#Platinum Mesh Electrode
Explore tagged Tumblr posts
Text
How Electrochemical Flow Cells Optimize Energy Storage
In the world of electrochemistry, precision, and innovation are paramount. Two tools that have transformed the field are the Platinum Mesh Electrode and the Electrochemical Flow Cell. Together, these components enable researchers and industrial players to conduct groundbreaking experiments, optimize processes, and achieve consistent results.
0 notes
Text
A smart second skin gets all the power it needs from sweat
by Wei Gao

The Research Brief is a short take about interesting academic work.
The big idea
Skin is the largest organ of the human body. It conveys a lot of information, including temperature, pressure, pleasure and pain. Electronic skin (e-skin) mimics the properties of biological skin. Recently developed e-skins are capable of wirelessly monitoring physiological signals. They could play a crucial role in the next generation of robotics and medical devices.
My lab at Caltech is interested in studying human biology and monitoring human health by using advanced bioelectronic devices. The e-skin we have developed not only analyzes the chemical and molecular composition of human sweat, it’s fully powered by chemicals in sweat.
Why it matters
Existing e-skins and wearable devices primarily focus on monitoring physiological parameters like heart rate and can’t assess health information at the molecular level. Moreover, they typically require batteries to power them, and the batteries need to be recharged frequently.
Despite recent efforts to harvest energy from the human body, there are no reports of self-powered e-skins that are able to perform biosensing and transmit the information via standard Bluetooth wireless communications. This comes down to the lack of power efficiency. There is a need for a self-powered device that can continuously collect molecular as well as physical information and wirelessly transmit the information to other devices.
How we do this work
The approach we take to harvesting energy from the human body is based on biofuel cells. Fuel cells convert chemical energy to electricity. The biofuel cells we developed for our e-skin convert the lactic acid in human sweat to electricity. In addition to the biofuel cells, the e-skin contains biosensors that can analyze metabolic information like glucose, urea and pH levels, to monitor for diabetes, ischaemia another health conditions, as well as physical information like skin temperature. The e-skin, made of soft materials and attached to a person’s skin, performs real-time biosensing, powered solely by sweat.

The sweat-powered biofuel cells in this electronic skin provide enough electricity to power biological sensors and transmit the information wirelessly to other devices. Yu et al., Sci. Robot. 5, eaaz7946 (2020)
Previously developed wearable biofuel cells don’t produce a lot of power and aren’t very stable. We greatly improved the power output and stability of the biofuel cells by using novel nanomaterials for the cell’s two electrodes. The cathode of our biofuel cell is composed of a mesh of carbon nanotubes decorated with nanoparticles containing platinum and cobalt. The anode is a nanocomposite material that contains an enzyme that breaks down lactic acid.
The biofuel cells can generate a continuous, stable output as high as several milliwatts per square centimeter over multiple days in human sweat. That’s enough to power the biosensors as well as wireless communication. We demonstrated our e-skin by monitoring glucose, pH, ammonium ions and urea levels in studies using human subjects. We also used our e-skin as a human-machine interface to control the motion of a robotic arm and a prosthetic leg.
What’s next
We plan to further improve the power output of the biofuel cells and integrate different biosensors. The development of fully self-powered e-skin opens the door to numerous robotic and wearable health care possibilities. Wearable sensor arrays could be used for health monitoring, early disease diagnosis and potentially nutritional intervention. In addition, self-powered e-skin could be used to design and optimize next generation prosthetics.
About The Author:
Wei Gao is Assistant Professor of Medical Engineering at California Institute of Technology
This article is republished from our content partners over at The Conversation under a Creative Commons license.
15 notes
·
View notes
Photo
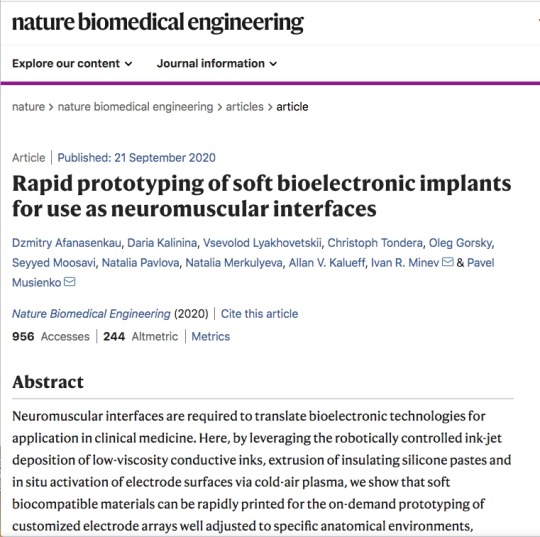
https://www.nature.com/articles/s41551-020-00615-7
References
Wagner, F. B. et al. Targeted neurotechnology restores walking in humans with spinal cord injury. Nature 563, 65–71 (2018).
CAS PubMed Google Scholar
Granata, G. et al. Phantom somatosensory evoked potentials following selective intraneural electrical stimulation in two amputees. Clin. Neurophysiol. 129, 1117–1120 (2018).
PubMed Google Scholar
Ajiboye, A. B. et al. Restoration of reaching and grasping movements through brain-controlled muscle stimulation in a person with tetraplegia: a proof-of-concept demonstration. Lancet 389, 1821–1830 (2017).
PubMed PubMed Central Google Scholar
Liu, Y. et al. Soft and elastic hydrogel-based microelectronics for localized low-voltage neuromodulation. Nat. Biomed. Eng. 3, 58–68 (2019).
CAS PubMed Google Scholar
Minev, I. R. et al. Electronic dura mater for long-term multimodal neural interfaces. Science 347, 159–163 (2015).
CAS PubMed Google Scholar
Fu, T.-M., Hong, G., Viveros, R. D., Zhou, T. & Lieber, C. M. Highly scalable multichannel mesh electronics for stable chronic brain electrophysiology. Proc. Natl Acad. Sci. USA 114, E10046–E10055 (2017).
