#DNA modelization in biomedical image
Explore tagged Tumblr posts
Text

Editing the Future
Jennifer Doudna – born on this day (19th February)– shared the 2020 Nobel Prize for Chemistry with Emmanuelle Charpentier for developing a genetic engineering technique called CRISPR-Cas9. Based on a naturally occurring defence system used by bacteria to expunge foreign DNA from their genome, CRISPR-Cas9 has revolutionised both biomedical and plant research readily revealing the impact of editing genes in living cells and model organisms, and is being applied in human genome editing to correct disease-causing gene faults and deliver gene therapies
Image by Christopher Michel, on Flickr
Image originally published with a Creative Commons Attribution 2.0 Generic (CC BY 2.0)
You can also follow BPoD on Instagram, Twitter and Facebook
#science#biomedicine#biology#chemistry#nobel prize#crispr#crispr cas9#genome editing#gene editing#born on this day
6 notes
·
View notes
Text
Noninvasive Technique Reveals How Cells’ Gene Expression Changes Over Time - Technology Org
New Post has been published on https://thedigitalinsider.com/noninvasive-technique-reveals-how-cells-gene-expression-changes-over-time-technology-org/
Noninvasive Technique Reveals How Cells’ Gene Expression Changes Over Time - Technology Org
MIT researchers can now track a cell’s RNA expression to investigate long-term processes like cancer progression or embryonic development.
DNA – artistic impression. Image credit: Image by kjpargeter on Freepik
Sequencing all of the RNA in a cell can reveal a great deal of information about its function and what it is doing at a given time. However, the sequencing process destroys the cell, making it difficult to study ongoing changes in gene expression.
An alternative approach developed at MIT could enable researchers to track such changes over extended periods of time. The new method, which is based on a noninvasive imaging technique known as Raman spectroscopy, doesn’t harm cells and can be performed repeatedly.
Using this technique, the researchers showed that they could monitor embryonic stem cells as they differentiated into several other cell types over several days. This technique could enable studies of long-term cellular processes such as cancer progression or embryonic development, and one day might be used for diagnostics for cancer and other diseases.
“With Raman imaging you can measure many more time points, which may be important for studying cancer biology, developmental biology, and a number of degenerative diseases,” says Peter So, a professor of biological and mechanical engineering at MIT, director of MIT’s Laser Biomedical Research Center, and one of the authors of the paper.
Koseki Kobayashi-Kirschvink, a postdoc at MIT and the Broad Institute of Harvard and MIT, is the lead author of the study, which appears today in Nature Biotechnology. The paper’s senior authors are Tommaso Biancalani, a former Broad Institute scientist; Jian Shu, an assistant professor at Harvard Medical School and an associate member of the Broad Institute; and Aviv Regev, executive vice president at Genentech Research and Early Development, who is on leave from faculty positions at the Broad Institute and MIT’s Department of Biology.
Imaging gene expression
Raman spectroscopy is a noninvasive technique that reveals the chemical composition of tissues or cells by shining near-infrared or visible light on them. MIT’s Laser Biomedical Research Center has been working on biomedical Raman spectroscopy since 1985, and recently, So and others in the center have developed Raman spectroscopy-based techniques that could be used to diagnose breast cancer or measure blood glucose.
However, Raman spectroscopy on its own is not sensitive enough to detect signals as small as changes in the levels of individual RNA molecules. To measure RNA levels, scientists typically use a technique called single-cell RNA sequencing, which can reveal the genes that are active within different types of cells in a tissue sample.
In this project, the MIT team sought to combine the advantages of single-cell RNA sequencing and Raman spectroscopy by training a computational model to translate Raman signals into RNA expression states.
“RNA sequencing gives you extremely detailed information, but it’s destructive. Raman is noninvasive, but it doesn’t tell you anything about RNA. So, the idea of this project was to use machine learning to combine the strength of both modalities, thereby allowing you to understand the dynamics of gene expression profiles at the single cell level over time,” Kobayashi-Kirschvink says.
To generate data to train their model, the researchers treated mouse fibroblast cells, a type of skin cell, with factors that reprogram the cells to become pluripotent stem cells. During this process, cells can also transition into several other cell types, including neural and epithelial cells.
Using Raman spectroscopy, the researchers imaged the cells at 36 time points over 18 days as they differentiated. After each image was taken, the researchers analyzed each cell using single molecule fluorescence in situ hybridization (smFISH), which can be used to visualize specific RNA molecules within a cell. In this case, they looked for RNA molecules encoding nine different genes whose expression patterns vary between cell types.
This smFISH data can then act as a link between Raman imaging data and single-cell RNA sequencing data. To make that link, the researchers first trained a deep-learning model to predict the expression of those nine genes based on the Raman images obtained from those cells.
Then, they used a computational program called Tangram, previously developed at the Broad Institute, to link the smFISH gene expression patterns with entire genome profiles that they had obtained by performing single-cell RNA sequencing on the sample cells.
The researchers then combined those two computational models into one that they call Raman2RNA, which can predict individual cells’ entire genomic profiles based on Raman images of the cells.
Tracking cell differentiation
The researchers tested their Raman2RNA algorithm by tracking mouse embryonic stem cells as they differentiated into different cell types. They took Raman images of the cells four times a day for three days, and used their computational model to predict the corresponding RNA expression profiles of each cell, which they confirmed by comparing it to RNA sequencing measurements.
Using this approach, the researchers were able to observe the transitions that occurred in individual cells as they differentiated from embryonic stem cells into more mature cell types. They also showed that they could track the genomic changes that occur as mouse fibroblasts are reprogrammed into induced pluripotent stem cells, over a two-week period.
“It’s a demonstration that optical imaging gives additional information that allows you to directly track the lineage of the cells and the evolution of their transcription,” So says.
The researchers now plan to use this technique to study other types of cell populations that change over time, such as aging cells and cancerous cells. They are now working with cells grown in a lab dish, but in the future, they hope this approach could be developed as a potential diagnostic for use in patients.
“One of the biggest advantages of Raman is that it’s a label-free method. It’s a long way off, but there is potential for the human translation, which could not be done using the existing invasive techniques for measuring genomic profiles,” says Jeon Woong Kang, an MIT research scientist who is also an author of the study.
Written by Anne Trafton
Source: Massachusetts Institute of Technology
You can offer your link to a page which is relevant to the topic of this post.
#aging#Aging news#algorithm#approach#Biology#biotechnology#Biotechnology news#blood#blood glucose#breast cancer#Broad Institute#Cancer#cell#cell types#Cells#change#chemical#Composition#data#deal#development#diagnostics#Diseases#DNA#dynamics#embryonic development#embryonic stem cells (ESC)#engineering#Evolution#Faculty
2 notes
·
View notes
Text
Biopreservation Market Key Companies, Growth and Forecast Report, 2030
The global biopreservation market size was valued at USD 2.18 billion in 2022 and is projected to grow at a compound annual growth rate (CAGR) of 28.30% from 2023 to 2030.
Biopreservation is experiencing growth due to various factors such as extensive usage in hospitals and labs, rising funding assistance from government and private partners in healthcare, an upsurge in research & development, and growing advancement in terms of product development. For example, in April 2021, BioLife Solutions launched a new high-capacity controlled freezer to cater to the cell and gene therapy market, which helped the company expand its existing product portfolio. The market experienced a major hindrance during the COVID-19 pandemic with supply chain issues, unavailability of raw materials, and hindrances in research activities among other reasons. However, the pandemic also accelerated the growth of novel biologics, and vaccine technologies. Due to these factors, opportunities for biologics manufacturing and preservation are anticipated to increase, thus generating further demand for biopreservation.
Biopreservation is a process that helps maintain the integrity and functionality of biological products such as stem cells, DNA, tissues, and organs under different temperatures, thereby prolonging their lifespan outside their natural environment. Technology innovations include microarrays or incorporation of a wide range of predictive models, such as hybrid models, and various API algorithms which help reduce the time, cost and complexity of the overall process. Furthermore, the advent of multiplex cellular imaging platforms is anticipated to enhance the biopreservation ability to help understand disease progression and suggest suitable diagnostic & treatment measures to follow, specifically for cardiology, gynecology, and point-of-care applications.
Gather more insights about the market drivers, restrains and growth of the Biopreservation Marke
Biopreservation Market Report Highlights
• By product, the equipment segment held the largest revenue share of over 78.75% in 2022 due to the rising demand for bio-banking to preserve stem cells, DNA, plasma, and tissue culture. Increasing demand for modified genetic material in the global market is also fueling the growth
• The bio-banking application segment held the largest revenue share in 2022. This can be attributed to rising awareness regarding stem cell preservation, increasing acceptance of sperm and egg banking, and utilization of assistant reproductive technology in animals
• North America dominated the global market in 2022 due to the advancement in biomedical research, the development of advanced therapies, and the upsurge in research activities related to DNA sequencing and bioinformatics
• Asia Pacific is expected to grow considerably in the future owing to rising investments in Asian countries and increasing partnerships and collaborations with key players
Browse through Grand View Research's Biotechnology Industry Research Reports.
• The global cancer stem cells market size was valued at USD 2.89 billion in 2023 and is projected to grow at a compound annual growth rate (CAGR) of 9.5% from 2024 to 2030.
• The global DNA methylation market size was valued at USD 1.47 billion in 2023. It is expected to expand at a compound annual growth rate (CAGR) of 14.1% from 2024 to 2030.
Biopreservation Market Segmentation
Grand View Research has segmented the global biopreservation market on the basis of product, application, and region:
Biopreservation Product Outlook (Revenue, USD Billion, 2018 - 2030)
• Equipment
o Freezers
o Refrigerators
o Consumables
o Vials
o Straws
o Microtiter Plates
o Bags
o Liquid Nitrogen
• Media
o Pre-formulated
o Home-brew
• Laboratory Information Management System (LIMS)
Biopreservation Application Outlook (Revenue, USD Billion, 2018 - 2030)
• Regenerative Medicine
o Cell Therapy
o Gene Therapy
o Others
• Bio-banking
o Human Eggs
o Human Sperms
o Veterinary IVF
• Drug Discovery
Biopreservation Regional Outlook (Revenue, USD Billion, 2018 - 2030)
• North America
o U.S.
o Canada
• Europe
o Germany
o U.K.
o France
o Italy
o Spain
o Denmark
o Sweden
o Norway
• Asia Pacific
o China
o Japan
o India
o Australia
o Thailand
o South Korea
• Latin America
o Brazil
o Mexico
o Argentina
• Middle East and Africa (MEA)
o South Africa
o Saudi Arabia
o UAE
o Kuwait
Order a free sample PDF of the Biopreservation Market Intelligence Study, published by Grand View Research.
#Biopreservation Market#Biopreservation Market size#Biopreservation Market share#Biopreservation Market analysis#Biopreservation Industry
0 notes
Text
Peptides with Fluorescent Labels
Different fluorescent dyes can be attached to peptides in different locations to label them. For a wide variety of imaging and diagnostic applications, fluorescent tags or probes are perfect. Applications for fluorescent peptide synthesis include localization investigations, peptide-protein interactions, enzyme activity assays, in vivo biomedical imaging research, and the creation of new disease models. Fluorescence microscopy and other fluorescence visualization methods can be used to see fluorochrome-conjugated peptides. An indirect method of labeling is to use an amino acid that has been biotinylated. The acceptor and quencher components may be joined inside or outside. For longer sequences, the internal connection is advised.
Studies on protein binding and localization, as well as in vivo biomedical imaging, typically make use of fluorescently tagged peptides. Fluorescence microscopy and other fluorescence visualization methods can be used to see fluorochrome-conjugated peptides. There are several different fluorescent labels. These labels can be inserted into your peptide at any desired location. Different fluorescent dyes can be attached to peptides in different locations to label them.
To meet your needs, we provide a variety of luminous labeling choices. The fluorescein derivative is a carboxyfluorescein that exhibits emission. The fluorescent dye is most frequently used to bind to peptides. Additionally, FAM is utilized with the majority of fluorescence-detecting tools. It can be linked to either the carboxy- or amino-terminal and is most frequently utilized in the pH range of 7.5 to 8.5. Nucleotides and nucleic acids are labeled and sequenced using this fluorescein. Rhodamine derivative stands for tetramethylrhodamine. It is most frequently utilized to make bioconjugates, like fluorescent antibodies used in cellular imaging and immunochemistry research. However, DNA sequencing and oligonucleotide tagging are other applications.
The biotech industry makes use of cyanine dyes. In fact, cyanine dyes are well-known for use in immunoassays, research in proteomics, transcriptomics, and genomic hybridization. Peptides, proteins, and antibodies are labeled with cyanine dyes for fluorescence detection methods like flow cytometry, microscopy, and microarrays.
0 notes
Text
Review on Applications of Genetic Engineering And Cloning in Farm animals-Juniper Publishers
JUNIPER PUBLISHERS-OPEN ACCESS JOURNAL OF DAIRY & VETERINARY SCIENCES
Abstract
Genetic engineering involves producing transgenic animal’s models by using different techniques such as exogenous pronuclear DNA microinjection in zygotes, injection of genetically modified embryonic stem cells into blastocysts and retrovirus mediated gene transfer. It is highly applicable and crucial technology which involves increasing animal production and productivity, increases animal disease resistance and biomedical application. Cloning involves the production of animals that are genetically identical to the donor nucleus. The most commonly applied and recent technique is somatic cell nuclear transfer in which the nucleus from body cell is transferred to an egg cell to create an embryo that is virtually identical to the donor nucleus. There are different applications of cloning which includes: rapid multiplication of desired livestock, animal conservation and research model. However, at present it is an inefficient process due to parturition difficulties, placental abnormalities and post-natal viabilities. Beside to this Food safety, animal welfare, public and social acceptance and religious institutions are the most common challenges for the development of this technology. In developing country including Ethiopia the science is not yet conceived and the concerned body should pay great attention to such valuable aspects of biotechnological advancements.
