#Thermal Fluid Heating System
Explore tagged Tumblr posts
Text
Once you have decided that a thermal fluid heating system is the best option for your process heating needs, what should you consider next? In this blog, we will highlight the top five things that you need to know about thermal fluids heaters.
0 notes
Text
Gas Fired Thermic Fluid Heater
Introducing Avon Engineering state-of-the-art Gas Fired Thermic Fluid Heater – an embodiment of innovation in industrial heating solutions. This advanced heater redefines heat transfer, offering a seamless fusion of speed, efficiency, durability, and precise temperature control for a wide range of industrial applications.

Key Features:
Experience the efficiency of quick and uniform heat transfer using thermic fluid with Avon Engineering Gas Fired Thermic Fluid Heater. Furthermore, it adeptly converts gas into heat, ensuring rapid and reliable heat exchange for various operational needs. In addition, embrace the efficiency of gas as an energy source. Our heater taps into this reliable fuel type, providing a continuous and dependable heat source for diverse applications. Moreover, designed with longevity in mind, our heater features robust construction that can withstand the demands of industrial environments. Its sturdy build guarantees consistent performance and reliability over time. Additionally, achieve accurate temperature control within your processes with our Product. Its advanced control systems enable you to maintain the desired temperature ranges, crucial for applications requiring specific heat levels. @avonengineering Follow For All Updates
Experience Cutting-Edge Heat Transfer: Our Product introduces a new realm of industrial heating. Whether for manufacturing, chemical processing, or other sectors, this advanced heater delivers reliable, efficient, and precise heat transfer, optimizing your processes. It integrates technology, efficiency, durability, and precision, offering a forward-focused solution that aligns with your energy and operational goals. Elevate your heat transfer processes and invest in a product that ensures exceptional performance. Embrace a new era of heat exchange with our state-of-the-art Gas Fired Thermic Fluid Heater.
#Gas Fired Thermic Fluid Heater#Thermic Fluid Heater#Gas Heater#Industrial Heat Transfer Equipment#Fluid Heating System#High Efficiency Heating Solutions#Rapid Heat Exchange#Industrial Fluid Heating Technology#Gas Powered Heating System#Industrial Process Heating#Thermic Fluid Heating Equipment#Reliable Heat Transfer Solutions#Energy Efficient Fluid Heater#Thermal Fluid Heating System#Industrial Heat Generation Technology
0 notes
Text
Efficient and Reliable Thermal Fluid Heaters
Thermodyne revolutionizes industry standards with our cutting-edge thermal fluid heaters, setting a new benchmark for efficiency and dependability in heat transfer solutions. Thermodyne thermal fluid heaters are meticulously engineered for precise and efficient heat transfer, ensuring optimal performance in a wide range of industrial applications. Our thermal fluid heaters are designed to meet the ever-changing needs of modern industries, providing a sustainable and energy-efficient heating solution. We recognize that each industry has unique heating requirements. Thermodyne offers customizable thermal fluid heaters tailored to specific specifications, ensuring a seamless integration with your operations. For more information contact us at https://www.thermodyneboilers.com/thermic-fluid-heaters/
#Thermal Fluid Heater#Industrial Boilers#Heat Transfer Solutions#Efficient Heating Systems#Energy-Efficient Boilers
0 notes
Text
How Can Computational Fluid Dynamics (CFD) Be Used For Designing Better EV Battery And Thermal Management Systems (BTMS)
Introduction Electric vehicle batteries are transforming how we move, live, and work. Architects and engineers have a crucial role in shaping the future of EVs and their integration with buildings and infrastructure. By understanding electric vehicle batteries’ challenges and opportunities, they can create innovative and sustainable solutions that benefit both people and the planet. However, EVs…
View On WordPress
#Battery Heat Dissipation#BTMS#BTMS Optimization#CFD#CFD for Battery Design#CFD in BTMS#CFD Simulation for EV Batteries#Computational Fluid Dynamics#Computational Fluid Dynamics for EV Batteries#Computational Fluid Dynamics for Thermal Management#Designing Better EV Battery#EV Battery Cooling#EV Battery Cooling Solutions#EV Battery Design#EV Battery Efficiency#Thermal Management in EV Batteries#Thermal Management Systems
0 notes
Text

Tesla-inspired method can control the direction of heat flow in graphite crystals
Researchers from the Institute of Industrial Science, The University of Tokyo, have developed a method to control the direction of heat flow in crystals. This miniature device could eventually be used to create advanced thermal-management systems in electronic devices to prevent overheating. Unwanted heating of electronic components hinders the performance of many devices. For example, the processing speed and memory available to silicon-based computer chips depend strongly on the ability to dissipate heat effectively. Unfortunately, despite high demand, thermal management remains challenging. In their study published in Nature, the research team demonstrates the ability to control heat transfer in graphite crystals. Their novel approach applies concepts from fluid dynamics to phonons in solid-state crystals. The article is titled "A graphite thermal Tesla valve driven by hydrodynamic phonon transport."
Read more.
#Materials Science#Science#Graphite#Heat flow#Temperature#University of Tokyo#Fluid dynamics#Phonons
12 notes
·
View notes
Text



Research abounds at the International Space Station
At the International Space station, researchers are making strides in everything from Earth science to chemical properties. Here's what they're up to and why it matters.
Recently, researchers have found that eddies, or swirling wind patterns, increased moisture evaporation in an alfalfa field. A better understanding of the complex exchange of water and heat between the ground and atmosphere could improve remote sensing products and their use in agricultural water management.
The station's ECOSTRESS instrument takes high-resolution thermal infrared measurements of Earth's surface that provide data on changes in water availability, vegetation water stress, and agricultural water use. Researchers use observations from the USGS Landsat 8 and 9 satellites and ECOSTRESS to validate climate models and update data on Earth's surface energy (the amount of energy absorbed from the sun and radiated back into the atmosphere).
Properties of flow boiling
Researchers have identified various properties for flow boiling using n-perfleurohexane, a fluid used to cool electronics. A better understanding of this process can improve models for designing thermal cooling systems used in the electronics, energy, aerospace, and other industries.
Flow boiling, a method of thermal management, uses the heat generated by a device to boil a liquid, generating vapor bubbles that lift the heat from the surface. The Flow Boiling and Condensation Experiment (FBCE) tested a flow boiling method in microgravity, where the process is less efficient; in the absence of buoyancy, bubbles grow larger and remain near the surface.
A new radiation-resistant polymer
Researchers successfully manufactured a polymer of rare metals and other elements that showed high radiation resistance and has a suitable size and weight for use in space. This result provides knowledge that can support development of improved shielding for future spacecraft and extraterrestrial habitats.
