#Low-Carbon Propulsion
Explore tagged Tumblr posts
Text
Low-Carbon Propulsion Market Research & Forecast till 2033
Low-Carbon Propulsion Market is expected to grow at a CAGR of 21.5% during the forecasting period 2024-2033.
The competitive analysis of the Low-Carbon Propulsion Market offers a comprehensive examination of key market players. It encompasses detailed company profiles, insights into revenue distribution, innovations within their product portfolios, regional market presence, strategic development plans, pricing strategies, identified target markets, and immediate future initiatives of industry leaders. This section serves as a valuable resource for readers to understand the driving forces behind competition and what strategies can set them apart in capturing new target markets.
Market projections and forecasts are underpinned by extensive primary research, further validated through precise secondary research specific to the Low-Carbon Propulsion Market. Our research analysts have dedicated substantial time and effort to curate essential industry insights from key industry participants, including Original Equipment Manufacturers (OEMs), top-tier suppliers, distributors, and relevant government entities.
Receive the FREE Sample Report of Low-Carbon Propulsion Market Research Insights @ https://stringentdatalytics.com/sample-request/low-carbon-propulsion-market/13361/
Market Segmentations:
Global Low-Carbon Propulsion Market: By Company • Tesla • BYD Company Ltd. • YUTONG • Nissan • Bombardier • Siemens • Alstom • Toyota • Honda Motor Co. Ltd. • Hyundai Motor Group Global Low-Carbon Propulsion Market: By Type • Heavy-Duty Vehicle • Light-Duty Vehicle Global Low-Carbon Propulsion Market: By Fuel Type • Compressed Natural Gas (CNG) • Liquefied Natural Gas (LNG) • Ethanol • Hydrogen • Electric
Regional Analysis of Global Low-Carbon Propulsion Market
All the regional segmentation has been studied based on recent and future trends, and the market is forecasted throughout the prediction period. The countries covered in the regional analysis of the Global Low-Carbon Propulsion market report are U.S., Canada, and Mexico in North America, Germany, France, U.K., Russia, Italy, Spain, Turkey, Netherlands, Switzerland, Belgium, and Rest of Europe in Europe, Singapore, Malaysia, Australia, Thailand, Indonesia, Philippines, China, Japan, India, South Korea, Rest of Asia-Pacific (APAC) in the Asia-Pacific (APAC), Saudi Arabia, U.A.E, South Africa, Egypt, Israel, Rest of Middle East and Africa (MEA) as a part of Middle East and Africa (MEA), and Argentina, Brazil, and Rest of South America as part of South America.
Click to Purchase Low-Carbon Propulsion Market Research Report @ https://stringentdatalytics.com/purchase/low-carbon-propulsion-market/13361/?license=single
Key Report Highlights:
Key Market Participants: The report delves into the major stakeholders in the market, encompassing market players, suppliers of raw materials and equipment, end-users, traders, distributors, and more.
Comprehensive Company Profiles: Detailed company profiles are provided, offering insights into various aspects including production capacity, pricing, revenue, costs, gross margin, sales volume, sales revenue, consumption patterns, growth rates, import-export dynamics, supply chains, future strategic plans, and technological advancements. This comprehensive analysis draws from a dataset spanning 12 years and includes forecasts.
Market Growth Drivers: The report extensively examines the factors contributing to market growth, with a specific focus on elucidating the diverse categories of end-users within the market.
Data Segmentation: The data and information are presented in a structured manner, allowing for easy access by market player, geographical region, product type, application, and more. Furthermore, the report can be tailored to accommodate specific research requirements.
SWOT Analysis: A SWOT analysis of the market is included, offering an insightful evaluation of its Strengths, Weaknesses, Opportunities, and Threats.
Expert Insights: Concluding the report, it features insights and opinions from industry experts, providing valuable perspectives on the market landscape.
Report includes Competitor's Landscape:
➊ Major trends and growth projections by region and country ➋ Key winning strategies followed by the competitors ➌ Who are the key competitors in this industry? ➍ What shall be the potential of this industry over the forecast tenure? ➎ What are the factors propelling the demand for the Low-Carbon Propulsion? ➏ What are the opportunities that shall aid in significant proliferation of the market growth? ➐ What are the regional and country wise regulations that shall either hamper or boost the demand for Low-Carbon Propulsion? ➑ How has the covid-19 impacted the growth of the market? ➒ Has the supply chain disruption caused changes in the entire value chain? Customization of the Report:
This report can be customized to meet the client’s requirements. Please connect with our sales team ([email protected]), who will ensure that you get a report that suits your needs. You can also get in touch with our executives on +1 346 666 6655 to share your research requirements.
About Stringent Datalytics
Stringent Datalytics offers both custom and syndicated market research reports. Custom market research reports are tailored to a specific client's needs and requirements. These reports provide unique insights into a particular industry or market segment and can help businesses make informed decisions about their strategies and operations.
Syndicated market research reports, on the other hand, are pre-existing reports that are available for purchase by multiple clients. These reports are often produced on a regular basis, such as annually or quarterly, and cover a broad range of industries and market segments. Syndicated reports provide clients with insights into industry trends, market sizes, and competitive landscapes. By offering both custom and syndicated reports, Stringent Datalytics can provide clients with a range of market research solutions that can be customized to their specific needs.
Reach US
Stringent Datalytics
+1 346 666 6655
Social Channels:
Linkedin | Facebook | Twitter | YouTube
0 notes
Text
Low-Carbon Propulsion Market Segmentation and Regional Analysis

The Low-Carbon Propulsion Market is a burgeoning sector driven by the global imperative to reduce greenhouse gas emissions and combat climate change. This market encompasses a wide array of technologies and solutions aimed at reducing carbon emissions in transportation and industrial sectors, including electric vehicles (EVs), hydrogen fuel cells, biofuels, and other alternative propulsion systems.
One of the key drivers of the Low-Carbon Propulsion Market is the increasing awareness of environmental sustainability among consumers, businesses, and governments worldwide. Stringent regulations and policies aimed at curbing carbon emissions from vehicles and industries have accelerated the adoption of low-carbon propulsion technologies. Governments are incentivizing the adoption of electric vehicles through subsidies, tax incentives, and infrastructure investments, thereby propelling the growth of the market.
Electric vehicles, in particular, have emerged as a frontrunner in the low-carbon propulsion landscape. The advancements in battery technology have improved the range, performance, and affordability of electric vehicles, making them a viable alternative to traditional internal combustion engine vehicles. Major automotive manufacturers are increasingly investing in electric vehicle research and development, aiming to capture a larger market share and meet regulatory requirements for reducing fleet emissions.
Hydrogen fuel cells also play a significant role in the Low-Carbon Propulsion Market, especially in heavy-duty transportation such as buses, trucks, and trains. Hydrogen fuel cells offer zero-emission propulsion with fast refueling times, making them suitable for long-range applications where battery electric vehicles may face limitations.
Biofuels, derived from renewable biomass sources such as crops, waste oils, and algae, are another key component of the low-carbon propulsion ecosystem. Biofuels can be blended with traditional fuels or used as standalone alternatives, offering a lower carbon footprint compared to fossil fuels.
Overall, the Low-Carbon Propulsion Market is characterized by rapid technological advancements, increasing investments, and a growing array of options for reducing carbon emissions across various industries. As sustainability concerns intensify and regulatory pressures mount, the market is poised for continued growth, innovation, and adoption of cleaner propulsion technologies in the global quest for a low-carbon future.