Keywords: Cloning; Genetic engineering; Nuclear transfer; Transgenic animal; Animal welfare
Abbreviations: DNA: Deoxy ribose Nucleic Acid; SCNT: Somatic Cell Nuclear Transfer; ES: Embryonic stem; EGF: Epidermal Growth factor; TGF: Transforming Growth Factor; MMA: Mastitis Metritis Agalactia; NT: Nuclear Transfer; FDA: Food and Drug Administration; MAS: Marker-Assisted Selection; MHC: Major Histocompatibility Complex
Introduction
Biotechnology has contributed to the genetic improvement of farm animals for decades, through artificial insemination and embryo transfers. The advent of modern biotechnology provides new avenues for genetic improvement in the production of farm animals. During the past decades, however, the term biotechnology has come to be associated more with molecular- based technologies, such as gene cloning and genetic engineering [1]. Now a days, biotechnology typically genetic engineering and cloning play an important role on both basic and applied research becoming an essential tool for the understanding of the biology and development of animal biotechnology. Such technology presents a wide range of applications, such as the production of biopharmaceuticals, studies on gene expression and its regulation, the improvement of animal production, production of herds resistant to specific diseases and many other biomedical and medical purposes [2].
Through the biotechnology of gene therapy, scientists are making efforts at curing genetic diseases by attempting to replace defective genes with the correct version and also used to produce more effective and efficient vaccines, therapeutic antibodies, antibiotics and other pharmaceuticals. There are more than 370 drug products and vaccines obtained through biotechnology currently in clinical trials, targeting more than 200 diseases including various cancers, Alzheimer’s disease, heart disease, diabetes, multiple sclerosis and arthritis [3].
A genetically engineered or transgenic animal is an animal that carries a known sequence of recombinant Deoxy ribose Nucleic Acid (DNA) in its cells, and which passes that DNA onto its offspring [4]. Recombinant DNA refers to DNA fragments that have been joined together in a laboratory. The resultant recombinant DNA construct is usually designed to express the proteins that are encoded by the genes included in the construct, when present in the genome of a transgenic animal. Because the genetic code for all organisms is made up of the same four nucleotide building blocks, this means that a gene makes the same protein whether it is made in an animal, a plant or a microbe. Proteins that have been expressed in transgenic animals include therapeutic proteins for the treatment of human diseases [5]; proteins that enable animals to better resist disease and proteins that result in the production of more healthful animal products (milk, eggs or meat) for consumers [6].
Cloning refers to producing genetically identical individual to donor cells and copying gene, which involves the creation of an animal or individual that derives its genes from a single other individual; it is also referred as asexual reproduction [7]. Cloned offspring in human and farm animals sometimes produced in nature when early embryo splits in to two (or sometime, more) species of just a few days after fertilization, before the cells have become too specialized. However, there are a number of artificial methods to produce genetically identical mammals. Of these, the nuclear cloning technology is considered to have the greatest potential application for animal agriculture and medicine [8].
Scientists have been attempting to clone animals through nuclear transfer of somatic cell (SCNT) for several decades. SCNT is an efficient way to create herds of genetically modified cloned animals, preservation of endangered species, production of human therapeutic proteins in genetically modified clone animals, use of genetically modified cloned animals as a source of organs for human transplantation, gaining a better understanding of cellular differentiation and reprogramming capabilities that could be the basis for human cellular therapies, and better models to study new treatments for human disease. However, SCNT cloning thus far has been very inefficient process and cloned animals have exhibited serious health problems [9].
The genetic engineering of animals has increased significantly in recent years, and the use of this technology brings with it ethical issues, some of which relate to animal welfare defined by the World Organization for Animal Health [10]. It is important to try to reflect societal values within scientific practice and emerging technology, especially publicly funded efforts that aim to provide societal benefits, but that may be deemed ethically contentious [11]. Even though, genetic engineering and cloning are interesting sciences with wide range of application, they have also some uncertainties and challenges and further investigation are needed to develop the technology. Therefore the objectives of this seminar paper area follow
• To review the application of genetic engineering and cloning,
• To highlight the concepts, techniques and challenges of genetic engineering and cloning.
Literature Review
Review History of genetic engineering and cloning time line
Genetic modification caused by human activity has been occurring since around 12,000 BC, when humans first began to domesticate organisms. Genetic engineering is the direct manipulation of an organism’s genome using certain biotechnology techniques that have only existed since the 1970s. Genetic engineering as the direct transfer of DNA from one organism to another was first accomplished by Herbert Boyer and Stanley Cohen in 1973 [12].
Human directed genetic manipulation was occurring much earlier, beginning with the domestication of plants and animals through artificial selection. The dog is believed to be the first animal domesticated, possibly arising from a common ancestor of the wolf. The first genetically modified animal was a mouse created in 1973 by Rudolf Jahnish [12]. Cloning research has been underway since the 1890s.The first animal cloning research was an attempt to produce identical organisms by splitting animal embryos at early stages of development. Work continued in the field of animal cloning and in 1952 the nuclear transfer procedure was invented. Work with nuclear transfer resulted in the successful cloning of many species from embryonic nuclei. In the 1980’s, nuclear transfer was used to clone cattle and sheep using cells taken directly from early embryos [13].
In 1995, living lambs, named Megan and Morag, were created for the first time from cultured cells. However, prior to 1997 the word clone conjured up images of creatures from Jurassic Park or other works of science fiction in the minds of most people. In July of 1996, Scottish scientists created the first animal cloned from an adult cell. On July 5, 1996, Dolly the sheep was born at the Rollin Institute in Edinburgh Scotland. The announcement of her birth in early 1997 shocked the scientific community and stirred debate over the possibility of cloning humans [14]. A process known as cell nuclear replacement created Dolly by transferring a mammary cell of a six-year-old white Welsh Mountain sheep into the egg cell of a Scottish blackface ewe. Since Dolly's birth, several other species have successfully been cloned including: mice, cattle, sheep, pigs, goats, rabbits and a cat [15] (Table 1).
Genetic engineering (transgenic animal) model
Genetic engineering is the name of a group of techniques used for direct genetic modification of organisms or population of organisms using recombination of DNA. These procedures are of use to identify, replicate, modify and transfer the genetic material of cells, tissues or complete organisms. Most techniques are related to the direct manipulation of DNA oriented to the expression of particular genes [16].
In a broader sense, genetic engineering involves the incorporation of DNA markers for selection (marker-assisted selection, MAS), to increase the efficiency of the so called traditional methods of breeding based on phenotypic information [17]. Transgenic animals can be created to gain knowledge of gene function and further decipher the genetic code, study gene control in complex organisms, build genetic disease models, improve animal production traits, and produce new animal products [18].
Technique of genetic engineering
Exogenous pronuclear DNA microinjection in zygotes:
Micro-injection is the first successful approach for the creation of transgenic animals based on the injection of a foreign DNA construct into a fertilized oocyte. The construct integrates randomly into the host oocyte genome, subsequently the zygote continues embryonic development and the embryo is transferred to a foster mother and eventually develops to a transgenic animal. However, this method has strong limitations: on average, less than 1% of embryos injected and 10% of animals born are transgenic, genes can only be added, not replaced or deleted, and multiple copies of the transgene are inserted at random, hindering the correct regulation of gene expression and possibly interfering with endogenous gene function. This requires large amounts of oocytes to be injected, as the overall efficiency of the process is very low [19].
Injection of genetically modified embryonic stem (ES) cells into blastocysts
Embryonic stem cells are derived from embryos at a very early stage (the blastula), and possess the important characteristic of pluripotency. Pluripotency is the ability of these cells to differentiate to any of the cell types and tissues found in the adult organism. Embryonic stem cells can be grown in culture for many passages and can be subjected to transformation with transgene constructs, resulting in modifications of their genome. The constructs used not only permit the selection of successfully transformed cells, but also allow gene targeting to be accomplished. Thus, genes can be specifically introduced, replaced or deleted (so-called knock-ins and knock-outs) [20].
Injection of genetically modified embryonic stem (ES) cells into blastocysts, mainly through the feature of gene targeting, allows a broad variety of genetic modifications to be introduced. For many years, several laboratories worldwide have tried to produce ES cells from farm animals, and although some success has been claimed, no robust and reproducible method has been published. Indeed, even in mice the production of ES cells is a costly and labor-intensive technology [18].
Retrovirus mediated gene transfer
Transgenesis may also be accomplished by employing virus- derived vectors, namely vectors based on the retrovirus-class of lenti viruses [21]. Genes that are essential for viral replication are deleted from the viral genome, maintaining only the capacity for integration of the viral genome into the host genome. The parts of the viral genes can replaced by the transgene of interest, then Viruses carrying the modified gene are produced in-vitro and subsequently injected into the perivitelline space of the zygote, resulting in infection of the zygote and integration of the viral genome into the host genome. Transgenesis rates reaching up to 100% of injected embryos have been described [22].
Major drawbacks of this method are a limited transgene size and random transgene integration. Random and possibly multiple transgene integration may lead to position effects, disturbance of the host genome and dose effects, as is the case with pronuclear injection. Solving these problems holds great promise for the further development and application of lentiviral vectors [22].
Uses of animal genetic engineering
Increase animal disease resistance
Genetic engineering of agricultural animals has the potential to improve disease resistance by introducing specific genes into livestock. Identification of single genes in the major histocompatibility complex (MHC), which influence the immune response, was instrumental in the recognition of the genetic basis of disease resistance/susceptibility [23]. The application of transgenic technology to specific aspects of the immune system should provide opportunities to genetically engineer livestock that are healthier and have superior disease resistance. One specific example where transgenesis has been applied to disease resistance in livestock is the attempt to produce cattle resistant to mastitis. Lysostaphin is an antimicrobial peptide that protects mammary glands against Staphylococcus aurous infection by killing the bacteria in a dose-dependent manner. Transgenic dairy cows that secrete lysostaphin into their milk have been produced to address the mastitis issue. The application of nuclear transfer technology, or cloning, will enable the augmentation of beneficial alleles and/or the removal (via gene knock-out) of undesirable alleles associated with disease resistance or susceptibility. By knocking-out the intestinal receptor for the K88 antigen lead to the absence of this antigen has been shown to confer resistance to infection of K88-positive E. coli [24].
Enhance growth and meat trait
Altering the fat or cholesterol composition of the carcass is valuable benefit that can be delivered via genetic engineering. By changing the metabolism or uptake of cholesterol and/ or fatty acids, the content of fat and cholesterol of meats, eggs and cheeses could be lowered. There is also the possibility of introducing beneficial fats such as the omega-3 fatty lipoprotein receptor gene and hormones like leptin are also potential targets that would decrease fat and cholesterol in animal products [6].
The use of genetic engineering to improve feed efficiency and/or appetite could profoundly impact livestock production and deliver significant benefits to producers, processors, and consumers. Increased uptake of nutrients in the digestive tract, by alteration of the enzyme profiles in the gut, could increase feed efficiency. The ability to introduce enzymes such as Phytase or xylanase into the gut of species where they are not normally present, such as swine or poultry, is particularly attractive [25].
The introduction of phytase would increase the bioavailability of phosphorus from phytic acid in corn and soy products. Golovan and his colleagues reported that the production of transgenic pigs expressing salivary phytase as early as seven days of age. The salivary phytase provided essentially complete digestion of the dietary phytate phosphorus in addition to reducing phosphorus output in waste by up to 75%. Furthermore, transgenic pigs required almost no inorganic phosphorus supplementation to the diet to achieve normal growth. The use of phytase transgenic pigs in commercial pork production could result in significantly decreased environmental phosphorus pollution from livestock operations [25].
Improve wool production
The control of the quality, color, yield and ease of harvest of hair, wool and fiber for fabric and yarn production has been an area of focus for genetic engineering in livestock. The manipulation of the quality, length, fineness and crimp of the wool and hair fiber from sheep and goats has been examined using transgenic methods. Transgenic methods also allow improvements to fiber elasticity, surface and strength. Decreasing the surface interactions between fibers could decrease shrinkage of garments made from such fibers [26].
Desired milk yield and composition
Advances in recombinant DNA technology have provided the opportunity either to improve the composition of milk or to produce entirely novel proteins in milk. These changes may add value to, as well as increase, the potential uses of milk. The improvement of livestock growth or survivability through the modification of milk composition requires production of genetically engineered animals that:
1) Produce a greater quantity of milk,
2) Produce milk of higher nutrient protein content. The major nutrients in milk are protein, fat and lactose.
Elevation of these components can improve growth and health of the developing offspring that consumer the enhanced milk [27].
Changing milk composition may improve animal growth is the addition or supplementation of beneficial naturally occurring hormones, growth factors or bioactive factors to the milk through the use of genetic engineering. It has been suggested that bioactive substances in milk possess important functions in the neonate with regard to regulation of growth, development and maturation of the gut, immune system and endocrine organs [28]. Transgenic alteration of milk composition has the potential to enhance the production of certain proteins and/or growth factors that are deficient in milk. The increased expression of a number of these proteins in milk may improve growth, development, health and survivability of the developing offspring. Some of these factors are insulin-like growth factor 1 (IGF-1), epidermal growth factor (EGF), transforming growth factor (TGF) and lactoferrin [29].
The properties’ of milk that bear consideration for modification are those that affect human and animal health. It has been shown that specific antibodies can be produced in genetically engineered animals. It is possible to produce antibodies in the mammary gland that are capable of preventing mastitis in cattle, sheep and goats and mastitis metritis agalactia (MMA) in pigs, and/or antibodies that aid in the prevention of domestic animal or human diseases. Other role is to increase proteins that have physiological roles within the mammary gland itself such as lactalbumin, lysozyme, lysostaphin or other antimicrobial peptides.
It is important to consider the use of transgenics to increase specific components, which are already present in milk for manufacturing purposes. An example might be to increase one of the casein components in milk. This could increase the value of milk in manufacturing processes such as production of cheese or yogurt. One might also alter the physical properties of a protein such as casein [30].
Human cell-based therapies
Direct applications of Nuclear Transfer (NT) technology in human therapies, principally therapeutic cloning as opposed to human reproductive cloning [31]. Patients with diseases or disorders in tissues like insulin-dependent diabetes, muscular dystrophy, spinal cord injury, certain cancers and various neurological disorders, including Parkinson’s disease could potentially generate their own immunologically compatible cells for transplantation, which would offer lifelong treatment without tissue rejection [32]. Initially, this approach could employ human NT and embryonic stem cells but, the use of this technique in human is controversial. In the longer term, however, fundamental understanding of reprogramming will enable one cell type to be directly trans-differentiated into another cell type specifically required for cell-based therapy [33].