The Roscosmos investigation Shielding Composite tested the absorbed radiation dose of two polymers during 225 days on the space station using monthly monitoring by the Pille-ISS investigation. The data showed that the material has high and stable radiation shielding characteristics. Protecting crew members and equipment from radiation is an important requirement for future long-duration space missions.
TOP IMAGE: The ECOSTRESS instrument on the International Space Station. Credit: NASA
CENTRE IMAGE: ECOSTRESS data shows evaporative stress in agricultural fields in California’s San Joaquin Valley. Credit: NASA
LOWER IMAGE: NASA astronauts Mark Vande Hei and Kayla Barron set up for the Flow Boiling and Condensation Experiment. Credit: NASA
11 notes
·
View notes
Text
Thermodynamics and Its Relevance to Mechanical Engineering
There are so many colleges but Arya College of Engineering & I.T. is the best college in Jaipur Rajasthan which provides information about thermodynamics it is a fundamental branch of mechanical engineering that deals with the principles governing energy, heat, and work. It is essential for understanding how energy is transformed and utilized in various systems, making it a cornerstone of mechanical engineering applications. This exploration of thermodynamics will cover its basic principles, laws, applications, and significance in mechanical engineering.
Fundamental Principles of Thermodynamics
Thermodynamics is primarily concerned with the relationships between heat and other forms of energy. It is governed by several key laws:
1. Zeroth Law of Thermodynamics: This law establishes the concept of temperature and thermal equilibrium. It states that if two systems are in thermal equilibrium with a third system, they are also in thermal equilibrium with each other.
2. First Law of Thermodynamics: Often referred to as the law of energy conservation, it states that energy cannot be created or destroyed, only transformed. Mathematically, it can be expressed as:
ΔU=Q−WΔU=Q−W
where ΔUΔU is the change in internal energy, QQ is the heat added to the system, and WW is the work done by the system.
3. Second Law of Thermodynamics: This law introduces the concept of entropy, indicating that energy transformations are not 100% efficient and that heat cannot spontaneously flow from a colder to a hotter object. It establishes the direction of natural processes and the limits of energy conversion.
4. Third Law of Thermodynamics: This law states that as a system approaches absolute zero, its entropy approaches a constant, usually zero. It provides a basis for defining absolute entropy.
Applications of Thermodynamics in Mechanical Engineering
Thermodynamics principles are applied in various areas of mechanical engineering, including:
1. Power Generation: Thermodynamics is crucial in the design and analysis of power plants, such as steam power plants, gas turbines, and internal combustion engines. It helps optimize the efficiency of energy conversion processes.
2. Refrigeration and Air Conditioning: The principles of thermodynamics are used to design and analyze refrigeration and air conditioning systems, ensuring efficient heat transfer and maintaining desired temperatures.
3. Fluid Mechanics: Thermodynamics is closely related to fluid mechanics, as it helps understand the behavior of fluids under different conditions, such as pressure and temperature changes.
4. Heat Transfer: Thermodynamics provides the foundation for understanding heat transfer mechanisms, including conduction, convection, and radiation, which are essential in the design of heat exchangers, boilers, and other thermal systems.
5. Combustion: Thermodynamics is used to analyze combustion processes, which are crucial in the design of internal combustion engines, gas turbines, and other systems that rely on burning fuels.
Significance of Thermodynamics in Mechanical Engineering
Thermodynamics is a fundamental aspect of mechanical engineering for several reasons:
1. It provides a framework for understanding energy transformations: Thermodynamics helps engineers analyze and optimize the conversion of energy from one form to another, ensuring efficient and effective systems.
2. It enables the design of thermal systems: Thermodynamics is essential in designing and analyzing thermal systems, such as power plants, refrigeration units, and HVAC systems, ensuring their proper functioning and efficiency.
3. It helps in material selection: Thermodynamics provides insights into the behavior of materials under different conditions, allowing engineers to select appropriate materials for specific applications.
4. It contributes to sustainability: By understanding the principles of energy conversion and the limitations imposed by the laws of thermodynamics, engineers can design more sustainable and environmentally friendly systems.
In conclusion, thermodynamics is a crucial aspect of mechanical engineering, providing a fundamental understanding of energy transformations and enabling the design of efficient and effective systems. Its applications span various domains, from power generation to refrigeration and air conditioning, making it an essential tool for mechanical engineers in their pursuit of innovation and progress.
4 notes
·
View notes
Text
Mechanical Engineering Basics: A Beginner's Guide

Welcome to the world of mechanical engineering—a field that drives innovation, shapes our daily lives, and fuels industries from aerospace to automotive. Whether you're contemplating a career in mechanical engineering or just curious about what it entails, this guide will walk you through the essentials. We'll break down what mechanical engineering is, its key areas, essential skills, educational pathways, and much more. By the end of this post, you’ll have a solid understanding of what it means to be a mechanical engineer and how you can embark on this exciting career.
Mechanical engineering is everywhere—from the cars we drive to the smartphones we use. It’s a diverse field that combines principles of physics, mathematics, and material science to design and build machines and systems. But what exactly does a mechanical engineer do? And why should you consider a career in mechanical engineering?
In this guide, I’ll help you unravel the mysteries of mechanical engineering. Whether you’re thinking about pursuing a degree in this field or just want to understand it better, we’ll cover all the basics you need to know.
1. What is Mechanical Engineering?
Definition
Mechanical engineering is a branch of engineering that focuses on the design, analysis, and manufacturing of mechanical systems. It involves applying principles of physics and materials science to solve engineering problems and create new technologies. Essentially, it’s about making things work efficiently and effectively.
Historical Background
Mechanical engineering has a rich history that dates back to ancient civilizations. From the early water wheels and windmills to the revolutionary steam engines of the Industrial Revolution, mechanical engineering has been instrumental in technological advancements. Understanding its history gives us insight into how far the field has come and its impact on modern technology.
Core Principles
At the heart of mechanical engineering are three core principles:
Forces and Motion: Understanding how forces interact with objects and how they move is fundamental. This includes studying concepts like torque, momentum, and kinematics.
Energy: Mechanical engineers work with various forms of energy, including thermal, mechanical, and potential energy, to design efficient systems and processes.
Materials: The properties of materials—such as strength, elasticity, and thermal conductivity—are crucial in selecting the right materials for different applications.
2. Key Areas of Mechanical Engineering
Thermodynamics
Thermodynamics deals with the principles of heat and energy transfer. It’s essential for designing systems like engines, refrigerators, and air conditioners. Understanding concepts like the laws of thermodynamics, heat cycles, and entropy helps engineers optimize energy use and improve system efficiency.
Fluid Mechanics
Fluid mechanics involves the study of fluids (liquids and gases) and their behavior under various conditions. It’s crucial for applications such as designing pumps, aircraft, and hydraulic systems. Engineers use fluid mechanics to analyze and predict how fluids will interact with surfaces and move through systems.