#Low-Carbon Propulsion Demand#Low-Carbon Propulsion Share#Low-Carbon Propulsion Trend#Low-Carbon Propulsion Size
1 note
·
View note
Text
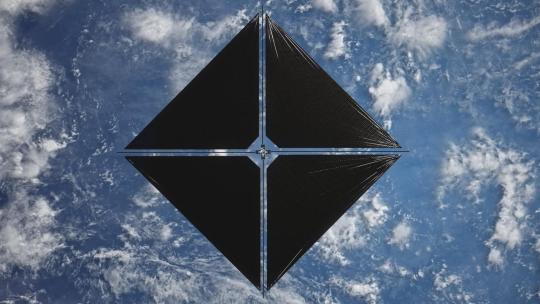
Setting Sail to Travel Through Space: 5 Things to Know about our New Mission
Our Advanced Composite Solar Sail System will launch aboard Rocket Lab’s Electron rocket from the company’s Launch Complex 1 in Māhia, New Zealand no earlier than April 23, at 6 p.m. EDT. This mission will demonstrate the use of innovative materials and structures to deploy a next-generation solar sail from a CubeSat in low Earth orbit.
Here are five things to know about this upcoming mission:
1. Sailing on Sunshine
Solar sails use the pressure of sunlight for propulsion much like sailboats harness the wind, eliminating the need for rocket fuel after the spacecraft has launched. If all goes according to plan, this technology demonstration will help us test how the solar sail shape and design work in different orbits.
2. Small Package, Big Impact
The Advanced Composite Solar Sail System spacecraft is a CubeSat the size of a microwave, but when the package inside is fully unfurled, it will measure about 860 square feet (80 square meters) which is about the size of six parking spots. Once fully deployed, it will be the biggest, functional solar sail system – capable of controlled propulsion maneuvers – to be tested in space.
3. Second NASA Solar Sail in Space
If successful, the Advanced Composite Solar Sail System will be the second NASA solar sail to deploy in space, and not only will it be much larger, but this system will also test navigation capabilities to change the spacecraft’s orbit. This will help us gather data for future missions with even larger sails.
4. BOOM: Stronger, Lighter Booms
Just like a sailboat mast supports its cloth sails, a solar sail has support beams called booms that provide structure. The Advanced Composite Solar Sail System mission’s primary objective is to deploy a new type of boom. These booms are made from flexible polymer and carbon fiber materials that are stiffer and 75% lighter than previous boom designs. They can also be flattened and rolled like a tape measure. Two booms spanning the diagonal of the square (23 feet or about 7 meters in length) could be rolled up and fit into the palm of your hand!
5. It’s a bird...it’s a plane...it’s our solar sail!
About one to two months after launch, the Advanced Composite Solar Sail System spacecraft will deploy its booms and unfurl its solar sail. Because of its large size and reflective material, the spacecraft may be visible from Earth with the naked eye if the lighting conditions and orientation are just right!
To learn more about this mission that will inform future space travel and expand our understanding of our Sun and solar system, visit https://www.nasa.gov/mission/acs3/.
Make sure to follow us on Tumblr for your regular dose of space!
2K notes
·
View notes
Text
Of Gods and Men
Targaryen Harvesters
main list (where the story is)
This is Dune/GOT/HOTD/FAB/ASOIAF crossover AU that you've voted for. If you always wanted to see House Targaryen in space, I got you. Please note how some of the lore of both universes is bent to blend in both worlds. This is my original idea that I've been cooking for at least two years. Be gentle with my work, and enjoy the ride.
The Targaryen Harvester, a marvel of advanced engineering and ancient design, stands as a testament to the ingenuity and technological prowess of House Targaryen. Built to operate in the most inhospitable environments, it is a perfect fusion of cutting-edge technology and the almost mystical properties of drakaon crystals, which form the core of all Targaryen machinery. This harvester is unlike any spice-collecting machine in the known Imperium, combining both efficiency and subtlety in ways that make it a formidable tool on Arrakis.
Exterior Design & Structure
Chassis and Hull: The harvester’s body is sleek and aerodynamic, crafted from composite alloys that blend carbon-based materials with a mysterious Valyrian steel derivative. This combination makes the harvester both lightweight and incredibly durable, capable of withstanding the harsh, abrasive sands and the extreme temperatures of desert worlds like Arrakis.
Stealth Coating: The exterior is coated with a black, matte material that absorbs and disperses radar and infrared signals, giving it a degree of stealth that makes it nearly invisible to most scanning technologies. This also helps the harvester blend seamlessly into the shadowed dunes during night operations.
Compact Size & Modular Design: Unlike the large and cumbersome spice harvesters of the Harkonnens, the Targaryen Harvester is compact, designed for mobility and ease of deployment. Its modular nature allows it to be quickly assembled or disassembled, enabling rapid deployment or withdrawal from the field when needed.
Propulsion & Movement
Hover Technology: The Targaryen Harvester employs an advanced hover propulsion system, powered by drakaon crystals. This system allows it to float just above the surface of the sands, minimizing disturbances and vibrations that might otherwise attract the attention of the sandworms. The hover technology is fine-tuned to maintain stability even in rough terrain, allowing the harvester to glide effortlessly over the undulating dunes.
Adaptive Wings: As part of its hover mechanism, the harvester has retractable wing-like structures that extend from its sides during operation. These wings are not for flight but rather serve as stabilizers that adjust to wind currents and shifting sands, ensuring smooth movement over the desert landscape. When not in use, the wings fold seamlessly into the body of the harvester, maintaining its streamlined shape.
Harvesting Mechanism
Siphoning Arrays: The core feature of the Targaryen Harvester is its siphoning arrays, located within the wing-like extensions. These arrays create a controlled vortex of air that draws in sand and spice, separating the two with a precision unmatched by traditional harvesters. The vortex is generated using ionized air currents created by the drakaon crystal cores, which create a powerful yet gentle pull that sifts through the sands.
Crystal-Based Filtration System: Once the sand and spice are drawn in, the harvester uses a series of crystal-based filters to isolate the precious melange from the surrounding sands. These crystals, sourced from Albiron, possess unique electrostatic properties that attract and bind to the spice particles, allowing the purified spice to be collected while the sand is expelled back onto the ground.
Non-Disruptive Extraction: The process of harvesting is incredibly silent compared to the rumbling, clunky harvesters of the Harkonnens. The low hum emitted by the crystal-powered engines is barely audible even in the stillness of the desert night. This low impact is essential in minimizing vibrations, reducing the risk of attracting shai-hulud, the great sandworms of Arrakis.
Energy Core & Power Source
Drakaon Crystal Core: The heart of the harvester’s power is the drakaon crystal core, a dense, multi-faceted crystal that draws energy from ambient solar radiation and stores it for continuous operation. This core allows the harvester to run for extended periods without needing external fuel sources, making it self-sufficient and capable of long-range operations in remote areas of the desert.
Solar Collectors: In addition to the crystal core, the harvester is equipped with solar collectors that deploy during the day. These collectors absorb solar energy, supplementing the crystal’s power reserve and ensuring that the harvester can operate continuously, even under the harsh sun of Arrakis.
Control Systems & Interfaces
Holographic Interface: The harvester features a holographic interface for its operators, projected from a crystal-based control console within the cockpit. This interface displays real-time data on the harvester’s status, spice yield, and environmental conditions, allowing for precise control of the siphoning process.
Neural Feedback System: Advanced Targaryen technology allows for a neural feedback system that connects the operator’s movements with the harvester’s controls. This creates a near-instantaneous response between the operator’s commands and the vehicle’s actions, enabling delicate maneuvers even in unstable terrain.
Remote Control Capabilities: The harvester can be operated remotely from a command ship or a Targaryen control station, making it possible to manage multiple harvesters simultaneously over a wide area. This remote control system is encrypted and designed to be impervious to conventional Imperial hacking methods.
Defensive Features
Energy Shields: Although primarily a civilian machine, the Targaryen Harvester is equipped with low-level energy shields derived from ancient Valyrian technology. These shields are designed to deflect micrometeor impacts and protect the harvester from smaller projectiles or environmental hazards. While not suitable for full combat, the shields provide an added layer of protection against sabotage.
Electrostatic Discharge Mechanism: To deter potential attacks or interference, the harvester can release a controlled electrostatic discharge through its siphoning arrays, disrupting nearby electronics and creating a localized EMP burst. This can disable smaller drones or tracking devices, allowing the harvester to slip away undetected.