One major application of animal transgenesis is the production of pharmaceutical products, also known as animal pharming. Since many human proteins cannot be produced in microorganisms and production in cell culture is often laborintensive with low yields, the production of biopharmaceuticals in transgenic animal bioreactors is an attractive alternative [34]. Many human proteins cannot be produced in micro-organisms, since they lack post-translational modification mechanisms that are essential for the correct function of many human proteins. Pharmaceutical proteins or other compounds can be produced in a variety of body fluids, including milk, urine, blood, saliva, chicken egg white and seminal fluid, depending on the use of tissue-specific promoters [35].
Protein based drug
Protein-based drugs differ from protein products synthesized in the blood in that they are produced in-vivo by other organs. This technology is even being applied to the development of complex proteins such as monoclonal antibodies as well as many other important human replacement proteins and protein drugs such as polyclonal antibodies and plasminogen activator [3]. Researchers recently created a line of transgenic swine that produce recombinant human erythropoietin a naturally occurring human hormone that boosts the body’s production of red blood cells. The transgenic swine produced the hormone in their milk through a potentially more efficient and lower cost process than traditional methods patients with diseased kidneys no longer able to produce the protein, as well as cancer patients being treated with chemotherapy who develop anemia as a consequence of bone marrow depletion from their cancer drug regimens. Erythropoetin-based drugs are some of the most widely used protein-based drugs, and are expensive to manufacture [36].
Xenotransplantation
Xenotransplantation is the transplantation of organs or cells from one species to another. Human to human transplantation are sometimes difficult due to scarcity of donor organ. Pig is considered the preferred candidate for xenotransplantation because of physiological compatibility and breeding characteristics. Large numbers of pathogen free pigs can be raised to provide organs for transplantation in to humans [37]. However, one of the problems associated with using pig organs for xenotransplantation is that the immune system of the human recipient attacks the transplanted organ, causing transplant rejection. Pigs naturally produce a sugar, called a1, 3-galactosyltransferase (aGalT) on the surface of their cells, which the human immune system recognizes as foreign [38]. The human immune system then forms antibodies to attack the cells which produce that sugar, resulting in tissue rejection. Through the use of genetic engineering and cloning, scientists have created pigs which are deficient for aGalT and do not produce it on the surface of their cells. Transfer of these genetically engineered tissues and organs into baboon recipients has increased the length of time before the organs are rejected by the recipient’s immune system.
Techniques of cloning
Cloning is a powerful technique by producing genetically identical individuals and potentially it could be used for multiplication of elite animals and minimizes the genetic variation in experimental animals. It can be used for the conservation as well as propagation of endangered species. It may be used also as a tool for the production of stem cells for therapeutic purposes, as therapeutic cloning. Cloning using somatic cells offers opportunities to select and multiply animals of specific merits. Cloned offspring in humans and animals are sometimes produced in nature when the early embryo splits in two (or sometimes, more) pieces just a few days after fertilization, before the cells have become too specialized. However, there are also a number of artificial methods to produce genetically identical mammals. Of these, the nuclear cloning methodology is considered to have the greatest potential application for animal agriculture and medicine [8].
Somatic Cell Nuclear Transfer (SCNT)
The transfer of a cell nucleus from a body cell into an egg from which the chromosomes have been removed or inactivated; is method used for cloning of organisms. Once the genome transferred with the egg cell then one cell embryo is created and the process of cloning is completed and further development of the clone can occur [39] (Figure 1).
Embryo splitting (embryo twining)
Embryo splitting may be considered the first true cloning procedure involving human intervention, and was first described by Willesden and Polge in 1981, when monozygotic twin calves were produced. Embryo splitting or the mechanical separation of cells can be used in very early embryos. Two-cell embryos derived from either in vitro fertilization or embryo rescue following in vivo fertilization are held in place with micropipettes under a microscope.
The zonapellucida (the clear layer of protein surrounding the oocyte and fertilized ovum) of these embryos is opened, and the two-celled embryo is then split into individual cells with a finely drawn needle or pipette. One of the cells is left in the original zonapellucida and the other is either placed into an empty zonapellucida or allowed to develop without a zonapellucida. These so-called demi-embryos can be cultured in vitro for a few days, inspected for appropriate growth and then transferred directly to synchronized recipient dams or frozen for future use (Figure 2).
Application of cloning
Rapid multiplication of desired livestock: Cloning could enable the rapid dissemination of superior genotypes from nucleus breeding flocks and herds, directly to commercial farmers. Genotypes could be provided that are ideally suited for specific product characteristics, disease resistance, or environmental conditions. Cloning could be extremely useful in multiplying outstanding F1 crossbred animals, or composite breeds, to maximize the benefits of both heterosis and potential uniformity within the colonal family [8]. These genetic gains could be achieved through the controlled release of selected lines of elite live animals or cloned embryos. More appropriately, given that cloning is not particularly efficient at present: a niche opportunity exists in the production of small numbers of cloned animals with superior genetics for breeding. These could be clones of performance tested animals, especially sires. This would be particularly relevant in the sheep and beef industries, where cloned sires could be used in widespread natural mating to provide an effective means of disseminating their superior genetics. This could be used as a substitute for artificial insemination, which in these more extensive industries is often expensive and inconvenient [40].
Animal conservation: Cloning can be used along with other forms of assisted reproduction to help preserve indigenous breeds of livestock, which have production traits and adaptability to local environments that should not be lost from the global gene pool. In some situations, inter-species NT and embryo transfer may be used to aid the conservation of some exotic species. At the very least, it is appropriate to consider the cryopreservation of somatic cells from these endangered animals as insurance against further Research model losses in diversity. Cryobanking of somatic cells from rare and endangered birds and animals against further losses of diversity or possible extinction of Wildlife to preserve endangered indigenous breeds of livestock adapted to particular environments [41].
Research model
Sets of cloned animals could be effectively used to reduce genetic variability and reduce the numbers of animals needed for some experimental studies. This could be conducted on a larger scale than is currently possible with naturally occurring genetically identical twins [42]. Lambs cloned from sheep selected either for resistance or susceptibility to nematode worms will be useful in studies aimed at discovering novel genes and regulatory pathways in immunology [43].
Problems and prospects of animal cloning
Placental abnormalities: A failure of the placenta to develop and function correctly is a common feature amongst clones. The majority of early pregnancy failures, before placentome formation, are attributed to an inadequate transition from yolk sac to allantoic-derived nutrition, with poor allantoic vascularisation in sheep [44]. Furthermore, there is reported evidence of immunological rejection contributing to early embryonic loss. Typically in cattle, 50% to 70% of pregnancies at day 50 are lost throughout the remainder of gestation and up to term. This is in stark contrast to only 0% to 5% loss with artificial insemination or natural mating over the same period. In extreme cases, placentomes are entirely absent at day 50. Shortly thereafter, these pregnancies fail. More commonly, cloned placentae only have half the normal number of placentomes, display compensatory overgrowth and are oedematous. Of particular concern are the losses in the second half of gestation; especially the occurrence of hydroallantois, i.e. the excess accumulation of fluid within the allantoises [45].
Post-natal viability: The viability of cloned offspring at delivery and up to weaning is reduced compared to normal, and this is despite greater than usual veterinary care. Data from our group shows that around 80% of cloned calves delivered at term are alive after 24hours [46]. Two-thirds of the mortality within this period is due to a spinal fracture syndrome through the cranial epiphyseal plate of the first lumbar vertebrae or to deaths that occurred either in utero or from dystocia. Surviving newborn clones have altered neonatal metabolism and physiology, possibly due to placental abnormalities, and it takes time for these processes to adjust to normal [47].
At Agriculture Research, typically an additional 15% of calves initially born alive die before weaning. In our experience, the most common mortality factors during this period are gastroenteritis and umbilical infections. Other abnormalities noted include defects in the cardiovascular, musculoskeletal and neurological systems, as well as susceptibility to lung infections and digestive disorders. Hydronephrosis is particularly common in sheep, with correspondingly elevated serum urea levels in some surviving clones.
Parturition difficulties: Intervention is often deemed necessary to deliver cloned offspring, as intervention gestation length in NT pregnancies is typically prolonged and the birth weight of cloned calves may be 25% heavier than normal. Newborn cloned calves display adrenal glands, so this extended gestation may be due to failure of the placentae to respond to foetal cortisol near term or to a lack of adreno corticotropic hormone release from the foetus. Oversized cloned offspring add to the birth complications. They are larger than artificially inseminated or naturally-mated controls. It has been reported that somatic cloned calves are heavier than embryonic clones. At Agriculture Research, the occurrence of prolonged gestation and the risk of dystocia initially prompted the delivery of clones by elective caesarean-section, following a brief exposure to exogenous corticosteroids.
Public opinion and food safety to genetically engineered and cloned animal: Public opinion against cloning is apparent throughout the world. According to The Euro Barometer poll conducted in 2008, 84% of European Union citizens feel that the long-term effects of animal cloning on nature were unknown. The same poll also revealed that 61% European Union citizens of citizens believe the cloning of animals to be morally wrong (The Gallup Organization, 2008). A2005 Pew Initiative on Food and Biotechnology poll found that two-thirds of United States consumers indicated that they are uncomfortable with animal cloning in general. An earlier Gallup poll reportedly found that two-thirds considered animal cloning morally wrong [48].
Cloning has given rise to a massive ethical debate, including reports by bioethics committees and many books and articles. There are few enthusiastic advocates of cloning, but a number of bioethicists have tried to show that popular responses and even the more sophisticated philosophical arguments against cloning are naïve, and cannot be sustained. These commentators have argued that people's opposition to cloning is a Yuk reaction, which cannot stand up to reasoned argument. In a similar, defensive way, liberals have argued that while cloning may not be very desirable, it should not stop other people from doing it, because that would interfere with freedom [49].
The composition of food products derived from clones have found that they have the same composition as milk or meat from conventionally-produced animals [50]. Milk and meat from clones produced by embryo splitting and nuclear transfer of embryonic cells have been entering the human food supply for over 20 years with no evidence of problems. However, in 2001, the Center for Veterinary Medicine at the Food and Drug Administration (FDA) determined that it should undertake a comprehensive risk assessment to identify hazards and characterize food consumption risks that may result from Somatic cell nuclear transfer (SCNT) animal clones and therefore asked companies not to introduce these cloned animals, their progeny, or their food products (milk or meat) into the human or animal food supply. As there is no fundamental reason to suspect that clones will produce novel toxins or allergens, the main underlying food safety concern was whether the Somatic cell nuclear transfer (SCNT) cloning process results in subtle changes in the composition of animal food products [43].
Although the amount of data describing the health of the progeny of clones is more limited than the amount describing the health of animal clones themselves, there is an underlying biological assumption behind the predicted health and resultant food safety of the sexually-produced progeny of clones. The genetic remodeling process that occurs during gametogenesis (i.e. the production of eggs and sperm), is thought to naturally reset any epigenetic anomalies that might result from the cloning process. Sexual reproduction effectively corrects any programming errors that may have been introduced into the cloned parent’s DNA, thereby resulting in the production of normal gametes and offspring. This assumption is supported by a study in mice where it has been observed that abnormalities present in cloned mice are not passed on to their sexually- derived progeny. In addition, observations on the relatively small number of progeny of bovine and swine clones that have been born support the premise of normal development [51].
Ethical issue of genetic engineering and cloning in respect to animal welfare
Some scientific, governmental and religious organizations oppose reproductive cloning since serious ethical concerns have been raised by the future possibility of harvesting organs from clones [51]. The majority of religious organizations distinguish between reproductive and therapeutic cloning. Since cloning is an unnatural born of an individual, no one has the right to undertake it except God. Many embryos develop abnormally and die in utero, while others may be infertile or born with developmental defects, some of which are attributable to these so-called insertional problems [52]. Still other health issues may not become apparent until later in life. Transgenic animals often exhibit variable or uncontrolled expression of the inserted gene, resulting in illness and death [53]. In one study, ten transgenic piglets were followed from birth through puberty. Half of the animals died or had to be euthanized due to severe health problems during the investigation, indicating a high mortality rate among genetically engineered piglets. In addition, three of the surviving piglets showed decreased cardiac output [54].
The genetic modification of sheep containing an extra copy of a growth hormone gene resulted in animals who reportedly grew faster, leaner, and larger than those conventionally bred; produced more wool; or produced milk for prolonged periods. Developing more economically profitable sheep reportedly resulted in negative welfare side effects from the excess growth hormone, including increased incidences of diabetes and susceptibility to parasites [55]. Cloning research also reveals abnormalities and high failure rates, problems widely acknowledged by scientists in the field and potentially indicative of poor animal welfare [56]. Seemingly healthy bioengineered animals are at risk for a variety of defects. All cloned babies have some sort of error. The list of problems from which clones have suffered is extensive, including diabetes, enlarged tongues, malformed faces, intestinal blockages, shortened tendons, deformed feet, weakened immune systems, respiratory distress, circulatory problems, and dysfunctional hearts, brains, livers, and kidneys.
Future perspectives of transgenesis
The techniques for obtaining transgenic animals in species of agricultural interest are still inefficient. Some approaches that may overcome this problem are based on cloning techniques. Using these techniques it is feasible to reduce to less than 50% the number of embryo receptor females, which is one of the most important economic limiting factor in domestic species. It would also facilitate the further proliferation of transgenic animals. Recent results relate these techniques with still low success rates [57], high rates of perinatal mortality and variable transgenic expression that requires to be evaluated before generalizing their application. Considerable effort and time is required to propagate the transgenic animal genetics into commercial dairy herds. Rapid dissemination of the genetics of the parental animals by nuclear transfer could result in the generation of mini herds in two to three years. However, the existing inefficiencies in nuclear transfer make this a difficult undertaking. It is noteworthy that the genetic merit of the 'cloned’ animals can be fixed, while continuous genetic improvements is introduced in commercial herds by using artificial insemination breeding programs [58].
In an alternative scenario of herd expansion, semen homozygous for the transgene may be available in four to five years. Extensive breeding programs will be critical in studying the interaction and co-adaptation of the transgene(s), with the background polygenes controlling milk production and composition. Controlling inbreeding and confirming the absence of deleterious traits so that the immediate genetic variability introduced by transgenesis is transformed into the greatest possible genetic progress is equally critical.
Cloning in Africa
The first healthy cloned calf in South Africa in 2003 was successful called Futhi [59]. It is African's first cloned (nuclear transferred) healthy calf, produced with handmade cloning. In situations regarding our country, there is no any reliable research conducted on genetic engineering and cloning. It is also difficult to perform or apply the technology not only because of technological insufficiency and financial limitation but also lack of skill and knowledge [60-62].