Mechanics of Materials
This area focuses on how materials deform and fail under different types of stress. Mechanics of materials is important for designing structures and components that can withstand forces without breaking. Engineers study stress, strain, and material properties to ensure reliability and safety in their designs.
Dynamics and Control
Dynamics deals with the motion of objects and forces acting on them. Control systems involve regulating and managing the behavior of dynamic systems. Together, they help engineers design systems that can move and operate smoothly, like robotics or automated manufacturing systems.
Manufacturing Processes
Manufacturing processes cover the techniques used to produce parts and products. This includes methods like casting, machining, welding, and additive manufacturing (3D printing). Understanding these processes helps engineers select the best methods for producing high-quality, cost-effective components.
Mechanical Design
Mechanical design involves creating and developing mechanical systems and components. It requires a combination of creativity and technical knowledge to develop designs that meet specific requirements and constraints. Engineers use design principles, CAD software, and prototyping to bring their ideas to life.
3. Essential Skills for Mechanical Engineers
Mathematical Proficiency
Mechanical engineering relies heavily on mathematics, including calculus, algebra, and differential equations. These mathematical tools are used to model physical systems, analyze forces, and solve complex problems. A strong grasp of math is essential for success in this field.
Problem-Solving Skills
Engineers are often faced with complex problems that require innovative solutions. Strong analytical and critical thinking skills help engineers identify issues, evaluate options, and implement effective solutions. Being a creative problem solver is a key trait of successful mechanical engineers.
Technical Knowledge
Mechanical engineers need a solid understanding of engineering principles, materials science, and mechanics. This technical knowledge allows them to design, analyze, and optimize mechanical systems effectively. Staying current with technological advancements is also important.
Software Skills
In today’s digital world, proficiency with engineering software is crucial. CAD (Computer-Aided Design) software, simulation tools, and other engineering applications help engineers create designs, test simulations, and visualize their projects. Familiarity with these tools enhances productivity and accuracy.
Communication Skills
Effective communication is essential for collaborating with team members, presenting ideas, and documenting work. Mechanical engineers often work in teams and must be able to explain complex concepts clearly and concisely. Strong communication skills help ensure that projects run smoothly and meet objectives.
4. Educational Pathways
Degrees and Certifications
To become a mechanical engineer, you typically need a bachelor’s degree in mechanical engineering or a related field. Advanced degrees, such as a master’s or Ph.D., can open up additional opportunities for specialization and research. Certifications, such as Professional Engineer (PE) licensure, may also be required for certain positions.
Accreditation
When choosing an educational program, it’s important to select a school that is accredited by a recognized body. Accreditation ensures that the program meets industry standards and provides a quality education. Look for programs accredited by organizations like the Accreditation Board for Engineering and Technology (ABET).
Continuing Education
Mechanical engineering is a constantly evolving field. To stay competitive and up-to-date, engineers often engage in continuing education and professional development. This can include attending workshops, earning certifications, or participating in industry conferences.
5. Tools and Technologies
Engineering Software
Modern mechanical engineers rely on various software tools to assist with design, analysis, and simulation. CAD software helps create detailed 2D and 3D models of components and systems. Simulation tools allow engineers to test and validate their designs under different conditions. Mastery of these tools is essential for modern engineering practice.
Lab Equipment
Mechanical engineering labs are equipped with a range of instruments and machinery used for testing and experimentation. Common lab equipment includes tensile testers, flow meters, and thermal chambers. Hands-on experience with these tools is crucial for understanding how theoretical concepts apply in practice.
Emerging Technologies
The field of mechanical engineering is rapidly advancing with new technologies. Innovations such as robotics, 3D printing, and artificial intelligence are transforming the way engineers design and manufacture products. Staying informed about these trends can open up exciting opportunities and keep your skills relevant.
6. Career Opportunities
Industry Sectors
Mechanical engineers work in a variety of sectors, including automotive, aerospace, energy, and manufacturing. Each sector offers unique challenges and opportunities. Whether you’re interested in designing vehicles, developing renewable energy solutions, or working on cutting-edge technologies, there’s likely a niche for you.
Job Roles
Mechanical engineers can pursue various roles, including design engineer, manufacturing engineer, project manager, and research scientist. Each role involves different responsibilities and requires a unique set of skills. Exploring different job roles can help you find a career path that aligns with your interests and goals.
Career Growth
Mechanical engineering offers numerous opportunities for career growth. With experience, you can move into higher-level positions, such as senior engineer or engineering manager. Specializing in a particular area or pursuing advanced degrees can also enhance your career prospects.
7. Real-World Applications
Case Studies
Mechanical engineering projects can range from developing new medical devices to designing efficient energy systems. For example, engineers working on medical devices might design prosthetic limbs that enhance patients' mobility, while those in energy might work on optimizing wind turbines for better performance.
Problem-Solving Examples
Mechanical engineers tackle a wide range of problems, from improving the fuel efficiency of cars to designing safer buildings. By applying engineering principles and creative solutions, they address real-world challenges and contribute to technological advancements.
8. Challenges and Future Trends
Current Challenges
Mechanical engineers face several challenges, including sustainability, resource management, and integrating new technologies. Addressing these challenges requires innovative thinking and a commitment to finding solutions that balance performance with environmental and economic considerations.
Future Trends
The future of mechanical engineering is bright and full of potential. Emerging trends such as smart materials, advanced robotics, and sustainable manufacturing are shaping the industry. Engineers who stay abreast of these trends will be well-positioned to lead and innovate in the coming years.
Mechanical engineering is a dynamic and rewarding field that plays a crucial role in shaping the world around us. By understanding its core principles, key areas, and career opportunities, you can gain a clearer picture of what it means to be a mechanical engineer. If you’re considering a career in mechanical engineering, it’s an exciting field with endless possibilities and opportunities for growth.
FAQs
What is mechanical engineering? Mechanical engineering is a branch of engineering that involves designing, analyzing, and manufacturing mechanical systems. It combines principles of physics, mathematics, and materials science to create and improve technologies.
What are the core principles of mechanical engineering? The core principles include forces and motion, energy, and materials. These principles help engineers understand how to design and build efficient mechanical systems.
What skills are important for a mechanical engineer? Important skills include mathematical proficiency, problem-solving abilities, technical knowledge, software skills, and strong communication skills.
What degree do I need to become a mechanical engineer? Typically, you need a bachelor’s degree in mechanical engineering or a related field. Advanced degrees and certifications can enhance career opportunities.
What types of industries employ mechanical engineers? Mechanical engineers work in various industries, including automotive, aerospace, energy, manufacturing, and robotics.
What are some common tools used in mechanical engineering? Common tools include CAD software for design, simulation tools for testing, and various lab equipment for experimentation.