CAS PubMed Google Scholar
Boutry, C. M. et al. A stretchable and biodegradable strain and pressure sensor for orthopaedic application. Nat. Electron. 1, 314–321 (2018).
Google Scholar
Kim, T.-i et al. Injectable, cellular-scale optoelectronics with applications for wireless optogenetics. Science 340, 211–216 (2013).
CAS PubMed PubMed Central Google Scholar
Reeder, J. et al. Mechanically adaptive organic transistors for implantable electronics. Adv. Mater. 26, 4967–4973 (2014).
CAS PubMed Google Scholar
Lu, C. et al. Flexible and stretchable nanowire-coated fibers for optoelectronic probing of spinal cord circuits. Sci. Adv. https://doi.org/10.1126/sciadv.1600955 (2017).
Sengeh, D. M. & Herr, H. A variable-impedance prosthetic socket for a transtibial amputee designed from magnetic resonance imaging data. J. Prosthet. Orthot. 25, 129–137 (2013).
Google Scholar
Filardo, G. et al. Novel alginate biphasic scaffold for osteochondral regeneration: an in vivo evaluation in rabbit and sheep models. J. Mater. Sci. Mater. Med. 29, 74 (2018).
PubMed Google Scholar
Ploch, C. C., Mansi, C. S., Jayamohan, J. & Kuhl, E. Using 3D printing to create personalized brain models for neurosurgical training and preoperative planning. World Neurosurg. 90, 668–674 (2016).
PubMed Google Scholar
Valentine, A. D. et al. Hybrid 3D printing of soft electronics. Adv. Mater. 29, 1703817 (2017).
Google Scholar
Lind, J. U. et al. Instrumented cardiac microphysiological devices via multimaterial three-dimensional printing. Nat. Mater. 16, 303–308 (2017).
CAS PubMed Google Scholar
Bachmann, B. et al. All-inkjet-printed gold microelectrode arrays for extracellular recording of action potentials. Flex. Print. Electron. 2, 035003 (2017).
Google Scholar
Athanasiadis, M. et al. Printed elastic membranes for multimodal pacing and recording of human stem-cell-derived cardiomyocytes. npj Flex. Electron. https://doi.org/10.1038/s41528-020-0075-z (2020).
Minev, I. R., Wenger, N., Courtine, G. & Lacour, S. P.Research update: platinum-elastomer mesocomposite as neural electrode coating.APL Mater. 3, 014701 (2015).
Google Scholar
Mo, L. et al. Nano-silver ink of high conductivity and low sintering temperature for paper electronics. Nanoscale Res. Lett. 14, 197 (2019).
PubMed PubMed Central Google Scholar
Van Noort, R., Black, M. M., Martin, T. R. P. & Meanley, S. A study of the uniaxial mechanical properties of human dura mater preserved in glycerol. Biomaterials 2, 41–45 (1981).
CAS PubMed Google Scholar
Kwan, M. K., Wall, E. J., Massie, J. & Garfin, S. R. Strain, stress and stretch of peripheral nerve rabbit experiments in vitro and in vivo. Acta Orthop. Scand. 63, 267–272 (1992).
CAS PubMed Google Scholar
Calvo, B. et al. Passive nonlinear elastic behaviour of skeletal muscle: experimental results and model formulation. J. Biomech. 43, 318–325 (2010).
CAS PubMed Google Scholar
Nicholson, K. J. & Winkelstein, B. A. in Neural Tissue Biomechanics (ed. Bilston, L. E.) 203–229 (Springer, 2011).
Harrison, D. E., Cailliet, R., Harrison, D. D., Troyanovich, S. J. & Harrison, S. O. A review of biomechanics of the central nervous system—part II: spinal cord strains from postural loads. J. Manipulative Physiol. Ther. 22, 322–332 (1999).
CAS PubMed Google Scholar
Diani, J., Fayolle, B. & Gilormini, P. A review on the Mullins effect. Eur. Polym. J. 45, 601–612 (2009).
CAS Google Scholar
Neto, J. P. et al. Does impedance matter when recording spikes with polytrodes? Front. Neurosci. https://doi.org/10.3389/fnins.2018.00715 (2018).
Cogan, S. F. Neural stimulation and recording electrodes. Annu. Rev. Biomed. Eng. 10, 275–309 (2008).
CAS PubMed Google Scholar
Biegler, T. An electrochemical and electron microscopic study of activation and roughening of platinum electrodes. J. Electrochem. Soc. 116, 1131 (1969).
CAS Google Scholar
Tondera, C. et al. Highly conductive, stretchable, and cell-adhesive hydrogel by nanoclay doping. Small 15, 1901406 (2019).
Google Scholar
Won, S. M. et al. Recent advances in materials, devices, and systems for neural interfaces. Adv. Mater. 30, 1800534 (2018).
Google Scholar
Capogrosso, M. et al. A brain–spine interface alleviating gait deficits after spinal cord injury in primates. Nature 539, 284–288 (2016).
PubMed PubMed Central Google Scholar
Musienko, P. E. et al. Spinal and supraspinal control of the direction of stepping during locomotion. J. Neurosci. 32, 17442–17453 (2012).
CAS PubMed PubMed Central Google Scholar
Harkema, S. et al. Effect of epidural stimulation of the lumbosacral spinal cord on voluntary movement, standing, and assisted stepping after motor complete paraplegia: a case study. Lancet 377, 1938–1947 (2011).
PubMed PubMed Central Google Scholar
Courtine, G. et al. Transformation of nonfunctional spinal circuits into functional states after the loss of brain input. Nat. Neurosci. 12, 1333–1342 (2009).
CAS PubMed PubMed Central Google Scholar
Wenger, N. et al. Spatiotemporal neuromodulation therapies engaging muscle synergies improve motor control after spinal cord injury. Nat. Med. 22, 138–145 (2016).