Conclusion and Recommendation
Genetic engineering is the processes of producing genetically modified animals by using different techniques such as exogenous pronuclear DNA microinjection in zygotes, injection of genetically modified embryonic stem cells into blastocysts and retrovirus mediated gene transfer. It has advanced application in various sectors including increased animal production and productivity, increase animal disease resistance and biomedical application. Cloning is the process of producing genetically identical individual to the donor cells by using different techniques such as somatic cell nuclear transfer and embryo splitting [63-65]. It has various applications such as rapid multiplication of desired livestock, animal conservation and research model [66,67]. Even if, transgenic and cloned animals have wide range application and the science is very interesting and valuable, many challenges from the food safety, animal welfare, public and social acceptance points of view, socio-cultural and religious obstacles and technical inefficiency are headache for the technology [68].
In most of developing country including Ethiopia have no attempt of animal genetic engineering and cloning which could be attributed to lack of knowledge and skill, technological insufficiency as well as financial limitation. Therefore; based on the above conclusion the following recommendations are forwarded:
a. Further study and research are needed to be conducted to improve the techniques and increase the successes rate of genetic engineering and cloning.
b. Much work is to be done on creating public awareness on genetic engineering and animal cloning to avert the sociocultural and religious problems facing this technological advancement.
c. Veterinarian should acquire basic knowledge about the application and techniques of animal genetic engineering and cloning and this should be part of the academic curriculum.
d. Concerned organizations/institution in developing country including Ethiopia should be involved in technology transfer researches and make use of the advantages of animal genetic engineering and cloning.
For more Open Access Journals in Juniper Publishers please click on: https://juniperpublishers.com
For more Open Access Journals in Juniper Publishers please click on: https://juniperpublishers.com/open-access.php
For more articles in Open Access Journal of Dairy & Veterinary sciences please click on: https://juniperpublishers.com/jdvs/index.php
For more Open Access Journals please click on: https://juniperpublishers.com
To know more about Juniper Publishers please click on:
https://juniperpublishers.business.site/
1 note
·
View note
Text
The Cardio Kinesiograph System
Authored by *
Abstract
The Cardio Kinesiograph System (CKinG) is a novel computerized diagnostic system incorporating a computer model of cardiac kinesis. Cardiac Kinesis (CK) is the interpretation of heart movement or electrical activity of specialized cardiac muscle cells in response to biochemical reactions. The mechanical events occurring during the cardiac cycle consist of changes in pressure in the ventricular chamber which cause(s) blood to move in and out of the ventricle [1]. The events of the cardiac cycle start with an electrical signal and proceed through excitation-contraction coupling (which involves chemical and mechanical events) to contraction of the ventricle (pressure generation) and ejection of blood (flow) into the pulmonary and systemic circulations [2]. Thus, we can characterize the cardiac cycle by tracking changes in ventricular volume (LVV), ventricular pressure (LVP), left atrial pressure (LAP) and aortic pressure (AoP) [3]. In the other hand, because electrical events always precede mechanical events in the cardiac cycle, distortions of a part or parts of the electrical signal have been used as diagnostic indicators of both electrical and mechanical dysfunctions of the heart muscle [4]. There are numerous methods and technical systems available for diagnosis or predict heart disease. However, medical errors and undesirable results [5] in these systems are reasons for a need for conventional computer-based diagnosis of heart diseases systems. The purpose of this study is to design and develop a system that can observe and analyze Kinesiographical Cardiac basted on statistical models and identifies some fundamental characteristic of heart motions.
Keywords: Cardiovascular biomechanics; Statistical analysis methods; Medical heart imaging modalities; DNA modelization in biomedical image
Introduction
Since 1950 cardiologists have studied the functions of heart moments to employ them in the diagnostics of ischemic heart disease (IHD). Indeed, changes of the movements have found their diagnostic application in this field. If blood supply to a certain area of ventricular myocardium is insufficient the con-tractions in this area diminish and even ceases. After systolic increase in ventricular pressure this area dilates and forces intercostal tissues out, causing a “bulge” wave on the record.
Cardiokymography was one of several noninvasive techniques able to detect coronary artery disease. It can qualitatively determine abnormal left ventricular motion, and, based on animal models, this can be directly related to abnormalities in the left coronary artery [7]. Nevertheless sensitivity of cardiokymography in detecting patients with ischaemic left ventricular wall motion abnormalities depended on the extent of left ventricular ischaemia [8]. Cardiokymography is no longer valid [9]. Today Computer Aided Diagnosis (CAD) is one of the trusted methods in the field of medicine [10]. Advances in medical imaging and image processing techniques have greatly enhanced interpretation of medical images. Computer aided diagnosis (CAD) systems based on these techniques play a vital role [11] in the early detection of cardiovascular diseases and hence reduce death rate. CAD is the most preferable method for the initial diagnosis of heart disease. The combination of Digital and Medical Image Pro-cessing, Cardiac Electrophysiology, Ventricular Pressure-Volume Technique and Phonocardiogram etc makes the CAD system more reliable and efficient. One example of the medical applications in computer aided diagnosis is the detection system for heart disease based on Cardiovascular Magnetic Resonance (CMR). Cardiovascular magnetic resonance of the heart provides a potentially useful way to assess cardiac mechanical function. Besides CMR, positron emission tomography (PET), and cardiac CT are able to illustrate kinesis of cardiac muscles. Perfusion imaging with cardiac PET is used clinically to produce images of myocardial blood flow, aiding the diagnosis of coronary artery disease and the monitoring of condition of coronary circulation in response to treatment [12].
Clinical imaging in positron emission tomography (PET) is often performed using single-time-point estimates of tracer uptake or static imaging that provides a spatial map of regional tracer concentration [13]. However, dynamic cardiac techniques (e.g. PET, Myocardial perfusion imaging and Dynamic cardiac SPECT [14]) are used to estimate rate parameters activity of myocardial blood flow, and there are limited studies evaluating the role of Cardiovascular Magnetic Resonance and cardiac PET and cardiac CT for the assessment of cardiac kinesis. Therefore, scientific communities in computational cardiovascular science [15] have contributed to developing mathematical models and algorithms to improve efficiency of cardiac safety data management in clinical trials. In this way in 2014 proposed a new method based on a mathematical model, “Fourier Transform” which calculates an amplitude parametric image for the assessment of cardiac kinetics. This image, calculated from the Cine MR images, allows the localization and quantification of abnormalities related to difference in contraction and their extent [16]. In 2015 Zakynthinaki [17] has also presented effective mathematical model of heart rate kinetics in response to movement. She made conclusion that the new model is able not only to provide important information regarding an individual’s cardiovascular condition but to also simulate and predict heart rate kinetics for any given exercise intensities. The existing models of cardiac kinetics focus mainly on amplitude images or are limited to simulation of biological transformation. The present study provides a novel mathematical model of cardiac kinesis based on visual presentation of numerical data (obtained through the cardiac kinesis) in the form of graph, with a particular focus on Human Cardio Kinesiograph Analysis. The purpose of this study was to de-sign, develop and evaluate a novel method for Kinesiograph of Cardiac for the clinical assessment of cardiac and vascular function.
Methods and Materials
The system includes the following steps:
Data collection: In order to obtain an accurate data, the Cardiovascular Magnetic Resonance imaging (CMR), Cardiovascular Ultrasound or Cardiac Computed Tomography are produced better performances for detection of cardiac mobility. However, CMR is provided the most comprehensive anatomic picture for patient selection [18]. The system therefore is obtained relevant information from the CMR.
Video quality assessment: Different medical imaging methods may introduce common artifacts include image distortion, signal pileup (bright regions), and image dropout (area without signal) [19], therefore the quality assessment is an important factor at the operational level. The assessment of quality of video depends upon the type of distortion [20]. Numerous video quality assessment methods and metrics have been proposed over the past years with varying computational complexity and accuracy [21]. We utilize different forms of quality assessment methods, however the merit of these methods is often judged by assessing the quality of a set of results through lengthy user studies [22].
Motion estimation and inertial measurements: Motion estimation is the process of determining the movement of blocks between adjacent video frames (MathWorks). Efficient and accurate motion estimation is an essential component in the domains of image sequence analysis [23] and medical video processing. The estimation of motion is also important from the viewpoint of matching metric technique, which is computed the context similarity between two images. There exist several methods for motion estimation image, and video processing (e.g. pixel-based motion estimation, block-based motion estimation, optical flow method). In this study in order to increase the computational accuracy and improve efficiency in solving problem, DNA Modeling (Dawoudi, 2017) method in biomedical image matching has been proposed. The method is based on the linear mapping and the one-to-one correspondences between point features extracted from the frames and on calculating similarities in pixel values. This correspondence is determined by comparing two strings constructed from pixel values of the frames. The method uses a table called the Quarter Code table, which is the set of characters and numbers. In this table every number between 0 and 255 is translated into a unique string of four letter alphabet. Letters A,C,G,T are chosen, since they are the same as used in DNA sequences. In this way it possible to utilize tools originally programmed to DNA sequences analysis. When all pixel values of the frames (images) are converted to virtual DNA sequences, one can show the differences between two virtual DNA sequences.
Visual representation of numerical data in the form of graphs: The E-value gives a measure of the similarity of sequences. From this function we can obtain the correlation coefficient which will give us a single value of similarity. The rate of similarity between sequences (frames) is plotted as a graph and it’s appearing in the Monitor.
Experiment Results
We demonstrate the system by performing experiment 2D cardiac CMR video (Source: HBSNS library). The practical framework consists of four steps:
A. Step 1: Extract frames from cardiac mri video
The information is obtained by extracting frames from CMR imaging video. There are different tools in order to extract frames from cardiac MRI Video (e.g. Free Video to JPG Converter, VLC or Virtual Dud) (Figure 1).
B. Step 2: Adjacent frames comparisons
The difference between two adjacent frames is used to estimate motion direction and magnitude. This process has been implemented within a tool called Image Diff. The Perforce image diff tool enables researchers to compare two adjacent frames. The following represents pixels difference value (Percent Changed; Pixels) and color difference value (Percent Change; Color) between adjacent Frames (Table 1).
C. Step 3: Presenting data in graphic form
In the final step the percentage of change (pixels and color) from one value to another, between frames are plotted as a graph (Figure 2 & 3).
Results
The results show that the proposed design approach works efficiently in the Cardio Kinesis System for clinical applications of cardiovascular assessment.
Conclusion
In conclusion, the proposed Cardio Kinesiograph System (CKinG) may become a robust and efficient tool for the clinical assessment of Cardiac and Vascular function. CKinGbased Computer-Aided Diagnosis (CAD) holds the promise of improving the diagnosis accuracy and reducing the cost.
Discussion
The developed system can be used as a prototype in the clinical sectors for the evaluation of cardiovascular diseases. However, a novel method for real time MRI of cardiac kinesis and simultaneously Kinesiographical Cardiac Analysis Based on Statistical Methods are proposed. The proposed methods may become efficient Medical Diagnostic Support tools (DSTs) for Heart Diseases.
Related Work
The publications below are based on Morbid Motion Monitor related topics:.
A. Dawoudi Mohammad Reza (2017) Morbid Motion Monitor. Current Treads in Biomedical Engineering & Biosciences. ISSN: 2572-1151.
B. Dawoudi MR (in press) (2017). Nursing and Technology foresight in Futures of a Complex World. European Journal of Futures Research.
To Know More About Current Trends in Biomedical Engineering & Biosciences Please Click on: https://juniperpublishers.com/ctbeb/index.php
To Know More About Open Access Journals Publishers Please Click on: Juniper Publishers
#Cardiovascular biomechanics#Statistical analysis methods#DNA modelization in biomedical image#Biomedical Engineering#biochemistry
0 notes
Text
Biomed Grid | Origin, Phylogeny and Natural Behavior of Mice: What is Their Influence on Welfare During their Maintenance in the House Facilities?
State of the Art
The mouse is an extremely interesting species. Its image has historically been associated with a plague (or pest) and a disease transmitter. Paradoxically, it has a high empathy for children, where we can find various entertainment products using the image of the mouse (toys, cartoons and others ...). However, his animal has a remarkable capacity for adaptation and survival that are extremely important for the discovery and treatment of diseases, especially for humans [1]. Currently, the number of publications indexed using the term “mouse” (or the keyword Mouse) on the PubMed (US National Library of Medicine Institutes of Health) website was 1.628, 275 publications, together with the “rat” (Rat = 1.690, 355 publications) the bio models with the highest number of scientific productions compared to other rodent species and lagomorph models:
a. Hamster (125.711 publications).
b. Guinea pig (149.049 publications).
c. Rabbits (348.520 publications) (Access: September 2019).
Highlight that the use of any animal species, including the mouse, is an activity that requires a thorough knowledge and study of ethical basis, in addition to the knowledge and with the legislation of each country, regarding the use of animals for purposes didactic and scientific [1]. In general, in all countries, the objective of applying ethical principles and legal regulation is primarily aimed at replacing animals with validated alternative methods that may replace their use. If this is not possible, the development of equipment, techniques and methodologies that can refine the use of animals should be emphasized, and all efforts should be related to the maintenance, promotion and elevation of animal welfare during biomedical assays [2]. In Brazil, our legal framework in the area of laboratory animal science, after 12 years in the National Congress, was the approval of Law No.11.794 [2]. Through this law the National Council for Control of Animal Experimentation (CONCEA) was structured on 2015 [2].
CONCEA Normative Resolution (RN) No 25 which describes the “General Introduction” chapter of the Brazilian Guide to the Production, Maintenance or Use of Animals for Teaching or Scientific Research Activities [3] and RN No 30 (2016), which establishes the Brazilian Directive for the Care and Use of Animals in Teaching or Scientific Research Activities (DBCA) which presents the principles and conducts that ensure the care and ethically correct management of animals produced, kept or used in teaching or scientific research activities in Brazil [4]. Finally, RN No 33 (2016), which proposes the chapter “Procedures - Rodents and Lagomorphs kept in facilities of educational or scientific research institutions” of the Brazilian Guide to the Production, Maintenance or Use of Animals in Animal Activities. Teaching or Scientific Research, in which it describes and guides animal welfare strategies in animal houses, such as care to consider for the welfare of rodents and lagomorphs [3,4]. In all this report, especially in RN No 33, we emphasize that the relationship between the performance of procedures and the welfare of rodents and lagomorphs, in our case highlighting the Mus musculus biomodel, imparts in certain sections that: “It is essential to know the biology of the species, race, lineage to work with, since the basic needs (physical, emotional or behavioral) to be met are not the same for all animals.” and “… promote the expression of speciesspecific natural behaviors and a decrease, if not the disappearance, of abnormal behaviors.It should be based on promoting a positive effect on the physical and psychological welfare of the animal “[3].