What is thermodynamics, and why is it important? Thermodynamics is the study of heat and energy transfer. It’s crucial for designing systems like engines and refrigerators to ensure they operate efficiently.
How can I stay updated with advancements in mechanical engineering? Staying updated involves continuing education, attending industry conferences, and following emerging technologies and trends.
What career opportunities are available for mechanical engineers? Career opportunities include roles such as design engineer, manufacturing engineer, project manager, and research scientist.
What are the future trends in mechanical engineering? Future trends include advancements in robotics, smart materials, and sustainable manufacturing practices.
I hope this guide has provided you with a comprehensive understanding of mechanical engineering. If you're considering a career in this field, it offers exciting opportunities and challenges that can make a significant impact on the world. Feel free to dive deeper into each topic and explore how you can contribute to the future of engineering.
3 notes
·
View notes
Text
Exploring the Growing $21.3 Billion Data Center Liquid Cooling Market: Trends and Opportunities
In an era marked by rapid digital expansion, data centers have become essential infrastructures supporting the growing demands for data processing and storage. However, these facilities face a significant challenge: maintaining optimal operating temperatures for their equipment. Traditional air-cooling methods are becoming increasingly inadequate as server densities rise and heat generation intensifies. Liquid cooling is emerging as a transformative solution that addresses these challenges and is set to redefine the cooling landscape for data centers.
What is Liquid Cooling?
Liquid cooling systems utilize liquids to transfer heat away from critical components within data centers. Unlike conventional air cooling, which relies on air to dissipate heat, liquid cooling is much more efficient. By circulating a cooling fluid—commonly water or specialized refrigerants—through heat exchangers and directly to the heat sources, data centers can maintain lower temperatures, improving overall performance.
Market Growth and Trends
The data centre liquid cooling market is on an impressive growth trajectory. According to industry analysis, this market is projected to grow USD 21.3 billion by 2030, achieving a remarkable compound annual growth rate (CAGR) of 27.6%. This upward trend is fueled by several key factors, including the increasing demand for high-performance computing (HPC), advancements in artificial intelligence (AI), and a growing emphasis on energy-efficient operations.
Key Factors Driving Adoption
1. Rising Heat Density
The trend toward higher power density in server configurations poses a significant challenge for cooling systems. With modern servers generating more heat than ever, traditional air cooling methods are struggling to keep pace. Liquid cooling effectively addresses this issue, enabling higher density server deployments without sacrificing efficiency.
2. Energy Efficiency Improvements
A standout advantage of liquid cooling systems is their energy efficiency. Studies indicate that these systems can reduce energy consumption by up to 50% compared to air cooling. This not only lowers operational costs for data center operators but also supports sustainability initiatives aimed at reducing energy consumption and carbon emissions.
3. Space Efficiency
Data center operators often grapple with limited space, making it crucial to optimize cooling solutions. Liquid cooling systems typically require less physical space than air-cooled alternatives. This efficiency allows operators to enhance server capacity and performance without the need for additional physical expansion.
4. Technological Innovations
The development of advanced cooling technologies, such as direct-to-chip cooling and immersion cooling, is further propelling the effectiveness of liquid cooling solutions. Direct-to-chip cooling channels coolant directly to the components generating heat, while immersion cooling involves submerging entire server racks in non-conductive liquids, both of which push thermal management to new heights.
Overcoming Challenges
While the benefits of liquid cooling are compelling, the transition to this technology presents certain challenges. Initial installation costs can be significant, and some operators may be hesitant due to concerns regarding complexity and ongoing maintenance. However, as liquid cooling technology advances and adoption rates increase, it is expected that costs will decrease, making it a more accessible option for a wider range of data center operators.
The Competitive Landscape
The data center liquid cooling market is home to several key players, including established companies like Schneider Electric, Vertiv, and Asetek, as well as innovative startups committed to developing cutting-edge thermal management solutions. These organizations are actively investing in research and development to refine the performance and reliability of liquid cooling systems, ensuring they meet the evolving needs of data center operators.
Download PDF Brochure :
The outlook for the data center liquid cooling market is promising. As organizations prioritize energy efficiency and sustainability in their operations, liquid cooling is likely to become a standard practice. The integration of AI and machine learning into cooling systems will further enhance performance, enabling dynamic adjustments based on real-time thermal demands.
The evolution of liquid cooling in data centers represents a crucial shift toward more efficient, sustainable, and high-performing computing environments. As the demand for advanced cooling solutions rises in response to technological advancements, liquid cooling is not merely an option—it is an essential element of the future data center landscape. By embracing this innovative approach, organizations can gain a significant competitive advantage in an increasingly digital world.
#Data Center#Liquid Cooling#Energy Efficiency#High-Performance Computing#Sustainability#Thermal Management#AI#Market Growth#Technology Innovation#Server Cooling#Data Center Infrastructure#Immersion Cooling#Direct-to-Chip Cooling#IT Solutions#Digital Transformation
2 notes
·
View notes
Text
Gate Valves in the Petrochemical Industry: Key Applications

In the complex and demanding environment of the petrochemical industry, the selection of appropriate valves is critical to ensuring the efficiency and safety of operations. Among the various types of valves used, gate valves play a crucial role due to their unique characteristics and versatility. This article explores the key applications of gate valves in the petrochemical industry and highlights their importance, especially for those seeking reliable suppliers such as a Valve Stockist in the Dubai or renowned Valve Manufacturers globally.
Understanding Gate Valves
Gate valves are primarily used to either completely stop or allow the flow of a fluid through a pipeline. Unlike other valve types, gate valves operate by lifting a gate out of the path of the fluid. This mechanism is ideal for applications that require the valve to be either fully open or fully closed, making them unsuitable for flow regulation but perfect for on-off control.
Key Applications in the Petrochemical Industry
Isolation of Flow:
Gate valves are extensively used for isolating sections of pipelines during maintenance or emergencies. Their ability to provide a tight seal ensures that no leakage occurs, which is vital in handling hazardous fluids. This makes them indispensable in petrochemical plants where safety is paramount.
Handling High-Pressure Fluids:
The petrochemical industry often deals with high-pressure fluids, and gate valves are well-suited to handle such conditions. Their robust design and capability to withstand high pressures make them a preferred choice for critical applications. Valve Manufacturers in Spain are known for producing high-quality gate valves that can operate efficiently under extreme conditions.
Controlling Corrosive Media:
Petrochemical processes frequently involve corrosive media. Gate valves made from specialized materials, such as stainless steel or alloy, are used to resist corrosion and prolong the valve’s service life. For industries looking for specialized valves, engaging with a reputable Gate Valves Supplier is crucial to ensure durability and reliability.