Environmental Adaptability
Climate Adaptation Systems: Designed to function in the extreme climates of desert worlds like Arrakis, the harvester is equipped with systems that regulate internal temperatures and prevent overheating. The materials used in its construction have been treated to withstand corrosive sands and thermal expansion, ensuring long-term durability in harsh conditions.
Low-Friction Hull Design: The harvester’s hull is coated with a low-friction material that prevents sand from accumulating on its surfaces, reducing wear and ensuring that the machine can maintain its optimal performance even during extended operations in sandstorms.
Unique Features
Spice Purification Module: A specialized chamber within the harvester is dedicated to refining the spice it collects. This module uses a process that enhances the purity of the melange, making it more potent and valuable. This capability is part of what makes Targaryen spice so desirable—and so mysterious.
Integrated Holographic Cloaking: For operations that require stealth, the harvester can activate a holographic cloaking field, bending light around it to become nearly invisible against the shifting sands. This feature is rarely used, as it drains the crystal core’s reserves significantly, but it can be invaluable for avoiding detection during sensitive missions.
#hotd x dune crossover#got x dune crossover#asoiaf x dune crossover#dune#crossover#fire and blood#harvester#house targaryen#house of the dragon#asoif/got#a song of ice and fire#game of thrones#dune x reader
7 notes
·
View notes
Text

Where Warehouses Are Built, Air Pollution Follows
The footprint of warehouses in the Los Angeles area is associated with higher levels of fine particulate pollution (PM2.5) in the air, recent research shows.
Scientists analyzed patterns and trends in atmospheric PM2.5 concentrations and found that ZIP codes with more or larger warehouses had higher levels of PM2.5 and elemental carbon than those with fewer warehouses. Elemental carbon is a type of PM2.5 that is produced by heavy-duty diesel engines. The NASA-funded study was published in September 2024 in GeoHealth.
This visualization shows the average concentration of PM2.5 pollution in the Los Angeles region from 2000 to 2018, along with the locations of nearly 11,000 new or existing warehouses during the same period. Areas with higher concentrations of PM2.5 are shown in dark orange, and locations of warehouses are indicated by small black dots (many of them clustered closely together).
The PM2.5 data came from models based on satellite observations, including from NASA’s MODIS (Moderate Resolution Imaging Spectroradiometer) and ASTER (Advanced Spaceborne Thermal Emission and Reflection Radiometer) instruments. The warehouse locations were derived from a commercial real estate database.
PM2.5 particles measure 2.5 micrometers or less and can be inhaled into the lungs and absorbed into the bloodstream. Particulate pollution has been linked to respiratory and cardiovascular diseases, some cancers, and adverse birth outcomes, including premature birth and low infant birth weight. As the e-commerce boom of recent decades has spurred warehouse construction, pollution in nearby neighborhoods has become a growing area for research.
NASA Earth Observatory image by Wanmei Liang, using data from Yang, Binyu, et al. (2024), county boundaries from the California Open Data Portal, state highways from The California Department of Transportation. Story by Andrew Wang, NASA’s Jet Propulsion Laboratory, adapted for NASA Earth Observatory.
2 notes
·
View notes
Text
Building a cargo spaceship capable of exploring our solar system based on current technology and the knowledge gleaned from our understanding of engineering, science, and chemistry requires us to work within practical and realistic constraints, given that we're not yet in an era of faster-than-light travel. This project would involve a modular design, reliable propulsion systems, life support, cargo handling, and advanced automation or AI. Here’s a conceptual breakdown:
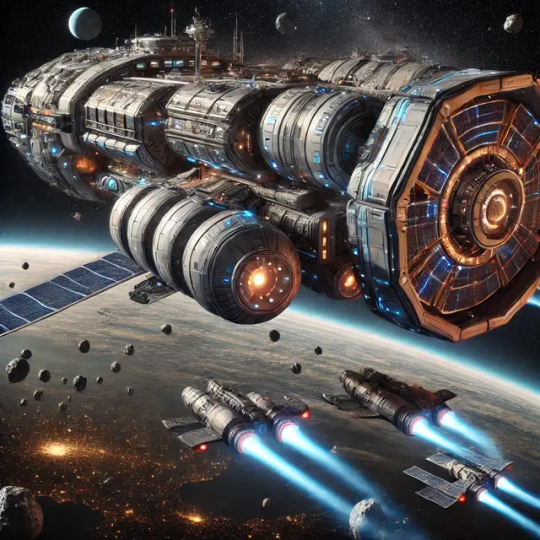
1. Ship Structure
Hull and Frame: A spaceship designed for deep space exploration needs a durable, lightweight frame. Advanced materials like titanium alloys and carbon-fiber composites would be used to ensure structural integrity under the stress of space travel while keeping the mass low. The outer hull would be made with multi-layered insulation to protect against micrometeorites and space radiation.
Dimensions: A cargo space vessel could be roughly 80-100 meters long and 30 meters wide, giving it sufficient space for cargo holds, living quarters, and propulsion systems.
Cost: $500 million (materials, assembly, and insulation).
2. Propulsion Systems
Primary Propulsion: Nuclear Thermal Propulsion (NTP) or Nuclear Electric Propulsion (NEP):
NTP would involve heating hydrogen with a nuclear reactor to achieve high exhaust velocities, providing faster travel times across the solar system. NEP converts nuclear energy into electricity, driving highly efficient ion thrusters. Both systems offer relatively efficient interplanetary travel.
A hybrid solution between NTP and NEP could optimize fuel efficiency for longer trips and maneuverability near celestial bodies.
Cost: $1 billion (development of nuclear propulsion, reactors, and installation).
Fuel: For NTP, hydrogen would be used as a propellant; for NEP, xenon or argon would be the ionized fuel. It would be replenished through in-space refueling depots or by mining water on asteroids and moons (future prospect).
Cost (fuel): $50 million.
3. Power Systems
Nuclear Fission Reactor: A compact fission reactor would power the ship’s life support, propulsion, and onboard systems. Reactors designed by NASA’s Kilopower project would provide consistent energy for long missions.
Backup Solar Arrays: Solar panels, optimized for efficiency beyond Mars’ orbit, would serve as secondary power sources in case of reactor failure.
Cost: $300 million (including reactors, solar panels, and energy storage systems).
4. Cargo Modules
The cargo holds need to be pressurized and temperature-controlled for sensitive materials or scientific samples, while some holds could be left unpressurized for bulk materials like metals, water, or fuel.
Modular Design: The ship should have detachable cargo pods for easy unloading and resupply at different planetary bodies or space stations.
Cost: $200 million (modular design, pressurization systems, automation).
5. Life Support Systems
Water and Oxygen Recycling: Systems like NASA’s Environmental Control and Life Support System (ECLSS) would recycle water, oxygen, and even waste. These systems are key for long-duration missions where resupply may be limited.
CO2 Scrubbers: To remove carbon dioxide from the air, maintaining breathable conditions for the crew.
Artificial Gravity (optional): A rotating section of the ship could generate artificial gravity through centripetal force, improving the crew’s health on longer missions. However, this would increase complexity and cost.
Cost: $200 million (life support systems, with optional artificial gravity setup).
6. AI and Automation
AI-Controlled Systems: AI would manage navigation, propulsion optimization, cargo handling, and even medical diagnostics. Automated drones could be used for ship maintenance and repairs in space.
Navigation: Advanced AI would assist in calculating complex orbital maneuvers, interplanetary transfers, and landings.
Autonomous Cargo Handling: Robotics and AI would ensure that cargo can be efficiently moved between space stations, planets, and the ship.
Cost: $150 million (AI development, robotics, automation).
7. Communication and Sensors
Communication Arrays: High-gain antennas would allow for deep-space communication back to Earth, supplemented by laser communication systems for high-speed data transfers.
Radars and Sensors: For mapping asteroid belts, detecting anomalies, and navigating planets, advanced LIDAR, radar, and spectrometers would be necessary. These sensors would aid in planetary exploration and mining operations.