CAS PubMed PubMed Central Google Scholar
Hoffman, P.Beitrage zur kenntnis der menschlichen reflexe mit besonderer berucksichtigung der elektrischen erscheinungen.Arch. F. Physiol. 1, 223–256 (1910).
Google Scholar
Shik, M. L. Control of walking and running by means of electrical stimulation of the midbrain. Biofizika 11, 659–666 (1966).
CAS PubMed Google Scholar
Fry, C. H., Wu, C. & Sui, G. P. Electrophysiological properties of the bladder. Int. Urogynecol. J. 9, 291–298 (1998).
CAS Google Scholar
Liu, D. W. & Westerfield, M. Function of identified motoneurones and co-ordination of primary and secondary motor systems during zebra fish swimming. J. Physiol. 403, 73–89 (1988).
CAS PubMed PubMed Central Google Scholar
Hains, B. C. & Waxman, S. G. Activated microglia contribute to the maintenance of chronic pain after spinal cord injury. J. Neurosci. 26, 4308–4317 (2006).
CAS PubMed PubMed Central Google Scholar
Sierra, A. et al. The “Big-Bang” for modern glial biology: translation and comments on Pío del Río-Hortega 1919 series of papers on microglia.Glia 64, 1801–1840 (2016).
PubMed Google Scholar
Musienko, P. et al. Somatosensory control of balance during locomotion in decerebrated cat. J. Neurophysiol. 107, 2072–2082 (2012).
PubMed PubMed Central Google Scholar
Nonnekes, J. et al. Neurological disorders of gait, balance and posture: a sign-based approach. Nat. Rev. Neurol. 14, 183–189 (2018).
PubMed Google Scholar
Musienko, P. E. et al. Neuronal control of posture and locomotion in decerebrated and spinalized animals. Ross. Fiziol. Zh. Im. I. M. Sechenova 99, 392–405 (2013).
CAS PubMed Google Scholar
Gill, M. L. et al. Neuromodulation of lumbosacral spinal networks enables independent stepping after complete paraplegia. Nat. Med. https://doi.org/10.1038/s41591-018-0175-7 (2018).
Kim, Y. et al. A bioinspired flexible organic artificial afferent nerve. Science 360, 998–1003 (2018).
CAS PubMed Google Scholar
Vu, P. P. et al. A regenerative peripheral nerve interface allows real-time control of an artificial hand in upper limb amputees. Sci. Transl. Med. 12, eaay2857 (2020).
PubMed Google Scholar
Vachicouras, N. et al. Microstructured thin-film electrode technology enables proof of concept of scalable, soft auditory brainstem implants. Sci. Transl. Med. 11, eaax9487 (2019).
PubMed Google Scholar
Borton, D. et al. Corticospinal neuroprostheses to restore locomotion after spinal cord injury. Neurosci. Res. 78, 21–29 (2014).
PubMed Google Scholar
Athanasiadis, M., Pak, A., Afanasenkau, D. & Minev, I. R. Direct writing of elastic fibers with optical, electrical, and microfluidic functionality. Adv. Mater. Technol. 4, 1800659 (2019).
CAS Google Scholar
Hudak, E. M., Mortimer, J. T. & Martin, H. B. Platinum for neural stimulation: voltammetry considerations. J. Neural Eng. 7, 026005 (2010).
CAS Google Scholar
Whelan, P. J. Control of locomotion in the decerebrate cat. Prog. Neurobiol. 49, 481–515 (1996).
CAS PubMed Google Scholar
Shik, M. L. & Orlovsky, G. N. Neurophysiology of locomotor automatism. Physiol. Rev. 56, 465–501 (1976).
CAS PubMed Google Scholar
Mori, S., Kawahara, K., Sakamoto, T., Aoki, M. & Tomiyama, T. Setting and resetting of level of postural muscle tone in decerebrate cat by stimulation of brain stem. J. Neurophysiol. 48, 737–748 (1982).
CAS PubMed Google Scholar
Iwahara, T., Atsuta, Y., Garcia-Rill, E. & Skinner, R. D. Spinal cord stimulation-induced locomotion in the adult cat. Brain Res. Bull. 28, 99–105 (1992).
CAS PubMed Google Scholar
Gerasimenko, Y. P. et al. Formation of locomotor patterns in decerebrate cats in conditions of epidural stimulation of the spinal cord. Neurosci. Behav. Physiol. 35, 291–298 (2005).
PubMed Google Scholar
Merkulyeva, N. et al. Activation of the spinal neuronal network responsible for visceral control during locomotion. Exp. Neurol. 320, 112986 (2019).
PubMed Google Scholar
Kruse, M. N. & de Groat, W. C. Spinal pathways mediate coordinated bladder/urethral sphincter activity during reflex micturition in decerebrate and spinalized neonatal rats. Neurosci. Lett. 152, 141–144 (1993).
CAS PubMed Google Scholar
Shefchyk, S. J. & Buss, R. R. Urethral pudendal afferent-evoked bladder and sphincter reflexes in decerebrate and acute spinal cats. Neurosci. Lett. 244, 137–140 (1998).
CAS PubMed Google Scholar
Gerasimenko, Y. et al. Propriospinal bypass of the serotonergic system that can facilitate stepping. J. Neurosci. 29, 5681–5689 (2009).
CAS PubMed PubMed Central Google Scholar
Westerfield, M. The Zebrafish Book. A Guide for the Laboratory Use of Zebrafish (Danio rerio) 4th edn (Univ. Oregon Press, 2000).
Martins, T., Valentim, A. M., Pereira, N. & Antunes, L. M. Anaesthesia and analgesia in laboratory adult zebrafish: a question of refinement. Lab. Anim. 50, 476–488 (2016).
CAS PubMed Google Scholar
Capogrosso, M. et al. Configuration of electrical spinal cord stimulation through real-time processing of gait kinematics. Nat. Protoc. 13, 2031–2061 (2018).