We emphasize that the excerpts “to know species biology (physical, emotional or behavioral)” and “promote the expression of species-specific natural behaviors and a decrease, if not disappearance, of abnormal behaviors” were the motivating factors of this work [3]. Our aim was based on the question: How to know the physical, emotional or behavioral needs and the possibility of the expression of species-specific natural behaviors in order to provide the welfare of mice in the house facilities? Thus, we carry out a detailed bibliographic from the mouse origin of the species and its phylogeny, the knowledge about its geographical distribution, its habitat and natural behavior until the beginning of the controlled creation for the use in the scientific assays so that we can demonstrate, through scientific results, how we can approach the physical, emotional and behavioral needs of the space- restrain mouse in our animal house facilities and during biomedical trials by maintaining or enhancing animal welfare.
Mouse: Origin of the Species
Some authors state that there is a divergence in paleontological and phylogenetic studies regarding time estimates when dealing with lineage diversification rates, evolutionary patterns and historical biogeography, among other factors [5,6]. Fossil data is the most common type of paleontological calibration used to estimate the time period of the descendant in the phylogenetic tree. However, as described by Graur & Martim [7], Dos Reis & Yang [8] the incorporation of fossils for phylogenetic tree structuring can present difficulties such as:
i. The integrity of the fossil record
ii. The accuracy of the age of fossils
iii. The correction of the placement in the phylogenetic fossil calibration [7,8]
Kimura et al. [9] Describe that for a more reliable determination of time and fossil descendants may be the use of a methodology based on the study of the first upper molar teeth (M1). In this study the researchers used murine fossil specimens collected from northern Pakistan and examined by digitizing dental images and using tests such as Cochran-Mantel-Haenszel (CMH) and Goodman- Kruskal’s gamma [9]. The questions evaluated in the teeth were the change in frequency distribution of the size and inclination of the region called metacone in relation to paracone and the inclination of the respective axes [9]. However, [10] used a refined set of fossil calibrations that were used to reconstruct a murine family dated phylogeny using a multilocus dataset method (six nuclear and nine mitochondrial gene fragments) spanning 161 species and 82 murine genera from four existing subfamilies (Deomyinae, Gerbillinae, Lophiomyinae and Murinae) [10].
Therefore, regarding muridae family speciation, several types of genetic markers can be applied to peripheral populations:
i. C-band chromosomal patterns [11,12].
ii. Several nuclear autosomal genes studied by protein electrophoresis or by RFLP DNA [13,14].
iii. Use of RFLP rDNA untranslated spacers [15].
iv. Immunoglobulins [16].
v. Satellite DNA [17].
vi. Antigens studied by serology [18].
vii. Some sequences of the Y chromosome [19] and mainly mitochondrial DNA [20].
The oldest known fossil murine, Antemus chinjiensis [9,21], was recorded in the region called Indo-Pakistan in the middle Miocene 14Ma (Millions of years ago), followed by Progonomys hussaini in the upper Miocene, which had a distribution including several regions in Asia, Europe and Africa. The Mus auctor, who represents the first specimen named Mus, also appeared in the subcontinent region of India and is also dated in the Miocene with about 5.5Ma [22]. From the specie M. auctor, the data suggest that there were several successive levels, with temporal proximity, of speciation events. The first level corresponds to the separation of the genus Mus from the other genres. This fact suggests that it occurred about 5 Ma where the genus Rattus, Apodemus, Nannomys (similar to fossils found in Africa 3Ma), Coelomys, Pyromys and Mus subgeneras such as M. cervicolor, M. cookii and M. caroli. The second level, not yet scientifically confirmed, suggests the division between the Indian branch of the genus Mus. This level of speciation refers to the separation between the Indian pygmy mice species as M. dunni and M. booduga (the so-called Leggada).
The third level corresponds to radiation from Asian species, which probably also occurred in India at approximately 2Ma. The fourth radiation step is the separation around 1Ma of western paleartic species, which migrated west to east Asia (Mediterranean), North Africa and Southern Europe. Speciation of M. spicilegus and M. macedonicus clearly occurred very recently, but their ancestor diverged almost simultaneously from M. spretus and M. musculus subspecies. Finally, the fifth radiation level, corresponding to the individualization, around 0.5Ma, of the main M. musculus subspecies, which probably originated from the Indian subcontinent, M. m. bactrianus, M. m. castaneus, M. m. musculus and M.m. domesticus [23].
Geographic Distribution of Mus Musculus
Through fossil studies (by various approaches), the most straightforward ancestor of the Mus genus is the Mus auctor, from the Progonomys class of origin in central Eurasia, mainly northeast India and Pakistan. Despite all the molecular, genetic and paleontological tools, it is very difficult to pinpoint the emergence and differentiation sites of existing species and subspecies. It is possible that the onset of subspecies division occurred during the expansion of India, however it is correct to state that radiation or geographic expansion of the M. musculus species occurred mainly through commensal behavior with humans [23]. According to biogeographic, genetic and archeozoological results, the M. musculus species has expanded from central Eurasia through two pathways, mainly according to human historical patterns. In the Middle East during the “Bronze Age” were found fossils of the genus Mus, but there are also records at the same time of fossils in other places such as central and northern Europe of this genus. This fact suggests that at least two routes were used by these animals to expand between Asia and Europe. Later, in the “Iron Age” we can infer that the species M. musculus colonized northwestern Europe [23,24].
Through the study of mitochondrial DNA four subspecies of M. musculus were defined
i. M. m. domesticus
ii. M. m. musculus
iii. M. m. castaneus
iv. M. m. bactrianus [24,25]
Mus musculus domesticus can be found in Western Europe, North Africa and the northern Mediterranean. It has the commensal habit but is capable of establishing wild populations. Mus musculus musculus presents its habitat between eastern Europe and northern Asia, is able to establish a permanent wild population where winter is mild and can be found as in Central Europe, where it forms with M. m. musculus a hybridization zone 30 to 40km wide. Mus musculus castaneus is a subspecies of Southeast Asia but can be found in the eastern part of the Indian subcontinent throughout Southeast Asia. It is known for its interrelationship withM. m. musculus in China/Japan and has formed a hybrid population called M. m. musculus. Finally, Mus musculus bactrianus can be found in southern Himalayas, in the mountain basins of Iran and northern India [25,26].
Habitat and Natural Behavior
One of the main characteristics related to the habitat and natural behavior of the mouse is its commensal relationship with humans. But commensalism must be accepted as the only one theory [23]. In its origin, the genus Mus was related to arid climatic conditions (Eurasia - Indian Subcontinent), with low humidity and variable temperatures. However, during its evolutionary biology and speciation in Mus subgenus, we can observe a migration of Mus musculus subspecies to various regions, with different geographical and climatic conditions. Probably, this colonization factor of habitats with different characteristics can be considered as selective pressure factors for their speciation [27]. Potter in 1978 didactically suggested the division of the subgenus Mus as wild and the subspecies of M. musculus as commensal by their habitat type and the natural behavior of the animals, as described in Table 1 [28].
Table 1: Habitat division between subgenus and subspecies.
However as described by Sage in 1981, the static classification between subgenus/subspecies wild and commensals should be considered with prudence. Migration of the subgenus Mus (often associated with passive transport with humans) and colonization on several continents may have been an important component in the process of speciation of M. musculus subspecies and demonstrating a flexible relationship between species that maintain their wild or dinner habit. This flexibility would be primarily related to food supply, population density, territorialism and reproducibility. A wild species can become commensal, such as several species that have been domesticated for laboratory use while for example M. m.musculus, characterized as commensal, can become wild, competing with other species for territory and food [28]. This competition feature illustrates one of the key behaviors of the mouse in nature that is aggressiveness, especially from male M. m. domesticus. This aggressiveness is so imposing that this subspecies is able to dominate males M. m. musculus [29].
Aggressive behavior is a hereditary trait, with complex genetic, epigenetic and environmental determinism. In addition, aggressive behavior is likely to be subject to natural and sexual selection [30]. Variation in the intensity of aggressiveness between subspecies may be the result of environmental conditions (territory, food and reproduction). This fact highlights another striking feature in the natural behavior of mice that is adaptability and, more specifically, social flexibility and has been suggested as a possible explanation for their evolutionary success. It is suggested that the emergence of commensal behavior with humans occurred in the search for a safe environment and the supply of food, which is constantly being supplied, thus reducing competition between subspecies, predators and climate pressures [31]. Food disponibility promotes high population density, there is an organization of access to food (developing omnivorous habits with varied diets, including grains, seeds and insects) through a hierarchical population structure [27].
Territorialism remains, both in the wild and during the commensal habit, an important aspect for social organization. The hierarchy is mainly composed of a dominant and breeding male who exhales varying amounts of pheromones such as brevicomin (2,3-dehydro-exo-brevicomin) and thiazoline (2-sec-butyl-4,5- dihydrothiazole) [32], few subordinate males and the remainder females. The group does not exceed 12 individuals. Behavioral observations have suggested that new individuals are not always readily integrated into the group, possibly due to genetic signaling expressed by pheromones, possibly due to caution in maintaining social cohesion. However, the integration of new individuals into the group can produce high genetic diversity, essentially due to extinctions in some situations of the dominant male reproductive. The acceptance of new group members, both male and female, has a successful trait when it induces genetic variability in the group. These animals, despite commensalism, have a very short life, rarely exceeding 7 months. Therefore, this rotation of individuals in the group can be considered beneficial for the maintenance of individuals and the genetic and evolutionary structure of mouse populations [23].
Territorialism remains, both in the wild and during the commensal habit, an important aspect for social organization. The hierarchy is mainly composed of a dominant and breeding male who exhales varying amounts of pheromones such as brevicomin (2,3-dehydro-exo-brevicomin) and thiazoline (2-sec-butyl-4,5- dihydrothiazole) [32], few subordinate males and the remainder females. The group does not exceed 12 individuals. Behavioral observations have suggested that new individuals are not always readily integrated into the group, possibly due to genetic signaling expressed by pheromones, possibly due to caution in maintaining social cohesion. However, the integration of new individuals into the group can produce high genetic diversity, essentially due to extinctions in some situations of the dominant male reproductive. The acceptance of new group members, both male and female, has a successful trait when it induces genetic variability in the group. These animals, despite commensalism, have a very short life, rarely exceeding 7 months. Therefore, this rotation of individuals in the group can be considered beneficial for the maintenance of individuals and the genetic and evolutionary structure of mouse populations [23].
Interestingly, female mice prefer males who have higher levels of these pheromones [34]. Soini et al. [35], demonstrated that the absence of thiazoline in M. spicilegus, which is a non-commensal, wild species described as monogamous, presented aggression levels similar to M. m. domesticus. Therefore, low thiazoline levels may not explain the lower aggressiveness of M.m. musculus compared to M. m. domesticus [35]. However, low levels of brevicomin may be part of the explanation. In addition, M. m. musculus and M. spicilegus share much of their distribution in Southeast Asia and may have experienced similar selective pressures, resulting in part in convergent changes in their pheromone patterns, although similarities may also have evolved by chance [35]. In addition, the M. m. musculus have higher selectivity of mate selection when compared with M. m. domesticus. This low selectivity corroborates its promiscuous polygamous mating pattern. The high selectivity in M. m. musculus does not indicate that this subspecies is monogamous like M. spicilegus. Intermediate systems are believed to exist between these two extreme types of mating system - promiscuity and monogamy - probably related to the genetics of each species or subspecies and the factors already described such as: habitat, nutritional availability and population density [36].
The relationship between the welfare of mice in the house facilities, their genealogy and natural behavior
The question that motivated this work was: “What would be the natural behavior of the mouse, especially the subspecies M. m. musculus in its habitat, considering from its origin? “ The relevance of this question is primarily based on the national legislation and regulations where one can find the suggestion that the welfare of the mice is related to the possibility of providing during their maintenance in animal facilities for scientific and didactic use to express their natural behavior [1]. By conducting a thorough and detailed bibliographical survey on the genealogy, phylogeny and the few data on the natural or wild behavior of mice, we were able to delineate an interesting and complex set of remarkable characteristics of this animal, which can undoubtedly directly influence the welfare of mice under restricted space in animal houses. Several points drew our attention about this animal, such as its origin having occurred thousands of years ago, having come, where it can be studied from an ancestor that branched into several species, such as the rat, gerbil and others [9]. While the mouse in its pedigree continued to improve its characteristics, under intense forces of natural selection and with remarkable evolutionary success, it can be found in the most varied regions of the world, from desert to arctic environments, varying their subspecies, but maintaining its primordial genetic characteristics [23].
In our study we can point out some points that will be important to reflect on your welfare in animal houses
i. We can consider these animals prepared physiologically for stress situations, especially the fight and flight system. It is important to maintain a routine and conditioning of management and procedures not to make stress harmful to the animal.
ii. They have a tremendous ability to adapt to harsh environments and conditions as long as there is time for them to adapt and maintain their routine
iii. Commensal behavior with humans is extremely beneficial for the mouse. Initially for the supply of food, but we can also observe that although stealthy, the proximity to the human increases their social flexibility
So, what we can say is that the relationship with humans was a path that these animals found given the ease of access to food and their ability to hide and escape risk situations. In addition, they are naturally non-isogenic animals with a natural control of variability and genetic perpetuation carried out through groups and the integration of new members to the group, mainly males and with high turnover, due to the low life expectancy of the dominant group. We may reflect that the welfare of this animal is much more tied to its own choices or preferences than when we try to relate our welfare state to that of this animal. Furthermore, we cannot compare our stress with the stress of this animal. It is an animal that already has a system, we can say, in stress. At this point, we believe that comes your ability to adapt. The suffering and discomfort of this animal start from the space restriction and its impossibility to move or seek a place of its choice (we believe more stable and safer). Therefore, we must look for ways to raise the welfare of this space-constrained animal so that its adaptation is close as possible to its preference and it is our obligation to maintain a routine and management always close to what the animal is adapted to avoiding the sudden changes as Ambiental variation in the house facilities.