Steam and Heat Transfer Systems:
In petrochemical plants, steam and heat transfer systems are integral to various processes. Gate valves are employed in these systems to manage the flow of steam and other high-temperature fluids. These valves can handle the thermal expansion and contraction that occur in such systems, making them a vital component of heat management.
Shutdown Systems:
In emergency shutdown systems, gate valves are often used due to their ability to quickly and completely stop the flow of hazardous fluids. This quick response capability is critical in preventing accidents and ensuring the safety of the plant and its personnel.
Sourcing the Right Gate Valves
For businesses in the petrochemical industry, sourcing the right gate valves from reliable suppliers is essential. A Valve Stockist offers a wide range of options, ensuring that industries can find the exact specifications they need. Moreover, working with a Ball valves Supplier, Globe Valves Supplier, or Butterfly valve supplier can provide comprehensive valve solutions for various applications.
Additionally, many industries are turning to international markets for their valve needs. Valve Manufacturers in Spain have earned a reputation for their quality and innovation, providing robust solutions for petrochemical applications. Engaging with such manufacturers ensures access to advanced technology and high-quality products.
Conclusion
Gate valves are indispensable in the petrochemical industry, serving critical functions across various applications. Whether isolating sections of a pipeline, handling high-pressure fluids, or managing corrosive media, these valves provide the reliability and safety that petrochemical plants demand. To ensure optimal performance and safety, it is crucial to source these valves from reputable suppliers, such as a Valve Stockist in UAE or leading Valve Manufacturers globally. Additionally, working with specialized suppliers like a Control valves supplier or Water Valves Supplier can further enhance the efficiency and safety of petrochemical operations
2 notes
·
View notes
Text
An immersed liquid-cooled load bank is a type of load bank that uses a liquid coolant, typically water or a dielectric fluid, to manage the heat generated during electrical testing. These load banks are designed to dissipate large amounts of heat efficiently, making them ideal for high-power applications where traditional air-cooled systems might be insufficient.
Key Features of Immersed Liquid-Cooled Load Banks:
Efficient Heat Dissipation: The use of liquid cooling allows for the rapid transfer of heat away from the resistive elements, maintaining optimal performance even during extended testing periods.
Compact Design: Because liquid cooling is more efficient than air cooling, these load banks can be more compact, fitting into smaller spaces while still handling significant power loads.
High-Power Capacity: Immersed liquid-cooled load banks are capable of handling very high power loads, making them suitable for testing large generators, UPS systems, and other high-power equipment.
Durability and Longevity: The liquid cooling system not only helps in managing heat but also extends the life of the resistive elements by preventing overheating and reducing thermal stress.
Versatile Applications: These load banks are used in various industries, including data centers, power generation, and renewable energy, where reliable load testing is crucial for maintaining system performance.

Applications:
Generator Testing: Ensures that generators can handle their maximum load without overheating, providing confidence in their reliability during operation.
Data Centers: Used for testing backup power systems to ensure they can sustain critical loads during power outages.
Renewable Energy Systems: Helps in testing the performance and reliability of renewable energy installations, such as wind turbines and solar power systems.

Advantages Over Air-Cooled Load Banks:
Higher Efficiency: Liquid cooling is more effective at heat removal than air cooling, which allows for continuous operation under high load conditions.
Smaller Footprint: The enhanced cooling efficiency allows for a more compact design, saving space in testing environments.
Reduced Noise: Liquid-cooled systems generally produce less noise compared to air-cooled systems, which rely on fans for cooling.

Considerations:
Maintenance: Liquid-cooled systems require regular maintenance to ensure the coolant is clean and the system is free of leaks.
Initial Cost: The upfront cost for an immersed liquid-cooled load bank may be higher than that of an air-cooled equivalent due to the complexity of the cooling system.
2 notes
·
View notes
Text
How Pipe Material Impacts the Efficiency of Your Solar System
As solar energy becomes increasingly popular, more households and industries are adopting solar systems as an efficient, sustainable source of power. While the focus is often on solar panels and inverters, one often-overlooked component in the system is the pipe material used to transport heat in solar thermal systems. The right pipes for solar system works can greatly impact the overall efficiency, longevity, and performance of the system. For this reason, choosing the right piping is essential to maximizing your solar system’s potential.

In this blog, we will explore how the selection of pipe materials affects the efficiency of solar systems, the available options, and the importance of working with reliable pipes for solar system works distributors in Vadodara and pipes for solar system works distributors in Gujarat.
The Role of Pipes in Solar Systems
Solar systems are commonly divided into two types: photovoltaic (PV) systems and solar thermal systems. Photovoltaic systems convert sunlight directly into electricity, while solar thermal systems capture heat from the sun to warm water or air for domestic or industrial use. Pipes are essential in solar thermal systems, as they transport the heated fluid (usually water or antifreeze) from the solar collectors to storage tanks or directly to the point of use.
The performance of a solar thermal system heavily depends on the pipes' material. Poor-quality pipes can lead to energy losses, increased wear and tear, and potential leaks, which reduces the system's overall efficiency. On the other hand, selecting the right pipes can enhance heat transfer, reduce energy losses, and ensure the system operates effectively over time.
Importance of Pipe Material in Solar Systems
The selection of the right pipe material is crucial in a solar thermal system because of the high temperatures and pressures involved. Pipes need to be durable, resistant to corrosion, and able to handle extreme conditions. Here are some key factors to consider when choosing pipe materials for solar systems:
Thermal Conductivity: Pipes with good thermal conductivity will efficiently transfer heat from the solar collector to the storage tank, reducing energy losses along the way. Materials like copper and stainless steel are highly conductive, making them ideal for solar systems.
Temperature Resistance: Solar systems often operate at high temperatures, especially in regions with abundant sunlight. The pipes must be able to withstand these temperatures without degrading or losing efficiency.
Corrosion Resistance: Since the pipes in solar systems are exposed to both heat and moisture, they need to be resistant to corrosion. Corrosion can lead to leaks and system failures, so using corrosion-resistant materials like stainless steel or specialized polymers is essential.
Pressure Resistance: The pipes in solar systems are subjected to constant pressure due to the circulation of fluid. Materials like copper, stainless steel, and high-quality polymers are able to maintain their integrity under pressure, ensuring long-lasting performance.
Durability and Longevity: Solar systems are designed to last for 20 to 25 years or more. The pipes must be durable enough to withstand the rigors of time, environmental conditions, and wear and tear, ensuring the system remains functional throughout its lifespan.
Common Pipe Materials Used in Solar Systems
There are several materials commonly used for piping in solar thermal systems. Each material has its advantages and disadvantages, depending on the specific needs of the system.