Cost: $100 million (communication systems, sensors, and diagnostics).
8. Radiation Protection
Water Shielding: Water, which is also used in life support, would double as a radiation shield around the living quarters.
Electromagnetic Shields: Experimental concepts involve creating a small electromagnetic field around the ship to deflect solar and cosmic radiation (early TRL, requires more development).
Cost: $50 million (radiation shielding).
9. Crew Quarters
Living Quarters: Designed for long-duration missions with the capability to house 4-6 crew members comfortably. The quarters would feature radiation protection, artificial lighting cycles to simulate day and night, and recreational facilities to maintain crew morale on multi-year missions.
Medical Bay: An AI-assisted medical bay equipped with robotic surgery and telemedicine would ensure the crew remains healthy.
Cost: $100 million (crew quarters, recreational facilities, medical systems).
10. Landing and Exploration Modules
Surface Exploration Vehicles: For landing on moons or planets like Mars or Europa, a modular lander or rover system would be required. These vehicles would use methane/oxygen engines or electric propulsion to take off and land on various celestial bodies.
Cost: $300 million (lander, rovers, exploration modules).
---
Total Estimated Cost: $2.95 Billion
Additional Considerations:
1. Launch Vehicles: To get the spacecraft into orbit, you would need a heavy-lift rocket like SpaceX’s Starship or NASA’s Space Launch System (SLS). Multiple launches may be required to assemble the ship in orbit.
Cost (launch): $500 million (several launches).
2. In-Space Assembly: The ship would likely be built and assembled in low-Earth orbit (LEO), with components brought up in stages by heavy-lift rockets.
Cost: $200 million (orbital assembly infrastructure and operations).
---
Grand Total: $3.65 Billion
This estimate provides a general cost breakdown for building a cargo spaceship that could explore and transport materials across the solar system. This concept ship is realistic based on near-future technologies, leveraging both nuclear propulsion and automation to ensure efficient exploration and cargo transportation across the solar system.
#canada#canadian politics#space#science#scifi#scifiart#sci fi and fantasy#nasa#nasa photos#elon musk#share#engineering#ideas#ai#scientificresearch#billionaire
6 notes
·
View notes
Text
Lots of companies sell seafood on the mass market without this sort of plastic fishing net waste. If you’re looking for what to avoid while waiting for companies to take responsibility for their own actions, stay away from purse seine and gillnet fishing. (If you can only avoid one of these, avoid purse seine). They use large, hard-to-recycle nets, that because of their size are also less likely to be made from biodegradable/recyclable materials like hemp. Longline has long, difficult-to-recycle lines, but this can be done in a way that lends itself to recycling the line easier than purse seine or gillnet. However, the bycatch (proportion of fish caught that are not the right species, or the wrong size) is worse than with purse seine methods. Troll fishing and pole/line methods are better from the angle of sustainable nets, because the lines are shorter and thus easier to recycle (bycatch is also low, but it is harder to sustain fishing stocks). This is not to say it is always easy, or always done. Also note that the more sustainable methods from the perspective of plastic are, by default, the least sustainable in terms of fossil fuel usage. This is an area where carbon-efficient (and indeed carbon-neutral) propulsion methods would be a big help. This is the main reason why the International Sustainable Seafood Foundation approves all 5 of the listed approaches in theory, and is more interested in how a given boat implements its chosen method in practice. Ask companies to make lines from hemp and other sustainable products. Ask them to recycle their lines and show how they are recycled (some companies have a strange definition of “recycle”). Ask them to repair their lines where feasible (bonus: it’s often cheaper for the company as well) instead of replacing them. Ask the company to research applicable sustainability practices for each type of fish/seafood they catch (sometimes the best method for one species is not the best method for another, although many practices work well across species) and apply them.
So, there's apparently research coming out now about microplastics being found in people's bloodstreams and the possible negative effects of that and I feel the need to get out ahead of the wave of corporate sponsored "be sure to recycle your bottles!" or "ban glitter!" campaigns and remind everyone: It's fishing nets. It's fishing nets. It is overwhelming fishing nets It always has been fishing nets. Unless regulations are changed, it will continue to be fishing nets. The plastic in the ocean in largely discarded nets from industrial fishing. The microplastics are the result of these nets breaking down. The "trash islands" are also, you guessed it. Mostly fishing nets and other discarded fishing industry equipment. Do not allow them to continue to twist the story. Do not come after disabled people who require single use plastics. Do not come after people using glitter in art projects and makeup. These things make up a negligible amount of the issue compared to corporate waste, specifically in the fishing industry. Do not let them shift the blame to the individual so they can continue to destroy the planet and our bodies without regulation.
158K notes
·
View notes
Text
The Future of the Hydrogen Valve Industry: Trends and Innovations
The hydrogen valve industry is poised for significant growth as the world pivots towards cleaner, sustainable energy sources. With the increasing demand for hydrogen as a clean fuel alternative, the importance of efficient and reliable hydrogen infrastructure becomes paramount. In this article, we will explore the future of the hydrogen valve industry, the technological innovations shaping it, and the key factors driving its growth.
The Growing Demand for Hydrogen
Hydrogen is increasingly being recognized as a clean, versatile energy source capable of powering everything from industrial applications to transportation. As governments and organizations focus on reducing carbon emissions, hydrogen fuel cells are becoming integral to the development of a low-carbon economy. The transition to a hydrogen-based energy system relies heavily on advanced hydrogen infrastructure, which includes storage, transportation, and distribution systems—making hydrogen valves crucial to maintaining system efficiency and safety.
Hydrogen Valve Industry Overview
The global hydrogen valve market was valued at USD 331 million in 2024 and is projected to reach USD 507 million by 2030, with a robust compound annual growth rate (CAGR) of 7.4% during the forecast period from 2024 to 2030. This growth reflects the increasing significance of hydrogen as a sustainable fuel for the future, driving demand for advanced infrastructure in hydrogen production, transportation, and storage.
Rising Demand for Hydrogen Infrastructure
As hydrogen continues to gain recognition as a key fuel source for a low-carbon future, the demand for hydrogen infrastructure, including valves, has escalated. Hydrogen can exist at different pressure levels—low, high, or cryogenic—and each state requires specialized equipment to handle it safely and efficiently. Valve systems are essential in regulating the flow and pressure of hydrogen at these various stages. Therefore, as the need for hydrogen grows, the demand for reliable, high-quality hydrogen valves is also expected to rise, especially as hydrogen becomes a more integral part of energy systems worldwide.
Hydrogen Valves in Industrial and Vehicle Applications
In addition to their critical role in various industrial sectors, hydrogen valves are also pivotal in the production of hydrogen-based internal combustion (IC) engines. The future of hydrogen-powered vehicles is on the horizon, especially as electric vehicles (EVs) may face limitations in the long term. Hydrogen-powered internal combustion engines (ICE) could provide an alternative solution to EVs. Recent advancements, such as Kawasaki’s successful testing of hydrogen-powered internal combustion engines for motorcycles, highlight the potential of hydrogen in the automotive sector. Kawasaki is expecting these hydrogen IC engines to be commercialized by 2023, paving the way for a significant shift in vehicle propulsion technology.
As the hydrogen industry evolves, valves will play an increasingly important role in ensuring safe, efficient, and reliable handling of hydrogen across various applications.
Download PDF Brochure @ https://www.marketsandmarkets.com/pdfdownloadNew.asp?id=27538308
Key Trends in the Hydrogen Valve Industry
Increased Focus on Safety and Reliability Hydrogen is a highly flammable and volatile gas, making safety a critical concern in hydrogen systems. The future of the hydrogen valve industry will be driven by innovations focused on enhancing safety, such as advanced sealing technologies, corrosion-resistant materials, and automated shut-off valves that improve the overall reliability of hydrogen systems.
Technological Advancements in Valve Design As the hydrogen industry evolves, so too does the demand for more efficient and durable valves. Future valve designs will likely incorporate smart technology, allowing real-time monitoring and diagnostics to ensure optimum performance. These advancements will help prevent leaks, reduce maintenance costs, and improve the overall efficiency of hydrogen storage and transport systems.