CAS PubMed Google Scholar
Jorfi, M., Skousen, J. L., Weder, C. & Capadona, J. R. Progress towards biocompatible intracortical microelectrodes for neural interfacing applications. J. Neural Eng. 12, 011001 (2014).
PubMed PubMed Central Google Scholar
Kirik, O. V., Sukhorukova, E. G. & Korzhevskiĭ, D. E. Calcium-binding Iba-1/AIF-1 protein in rat brain cells. Morfologiia 137, 5–8 (2010).
CAS PubMed Google Scholar
Schindelin, J. et al. Fiji: an open-source platform for biological-image analysis. Nat. Methods 9, 676–682 (2012).
CAS Google Scholar
Kreutzberg, G. W. Microglia: a sensor for pathological events in the CNS. Trends Neurosci. 19, 312–318 (1996).
CAS PubMed Google Scholar
Refolo, V. & Stefanova, N. Neuroinflammation and glial phenotypic changes in alpha-synucleinopathies. Front. Cell. Neurosci. https://doi.org/10.3389/fncel.2019.00263 (2019).
Pistohl, T., Schulze-Bonhage, A., Aertsen, A., Mehring, C. & Ball, T. Decoding natural grasp types from human ECoG. NeuroImage 59, 248–260 (2012).
PubMed Google Scholar
2 notes
·
View notes
Text
Some Interesting Facts About Water
Water (in Latin, Aqua) is a substance whose molecule consists of two atoms of hydrogen and one of oxygen (H2O). It is essential for the survival of all known forms of life. From the physical point of view, water is constantly circulated in a cycle of evaporation or transpiration (evapotranspiration), precipitation and flowing back to the sea.
The total amount of water on the planet in all its forms is called the hydrosphere and covers three quarters (71%) of the surface of the Earth. Water can be found almost anywhere in the biosphere and in the substance’s three states: solid, liquid and gas.
The human body is composed of 60% water, depending on height and build. In children the figure is 70 or 80%, in adults 60% and in elderly persons it is 50%. The recommended daily water intake varies depending on level of activity, temperature, humidity and other factors, although a majority of experts believe a minimum of between 6 and 9 glasses (1.5 L – 2 L) of mineral water is needed daily to maintain adequate hydration. Health officials are glad to see more water going down our throats because caffeinated soft drinks, coffee and alcohol all suck more water from our bodies than they provide.
Specifications of SWAFE Alkaline Antioxidant Healing Structured Water Ionizer 9- Plates
Electrodes- 9 plates (titanium dipped in platinum)
UV light- UV sterilization system for Antibacterial and safe drinking water.
Electrode- Flat advanced MESH Electrode
Power System- SMPS (switched mode power supply)
Power Consumption- Adjustable up to 450 Watts - (61 adjustable levels)
Rated Voltage- 110V / 220V
Settings- 4 Alkaline / 1 Purified / 2 Acidic
pH Output- up to 11.3*
ORP Output- up to -800
Filtration- Dual Internal + Custom Pre-Filter
Cleaning Function- Advanced RADC Cleaning System
Display- Full Color LED Display w/ Automatic Voice Prompting
Flow Control Automatic
Protection Automatic Heat Sensor
Installation Under Counter / Countertop
0 notes
Text
5 things you didn’t know about... floating solar fuels rig

Credit: Jack Davis/Columbia Engineering. Side view of bubbles evolved from two symmetric electrodes with a catalyst coated on both sides. A large mixed-composition (H2 + O2) bubble is seen to bridge the two electrodes.
1. Solar-powered water electrolysis could produce hydrogen for use as a fuel.
2. Researchers at Columbia Engineering, USA, have taken advantage of this technique, developing a stand-alone photovoltaic-powered electrolysis device that floats on water – known as a solar fuels rig.
3. Using solar power and water, the rig could produce clean fuel, without requiring fresh water or land.
4. Key to the development of this device is the absence of conventionally used expensive membranes in the electrolyser. In this device, they are separated and collected using electrode configuration and the buoyancy of the bubbles.
5. The device comprises porous mesh electrodes coated, on one side only, with a platinum electrocatalyst.
To find out more visit see our upcoming February issue.
To read Floating membraneless PV-electrolyzer based on buoyancy-driven product separation visit bit.ly/2A5rMBa
79 notes
·
View notes
Link
Platinum Plated Titanium Anode Mesh for Rhodium Plating Bath ...
0 notes
Text
Artificial Kidneys Are a Step Closer With This New Tech

10 percent of the global population suffers from some form of kidney disease. That includes 37 million people in the US, 100,000 of whom pass away each year awaiting a kidney transplant.
Our kidneys are crucial for keeping us alive and healthy. A sort of chemical computer that keeps our blood chemistry stable—whether we’re eating a sugary birthday cake or a vitamin-filled salad—they prevent waste buildup, stabilize our electrolyte levels, and produce hormones to regulate our blood pressure and make red blood cells.
Kidneys clean our blood using nephrons, which are essentially filters that let fluid and waste products through while blocking blood cells, proteins, and minerals. The latter get reintegrated into the blood, and the former leave the body in urine.
Scientists have struggled to come up with viable treatments for kidney disease and renal failure, and their complexity means kidneys are incredibly hard to synthetically recreate; each kidney contains around one million intricately-structured nephrons.
But new progress from chemical engineering researchers at the University of Arkansas has brought functioning artificial kidneys one step closer. The researchers created a device that was able to filter blood in a way similar to biological nephrons. They described the device in a recent paper published in Nature Communications Materials.
There are two basic processes that take place when blood passes through the kidneys. First, clusters of blood vessels called glomeruli let small molecules, waste, and water through, while proteins and blood cells stay behind. The material that gets through this first filter then flows into the nephron network, where it’s further filtered in a process called ion transport.
The researchers’ work focused on the second step, ion transport. They placed a porous mesh made of platinum between two ion-exchange wafers to create a wafer that pushes ions through membranes using an electric field. The platinum meshes serve as electrodes when voltage is applied, enabling the team to select different ions and adjust their transport rates independently. They tested the technology with various ions and were successfully able to mimic the ion transport done by the kidneys.