1. Copper
Copper is one of the most popular pipe materials used in solar thermal systems, especially for domestic hot water applications. It has excellent thermal conductivity, making it efficient in transferring heat from the solar collectors to the storage tank. Copper is also highly resistant to corrosion, which ensures a longer lifespan for the pipes. Additionally, copper pipes can withstand high temperatures and pressure, making them ideal for solar systems.
However, copper can be expensive compared to other materials. It is also susceptible to theft, so additional precautions may need to be taken in certain locations.
2. Stainless Steel
Stainless steel is another common choice for solar system pipes due to its high resistance to corrosion, excellent thermal conductivity, and ability to withstand extreme temperatures and pressures. Stainless steel is particularly well-suited for larger solar thermal systems used in commercial or industrial settings, as it can handle the demands of heavy-duty applications.
While stainless steel pipes are more expensive than some other materials, they offer exceptional durability and longevity, making them a cost-effective choice over the system’s lifespan.
3. PEX (Cross-Linked Polyethylene)
PEX pipes are made from a type of plastic that is flexible, durable, and resistant to corrosion. PEX is often used in solar thermal systems for domestic hot water applications due to its lower cost and ease of installation. PEX pipes are particularly useful in systems where the fluid temperature is moderate, as they can withstand temperatures up to 200°F (93°C).
However, PEX is not suitable for high-temperature or high-pressure solar systems. It is best used in smaller residential applications where the conditions are less extreme.
4. Polypropylene (PP)
Polypropylene pipes are increasingly being used in solar systems due to their excellent resistance to high temperatures, UV radiation, and corrosion. They are particularly well-suited for large-scale solar thermal systems and solar district heating systems. PP pipes are lightweight, easy to install, and can handle temperatures up to 248°F (120°C).
One of the main advantages of PP pipes is their environmental friendliness. They are fully recyclable and have a low carbon footprint, making them an excellent choice for sustainable energy systems.
The Importance of Choosing the Right Distributor
Selecting the right pipe material is only part of the equation. Working with a reputable pipes for solar system works distributor in Vadodara or pipes for solar system works distributor in Gujarat is equally important to ensure you receive high-quality products that meet your system's specifications.
Here is why partnering with the right distributor is crucial:
Quality Assurance: A reliable distributor will supply pipes that meet industry standards for durability, thermal conductivity, and corrosion resistance. This ensures that the materials you use will perform well under the demands of a solar thermal system.
Technical Support: Distributors with expertise in solar systems can provide valuable advice on selecting the best pipe material for your specific application. They can help you understand the advantages and disadvantages of different materials and guide you through the installation process.
Timely Supply: A good distributor will ensure that you have access to the pipes and materials you need, when you need them. Timely supply is critical for solar projects, where delays in sourcing materials can lead to extended installation times and increased costs.
Custom Solutions: Depending on the size and complexity of your solar system, you may require custom piping solutions. Reputable distributors often work closely with manufacturers to provide tailored products that meet the unique requirements of your system.
Working with Pipes for Solar System Works Distributors in Gujarat and Vadodara
In regions like Gujarat, where solar energy adoption is on the rise, finding a trusted pipes for solar system works distributor in Gujarat or Vadodara is essential. Gujarat, known for its abundant sunlight and progressive solar policies, has seen significant growth in the installation of both residential and commercial solar systems.
Distributors in these areas understand the unique demands of solar projects in the region and can provide the necessary materials to ensure optimal system performance. Whether you are installing a small domestic solar system or a large-scale industrial project, working with a local distributor ensures that you have access to the right products at competitive prices, backed by knowledgeable support and guidance.
Conclusion
When it comes to the efficiency and longevity of solar thermal systems, pipe material plays a crucial role. The right choice of pipes can enhance heat transfer, prevent energy losses, and ensure long-lasting performance, while the wrong choice can result in inefficiencies and costly repairs. By carefully considering the factors that impact pipe performance—such as thermal conductivity, temperature resistance, and durability—you can make an informed decision that maximizes the benefits of your solar system.
Working with reliable pipes for solar system works distributors in Vadodara and Gujarat ensures that you receive high-quality products and expert support tailored to your project’s needs. As solar energy continues to grow in importance, paying attention to the materials used in solar systems will help ensure their efficiency and success for years to come.
#Pipes for solar system works distributors in Gujarat#Pipes for solar system works distributors in Vadodara#Pipes for solar system works
4 notes
·
View notes
Text
Revolutionizing Industrial Heating With Thermal Fluid Heaters
Thermodyne's Thermal Fluid Heaters Efficiency, innovation, and reliability — all seamlessly integrated into our cutting-edge heating systems. Trust Thermodyne Engineering for unparalleled thermal solutions that set the standard in the industry. Designed for precision and efficiency, our heaters stand at the forefront of heat transfer technology. Experience seamless heat management as Thermodyne's Thermal Fluid Heaters excel in providing consistent and reliable performance. Thermodyne's commitment to quality shines through, guaranteeing robust and durable solutions that elevate your processes. For more information contact us at https://www.thermodyneboilers.com/thermic-fluid-heaters/
#Thermal Fluid Heaters#Efficient Boiler Systems#Thermal Solutions#Thermal Fluid Technology#Heat Transfer Solutions
0 notes
Text
Elements
All magic in the Next World falls under a certain elemental bias. Elements are odd though, for many reasons. Most notably, despite there only being seven magical elements, they are incredibly broad, and very abstract. There is no “fire” or “plant” or “water”, but rather elements that encompass those uses, as well as unimaginable amounts of others. Additionally, certain elements are very non-standard, and direct the usage of abilities not commonly seen in magic systems.
Toyhou.se information profile here! More up to date and will be updated.
However, even with the broad range in each element, an item or individual with an elemental affinity cannot manipulate any concept in an element’s range. Rather, actual usage of an element must be precise, and will inherently be channeled differently between users or devices. Two users of the same element are unlikely to have remotely similar magic abilities.
As mentioned prior, there are seven elements of magic:
Thermal is the element of temperatures, heat and cold, the properties of temperatures, and concepts heavily linked with temperatures.
Geologic is the element of rocks, minerals, metals and crystals, as the direct material, their properties, or common uses.
Power is the element of energy, as the usage of energy, creation of energy, and types of energy.
Organic is the element of living things and compounds, in the form of aspects of living things, organic materials, or associated properties.
Fluid is the element of liquids, properties of liquids, or liquid-like states.
Force is the element of unseen forces, in the form of physical forces and their effects, or conceptual forces and their effects.
Artificial is the element of manmade things, both physical and conceptual.
Every Mirribeast has one elemental association, and a specific usage of this element. As far as the element system goes, their magic usage is completely normal, minus their ability to use it on their own.
9 notes
·
View notes
Text

When the SR-71 was getting low on gas, its crew members pressure suits started to warm up. So aboard the Blackbird the fuel was used as a heat sink. Here’s how.