Integration with Renewable Energy Systems Hydrogen valves will play a key role in integrating hydrogen production, storage, and usage with renewable energy systems. As wind, solar, and other renewable energy sources become more prevalent, the need for flexible, high-performance valves that can handle varying pressures and temperatures will increase. Hydrogen infrastructure must be adaptable to changes in energy demand, requiring valves that can support dynamic energy systems.
Global Expansion of Hydrogen Infrastructure Governments worldwide are investing heavily in hydrogen infrastructure to support clean energy transitions. With initiatives like the European Union's hydrogen strategy and the U.S. Department of Energy's investment in hydrogen projects, the hydrogen valve industry is expected to see increased demand. Manufacturers will need to develop valves that can meet international standards and work efficiently in diverse environmental conditions.
Focus on Sustainability The future of the hydrogen valve industry is not just about technological improvements; sustainability will also be a driving force. Manufacturers will prioritize eco-friendly materials and energy-efficient designs to minimize the environmental impact of production. Moreover, valves will be designed for longer service lives to reduce waste and improve the overall sustainability of hydrogen systems.
The Role of Innovation in Hydrogen Valves
Innovation in materials science and engineering will be critical to the future of hydrogen valves. New materials, such as lightweight composites and corrosion-resistant alloys, will allow valves to withstand the challenging operating conditions of hydrogen systems. Additionally, digital technologies, including sensors and IoT-enabled devices, will facilitate smarter, more efficient valve operation. These innovations will not only improve the functionality of hydrogen systems but also contribute to lower operational costs and a higher level of safety.
The future of the hydrogen valve industry is bright, with immense potential for growth driven by technological advancements, global energy shifts, and increasing investment in hydrogen infrastructure. As hydrogen continues to emerge as a key player in the global energy transition, valves will play a crucial role in ensuring the safe, reliable, and efficient transportation and storage of hydrogen. Manufacturers who invest in cutting-edge technologies and sustainability will be well-positioned to lead the industry into the future.
FAQ
1. What is the role of valves in hydrogen systems? Valves are critical components in hydrogen systems, controlling the flow, pressure, and safety of hydrogen in pipelines, storage tanks, and fuel cells. They ensure that hydrogen is safely transported and stored while maintaining system integrity.
2. Why are safety features important for hydrogen valves? Hydrogen is highly flammable, so valves with enhanced safety features such as leak prevention, corrosion resistance, and automated shut-off mechanisms are essential for preventing accidents and ensuring system reliability.
3. How will smart technology impact the hydrogen valve industry? Smart technology will enable hydrogen valves to provide real-time monitoring and diagnostic information, leading to improved efficiency, reduced maintenance costs, and early detection of potential issues.
4. What materials are used in hydrogen valves? Hydrogen valves are typically made from materials that are resistant to corrosion and high pressures, such as stainless steel, alloys, and advanced composites. These materials ensure the valves remain durable and reliable in harsh conditions.
5. What are the global trends influencing the hydrogen valve market? The global push for clean energy, growing investments in hydrogen infrastructure, and technological advancements in valve design are the primary trends influencing the hydrogen valve industry. These trends are driving demand for more efficient, reliable, and safe valve solutions.
0 notes
Link
0 notes
Text
Find Your Perfect Fit: Top Tennis Shoes for Every Player
When it comes to tennis, having the right pair of shoes is crucial to your performance, comfort, and overall injury prevention. The right tennis shoes can help you move with ease, support your body during intense matches, and provide the durability you need for the court’s varying surfaces. Whether you’re an aggressive baseline player or a net-rushing doubles expert, the perfect tennis shoe can make all the difference. In this guide, we’ll explore some of the best tennis shoes for every type of player and provide tips on how to find the best fit for your game.

1. Best Tennis Shoes for Agility: Nike Air Zoom Vapor Pro
For players who prioritize speed and agility, lightweight shoes with excellent responsiveness are essential. Nike Air Zoom Vapor Pro delivers both comfort and speed, making it a top choice for quick, nimble players. These shoes feature a low-profile design that keeps your foot close to the ground for better stability during fast lateral movements.
The shoes also incorporate Zoom Air cushioning in the forefoot, which provides responsive cushioning to propel you forward during sprints. The durable rubber outsole offers excellent traction on various court surfaces, while the breathable mesh upper helps keep your feet cool throughout your match.
2. Best Tennis Shoes for Stability: Asics Gel-Resolution 9
If you play a more controlled game and need maximum stability and support during lateral movements, the Asics Gel-Resolution 9 is a solid choice. These shoes are designed to offer excellent support during intense side-to-side movements, making them ideal for players who tend to play a lot of baseline rallies or aggressive cross-court shots.
The Gel cushioning system in both the forefoot and the rearfoot absorbs shock during impact, reducing the strain on your joints and helping you maintain comfort during long matches. The Dynamic DuoMax Support System helps stabilize your foot and prevent overpronation, ensuring you're well-supported during aggressive lateral movements. Plus, the outsole provides great durability, making it a top choice for hard court players.
3. Best Tennis Shoes for Wide Feet: Babolat Propulse Fury
Finding tennis shoes that fit wide feet can be tricky, but the Babolat Propulse Fury is a great option for players who need extra room without compromising on performance. The 3D Matryx upper provides a flexible yet supportive fit, ensuring that your foot is held securely in place while still offering enough room for comfort.
The dual-density EVA midsole enhances shock absorption and cushioning, while the Michelin outsole provides excellent traction on both hard and clay courts. These shoes also have a customizable lacing system, which allows you to adjust the fit based on your foot shape. For players with wider feet, these shoes offer a snug fit without feeling too tight or restrictive.
4. Best Tennis Shoes for Lightweight Performance: Wilson Rush Pro 3.0
If you’re someone who values speed, agility, and lightweight footwear, the Wilson Rush Pro 3.0 is an excellent choice. These shoes are designed with carbon fiber technology, which makes them ultra-light yet highly durable. The Endofit inner-sleeve construction wraps your foot for a snug and supportive fit, while the proprietary Wilson’s Rush Pro outsole delivers a high level of traction on various court surfaces.
The Dynamic Fit 2.0 system helps ensure your foot stays secure while providing the flexibility to move freely. The lightweight cushioning allows you to remain quick on your feet, so you can outmaneuver your opponent on the court.
5. Best Tennis Shoes for Comfort & Performance: Nike Air Zoom Cage 4
For players who want the perfect combination of comfort and performance, the Nike Air Zoom Cage 4 is an exceptional choice. Designed for players who like to cover a lot of ground, these shoes are lightweight yet offer an impressive level of support and durability.
The Zoom Air cushioning in the forefoot provides responsive comfort, while the mesh upper allows breathability. The Nike Cage 4 also features a reinforced rubber outsole that ensures traction on any surface, giving you the grip you need to power through every rally. This shoe is a fantastic all-rounder for players who want top-notch performance without sacrificing comfort.
How to Find Your Perfect Fit
Finding the right tennis shoe for your game involves considering several factors:
Foot Type: Do you have flat feet, high arches, or wide feet? Understanding your foot type is crucial when selecting the right shoe. Look for shoes designed specifically for your foot shape and comfort.
Court Surface: Tennis shoes are designed with different outsoles depending on the court surface. Hard court shoes typically have durable rubber outsoles, while clay court shoes feature a herringbone pattern for better grip.
Play Style: Consider your playing style. Are you a baseliner who needs stability, or a serve-and-volley player looking for lightweight agility? Choose shoes that support your specific movements.
Comfort and Fit: Always prioritize comfort over anything else. Make sure there’s enough room in the toe box, the shoe fits snugly around the heel, and there’s no pinching or discomfort.