We have learned from the genealogy of these animals several interesting features, such as their great strength and adaptability to selective pressures. His commensal relationship as a human being that completely changes his behavior, however, comes from his need for access to food, but we can say that his relationship with the human being does not cause suffering or stress to the mouse. So, to promote the welfare of mice in animal houses facilities, it is necessary to find out what is the animal’s preference in all aspects, whether in cage size, group composition, floor type, room temperature, food offered, etc. These animals into stable conditions demonstrated adaptability and flexibility in the life routine. The natural behavior it´s aggressive behavior. Groups formed after weaning form a fraternal relationship, decreasing the incidence of aggression in males in adulthood. Them can be consider that stress and discomfort will only occur disruption of its routine or its adaptability to the environment, even if in space restriction and if possible, no doubt offer elements that can encourage the animal to express your natural behavior such as foraging, sheltering or nesting through environmental enrichment.
Read More About this Article: https://biomedgrid.com/fulltext/volume5/origin-phylogeny-and-natural-behavior-of-mice-what-is-their-influence-on-welfare.000946.php
For more about: Journals on Biomedical Science :Biomed Grid | Current Issue
#biomedgrid#american journal of biomedical science & research#journals on medical research#journals on biomedical science
0 notes
Photo

On the Spot
Biomedical discoveries can come from unexpected places. In a prime example, recent research has uncovered surprising links between the genetics of colouration in geckos and human skin cancer. Popular pets, leopard geckos (Eublepharis macularius) have been bred to produce many different colour varieties. For those of the Lemon Frost form (pictured), their striking colour pattern comes at a cost: more than 80% of them develop skin tumours, known as iridophoroma. By tracking the Lemon Frost pattern in a breeding colony of geckos, and sequencing their DNA, scientists were able to identify the region of DNA controlling this unusual appearance. Within it lies a single gene, SPINT1, known to function as a tumour suppressor, and mutations of which are involved in several forms of cancer, including skin cutaneous melanoma (SKCM) in humans. Linking a key gene to a visible colour difference could make leopard geckos a useful model for studying SKCM in the future.
Written by Emmanuelle Briolat
Image from work by Longhua Guo and colleagues
Department of Human Genetics, Department of Biological Chemistry, Howard Hughes Medical Institute, University of California, Los Angeles, CA, USA
Image copyright held by the original authors
Research published in PLOS Genetics, June 2021
You can also follow BPoD on Instagram, Twitter and Facebook
88 notes
·
View notes
Text
Angela Belcher delivers 2023 Dresselhaus Lecture on evolving organisms for new nanomaterials
New Post has been published on https://thedigitalinsider.com/angela-belcher-delivers-2023-dresselhaus-lecture-on-evolving-organisms-for-new-nanomaterials/
Angela Belcher delivers 2023 Dresselhaus Lecture on evolving organisms for new nanomaterials
“How do we get to making nanomaterials that haven’t been evolved before?” asked Angela Belcher at the 2023 Mildred S. Dresselhaus Lecture at MIT on Nov. 20. “We can use elements that biology has already given us.”
The combined in-person and virtual audience of over 300 was treated to a light-up, 3D model of M13 bacteriophage, a virus that only infects bacteria, complete with a pull-out strand of DNA. Belcher used the feather-boa-like model to show how her research group modifies the M13’s genes to add new DNA and peptide sequences to template inorganic materials.
“I love controlling materials at the nanoscale using biology,” said Belcher, the James Mason Crafts Professor of Biological Engineering, materials science professor, and of the Koch Institute of Integrative Cancer Research at MIT. “We all know if you control materials at the nanoscale and you can start to tune them, then you can have all kinds of different applications.” And the opportunities are indeed vast — from building batteries, fuel cells, and solar cells to carbon sequestration and storage, environmental remediation, catalysis, and medical diagnostics and imaging.
Belcher sprinkled her talk with models and props, lined up on a table at the front of the 10-250 lecture hall, to demonstrate a wide variety of concepts and projects made possible by the intersection of biology and nanotechnology.
Play video
2023 Mildred S. Dresselhaus Lecture: Angela Belcher Video: MIT.nano
Energy storage and environment
“How do you go from a DNA sequence to a functioning battery?” posed Belcher. Grabbing a model of a large carbon nanotube, she explained how her group engineered a phage to pick up carbon nanotubes that would wind all the way around the virus and then fill in with different cathode or anode materials to make nanowires for battery electrodes.
How about using the M13 bacteriophage to improve the environment? Belcher referred to a project by former student Geran Zhang PhD ’19 that proved the virus can be modified for this context, too. He used the phage to template high-surface-area, carbon-based materials that can grab small molecules and break them down, Belcher said, opening a realm of possibilities from cleaning up rivers to developing chemical warfare agents to combating smog.
Belcher’s lab worked with the U.S. Army to produce protective clothing and masks made of these carbon-based virus nanofibers. “We went from five liters in our lab to a thousand liters, then 10,000 liters in the army labs where we’re able to make kilograms of the material,” Belcher said, stressing the importance of being able to test and prototype at scale.
Imaging tools and therapeutics in cancer
In the area of biomedical imaging, Belcher explained, a lot less is known in near-infrared imaging — imaging in wavelengths above 1,000 nanometers — than other imaging techniques, yet with near-infrared scientists can see much deeper inside the body. Belcher’s lab built their own systems to image at these wavelengths. The third generation of this system provides real-time, sub-millimeter optical imaging for guided surgery.
Working with Sangeeta Bhatia, the John J. and Dorothy Wilson Professor of Engineering, Belcher used carbon nanotubes to build imaging tools that find tiny tumors during surgery that doctors otherwise would not be able to see. The tool is actually a virus engineered to carry with it a fluorescent, single-walled carbon nanotube as it seeks out the tumors.
Nearing the end of her talk, Belcher presented a goal: to develop an accessible detection and diagnostic technology for ovarian cancer in five to 10 years.
“We think that we can do it,” Belcher said. She described her students’ work developing a way to scan an entire fallopian tube, as opposed to just one small portion, to find pre-cancer lesions, and talked about the team of MIT faculty, doctors, and researchers working collectively toward this goal.
“Part of the secret of life and the meaning of life is helping other people enjoy the passage of time,” said Belcher in her closing remarks. “I think that we can all do that by working to solve some of the biggest issues on the planet, including helping to diagnose and treat ovarian cancer early so people have more time to spend with their family.”
Honoring Mildred S. Dresselhaus
Belcher was the fifth speaker to deliver the Dresselhaus Lecture, an annual event organized by MIT.nano to honor the late MIT physics and electrical engineering Institute Professor Mildred Dresselhaus. The lecture features a speaker from anywhere in the world whose leadership and impact echo Dresselhaus’s life, accomplishments, and values.
“Millie was and is a huge hero of mine,” said Belcher. “Giving a lecture in Millie’s name is just the greatest honor.”
Belcher dedicated the talk to Dresselhaus, whom she described with an array of accolades — a trailblazer, a genius, an amazing mentor, teacher, and inventor. “Just knowing her was such a privilege,” she said.
Belcher also dedicated her talk to her own grandmother and mother, both of whom passed away from cancer, as well as late MIT professors Susan Lindquist and Angelika Amon, who both died of ovarian cancer.
“I’ve been so fortunate to work with just the most talented and dedicated graduate students, undergraduate students, postdocs, and researchers,” concluded Belcher. “It has been a pure joy to be in partnership with all of you to solve these very daunting problems.”
#000#2023#250#3d#3D model#amazing#applications#Bacteria#batteries#battery#Biological engineering#Biology#Building#Cancer#carbon#Carbon sequestration#catalysis#Cells#chemical#clothing#detection#diagnostics#DMSE#DNA#echo#Electrical Engineering&Computer Science (eecs)#electrodes#energy#energy storage#engineering
0 notes
Text
Evaluation of Microneedle Drug Delivery System and Nanoparticles Use in COVID-19 Patients by Ahmed MA

Abstract
Microneedle is a new form of a transdermal delivery system, which increases its potential for the delivery of drugs to the site of action. Nanoparticles can be used as a drug delivery system that provides both enhancement of drug efficacy and a decrease in side effects. In this review we focused on their use for prevention and treatment of coronavirus disease-2019 (COVID-19).
Keywords: Coronavirus, COVID-19; Drug Delivery; Nanoparticles; Microneedle.
Introduction
At the end of 2019, a group of pneumonia patients of unknown cause emerged in Wuhan, China [1]. Subsequently, the World Health Organization (WHO) announced a standard format of Coronavirus Disease-2019 (COVID-19), according to its terminology, for this novel coronavirus pneumonia on February 11, 2020.
Nowadays, most methods used for the transdermal drug delivery system are topical creams, transdermal patches, hypodermic needles. The effect of most of the drugs and agents which used in this method are of low value due to the presence of stratum corneum layer in the skin which acts as barrier for molecules making few of them able to reach its site of action [2]. Therefore, the transdermal drug delivery system has been developed to appear another method which called microneedles.
Microneedle is a smart approach and a new form of a transdermal delivery system, which increases its potential for the delivery of drugs to the site of action. It is a needle that is micro-sized with a height of 10-2000 μm, and its width is 10-50 μm, which can penetrate to dermal tissue directly without pain. Microneedle can deliver molecules of different sizes and forms. It is considered as a device of drug and vaccine delivery. It can be capsulated with live or inactivated virus vaccine, DNA vaccine, or antigen. Hollow microneedle is used widely in influenza vaccinations. Microneedle has many advantages as its administration is feasible and painless and it increases penetration of the skin and delivers different size of drug and vaccine [3].
Nowadays, many studies have registered to study the effect of using microneedle in vaccinations of COVID-19 as the skin is an excellent site for immunization, as it is rich in antigen and accessory cells. Microneedle developed to physically breach the stratum corneum then rapidly dissolve in the epidermis and dermis. Therefore, the use of microneedle in delivery of vaccinations results in high-concentration delivery of the vaccine. The use of microneedle lead to a decrease in the required dose, decrease the toxicity and increase the efficacy of vaccines.
Kim and his colleagues reported the development of a vaccine against SARS-CoV-2 that seems to be effective in animal models [4]. They used a micro-needle system for vaccine administration; each one made of glucose and fragments of viral spikes proteins that penetrate through the skin and dissolve in the epidermis [4].
Nanoparticles (NPs) are the materials that have nano-scale in all dimensions. NPs have different and more attractive proprieties than bulker substances. By focusing on their achievements in medicine, we find numerous applications that include: (I) Body's imaging techniques before diagnosis, e.g., CT, MRI, SPECT, PET. (II) Laboratory biomedical analysis in-vitro identification of biological molecules and acting as biomarkers. (III) The revolution of utilizing NPs for drug delivery [5].
Advantages of Nanoparticles for Drug Delivery
NPs, as a drug delivery system, provide both enhancement of drug efficacy and a decrease in side effects. Making drugs in the nano-size or attaching it with nanocarriers can alter the physiochemical pharmacokinetic and pharmacodynamic properties of the drug. NPs introduction improves the absorption, distribution, and penetration features of the drug. This allows the availability of different routes of administration (parenteral, oral, and inhalation) [6]. Site-targeting is a promising advantage either passively by the increased permeation or activated by attaching recognition elements and selective sensors to receptors in the targeted tissues. NPs have a high capacity for drug loading, which means a higher concentration of drug can be delivered to maintain the effective therapeutic dose of the drug. Controlled and sustained release by using the multilayer technique is available [7].
Classification of NPs
NPs may be formulated in numerous forms [5-7]:
Liposomes: NPs which have both aqueous and lipid compartments so can entrap both hydrophilic and lipophilic agents as gene, protein, or vaccine.
Dendrimers (the hyperbranched NPs) are used mainly in long-circulating and targeted therapies.
Polymeric micelles are most important in improving the solubility of hydrophilic drugs and decrease their degradation and clearance from the body.
Polymeric NPs contain both colloidal vesicular and matrix systems.
Solid lipid nanoparticles (SLNs) are limited to drugs dissolved in solid lipid but have a high role in increasing the stability of easily degraded particles and targeting.
Nanostructure lipid carriers (NLCs) overcome the limitation of SLNs to be wider applicable.
Unfortunately, some disadvantages are discovered upon applying nano strategies. The balancing between the benefit and the related danger of NPs can determine the scope of utilization. It is a challenge for responsible seeking to diminish these unwanted side effects. On the other side, Nanotechnology has been achieved noticeable success in the medical application.
Antiviral therapies, prophylaxis, or even immunostimulant adjuvants can be supported by the incorporation of nanostructures depending on their preferable properties. Hydrogels NPs have the ability to entrap the viral′s vital components as the Influenza virus and are expected to be modified to suit the other viruses [8].
The world, nowadays, is suffering from the pandemic coronavirus disease-2019 (COVID-19). Great efforts have been oriented by scientists and researchers as attempts to overcome this crisis. Fighting COVID-19 demands high peoples′ awareness. It begins with adequate protection and highly sensitive and quick diagnosis, which are of the same importance as the treatment of the already infected persons.
Nanotechnology can help in different stages.
Forming highly protective masks with nano-attached filters and nano-dependant disinfectants is of far benefit.
The selectivity, bioavailability and penetration′s ability of antiviral, vaccines and immune-stimulant drugs can be synergized by attaching with NPs
Depending on the reports and the information published by the WHO about COVID-19, no official final protocol of treatment or even vaccination are available yet [9].
One of the aimed approaches is to decrease the severity of the life-threatening symptoms associated with COVID-19. Damage of many organs as lungs is motivated by the excessive release of inflammatory and immune cells as a response of the body against this viral infection. So, introducing a suitable anti-inflammatory agent is recommended. Methotrexate (MTX) is a suggested drug used mainly as an antineoplastic and antirheumatic drug [10]. Additionally, MTX affects the inflammatory mediators (e.g., interleukins, prostaglandins, cytokines, and others) either directly by alteration of their synthesis and degradation or indirectly by alteration of their response [11]. Currently, a clinical trial was registered and running in Brazil to evaluate the efficacy and safety of MTX-loaded nanoparticles to treat severe COVID-19 patients with three different doses [12].