SR-71 Blackbird crew members have said that they sometimes came down looking for a tanker, not so much because they were running out of gas but because their gas was getting too hot.
My Dad, Colonel Richard “Butch” Sheffield, SR-71 Blackbird Reconnaissance Systems Officer (RSO), wrote in his unpublished book “The Very First” that when they were getting low on gas in the SR-71, Blackbird crew members pressure suits started to get a little warm up. By contrast bringing on new fuel cooled them down. Aboard the SR-71 the fuel was used as a heat sink.
Everything about the SR-71 was complex yet incredibly engineered, so they have to find a way for the Blackbird to deal with the enormous amount of heat generated by its high-speed flight.
‘Flying at over Mach 3 is a thermal problem. Everything is too hot, including any air you slow down to interact with the vehicle. You are trying to make the vehicle (and the pilots inside) survive for hours in a pizza oven, while they are getting cozy with two 500 million BTU/hour flamethrowers,’ Iain McClatchie, an aviation and turbine engine expert, says on Quora.
When the SR-71 was getting low on gas, its crew members pressure suits started to warm up. So aboard the Blackbird the fuel was used as a heat sink. Here’s how.
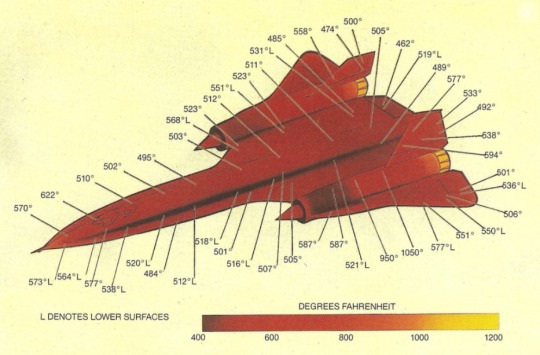
‘When you look at a graph like this, your first impression might be that the vehicle is this glowing hot thing slicing through the icy -52 C air at 80,000 feet. So naturally, you think of the air as cooling the airplane down.
‘Not so much. The air has to change to the vehicle’s speed to touch the vehicle, and that requires work. That work heats the air. At Mach 3.2, the stagnation temperature of the air is 740 F, which is hotter than every (labelled) point on the above graph! (The nacelles around the engine afterburners, unlabelled, are in fact hotter than the air around them.)
‘I know, it seems unbelievable. Use this handy graph and see for yourself.
When the SR-71 was getting low on gas, its crew members pressure suits started to warm up. So aboard the Blackbird the fuel was used as a heat sink. Here’s how.
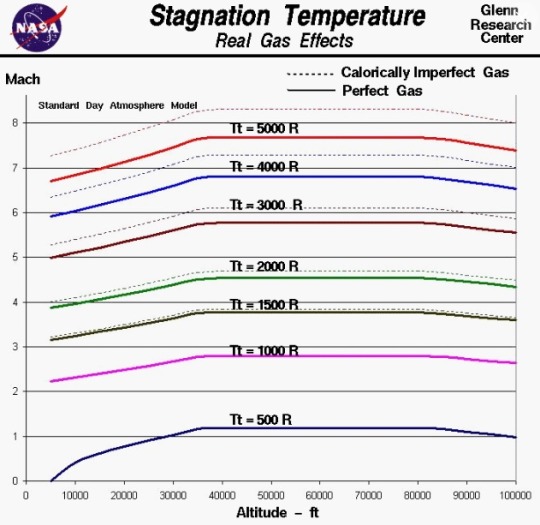
‘Basically, the shocks from the airplane heat the air around it, but the vehicle itself cools the air in contact with it down. Once the airplane passes by, all that disturbed air tumbles to a stop, leaving a path of hot air through the upper atmosphere.
‘So back to life in the pizza oven. The basic solution is (a) leave most of the airframe hot and make it out of stuff like titanium and stainless steel that are strong when hot, and (b) start with a large amount of cold fuel, and then dump heat from critical areas into the fuel before burning it. When decoupling from an aerial tanker, half the SR-71’s weight was fuel.
‘A special type of kerosene fuel, JP-7, was developed for the SR-71 to be good as a heat sink. It boils away at 285 C at 1 atmosphere pressure, which is the upper end of the kerosene range. When the plane tanked up at 30,000 feet, the kerosene might start below 0 C. At speed, it would be used to cool the avionics and cockpit, and by the time it arrived at the engine it would get up to 177 C. It was then used as hydraulic fluid for the various engine actuators, primarily the variable geometry nozzle. By the time it got to the fuel injectors it had gotten up to 316 C (but wasn’t boiling because it was at several atmospheres of pressure). At cruise the burner cans were at 330 kPa (about 3.3x the pressure at sea level), so the fuel still didn’t boil as it left the nozzles but the droplets would have evaporated very quickly.’
When the SR-71 was getting low on gas, its crew members pressure suits started to warm up. So aboard the Blackbird the fuel was used as a heat sink. Here’s how.
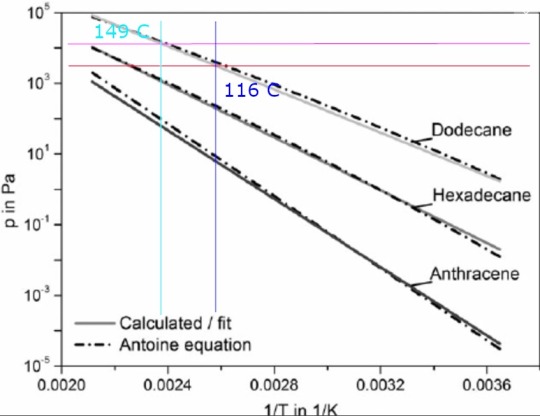
McClatchie continues;
‘JP-7 is mostly a mix of hydrocarbons centered around C12H26 (dodecane). The graph above shows the vapor pressure of dodecane as a function of reciprocal absolute temperature. That makes it a bit hard to read. 0.0024, for instance, is 417 Kelvin which is 143 Celsius. Liquids start to boil when their vapor pressure is greater than the ambient pressure. I’ve labelled the boiling point of dodecane at 2900 Pa, which is the absolute pressure at 80,000 feet, and 13000 Pa, which is the minimum absolute pressure in the SR-71 fuel tanks. Note that the dodecane component of JP-7 starts to boil at 162 C at sea level… quite a bit less than the advertised 285 C which is actually when the stuff boils away completely.
‘The flash point of JP-7 is 60 C. The fuel was held in tanks whose walls were formed of the skin of the vehicle. Since fuel vapor against the top skin of the vehicle would be well over 60 C during cruise, if air was allowed in any ignition source in the tank would cause a deflagration and destruction of the vehicle. Instead, nitrogen gas from a 260-liter liquid nitrogen dewar was used to pressurize the tanks. This would have mostly been an issue during descent, when the ambient pressure rose and extra gas was needed to fill the tank ullage space.