Conclusion
Finding the perfect tennis shoe can significantly enhance your performance on the court. Whether you’re looking for speed, support, comfort, or durability, there’s a shoe out there designed to meet your unique needs. By understanding your foot type, playing style, and the surface you’ll be playing on, you can select a shoe that keeps you comfortable, supported, and ready to dominate your next match.
0 notes
Text
🚄 Hyperloop Infrastructure Market: Racing to $12.4B by 2034
Hyperloop Infrastructure Market is transforming the future of travel and logistics with its innovative high-speed, low-pressure tube networks. Designed to transport passengers and cargo with unprecedented efficiency, this market includes the development of vacuum tubes, advanced propulsion systems, safety mechanisms, and station infrastructure. With its potential to reduce travel times and environmental impact, Hyperloop technology is driving global investment and collaboration among technology firms, infrastructure developers, and governments.
To Request Sample Report: https://www.globalinsightservices.com/request-sample/?id=GIS10999 &utm_source=SnehaPatil&utm_medium=Article
Passenger transportation infrastructure leads the market, holding a 55% share, as urban areas seek sustainable and rapid transit solutions. Freight transportation infrastructure follows with a 30% share, driven by the demand for cost-effective logistics. North America dominates the market, fueled by advanced R&D and substantial investments, particularly in the United States. Europe is a strong contender, with government-backed initiatives to cut carbon emissions. Meanwhile, emerging economies like China and India are rapidly scaling up their Hyperloop infrastructure to support urbanization and enhance their transportation networks.
In 2023, the market was valued at 150 million metric tons, with projections to grow to 400 million metric tons by 2033, reflecting a 15% annual growth rate. The competitive landscape features key players like Virgin Hyperloop, Hyperloop Transportation Technologies, and TransPod. Virgin Hyperloop focuses on commercial applications, while Hyperloop Transportation Technologies drives innovation through advanced technologies like magnetic levitation and linear induction motors.
Despite challenges such as high initial costs and regulatory complexities, the market outlook is promising. Strategic partnerships, R&D, and government incentives are paving the way for growth. The Hyperloop’s potential to redefine intercity travel and freight logistics positions it as a cornerstone of the sustainable transport revolution.
#HyperloopRevolution #SustainableTransport #HighSpeedTravel #FutureOfMobility #UrbanTransit #FreightInnovation #EcoFriendlyTravel #VacuumTransport #TransportInnovation #HyperloopTech #MagneticLevitation #GreenInfrastructure #UrbanMobility #IntercityTransit #RapidTransitSolutions #TechForGood #CarbonReduction #NextGenTransport #SmartMobility #FutureOfLogistics #InnovativeTransport #GlobalConnectivity #GreenTech #RethinkTransportation #HyperloopJourney
0 notes
Text
0 notes
Text
Toward sustainable decarbonization of aviation in Latin America
New Post has been published on https://sunalei.org/news/toward-sustainable-decarbonization-of-aviation-in-latin-america/
Toward sustainable decarbonization of aviation in Latin America

According to the International Energy Agency, aviation accounts for about 2 percent of global carbon dioxide emissions, and aviation emissions are expected to double by mid-century as demand for domestic and international air travel rises. To sharply reduce emissions in alignment with the Paris Agreement’s long-term goal to keep global warming below 1.5 degrees Celsius, the International Air Transport Association (IATA) has set a goal to achieve net-zero carbon emissions by 2050. Which raises the question: Are there technologically feasible and economically viable strategies to reach that goal within the next 25 years?
To begin to address that question, a team of researchers at the MIT Center for Sustainability Science and Strategy (CS3) and the MIT Laboratory for Aviation and the Environment has spent the past year analyzing aviation decarbonization options in Latin America, where air travel is expected to more than triple by 2050 and thereby double today’s aviation-related emissions in the region.
Chief among those options is the development and deployment of sustainable aviation fuel. Currently produced from low- and zero-carbon sources (feedstock) including municipal waste and non-food crops, and requiring practically no alteration of aircraft systems or refueling infrastructure, sustainable aviation fuel (SAF) has the potential to perform just as well as petroleum-based jet fuel with as low as 20 percent of its carbon footprint.
Focused on Brazil, Chile, Colombia, Ecuador, Mexico and Peru, the researchers assessed SAF feedstock availability, the costs of corresponding SAF pathways, and how SAF deployment would likely impact fuel use, prices, emissions, and aviation demand in each country. They also explored how efficiency improvements and market-based mechanisms could help the region to reach decarbonization targets. The team’s findings appear in a CS3 Special Report.
SAF emissions, costs, and sources
Under an ambitious emissions mitigation scenario designed to cap global warming at 1.5 C and raise the rate of SAF use in Latin America to 65 percent by 2050, the researchers projected aviation emissions to be reduced by about 60 percent in 2050 compared to a scenario in which existing climate policies are not strengthened. To achieve net-zero emissions by 2050, other measures would be required, such as improvements in operational and air traffic efficiencies, airplane fleet renewal, alternative forms of propulsion, and carbon offsets and removals.
As of 2024, jet fuel prices in Latin America are around $0.70 per liter. Based on the current availability of feedstocks, the researchers projected SAF costs within the six countries studied to range from $1.11 to $2.86 per liter. They cautioned that increased fuel prices could affect operating costs of the aviation sector and overall aviation demand unless strategies to manage price increases are implemented.
Under the 1.5 C scenario, the total cumulative capital investments required to build new SAF producing plants between 2025 and 2050 were estimated at $204 billion for the six countries (ranging from $5 billion in Ecuador to $84 billion in Brazil). The researchers identified sugarcane- and corn-based ethanol-to-jet fuel, palm oil- and soybean-based hydro-processed esters and fatty acids as the most promising feedstock sources in the near term for SAF production in Latin America.
“Our findings show that SAF offers a significant decarbonization pathway, which must be combined with an economy-wide emissions mitigation policy that uses market-based mechanisms to offset the remaining emissions,” says Sergey Paltsev, lead author of the report, MIT CS3 deputy director, and senior research scientist at the MIT Energy Initiative.
Recommendations
The researchers concluded the report with recommendations for national policymakers and aviation industry leaders in Latin America.
They stressed that government policy and regulatory mechanisms will be needed to create sufficient conditions to attract SAF investments in the region and make SAF commercially viable as the aviation industry decarbonizes operations. Without appropriate policy frameworks, SAF requirements will affect the cost of air travel. For fuel producers, stable, long-term-oriented policies and regulations will be needed to create robust supply chains, build demand for establishing economies of scale, and develop innovative pathways for producing SAF.
Finally, the research team recommended a region-wide collaboration in designing SAF policies. A unified decarbonization strategy among all countries in the region will help ensure competitiveness, economies of scale, and achievement of long-term carbon emissions-reduction goals.
“Regional feedstock availability and costs make Latin America a potential major player in SAF production,” says Angelo Gurgel, a principal research scientist at MIT CS3 and co-author of the study. “SAF requirements, combined with government support mechanisms, will ensure sustainable decarbonization while enhancing the region’s connectivity and the ability of disadvantaged communities to access air transport.”
Financial support for this study was provided by LATAM Airlines and Airbus.
0 notes
Text
Toward sustainable decarbonization of aviation in Latin America
New Post has been published on https://thedigitalinsider.com/toward-sustainable-decarbonization-of-aviation-in-latin-america/
Toward sustainable decarbonization of aviation in Latin America


According to the International Energy Agency, aviation accounts for about 2 percent of global carbon dioxide emissions, and aviation emissions are expected to double by mid-century as demand for domestic and international air travel rises. To sharply reduce emissions in alignment with the Paris Agreement’s long-term goal to keep global warming below 1.5 degrees Celsius, the International Air Transport Association (IATA) has set a goal to achieve net-zero carbon emissions by 2050. Which raises the question: Are there technologically feasible and economically viable strategies to reach that goal within the next 25 years?
To begin to address that question, a team of researchers at the MIT Center for Sustainability Science and Strategy (CS3) and the MIT Laboratory for Aviation and the Environment has spent the past year analyzing aviation decarbonization options in Latin America, where air travel is expected to more than triple by 2050 and thereby double today’s aviation-related emissions in the region.