In their paper, the team points out that other research groups have tried creating artificial nephrons using living, cell-based systems, including stem cells; but outside a native, living environment and absent the physical and hormonal signaling that control their function, biologically-based systems have struggled to replicate the nephrons’ function, especially ion transport.
Christa Hestekin, Arkansas associate professor of chemical engineering and the lead author of the paper, said, “The system could work as a stand-alone device or in conjunction with peritoneal dialysis to control the chemistry of solutions used in treatment. And, minor modifications to the device could enable it to function as a wearable and potentially implantable artificial kidney.”
In the US alone, over 93,000 people are currently on the waiting list for a kidney transplant. Though a fully-functioning artificial kidney is likely still years away at best, scientists are making incremental progress in recreating this vital organ; an artificial nephron like the one described here is just one piece of a complex puzzle.
Another crucial piece is a functioning network of blood vessels. In 2015, scientists at Lawrence Livermore National Laboratory created bioprinted kidney tissue that replicated some of the functions of biological nephrons. In 2016 a group at Harvard’s Lewis Lab used 3D printing to re-create the nephrons’ tubules, complete with a vascular network for blood flow—but they only stayed alive for a little over two months.
The fully synthetic nature of the Arkansas team’s technology could thus have a leg up on biologically-based approaches. According to Hestekin, the nephron could be combined with ultrafiltration, nanofiltration, or reverse osmosis systems and integrated into an artificial kidney.
Given the vast number of people in need of them, artificial kidneys can’t come soon enough, and will be a miracle of modern science when they do arrive. Though it’ll be some time yet, incremental progress like this gives us the confidence to say “when” instead of “if.”
Image Credit: crystal light / Shutterstock.com
0 notes
Link
“...researchers from Lieber group at Harvard University recently designed a mini mesh probe that looks so much like real neurons that the brain cannot identify the impostors. These bio-inspired electronics consist of platinum electrodes and ultra-thin gold wires encapsulated by a polymer with size and flexibility similar to neuron cell bodies and neural nerve fibres.”
0 notes
Text
CHAPTER 32 — PART 2/3
→ Chromatographic Detectors
Characteristics of the Ideal Detector:
Adequate sensitivity. In general, the sensitivities of present-day detectors lie in the range of 10–8 to 10–15 g solute/s.
Good stability and reproducibility.
A linear response to solutes that extends over several orders of magnitude.
A temperature range from room temperature to at least 4008C.
A short response time that is independent of flow rate.
High reliability and ease of use. To the greatest extent possible, the detector should be foolproof in the hands of inexperienced operators.
Similarity in response toward all solutes or, alternatively, a highly predictable and selective response toward one or more classes of solutes.
Nondestructive of sample.
Flame Ionization Detectors
The flame ionization detector (FID) is the most widely used and generally applicable detector for gas chromatography. The organic compounds are detected by monitoring the current produced by collecting the ions and electrons. A few hundred volts applied between the burner tip and a collector electrode located above the flame serves to collect the ions and electrons. The resulting current (,10–12 A) is then measured with a sensitive picoammeter.
The FID is insensitive toward noncombustible gases, such as H2O, CO2, SO2, and NOx. These properties make the flame ionization detector a most useful general detector for the analysis of most organic samples including those that are contaminated with water and the oxides of nitrogen and sulfur.
The FID exhibits a high sensitivity (,10–13 g/s), large linear response range (,107), and low noise. It is generally rugged and easy to use. This detector has the advantage that changes in flow rate of the mobile phase have little effect on detector response and disadvantages that destroys the sample during the combustion step and requires additional gases and controllers.
Thermal Conductivity Detectors
The thermal conductivity detector (TCD) was one of the earliest detectors for gas chromatography. This device consists of an electrically heated source whose temperature at constant electric power depends on the thermal conductivity of the surrounding gas. The heated element may be a fine platinum, gold, or tungsten wire or, alternatively, a small thermistor. The electrical resistance of this element depends on the thermal conductivity of the gas.
Detection by thermal conductivity is less satisfactory with carrier gases whose conductivities closely resemble those of most sample components. The advantages of the TCD are its simplicity, its large linear dynamic range (about five orders of magnitude), its general response to both organic and inorganic species, and its nondestructive character, which permits collection of solutes after detection. The chief limitation of this detector is its relatively low sensitivity (,10 8 g/s solute/mL carrier gas). Other detectors exceed this sensitivity by factors of 104 to 107. The low sensitivities of TCDs often precludes their use with capillary columns where sample amounts are very small.
Electron Capture Detectors
The electron capture detector (ECD) has become one of the most widely used detectors for environmental samples because this detector selectively responds to halogen-containing organic compounds, such as pesticides and polychlorinated biphenyls. In this detector, the sample eluate from a column is passed over a radioactive b emitter, usually nickel-63. Compounds, such as halogens, peroxides, quinones, and nitro groups, are detected with high sensitivity. The detector is insensitive to functional groups such as amines, alcohols, and hydrocarbons. Compounds, such as halogens, peroxides, quinones, and nitro groups, are detected with high sensitivity.
Electron capture detectors are highly sensitive and have the advantage of not altering the sample significantly (in contrast to the flame ionization detector, which consumes the sample). The linear response of the detector, however, is limited to about two orders of magnitude.
Mass Spectrometry Detectors
One of the most powerful detectors for GC is the mass spectrometer. The combination of gas chromatography and mass spectrometry is known as GC/MS.3 A mass spectrometer measures the mass-to-charge ratio (m/z) of ions that have been produced from the sample. The flow rate from capillary columns is usually low enough that the column output can be fed directly into the ionization chamber of the mass spectrometer. The most common ion sources for GC/MS are electron impact and chemical ionization and the most common mass analyzers are quadrupole and ion-trap analyzers.