Another study was registered in Australia to evaluate the immunogenicity and safety of the SARS-CoV-2 rS nanoparticle vaccine in healthy participants [13]. Further trials will be planned sooner to utilize the advantages of nanoparticles for prophylaxis against the infection and treatment of COVID-19 patients.
In conclusion, using the microneedle drug delivery system and nanoparticles for the development of COVID-19 vaccines or enhanced delivery of some medications seems to be effective and successful nearly.
For more information about Journal : https://ijclinmedcasereports.com/
https://ijclinmedcasereports.com/pdf/IJCMCR.SC.ID.00037.pdf https://ijclinmedcasereports.com/ijcmcr-sc-id-00037/
0 notes
Text
Consumer Genomics Market Expectations & Growth Trends Highlighted until 2026
Market Analysis and Insights: Consumer Genomics Market
Consumer Genomics Market By Application (Genetic Relatedness, Diagnostics, Lifestyle, Wellness, & Nutrition, Ancestry, Reproductive Health, Personalized Medicine & Pharmacogenetic Testing, Sports Nutrition & Health, Others), Technology (PCR, Sequencing, Microarray, Nucleic Acid Extraction and Purification, Other Technologies), Product and Service (Consumables, Systems & Software, Services), Geography (North America, Europe, Asia-Pacific, South America, Middle East and Africa) – Industry Trends & Forecast to 2026
Global consumer genomics market is set to witness a healthy CAGR of 20.45% in the forecast period of 2019-2026. The report contains data of the base year 2018 and historic year 2017. Increasing trend of DTC testing and growing application areas of genomics are the factor for the growth of this market.
Request Sample is Available @ https://www.databridgemarketresearch.com/request-a-sample/?dbmr=global-consumer-genomics-market
Competitive Analysis:
Global consumer genomics market is highly fragmented and the major players have used various strategies such as new product launches, expansions, agreements, joint ventures, partnerships, acquisitions, and others to increase their footprints in this market. The report includes market shares of consumer genomics market for global, Europe, North America, Asia-Pacific, South America and Middle East & Africa.
Market Definition: Global Consumer Genomics Market
Consumer genomics is related with the sequencing, interpretation and sequencing of the genome. They are widely used in application such as diagnostics, wellness and nutrition, reproductive healthcare, genetic relatedness, ancestry and others. If genotypes are known, this helps the individual to know about their ancestry inference, disease risks and other trait expression. Increasing prevalence for personalized genomics is the factor fueling the growth of this market.
Market Drivers
Rising demand for personalized genomics is driving the market
Increasing access to DTC service due to decrease rate of genotyping will also drive market
Rising investments by companies in consumer genomics will also propel the market growth
Growing consumer and physicians interest in DTC kits & consequent is also contributing as a factor for the growth of this market
Market Restraints
Increasing clinical and ethical problems related to the use of DTC products & personalized treatment will restrain market growth
Strict norms associated with the use of DTC genetic tests will also hamper the market growth
Lack of adoption of home-based genetic tests due to misleading results will also restrict the growth of the market
Segmentation: Global Consumer Genomics Market
By Application
Genetic Relatedness
Diagnostics
Lifestyle
Wellness, & Nutrition
Ancestry
Reproductive Health
Personalized Medicine & Pharmacogenetic Testing
Sports Nutrition & Health
Others
By Technology
PCR
Sequencing
Microarray
Nucleic Acid Extraction and Purification
Other Technologies
By Product and Service
Consumables
Systems & Software
Services
For Further Detailed insights and ‘Any Query About this report @ https://www.databridgemarketresearch.com/inquire-before-buying/?dbmr=global-consumer-genomics-market
By Geography
U.S.
Canada
Mexico
Germany
Italy
U.K.
France
Spain
Netherlands
Belgium
Switzerland
Turkey
Russia
Rest of Europe
Japan
China
India
South Korea
Australia
Singapore
Malaysia
Thailand
Indonesia
Philippines
Rest of Asia-Pacific
Brazil
Rest of South America
South Africa
Rest of Middle East and Africa
Key Developments in the Market:
In April 2019, PerkinElmer along with Helix announced the launch of their new consumer gene testing product GenePrism. This product has the ability to analyze 59 genes and is very suitable for individuals who don’t have any information about their family history of disease. Helix will be sequencing the user’s DNA and further passing the result to PerkinElmer medical geneticists so that they can provide data analysis and interpretative service. With this product launch the company has expanded its product portfolio in the market.
In November 2018, Nebula Genomics announced the launch of consumer genetics: the ability for individuals to have their full genome sequenced. This new platform will connect the consumer with the researchers so that they can help them in advance biomedical research. This is specially designed so the consumer can share their genomic data in a fair and transparent ecosystem and assisting the scientists in performing the transformative research.
Request for complete Toc @ https://www.databridgemarketresearch.com/toc/?dbmr=global-consumer-genomics-market
Key Market Competitors:
Few of the major competitors currently working in the global consumer genomics market are 23andMe, Inc., Gene by Gene, Mapmygenome - Know Yourself, Color Genomics, Inc., Helix OpCo LLC, MyHeritage Ltd, Pathway Genomics, Veritas Genetics, Myriad Genetics, Inc., Illumina, Inc., Xcode Life, Diagnomics, Inc., Toolbox Genomics, Thermo Fisher Scientific Inc., QIAGEN, Eurofins Scientific, Genomic Health, Invitae Corporation, Nebula Genomics, PerkinElmer Inc., Oxford Nanopore Technologies and others
Research Methodology: Global Consumer Genomics Market
Data collection and base year analysis is done using data collection modules with large sample sizes. The market data is analysed and forecasted using market statistical and coherent models. Also market share analysis and key trend analysis are the major success factors in the market report. To know more please request an analyst call or can drop down your enquiry.
The key research methodology used by DBMR research team is data triangulation which involves data mining, analysis of the impact of data variables on the market, and primary (industry expert) validation. Apart from this, other data models include Vendor Positioning Grid, Market Time Line Analysis, Market Overview and Guide, Company Positioning Grid, Company Market Share Analysis, Standards of Measurement, Top to Bottom Analysis and Vendor Share Analysis. To know more about the research methodology, drop in an inquiry to speak to our industry experts.
Primary Respondents
Demand Side: Doctors, Surgeons, Medical Consultants, Nurses, Hospital Buyers, Group Purchasing Organizations, Associations, Insurers, Medical Payers, Healthcare Authorities, Universities, Technological Writers, Scientists, Promoters, and Investors among others.
Supply Side: Product Managers, Marketing Managers, C-Level Executives, Distributors, Market Intelligence, and Regulatory Affairs Managers among others.
Reasons to Purchase this Report
Current and future of global consumer genomics market outlook in the developed and emerging markets
The segment that is expected to dominate the market as well as the segment which holds highest CAGR in the forecast period
Regions/Countries that are expected to witness the fastest growth rates during the forecast period
The latest developments, market shares, and strategies that are employed by the major market players
Read other Report
Asia-Pacific Flexible Digital Video Cystoscopes Market
Asia-Pacific Intraoperative Imaging Market
Asia-Pacific Skin Tightening Market
Dental Diagnostic & Surgical Equipment Market
Europe Cardiac Pacemakers Market
About Data Bridge Market Research:
Data Bridge Market Research set forth itself as an unconventional and neoteric Market research and consulting firm with unparalleled level of resilience and integrated approaches. We are determined to unearth the best market opportunities and foster efficient information for your business to thrive in the market
Contact: Data Bridge Market Research
Tel: +1-888-387-2818
Email: [email protected]
0 notes
Text
NIBMG PhD Program Admission 2021 – Official Notification Released
New Post has been published on https://biotechtimes.org/2020/12/11/nibmg-phd-program-admission-2021-official-notification-released/
NIBMG PhD Program Admission 2021 – Official Notification Released

NIBMG PhD Program Admission 2021
NIBMG PhD Program Admissions 2021 – Official Notification Released. National Institute of Biomedical Genomics has released NIBMG PhD Admission 2021 Official Notification. Candidates interested in NIBMG PhD Program can check out the below-mentioned information.
NATIONAL INSTITUTE OF BIOMEDICAL GENOMICS (An Autonomous Institution of the Government of India) P.O.: N.S.S., Kalyani 741251, West Bengal, India
NO: NIBMG/ADMIN/ESTB/2020-21/ 299
Applications are invited from suitable candidates to pursue doctoral research at NIBMG. The selected students are expected to work on human diseases with emphasis on gaining biological insights into disease mechanisms using clinical samples and appropriate models, both in silico and in vivo.
NIBMG is equipped with state-of-the-art technologies which include single-cell sequencing and bulk DNA sequencing NGS platforms, extensive computational and bioinformatics facility, genetic engineering including cutting-edge CRISPR-Cas Genome editing laboratories, FACS (cell sorter), low and high-resolution imaging facility, Multiplex Elisa/ALPHA-LISA, state-of-the-art zebrafish, proteomics upcoming microbiome facilities.
The broad areas of research on which students are expected to work are listed below:
1) Biomedical Genomics and the functional implications in the context of:
Infectious disease
Chronic Disease
Cancer
2) Computational Biology and Statistical Genomics
15% of the seats are reserved for the SC category, 7.5% for the ST category, and 27% for the non-creamy layer OBC category. Certificates from relevant authorities are to be provided as proof before the interview.
The minimum eligibility criteria for accepting students at NIBMG to pursue research leading to a Ph.D. degree are:
Category A
• Master’s degree in any discipline of Biological Sciences (Genetics, Molecular Biology, Biochemistry, Biotechnology, Statistics, Bioinformatics, Computer Science, Zoology, Botany, Physiology, Biophysics or related disciplines).
• Candidates in the general category must have secured at least 55% aggregate in the Master’s degree. This will be relaxed for reserved categories as they are only required to successfully complete the Master’s degree.
• An applicant must have qualified in the junior research fellowship conducted by UGC, CSIR, ICMR, DBT (category I only), NBHM, BINC, and must have a valid award letter of Research Fellowship at the time of interview. DST-INSPIRE students with a provisional certificate from DST are also eligible to apply. Candidates who have qualified for Rajiv Gandhi National Fellowship or any other fellowship for the reserved categories are encouraged to apply.
Category B:
• Should have an MBBS or B.Tech (Biotechnology) with at least 55% marks along with a valid junior research fellowship from UGC, CSIR, ICMR, DBT (category I only).
• Preference will be given to candidates with demonstrable research training in the form of summer training or short-term courses in established research laboratories in preparation for a research career in biomedical sciences. This condition is not applicable to individuals belonging to reserved categories.
How to Apply
Please apply online via web link https://apply.nibmg.ac.in/ (no other form of application will be accepted). Please indicate online whether you belong to a reserved category or the general category. The online application will be accepted until 5 PM on 23rd December 2020. Short-listed candidates will be called for a formal interview to make the final selection. The interview will be in January 2020.
Download Notification
Apply Now
0 notes
Text
Women in Biomedicine
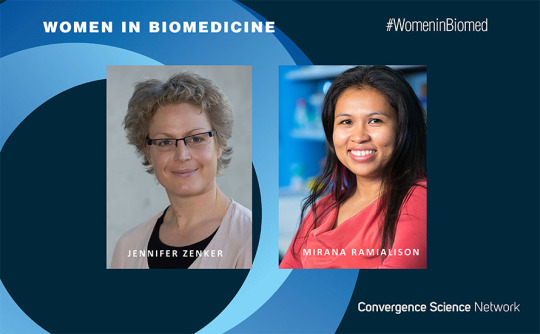
New Seminar Series Launch Wednesday 18 November 2020
REGISTER
The talents of women in science, technology, engineering, and mathematics (STEM) are vital to the future of Australia’s health and economy. Yet it is no secret that women are poorly represented in the STEM workforce and earn less than their male counterparts. Women makeup approximately half of junior academics in STEM, but only around one-fifth of senior professors.
The Convergence Science Network is launching a new event series, Women in Biomedicine, to showcase and celebrate the success of women in biomedicine who are shaping the future of healthcare. We are delighted to launch the series with Associate Professor Mirana Ramialison and Dr Jennifer Zenker of the Australian Regenerative Medicine Institute at Monash University. Our women researchers will share their research in a pre-recorded video, to be followed by a live webinar where they will discuss their experiences, the challenges confronting women scientists and provide an insight into the world of biomedicine to inspire the next generation of scientists.
The webinar with Mirana and Jennifer will be held on Wednesday, 18 November at 12.30 pm AEDT. View Mirana’s and Jennifer’s video below to learn about their research.
More information about them and where to register to attend the webinar is available here.
The webinar will be moderated by Catriona Nguyen-Roberston, a Science Communication Officer at the Convergence Science Network.
We invite you to join this event as we acknowledge the role played by women in progressing biomedical science and to be inspired by the journey our women have followed and challenges they have overcome as they share their experience for the next generation of women scientists. You will be able to put your questions to Mirana and Jennifer in writing or by “raising your hand” to be seen and heard.
We can’t wait to launch this important series and we look forward to welcoming you on the 18th November.
OUR SPEAKERS
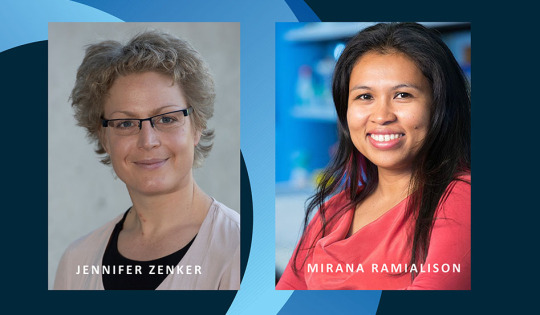
Dr Jennifer Zenker, Australian Regenerative Medicine Institute
A look behind the scenes of a cell transforming into a new life
The astonishing transformation of the embryo from a soccer ball-like structure into a newborn with four limbs, a face and a bumping heart is one of the most incredible but also most enigmatic processes of our lives. Pluripotent stem cells are the only cells which can transform themselves into any kind of specialised cell. These incredible smart cells are the ancestors of every human being, animal and plant. The Zenker Lab aims to unravel the secrets on the inner structural aspects of pluripotency using cutting-edge imaging technologies of the living mouse embryo and to repurpose those same principles for the application of induced pluripotent stem cells in regenerative medicine.