Chief among those options is the development and deployment of sustainable aviation fuel. Currently produced from low- and zero-carbon sources (feedstock) including municipal waste and non-food crops, and requiring practically no alteration of aircraft systems or refueling infrastructure, sustainable aviation fuel (SAF) has the potential to perform just as well as petroleum-based jet fuel with as low as 20 percent of its carbon footprint.
Focused on Brazil, Chile, Colombia, Ecuador, Mexico and Peru, the researchers assessed SAF feedstock availability, the costs of corresponding SAF pathways, and how SAF deployment would likely impact fuel use, prices, emissions, and aviation demand in each country. They also explored how efficiency improvements and market-based mechanisms could help the region to reach decarbonization targets. The team’s findings appear in a CS3 Special Report.
SAF emissions, costs, and sources
Under an ambitious emissions mitigation scenario designed to cap global warming at 1.5 C and raise the rate of SAF use in Latin America to 65 percent by 2050, the researchers projected aviation emissions to be reduced by about 60 percent in 2050 compared to a scenario in which existing climate policies are not strengthened. To achieve net-zero emissions by 2050, other measures would be required, such as improvements in operational and air traffic efficiencies, airplane fleet renewal, alternative forms of propulsion, and carbon offsets and removals.
As of 2024, jet fuel prices in Latin America are around $0.70 per liter. Based on the current availability of feedstocks, the researchers projected SAF costs within the six countries studied to range from $1.11 to $2.86 per liter. They cautioned that increased fuel prices could affect operating costs of the aviation sector and overall aviation demand unless strategies to manage price increases are implemented.
Under the 1.5 C scenario, the total cumulative capital investments required to build new SAF producing plants between 2025 and 2050 were estimated at $204 billion for the six countries (ranging from $5 billion in Ecuador to $84 billion in Brazil). The researchers identified sugarcane- and corn-based ethanol-to-jet fuel, palm oil- and soybean-based hydro-processed esters and fatty acids as the most promising feedstock sources in the near term for SAF production in Latin America.
“Our findings show that SAF offers a significant decarbonization pathway, which must be combined with an economy-wide emissions mitigation policy that uses market-based mechanisms to offset the remaining emissions,” says Sergey Paltsev, lead author of the report, MIT CS3 deputy director, and senior research scientist at the MIT Energy Initiative.
Recommendations
The researchers concluded the report with recommendations for national policymakers and aviation industry leaders in Latin America.
They stressed that government policy and regulatory mechanisms will be needed to create sufficient conditions to attract SAF investments in the region and make SAF commercially viable as the aviation industry decarbonizes operations. Without appropriate policy frameworks, SAF requirements will affect the cost of air travel. For fuel producers, stable, long-term-oriented policies and regulations will be needed to create robust supply chains, build demand for establishing economies of scale, and develop innovative pathways for producing SAF.
Finally, the research team recommended a region-wide collaboration in designing SAF policies. A unified decarbonization strategy among all countries in the region will help ensure competitiveness, economies of scale, and achievement of long-term carbon emissions-reduction goals.
“Regional feedstock availability and costs make Latin America a potential major player in SAF production,” says Angelo Gurgel, a principal research scientist at MIT CS3 and co-author of the study. “SAF requirements, combined with government support mechanisms, will ensure sustainable decarbonization while enhancing the region’s connectivity and the ability of disadvantaged communities to access air transport.”
Financial support for this study was provided by LATAM Airlines and Airbus.
#2024#2025#Accounts#acids#agreement#air#Air traffic#aircraft#America#author#aviation#billion#Brazil#carbon#Carbon dioxide#carbon dioxide emissions#carbon emissions#carbon footprint#Center for Sustainability Science and Strategy#Cleaner industry#climate#climate change#Collaboration#connectivity#corn#crops#decarbonization#deployment#development#double
0 notes
Text




NASA scientists recreate Mars's spider-shaped geologic formations in lab for the first time
Tests on Earth appear to confirm how the red planet's spider-shaped geologic formations are carved by carbon dioxide.
Since discovering them in 2003 via images from orbiters, scientists have marveled at spider-like shapes sprawled across the southern hemisphere of Mars. No one is entirely sure how these geologic features are created. Each branched formation can stretch more than a half-mile (1 kilometer) from end to end and include hundreds of spindly "legs." Called araneiform terrain, these features are often found in clusters, giving the surface a wrinkled appearance.
The leading theory is that the spiders are created by processes involving carbon dioxide ice, which doesn't occur naturally on Earth. Thanks to experiments detailed in a new paper published in The Planetary Science Journal, scientists have, for the first time, re-created those formation processes in simulated Martian temperatures and air pressure.
"The spiders are strange, beautiful geologic features in their own right," said Lauren Mc Keown of NASA's Jet Propulsion Laboratory in Southern California. "These experiments will help tune our models for how they form."
The study confirms several formation processes described by what's called the Kieffer model: Sunlight heats the soil when it shines through transparent slabs of carbon dioxide ice that built up on the Martian surface each winter.
Being darker than the ice above it, the soil absorbs the heat and causes the ice closest to it to turn directly into carbon dioxide gas—without turning to liquid first—in a process called sublimation (the same process that sends clouds of "smoke" billowing up from dry ice). As the gas builds in pressure, the Martian ice cracks, allowing the gas to escape. As it seeps upward, the gas takes with it a stream of dark dust and sand from the soil that lands on the surface of the ice.
When winter turns to spring and the remaining ice sublimates, according to the theory, the spiderlike scars from those small eruptions are what's left behind.
Recreating Mars in the lab
For Mc Keown and her co-authors, the hardest part of conducting these experiments was re-creating conditions found on the Martian polar surface: extremely low air pressure and temperatures as low as minus 301 degrees Fahrenheit (minus 185 degrees Celsius). To do that, Mc Keown used a liquid-nitrogen-cooled test chamber at JPL, the Dirty Under-vacuum Simulation Testbed for Icy Environments, or DUSTIE.
"I love DUSTIE. It's historic," Mc Keown said, noting that the wine barrel-size chamber was used to test a prototype of a rasping tool designed for NASA's Mars Phoenix lander. The tool was used to break water ice, which the spacecraft scooped up and analyzed near the planet's north pole.
For this experiment, the researchers chilled Martian soil simulant in a container submerged within a liquid nitrogen bath. They placed it in the DUSTIE chamber, where the air pressure was reduced to be similar to that of Mars's southern hemisphere. Carbon dioxide gas then flowed into the chamber and condensed from gas to ice over the course of three to five hours. It took many tries before Mc Keown found just the right conditions for the ice to become thick and translucent enough for the experiments to work.
Once they got ice with the right properties, they placed a heater inside the chamber below the simulant to warm it up and crack the ice. Mc Keown was ecstatic when she finally saw a plume of carbon dioxide gas erupting from within the powdery simulant.
"It was late on a Friday evening and the lab manager burst in after hearing me shrieking," said Mc Keown, who had been working to make a plume like this for five years. "She thought there had been an accident."
The dark plumes opened holes in the simulant as they streamed out, spewing simulant for as long as 10 minutes before all the pressurized gas was expelled.
The experiments included a surprise that wasn't reflected in the Kieffer model: Ice formed between the grains of the simulant, then cracked it open. This alternative process might explain why spiders have a more "cracked" appearance. Whether this happens or not seems dependent on the size of soil grains and how embedded water ice is underground.
"It's one of those details that show that nature is a little messier than the textbook image," said Serina Diniega of JPL, a co-author of the paper.
What's next for plume testing
Now that the conditions have been found for plumes to form, the next step is to try the same experiments with simulated sunlight from above, rather than using a heater below. That could help scientists narrow down the range of conditions under which the plumes and ejection of soil might occur.