A computer data system is needed to process the large amount of data obtained. The data can be analyzed in several way:
o The ion abundance in each spectrum can be summed and plotted as a function of time to give a total-ion chromatogram. o Also, displays the mass spectrum at a time during the chromatogram to identify the species eluting at that time. o A single mass-to-charge (m/z) value can be selected and monitored throughout the chromatographic experiment, a technique known as selected-ion monitoring.
Mass spectrometry can not only determine a peak is due to the more than one component, but it can identify the various unresolved species. GC has also been coupled with tandem mass spectrometers and with Fourier transform mass spectrometers to give GC/MS/MS or GC/MSn systems, which are very powerful tools for identifying components in mixtures.
Typical outputs for a GC/MS system.
LC-MS analysis of an extract from the corpora cardiaca of Ceroplesis capensis. Material from the CC of the cerambycid beetle, Ceroplesis capensis was extracted with 80% methanol and analysed by LC-MS. A The total ion chromatogram B A full scan positive electrospray ionisation (ESI) mass spectrum of the peak shown in ( A ) with a retention time of 5.40 min
Gas Chromatographic Columns and Stationary Phases
→ Capillary Columns (Tubular Column)
2 basic types:
o Wall-coated open tubular (WCOT)- are capillary tubes coated with a thin layer of the liquid stationary phase. Early WCOT columns were constructed of stainless steel, aluminum, copper, or plastic. Subsequently, glass was used.
o Support-coated open tubular (SCOT)- the inner surface of the capillary is lined with a thin film (,30 mm) of a solid support material, such as diatomaceous earth, on which the liquid stationary phase is adsorbed. This type of column holds several times as much stationary phase as does a wall-coated column and thus has a greater sample capacity.
· Fused-silica open tubular (FSOT) columns- Fused-silica capillaries are drawn from specially purified silica that contain minimal amounts of metal oxides. These capillaries have much thinner walls than their glass counterparts. Commercial fused silica columns offer several important advantages over glass columns, such as physical strength, much lower reactivity toward sample components, and flexibility. For most applications, they have replaced the older type WCOT glass columns.
· Megabore columns- Capillary columns with 530 mm inside diameters, tolerate sample sizes that are similar to those for packed columns. The performance characteristics of megabore capillary columns are not as good as those of smaller diameter columns but significantly better than those of packed columns.
→ Packed Columns
Modern packed columns are fabricated from glass or metal tubing and are typically 2 to 3 m long and have inside diameters of 2 to 4 mm. These tubes are densely packed with a uniform, finely divided packing material, or solid support, that is coated with a thin layer (0.05 to 1 mm) of the stationary liquid phase. The tubes are usually formed as coils with diameters of roughly 15 cm so that they can be conveniently placed in a temperature-controlled oven.
o Solid Support Materials- The packing (solid support), in a packed column serves to hold the liquid stationary phase in place so that as large a surface area as possible is exposed to the mobile phase. The ideal support consists of small, uniform, spherical particles with good mechanical strength and a specific surface area of at least 1 m2/g.
o Particle Size of Supports- The efficiency of a gas chromatographic column increases rapidly with decreasing particle diameter of the packing. The pressure difference required to maintain an acceptable flow rate of carrier gas, however, varies inversely as the square of the particle diameter. The usual support particles are 60 to 80 mesh (250 to 170 mm) or 80 to 100 mesh (170 to 149 mm).
→ Liquid Stationary Phases
Desirable properties for the immobilized liquid phase:
1. Low volatility 2. Thermal stability 3. Chemical inertness 4. Solvent characteristics such that k and a value for the solutes to be resolved fall within a suitable range.
The retention time for an analyte on a column depends on its distribution constant, which in turn is related to the chemical nature of the liquid stationary phase. To separate various sample components, their distribution constants must be sufficiently different to accomplish a clean separation.
To have a reasonable residence time on the column, an analyte must show some degree of compatibility (solubility) with the stationary phase. The polarity of a molecule, as indicated by its dipole moment, is a measure of the electric field produced by separation of charge within the molecule. Generally, the polarity of the stationary phase should match that of the sample components. When the match is good, the order of elution is determined by the boiling point of the eluents.
The most widely used stationary phases for both packed and open tubular column gas chromatography in order of increasing polarity. These six liquids can probably provide satisfactory separations for 90% or more of samples.
→ Bonded and Cross-Linked Stationary Phases
The purpose of bonding and cross-linking is to provide a longer lasting stationary phase that can be rinsed with a solvent when the film becomes contaminated.
One way of cross-linking is to incorporate a peroxide into the original liquid. When the film is heated, reaction between the methyl groups in the polymer chains is initiated by a free radical mechanism. The resulting films are less extractable and have considerably greater thermal stability than do untreated films. Cross-linking has also been initiated by exposing the coated columns to gamma radiation.
→ Film Thickness
Commercial columns are available having stationary phases that vary in thickness from 0.1 to 5 mm. Film thickness primarily affects the retentive character and the capacity of a column. Thin films are useful for separating species of low volatility in a reasonable length of time. For most applications with 0.25- or 0.32-mm columns, a film thickness of 0.25 mm is recommended. With megabore columns, 1 to 1.5 mm films are often used. Today, columns with 8-mm films are marketed.