‘But nitrogen gas fill was not enough. The fuel was heated in the tank by the bottom surface of the vehicle, just as water in a pot is heated by the flame on a stove. In this case the fluid was over a meter deep in the tank and consequently took longer than a pot does to boil. At ambient cruise pressure the fuel would have begun to simmer in the tanks at 116 C. By pressurizing the tank to 10 kPa over ambient, the tolerable tank temperature rose by 33 C. This temperature limit put a time limit on how long the SR-71 could stay at cruise before it began to lose fuel to boiling.
Blackbird Pilot explains why the SR-71 had to refuel right after takeoff (and it’s not because it leaked fuel)

‘The fuel pumps in the tanks raised the fuel pressure so that boiling was no longer a problem once in the fuel system. The limit of how much heat could be absorbed by the fuel was rather set by it’s coking temperature — the temperature at which the fuel begins to deposit varnish on the interior of whatever plumbing it is in. I don’t have a specific number on JP-7, but it must be higher than 316 C. There was another experimental hydrocarbon blend developed, called JP-900, which resists coking up to 482 C. This was intended for a higher speed vehicle that was never built.
‘They were not able to get the wind tunnel behavior to match the actual behavior of the airplane. Kelly Johnson speculated that this was because during cruise, the fuel sitting against the lower skin of the fuselage and inner wing kept that portion of the airframe cooler than the upper skin. This caused the vehicle to bow from the differential temperature expansion, which would have made the wings slightly anhedral and would have made the vehicle unstable in roll.’
McClatchie concludes;

SR-71 print
This print is available in multiple sizes from AircraftProfilePrints.com – CLICK HERE TO GET YOURS. SR-71A Blackbird 61-7972 “Skunkworks”
‘Heating of the fuel while in the tanks caused yet another problem. As I said earlier, the engines can take the fuel at a maximum temperature of 177 C. So as the fuel in the tanks heats up, it’s ability to absorb heat on the way to the engine decreases. Flight crews have said that they sometimes came down looking for a tanker, not so much because they were running out of gas but because their gas was getting too hot.
‘Using fuel as a heat sink is common in fast jets. The Concorde did it, the F-15, F-16, F-18, F-22, and F-35 do it, and probably all other supersonic aircraft.’
Be sure to check out Linda Sheffield Miller (Col Richard (Butch) Sheffield’s daughter, Col. Sheffield was an SR-71 Reconnaissance Systems Officer) Facebook Pages Habubrats SR-71 and Born into the Wilde Blue Yonder for awesome Blackbird’s photos and stories.
8 notes
·
View notes
Text


Nuclear electric propulsion technology could make missions to Mars faster
The trip to Mars and back is not one for the faint of heart. We're not talking days, weeks, or months. But there are technologies that could help transport a crew on that round-trip journey in a relatively quick two years.
One option NASA is exploring is nuclear electric propulsion, which employs a nuclear reactor to generate electricity that ionizes, or positively charges, and electrically accelerates gaseous propellants to provide thrust to a spacecraft.
Researchers at NASA's Langley Research Center in Hampton, Virginia, are working on a system that could help bring nuclear electric propulsion one significant, technology-defining step closer to reality.
Modular Assembled Radiators for Nuclear Electric Propulsion Vehicles, or MARVL, aims to take a critical element of nuclear electric propulsion, its heat dissipation system, and divide it into smaller components that can be assembled robotically and autonomously in space.
"By doing that, we eliminate trying to fit the whole system into one rocket fairing," said Amanda Stark, a heat transfer engineer at NASA Langley and the principal investigator for MARVL. "In turn, that allows us to loosen up the design a little bit and really optimize it."
Loosening up the design is key, because as Stark mentioned, previous ideas called for fitting the entire nuclear electric radiator system under a rocket fairing, or nose cone, which covers and protects a payload. Fully deployed, the heat dissipating radiator array would be roughly the size of a football field. You can imagine the challenge engineers would face in getting such a massive system folded up neatly inside the tip of a rocket.
The MARVL technology opens a world of possibilities. Rather than cram the whole system into an existing rocket, this would allow researchers the flexibility to send pieces of the system to space in whatever way would make the most sense, then have it all assembled off the planet.
Once in space, robots would connect the nuclear electric propulsion system's radiator panels, through which a liquid metal coolant, such as a sodium-potassium alloy, would flow.
While this is still an engineering challenge, it is exactly the kind of engineering challenge in-space-assembly experts at NASA Langley have been working on for decades. The MARVL technology could mark a significant first milestone. Rather than being an add-on to an existing technology, the in-space assembly component will benefit and influence the design of the very spacecraft it would serve.
"Existing vehicles have not previously considered in-space assembly during the design process, so we have the opportunity here to say, 'We're going to build this vehicle in space. How do we do it? And what does the vehicle look like if we do that?' I think it's going to expand what we think of when it comes to nuclear propulsion," said Julia Cline, a mentor for the project in NASA Langley's Research Directorate, who led the center's participation in the Nuclear Electric Propulsion tech maturation plan development as a precursor to MARVL. That tech maturation plan was run out of the agency's Space Nuclear Propulsion project at Marshall Space Flight Center in Huntsville, Alabama.
NASA's Space Technology Mission Directorate awarded the MARVL project through the Early Career Initiative, giving the team two years to advance the concept. Stark and her teammates are working with an external partner, Boyd Lancaster, Inc., to develop the thermal management system. The team also includes radiator design engineers from NASA's Glenn Research Center in Cleveland and fluid engineers from NASA's Kennedy Space Center in Florida. After two years, the team hopes to move the MARVL design to a small-scale ground demonstration.
The idea of robotically building a nuclear propulsion system in space is sparking imaginations.
"One of our mentors remarked, "This is why I wanted to work at NASA, for projects like this,'" said Stark, "which is awesome because I am so happy to be involved with it, and I feel the same way."
Additional support for MARVL comes from the agency's Space Nuclear Propulsion project. The project's ongoing effort is maturing technologies for operations around the moon and near-Earth exploration, deep space science missions, and human exploration using nuclear electric propulsion and nuclear thermal propulsion.
TOP IMAGE: Modular Assembled Radiators for Nuclear Electric Propulsion Vehicles, or MARVL, aims to take a critical element of nuclear electric propulsion, its heat dissipation system, and divide it into smaller components that can be assembled robotically and autonomously in space. This is an artist’s rendering of what the fully assembled system might look like. Credit: NASA
LOWER IMAGE: An artist’s rendering that shows the different components of a fully assembled nuclear electric propulsion system. Credit: NASA
2 notes
·
View notes