There are still many questions about the spiders that can't be answered in a lab. Why have they formed in some places on Mars but not others? Since they appear to result from seasonal changes that are still occurring, why don't they seem to be growing in number or size over time? It's possible that they're left over from long ago, when the climate was different on Mars—and could therefore provide a unique window into the planet's past.
For the time being, lab experiments will be as close to the spiders as scientists can get. Both the Curiosity and Perseverance rovers are exploring the red planet far from the southern hemisphere, which is where these formations appear (and where no spacecraft has ever landed). The Phoenix mission, which landed in the northern hemisphere, lasted only a few months before succumbing to the intense polar cold and limited sunlight.
TOP IMAGE: Spider-shaped features called araneiform terrain are found in the southern hemisphere of Mars, carved into the landscape by carbon dioxide gas. This 2009 image taken by NASA’s Mars Reconnaissance Orbiter shows several of these distinctive formations within an area three-quarters of a mile (1.2 kilometers) wide. Credit: NASA / JPL-Caltech / University of Arizona
CENTRE IMAGE: These formations similar to the Red Planet’s “spiders” appeared within Martian soil simulant during experiments in JPL’s DUSTIE chamber. Carbon dioxide ice frozen within the simulant was warmed by a heater below, turning it back into gas that eventually cracked through the frozen top layer and formed a plume. Credit: NASA / JPL-Caltech
LOWER IMAGE: Dark splotches seen in this example of araneiform terrain captured by NASA’s Mars Reconnaissance Orbiter in 2018 are believed to be soil ejected from the surface by carbon dioxide gas plumes. A set of experiments at JPL has sought to re-create these spider-like formations in a lab. Credit: NASA / JPL-Caltech / University of Arizona
BOTTOM IMAGE: Here’s a look inside of JPL’s DUSTIE, a wine barrel-size chamber used to simulate the temperatures and air pressure of other planets – in this case, the carbon dioxide ice found on Mars’ south pole. Experiments conducted in the chamber confirmed how Martian formations known as “spiders” are created. Credit: NASA / JPL-Caltech
2 notes
·
View notes
Text
A Decade of Change: Transformations in Ocean Freight Logistics (2014-2024)

Over the past decade, ocean freight logistics has undergone remarkable transformations, driven by technological advancements, evolving global trade dynamics, and the growing need for sustainable practices. From digitalization tointroducing green shipping methods, the ocean freight industry has made significant strides in efficiency, transparency, and environmental responsibility. This article explores the key developments in ocean freight logistics from 2014 to 2024, shedding light on how these changes have reshaped global shipping.
1. The Rise of Digitalization
One of the most notable changes in ocean freighthas been the widespread adoption of digital technologies. Over the past ten years, shipping companies have embraced innovations such as blockchain, artificial intelligence (AI), and the Internet of Things (IoT) to streamline operations.
Blockchain for Transparency: Blockchain technology has improved supply chain visibility and reduced fraud. By creating a secure, immutable ledger, blockchain enables seamless tracking of shipments, ensuring that all parties have access to accurate and reliable data.
AI and Predictive Analytics: AI-driven tools have revolutionized route optimization, demand forecasting, and risk management. Predictive analytics help freight forwarders avoid delays by analysing historical data and predicting potential disruptions.
IoT for Real-Time Monitoring: IoT devices, such as smart containers, provide real-time data on cargo conditions, including temperature, humidity, and location. This technology is especially crucial for industries like pharmaceuticals and perishables.
2. E-Commerce and Its Impact
The exponential growth of e-commerce has reshaped international cargo shipping. With more consumers demanding faster delivery and smaller shipment sizes, the industry has adapted to accommodate these changes.
Shift Toward LCL Shipments: Less-than-container-load (LCL) shipments have gained popularity as businesses prioritize cost-effectiveness and flexibility. This trend has allowed small and medium-sized enterprises (SMEs) to access global markets more easily.
Streamlined Last-Mile Logistics: Collaboration between ocean freight forwarders and last-mile delivery companies has become essential to meet e-commerce demands. Efficient handoffs ensure timely delivery to consumers.
3. Sustainability in Shipping
Environmental concerns have driven the sea freight shipping industry to adopt greener practices. Over the past decade, sustainability has moved from being an optional consideration to a core focus.
IMO 2020 Regulations: The International Maritime Organization (IMO) introduced stricter sulphur emission limits in 2020, pushing carriers to switch to low-sulphur fuels or invest in scrubber technology.
Alternative Fuels: Shipping companies have begun exploring alternative fuels like liquefied natural gas (LNG), biofuels, and even hydrogen to reduce their carbon footprint.
Energy-Efficient Vessels: New ship designs incorporate advanced technologies, such as wind-assist propulsion and hull optimization, to improve fuel efficiency and reduce emissions.
4. Automation in Ports and Warehousing
The automation of ports and warehouses has significantly enhanced efficiency and reduced operational costs. Automated cranes, guided vehicles, and robotic systems have streamlined cargo handling and reduced human error.
Smart Ports: Ports like Singapore and Rotterdam have embraced automation and digitalization to become global hubs of efficiency. Smart technologies enable faster turnaround times and optimized cargo flow.
Automated Warehousing: The integration of automated storage and retrieval systems (ASRS) has improved inventory management and reduced lead times for cargo handling.
5. Challenges and Resilience
The past decade has also tested the resilience of the international shipping industry. Geopolitical tensions, trade wars, and the COVID-19 pandemic presented unprecedented challenges.
COVID-19 Disruptions: The pandemic caused widespread port closures, container shortages, and labour constraints, highlighting vulnerabilities in global supply chains. However, it also accelerated the adoption of digital solutions to enhance flexibility and resilience.
Trade Wars and Tariffs: Trade disputes, particularly between major economies, disrupted shipping routes and led to shifts in global trade patterns. Carriers had to adapt to changing demands and regulations.
6. The Role of Big Data
Big data analytics has become a cornerstone of decision-making in freight forwarding and ocean freight logistics. By analysing vast amounts of data, shipping companies can optimize routes, reduce costs, and improve customer satisfaction.
Demand Forecasting: Accurate demand forecasting helps carriers allocate resources efficiently, avoiding overcapacity and underutilization of vessels.
Customer Insights: Big data enables personalized services by analysing customer preferences and shipping histories.
7. Collaboration Across the Supply Chain
Collaboration between stakeholders has become essential to meet the complexities of modern logistics. Integrated platforms and partnerships have enhanced coordination and reduced inefficiencies.
Digital Freight Platforms: Digital platforms have revolutionized the booking process, providing instant international shipping quotes and comparisons to streamline decision-making.
Strategic Alliances: Carrier alliances, such as the Ocean Alliance and 2M Alliance, have allowed companies to pool resources and optimize routes, reducing costs and emissions.
8. Future Trends
Looking ahead, the ocean freight industry is poised for further innovation. Emerging technologies like autonomous ships, artificial intelligence, and advanced analytics will continue to shape the sector.
Autonomous Shipping: The development of unmanned vessels promises to reduce costs and improve safety. Although still in its infancy, autonomous shipping is expected to become more prevalent in the coming years.
Decarbonization Efforts: The industry will likely continue its push toward decarbonization, with an emphasis on zero-emission vessels and renewable energy integration.
Conclusion
The past decade has been a transformative period for ocean freight logistics. From digitalization and e-commerce growth to sustainability and automation, the industry has adapted to meet the demands of a rapidly changing world. These advancements have not only improved efficiency and transparency but also set the stage for a more sustainable future. As the industry continues to evolve, collaboration, innovation, and resilience will remain key to navigating the challenges and opportunities of the next decade.
Businesses shipping from China to Dubai or China to Singapore can leverage these advancements for smoother, more efficient operations. Whether you need international shipping quotes or reliable freight forwarding services, choosing a trusted ocean freightforwarder ensures success in global trade.
#freight forwarder#freight forwarding#international cargo shipping#international shipping#sea freight shipping#China to Dubai shipping#shipping from China to Dubai#shipping from China to Singapore#ocean freight forwarder#international shipping quote#LCL shipments.
0 notes