibody Reagents
The therapeutic landscape has been revolutionized by the development of monoclonal antibody therapies. Monoclonal antibodies, derived from a single clone of cells, are engineered to target specific antigens involved in diseases like cancer, autoimmune disorders, and inflammatory conditions. These antibodies can block harmful pathways, enhance immune responses against tumors, or deliver therapeutic payloads directly to affected cells.
Examples of successful monoclonal antibody therapies include trastuzumab for HER2-positive breast cancer and rituximab for B-cell lymphomas. The precision and efficacy of these therapies highlight the therapeutic potential of antibody reagents in personalized medicine.
Challenges and Future Directions
Despite their remarkable utility, antibody reagents face challenges such as batch-to-batch variability, stability issues, and the potential for immunogenicity. Researchers are actively addressing these issues through advanced techniques in antibody engineering and production.
The future of antibody reagents lies in developing novel formats and improving their specificity and affinity. Emerging technologies like bispecific antibodies, antibody-drug conjugates, and antibody fragments hold promise for enhancing therapeutic outcomes and expanding the scope of antibody-based applications.
Conclusion
In conclusion, antibody reagents represent a cornerstone of modern biomedical research and healthcare. From elucidating molecular pathways in basic science to enabling precise diagnostics and targeted therapies, antibodies continue to drive innovation and impact patient outcomes. As technology advances and our understanding of antibody biology deepens, the potential of antibody reagents in biomedical applications is poised to grow exponentially, ushering in a new era of personalized medicine and transformative therapies.
Through their versatility, specificity, and therapeutic potential, antibody reagents stand as testament to the enduring power of immunological tools in improving human health and combating disease.
0 notes
Link
0 notes