*CHAPTER 24 CONTINUATION ON THE NEXT POST
0 notes
Text
Device simulates filtering and ion transport functions of human kidney
Chemical engineering researchers at the University of Arkansas have developed a device that simulates the blood filtering and ion transport functions of the human kidney. The technology could transform treatment options for people in the final stage of renal disease. "Basically we created a synthetic nephron—the structure that filters blood to disperse nutrients to the body and remove waste material," said Christa Hestekin, associate professor of chemical engineering and principal researcher. "The system could work as a stand-alone device or in conjuction with peritoneal dialysis to control the chemistry of solutions used in treatment. And, minor modifications to the device could enable it to function as a wearable and potentially implantable artificial kidney." Hestekin works on a team including Jamie Hestekin, professor of chemicial engineering; Ira Kurtz, professor of medicine and chief of nephrology at UCLA Health; and several students in the Ralph E. Martin Department of Chemical Engineering at the University of Arkansas. Funded by the US Kidney Research Corporation, the work was published in Communications Materials, a Nature publication. The researchers' system simulates the critical ion transport work of the nephron. As the structural and functional unit of the kidney, the nephron regulates blood chemistry through filtration of the blood that delivers ions and organic molecules to the body before generating urine to be excreted. To simulate tha filtration process, researchers inserted platinum porous meshes between two ion-exchange wafers to create a single electrodeionization wafer that uses an electric field to force ions through membranes. The meshes serve as electrodes when voltage is applied. The mesh electrodes enabled independent control of transport chambers within the device, which in turn allowed researchers to select different ions and adjust transport rates independently. Hestekin's team successfully tested the technology with several physiologically relevant ions, mimicking the specific control of ion transport by the kidney. Combined with ultrafiltration, nanofiltration or reverse osmosis systems, the researchers' technology could be integrated into an artificial kidney, Hestekin said. According to the Centers for Disease Control and Prevention, 37 million people in the United States suffer with some form of chronic kidney disease. Of these, about 700,000 people per year will develop end-stage, renal disease, which requires dialysis or, as a last resort, a kidney transplant. The CDC reports that the average duration of patient survival on dialysis is slightly longer than seven years, and patients generally must wait about 10 years to receive a donated kidney. Roughly 100,000 people die each year while waiting for a kidney transplant. Provided by: University of Arkansas More information: Christa N. Hestekin et al. Simulating nephron ion transport function using activated wafer electrodeionization. Communications Materials (2020). DOI: 10.1038/s43246-020-0016-3 Image Credit: CC0 Public Domain Read the full article
0 notes
Text
Dynamic Solutions: Platinum Mesh Electrodes in Electrochemical Flow Cells
The platinum mesh electrode and electrochemical flow cell are pivotal tools in modern electrochemical research, providing precision and efficiency in diverse applications such as electrocatalysis, energy storage, and environmental monitoring.
0 notes
Text
Floating Solar Rig Produces Hydrogen Fuel
A new device uses solar-powered electrolysis to separate hydrogen from seawater without any membranes or pumps
Illustration: Justin Bui/Columbia Engineering
An artist’s rendering shows a hypothetical “solar fuels rig” floating on the open sea.
<!– @page { margin: 0.79in } P { margin-bottom: 0.08in } A:link { so-language: zxx } –>
A floating “solar fuels rig” could one day use solar energy to split apart seawater and generate hydrogen fuel. A team of scientists recently described the design for the new rig in the International Journal of Hydrogen Energy. A scaled-up version of their prototype could someday float out on the open sea, they say, producing renewable fuel from sunlight and seawater.
Scientists have long sought ways to harness sunlight to produce storable fuels that could be put to work when the sun doesn’t shine. One strategy aims to use solar-generated electricity to drive the electrolysis of water—the splitting of water (H2O) into hydrogen (H2) and oxygen (O2). Hydrogen is a clean fuel, the burning of which generates only water as its byproduct.
When commercial electrolyzers split water, they rely on membranes to separate the resulting hydrogen and oxygen gases, since mixtures of these gases are potentially explosive. But these membranes are expensive, and prone to degradation and failure.
Moreover, solar-powered hydrogen fuel production would ideally make use of cheap, abundant seawater. But commercial electrolyzers require very pure water, because seawater contains impurities and microbes that can easily destroy their membranes.
Previously, scientists had tried to develop electrolyzers that did not require membranes. However, these electrolyzers used flowing electrolyte fluids to separate hydrogen and oxygen, which required pumps and added a layer of mechanical complexity to these devices.
Photo: Jack Davis
This photos shows a prototype of a new solar-powered electrolyzer floating in a reservoir of liquid. Solar cells on top of the device convert sunlight into electricity that powers an electrolyzer submerged below.
Now researchers have developed what they suggest “is the first demonstration of a practical, floating photovoltaic-driven electrolysis device that is membrane-free and pump-free,” says Daniel Esposito, a chemical engineer at Columbia University in New York who helped build the rig.
Esposito and his colleagues envision platforms of their solar-powered electrolyzers floating on the sea to make use of abundant sunlight and water to generate hydrogen fuel. These “solar fuel rigs” are reminiscent in some respects to deep sea rigs used to harvest fossil fuels today, they say. “About 71 percent of the Earth’s surface is covered by water—why not use some of that space to harvest energy?” Esposito says.
The new electrolyzer uses electrodes made of sheets of titanium mesh that are suspended in water. A platinum catalyst coats just one side of each sheet. “These mesh electrodes are similar in nature to the metal window screens that allow desirable exchange of air between indoors and the outdoors while keeping bugs out,” Esposito says.
“About 71 percent of the Earth’s surface is covered by water—why not use some of that space to harvest energy?” —Daniel Esposito, Columbia University
When a mesh electrode is negatively charged, hydrogen bubbles develop on the side coated with the catalyst. When a mesh electrode is positively charged, oxygen bubbles develop on the catalyst-coated side instead.
The mesh electrodes are each placed at diagonal angles in the water. When the bubbles of gas on them grow large enough, the bubbles’ buoyancy makes them detach from the mesh and float upward unimpeded. Depending on the arrangement of these electrodes, hydrogen bubbles can float into one set of chambers while oxygen bubbles can float into a separate array that vents the oxygen out into the atmosphere. The simplicity of the device suggests that relatively few parts are required, which lowers the materials and assembly costs, Esposito says.
In experiments, the floating electrolyzer the researchers developed could produce hydrogen gas with a purity as high as 99 percent. “By using these electrodes, we can achieve efficient electrolysis with high product purity without a membrane and without a pump,” Esposito says.
Future work can refine this design for more efficient operation in real seawater. For example, the researchers suggest developing catalysts that do not generate toxic chlorine gas from chloride ions dissolved in seawater. The researchers also plan to develop modular versions of their device that are suitable for implementation in large-scale rigs.
Floating Solar Rig Produces Hydrogen Fuel syndicated from http://ift.tt/2Bq2FuP
0 notes
Text
Under Sink Alkaline Water Filter- Insights
Clarity Water Perfect water ionizer is a high performance filtration system that can give you clean and healthy water. But it provides you more than just alkaline water as it also takes out the clutter on top of your kitchen sink. The latest in Emco Tech alkaline water machines, Clarity Water Perfect is fitted under the sink. All that you see is an elegant tower-slash-faucet made from stainless steel and activated via soft touch control pad. With this user-friendly control, you can easily choose from among the different alkaline levels right at the flick of your fingertips. You would even get an alarm message whenever there is a low water flow or when ionization system has been disabled. Now that’s total convenience and style. You may want to check out water filters for more.
But it must be emphasized that a Clarity Water Perfect water ionizer is not all about form and elegance. Just like all the top performing filters from Emco Tech, Clarity Water Perfect can deliver in both performance and durability. This unit has the cutting-edge Biostone Filtration System, which is an alkalinizing filter that makes use of activated carbon granules, calcium, and a composition of silicate minerals. If you want to increase the range of pH levels in the water output, you can add more calcium via a slot in the main under sink assembly. With this kind of filtration system, Clarity Water Perfect can provide you and your family with the finest level of filtration.

The Clarity Water Perfect water ionizer is also fitted with advanced mesh electrode plates. This unique structuring of the ionization electrodes make it possible for the whole system to provide increased ionizing power without requiring too much electricity. The mesh electrodes are noticeably smaller than the plates in other water ionizers. But size really does not matter when it comes to electrode plates. Made from titanium and coated with platinum, Clarity Water Perfect’s electrode plates are more efficient at ionizing water and they are far more durable than other types of ionizing plates. A cross section of the electrodes, when magnified several times, will reveal that they are coated with a very fine mesh material that enhances durability and conductivity of the ionization plates.
Clarity Water Perfect water ionizer is indeed at the forefront of water ionization technology. Right from the start, it was designed both for elegance and functionality. It even has an LCD on the faucet that displays the pH level you selected and the time when you should change the filter. But above all these features, Clarity Water Perfect is all about giving you a healthy water to drink.
youtube
0 notes
Text
Economical and efficient Hydrogen production by mesh cathode

Microbial electrolytic cells present an efficient method to produce hydrogen from renewable biomass and wastewater. An economical and efficient cathode development is always a great challenge to the technology advancement. Considering the factor that stainless steel is more economical than platinum as catalyst, stainless steel mesh with larger surface areas than flat sheets were evaluated for the first time as cathodes in Microbial electrolytic cells.
Evaluation of electrochemically active surface area by cyclic voltammetry described that mesh could have about three times the active surface area of a flat sheet. The relative ranking of mesh considering current densities in MECs was in agreement with linear voltammetry studies at small bubble coverage. An optimal voltage of 0.9 V was shown in respect to high hydrogen recovery and overall energy efficiency. Hydrogen was developed by using the best mesh with thick wire size and medium pore size with a specific surface area. By using mesh cathodes a great promise have been seen for the development of economical reactors. Advanced designs by using heat processed carbon mesh as anode and stainless steel mesh as cathode cost only about 3 – 5% of the traditional materials comprising of carbon, platinum and binders.
In microbial electrolysis cells, bacteria on the anode oxidize the organic matter and convert energy available in bio-convertible substrate into electricity. By using the process, combined with small electrical input, hydrogen is developed on an anaerobic cathode, often by using catalyst.
The benefits of this biocatalyzed electrolysis to develop hydrogen from waste biomass sources over traditional technology are many. First is reduced electricity consumption. Second is no expensive metals were used on the anode because of self-sustaining microbial biocatalysts. Third, hydrogen can be reclaimed from the end products of fermentation, receiving higher hydrogen yield in a fermentation systems combined with biocatalyzed electrohydrogenesis system that a fermentation system alone. Additionally higher purity on the produced hydrogen is another benefit compared to the biohydrogen production methods from organic materials comprising biogases mixture and hence costly gas purification is not required.
Various advancements in MEC performance have been made, creating an economical and scalable design challenge for the MEC to become a proven hydrogen production technology. MEC can develop hydrogen with economical high surface area stainless steel brush at similar hydrogen production rate and efficiencies with platinum catalyzed carbon cloth cathodes, showing that expensive precious metal catalysts are not required.
Stainless steel mesh that is flat can permit closer electrode spacing of the cathode to the anode and it can have a larger surface area than flat sheet lacking pores. The main objective of this thesis was to specify the properties of the best SS mesh considering hydrogen evolution rate in single chamber membraneless MECs. There is a wide range of stainless steel mesh cathode depending on the mesh count, wire thickness and pore size, linear voltammetry was performed in an electrochemical cell to better choose the suitable mesh. Electrocemically active surface areas measured by cyclic voltammetry offered an approach to specify the effect of these factors on current production.
0 notes
Text
Precision and Performance: The Advantages of Platinum Mesh Electrodes in Electrochemistry
Platinum mesh electrodes are crucial components in electrochemical flow cells, widely used in various applications, including energy storage, sensors, and chemical analysis. Their unique properties, such as high conductivity, excellent stability, and resistance to corrosion, make them ideal for facilitating electrochemical reactions.
0 notes
Text
Platinum Mesh Electrode and Electrochemical Flow Cell: A Synergy for Advanced Research
In the field of electrochemistry, the integration of a platinum mesh electrode within an electrochemical flow cell represents a powerful combination for conducting advanced research and industrial applications. Both the platinum mesh electrode and the electrochemical flow cell are integral to achieving precise, efficient, and scalable electrochemical processes.
0 notes