How to build a smart cell? Similar to cities, transport can make a critical difference. Inside a cell, organelles and proteins are usually not randomly distributed but assigned to regions where they are needed. The cell utilises a network of filament-like structures, the microtubule cytoskeleton, as a road map to localise organelles. Based on our discoveries of an unprecedented organisation of the microtubule cytoskeleton in the early mouse embryo, the Zenker Lab is investigating how the layout of the embryonic roadmap will ultimately determine the movement, positioning and interactions of the organelles in pluripotent stem cells.
Bio:
Dr. Jennifer Zenker is the head of the Zenker lab at the Australian Regenerative Medicine Institute (ARMI, Monash University). She is an emerging leader in the field of cellular architecture of pluripotent stem cells who is applying cutting-edge imaging approaches on the living mouse embryo. Her capacity to conduct high quality, innovative research is evident in her impactful publication record.
Dr. Zenker obtained her PhD (2012) in Neurobiology, identifying novel cellular mechanisms underlying diabetic peripheral neuropathy including ion channel mis-localisation and mitochondrial defects, using various mouse models (first author papers in Journal of Neuroscience (2012), Glia (2014) and a review in Trends in Neurosciences (2013)). During her Postdoctoral studies, Dr. Zenker specialised on live imaging of the early mouse embryo which led to a number of seminal discoveries, including first author papers in Science (2017), Cell (2018) and Nature Protocols (2017), plus two reviews in Develpomental Cell (2018) and Current Opinion in Cell Biology (2017). She was awarded three international postdoctoral fellowships, from the prestigious Human Frontier Science Program (HFSP), the German and Swiss National Science Foundation (DFG and SNF).
In November 2018, Dr. Zenker embarked on a career as an independent group leader at ARMI. In 2019, she was awarded the highly competitive Canadian Institute for Advanced Research (CIFAR) Azrieli Scholarship as a real mark of her distinction and scientific excellence. Her interdisciplinary approach to study the living mouse embryo has significantly advanced our knowledge how the microtubule cytoskeleton is required for pluripotency and cell fate allocation. With her extensive expertise in innovative live imaging, molecular manipulations, drug treatments and advanced quantitative single-cell imaging, she is in a unique position to unravel the fundamental cell biological principles of pluripotent stem cells.
Associate Professor Mirana Ramialison, Australian Regenerative Medicine Institute
The secret life of junk DNA
The publication of the first draft sequence of the human genome revealed several surprises including the discovery that genes compose only 2% of our genome. To date, the remainder 98% of the human genome, that does not contain genes and often referred to as “junk DNA”, has revealed to be of extreme importance in regulating the expression of genes. Here I discuss the scientific journey to understand more about our “junk DNA”.
Bio:
Associate Professor Ramialison received her Engineering degree from the University of Luminy, France, after which she worked as a programmer at the ERATO differentiation project in Kyoto. After obtaining her PhD in Developmental Genomics from the European Molecular Biology Laboratory in Heidelberg, Germany, she joined the Victor Chang Cardiac Research Institute in Sydney as an EMBO and HFSP Post-Doctoral Fellow. With an NHMRC/Heart Foundation Career Development Fellow, she relocated to Melbourne here she currently leads the Systems Developmental Biology Laboratory at the Australian Regenerative Medicine Institute and the Transcriptomics and Bioinformatics Laboratory at the Murdoch Children’s Research Institute.
REGISTER
youtube
youtube
0 notes
Text
Waiting for a ‘smart’ toilet? It’s nearly here
Think back to the last time you pooped. Was it hard or soft? Was it dark or light brown? Was there corn in it? You might find these questions uncomfortably personal. Yet the wastes our bodies shed can say a lot about our health. And it can be hard to remember past poops and pees well enough to describe to doctors once we realize that we’re sick. There’s also a lot of stuff in those wastes not visible to the eye. But new technology can help. You can just think of these systems as smart toilets.
Scientists Say: Data
They gather health data by analyzing poop and pee so you don’t have to. Scientists hope that one day such toilets will be able to diagnose cancer and viruses. In fact, scientists are already using human feces (although not via toilets) to track the COVID-19 virus.
But that’s for the future. The smart toilet being developed at Stanford University in Palo Alto, Calif., can’t do nearly that much. The prototype can take pictures of your feces, track how often you go and measure how long each poop takes. Artificial intelligence then evaluates the consistency of the poop using a so-called Bristol scale. (Its seven categories range from “rabbit droppings” through sausage-shaped to “gravy.”) The toilet also can measure the number of white blood cells and types of protein in urine. Such information could indicate an infection of the urinary tract or bladder.
Seung-min Park is a biomedical engineer working on the project at Stanford. Data on stool consistency can help patients with irritable bowel syndrome (IBS), he says. IBS patients need to monitor what their poop looks like over time. This toilet can do that for them.
The data gleaned will move through the internet to a secure computer. An artificial-intelligence program then will assess the data. If it finds a problem, the program can notify a patient’s doctor.
Sonia Grego is an electrial and computer engineer leading another smart-toilet project at Duke University. It’s in Durham, N.C. When it comes to medicine, Grego says, human wastes “are an untapped resource.”
Potty confidential
As a toilet collects health data, those data must be linked to the right person. But how is the toilet to know that you are the one on the seat and not your brother, parents or some visiting guest? Right now, there’s a camera.
Everyone’s anus has unique curves and other patterns. It’s distinct, like a fingerprint. Those patterns can be used to identify who sits on a toilet.

This diagram of the current Stanford prototype shows how the toilet evaluates poop and captures the user’s unique anal print.Adapted from S. Park et al/Nature Biomedical Engineering 2020 and Gambhir lab’s drawing
Park and his team chose to have their toilet use a scanner to image those patterns. This doesn’t require that people who use the toilet do anything special. Just sit down on the seat, as usual. An image of the butt will later be used to identify data obtained from that person’s toilet visit.
Engineers loved this ID system. Potential users, not so much. “They didn’t want to have a camera underneath their butts,” Park says. So on the next model, his team will replace the camera with an infrared or laser scanner. That scan’s output won’t look like a photo.
Another type of “smarts”

A member of the Smart Toilet research team tests its technology using a regular toilet on a research bench.Duke Center for WaSH-AID
At Duke University, Grego’s team is working on an alternative model. It waits to analyze wastes as the toilet bowl’s water is flushed away.
The team working on this project also wants to keep a user’s experience as normal as possible. They haven’t started thinking about an identification system yet, but Grego says this device won’t take pictures of any body parts.
“I think we would identify an individual the same way as other systems would,” she says. She’s referring to technology for facial recognition and fingerprint IDs.
Scanning one’s privates is hardly the only privacy issue with smart toilets. What comes out of your body says a lot about you, or at least about your body. Such data can help doctors track your health. But that data also could be misused by insurance companies or others. And some toilets might one day be able to test your DNA. Those genetic data may have details you don’t want to share with anyone — perhaps not even with yourself.
Explainer: How DNA testing works
Park appreciates such privacy concerns. However, he also thinks the benefits may outweigh the risks. “The more you expose your privacy,” he believes, “the better the health care.”
Grego and her team at Duke are hoping to have a toilet that can be tested by people within the next two years. Park says his team will come out with its next version within the year. Their eventual goal is to be able to isolate RNA or DNA, details that could detect cancers and viruses.
“We want to measure everything that can be extracted from the human body over a lifetime,” he says.
Waiting for a ‘smart’ toilet? It’s nearly here published first on https://triviaqaweb.tumblr.com/
0 notes
Text
Google and ZebiAI launch Chemome Initiative to identify ‘chemical probes’ with AI models
In a study published this week in the Journal of Medicinal Chemistry, researchers at Google, in collaboration with X-Chem Pharmaceuticals, demonstrated an AI approach for identifying biologically active molecules using a combination of physical and virtual screening processes. It led to the creation of the Chemome Initiative, which launches today — a collaboration between Google’s Accelerated Science team and startup ZebiAI that aims to enable the discovery of many more small molecule chemical probes for biological research.
As part of the Chemome Initiative, Google says that ZebiAI will work with researchers to identify proteins of interest and source screening data the Accelerated Science team will use to train AI models. These models will make predictions on commercially available libraries of small molecules — chemical probes that aren’t useful as drugs, but that selectively inhibit or promote the function of specific proteins — that will be provided to researchers for activity testing to advance some programs through discovery.
Making sense of the biological networks that support life and produce disease is a complex task. One approach is using small molecules; in a biological system (e.g., cancer cells growing in a dish), they can be added at a specific time to observe how the system responds when a protein has increased or decreased activity.
Despite how useful chemical probes are for this kind of biomedical research, only 4% of human proteins have a known chemical probe available. In an effort to isolate new ones, Google and X-Chem Pharmaceuticals turned to the field of AI and machine learning.
VB Transform 2020 Online – July 15-17. Join leading AI executives: Register for the free livestream.
As the coauthors of the study explain, chemical probes are identified by scanning the space of small molecules in a target protein to distinguish “hit” molecules that can be further tested. The physical part of the process uses DNA-encoded small molecule libraries (DELs) that contain many distinct small molecules in one pool, each of which is attached to a fragment of DNA serving as a “barcode” for that molecule. One generates many chemical fragments along with a common chemical handle. The results are pooled and split into separate reactions, where a set of distinct fragments with another chemical handle are added.
The chemical fragments from the two steps react and fuse together at the common chemical handles, and they’re connected to build one continuous barcode for each molecule. Once a library has been generated, it can be used to find the small molecules that bind to the protein of interest by mixing the DEL with the protein and washing away the small molecules that don’t attach. Sequencing the remaining DNA barcodes produces millions of individual reads of DNA fragments that can then be processed to estimate which of the billions of molecules in the original DEL interact with the protein.
Above: The fraction of molecules from those tested showing various levels of activity, comparing predictions from the classifier and random forests on three protein targets.
Image Credit: Google
To predict whether an arbitrarily chosen small molecule will bind to a target protein, the researchers built a machine learning model — specifically a graph convolutional neural network, a type of model designed for graph-like inputs like small molecules. The physical screening with the DEL provides positive and negative examples for a classifier, such that the small molecules remaining at the end of the screening process are positive examples and everything else is negative examples.
The team physically screened three diverse proteins using DEL libraries: sEH (a hydrolase), ERα (a nuclear receptor), and c-KIT (a kinase). Using the DEL-trained models, they then virtually screened large make-on-demand libraries from drug discovery platform Mcule and an internal molecule library at X-Chem to identify a set of molecules predicted to show affinity with each protein target. Lastly, they compared the results of their classifier to a random forest model, a common method for virtual screening that uses standard chemical fingerprints. They report that the classifier significantly outperformed the RF model in discovering potent candidates.
The team tested almost 2,000 molecules across the three targets, which it claims is the largest published prospective study of virtual screening to date.
“We’re excited to be a part of the Chemome Initiative enabled by the effective ML techniques described here and look forward to its discovery of many new chemical probes. We expect the Chemome will spur significant new biological discoveries and ultimately accelerate new therapeutic discovery for the world,” Google wrote in a blog post. “While more validation must be done to make the hit molecules useful as chemical probes, especially for specifically targeting the protein of interest and the ability to function correctly in common assays, having potent hits is a big step forward in the process.”
Source link
The post Google and ZebiAI launch Chemome Initiative to identify ‘chemical probes’ with AI models appeared first on The Bleak Report.
from WordPress https://bleakreport.com/google-and-zebiai-launch-chemome-initiative-to-identify-chemical-probes-with-ai-models/
0 notes
Text
Chromatin organizes itself into 3-D 'forests' in single cells
https://sciencespies.com/biology/chromatin-organizes-itself-into-3-d-forests-in-single-cells/
Chromatin organizes itself into 3-D 'forests' in single cells


A 3D forest of chromatin predicted by the researchers’ model. Tree domains are colored from red to green according to their sizes. Each particle represents 2 kilobases of DNA. Credit: Northwestern University
A single cell contains the genetic instructions for an entire organism. This genomic information is managed and processed by the complex machinery of chromatin—a mix of DNA and protein within chromosomes whose function and role in disease are of increasing interest to scientists.
A Northwestern University research team—using mathematical modeling and optical imaging they developed themselves—has discovered how chromatin folds at the single-cell level. The researchers found chromatin is folded into a variety of tree-like domains spaced along a chromatin backbone. These small and large areas are like a mixed forest of trees growing from the forest floor. The overall structure is a 3-D forest at microscale.
Chromatin is responsible for packing DNA into the cell nucleus. In humans, that’s about six feet of DNA in each cell. The new work suggests that chromatin is more structured and hierarchical in single cells than previously thought. Learning how chromatin correctly operates will help scientists understand what goes wrong with it in cancer and other diseases.
“By integrating theoretical and experimental work, we have produced a new chromatin folding picture that helps us see how the 3-D genome is organized at the single-cell level,” said Igal Szleifer, the Christina Enroth-Cugell Professor of Biomedical Engineering at Northwestern’s McCormick School of Engineering. He co-led the research team with Vadim Backman.

Northwestern University researchers have discovered how chromatin folds at the single-cell level. They found that it folds into a variety of tree-like domains spaced along a chromatin backbone. Credit: Northwestern University
Details of the interdisciplinary study will be published Jan. 10 in the journal Science Advances.
“If genes are the hardware, chromatin is the software,” said Backman, the Walter Dill Scott Professor of Biomedical Engineering and director of the Center for Physical Genomics and Engineering. “If the structure of chromatin changes, it can alter the processing of the information stored in the genome, but it does not alter the genes themselves. Understanding chromatin folding holds the key to understanding how cells differentiate and how cancer happens.”
Advances in genomic, imaging and information technologies are just beginning to enable scientists to better understand how chromatin works. The Northwestern researchers used a Partial Wave Spectroscopic (PWS) microscope, optical imaging developed by Backman and colleagues, to peer deep into live cells and “sense” alterations in chromatin packing. They also used electron imaging.
“Our paradigm-shifting picture of chromatin folding is an important missing piece in the holistic view of genomic structure,” said Kai Huang, the study’s first author. Huang is a postdoctoral fellow in Backman’s research group. “The results should inspire new strategies to fight cancer.”
Explore further
Disorderly DNA helps cancer cells evade treatment
More information: “Physical and data structure of 3D genome” Science Advances (2020). advances.sciencemag.org/content/6/1/eaay4055
Provided by Northwestern University
Citation: Chromatin organizes itself into 3-D ‘forests’ in single cells (2020, January 10) retrieved 10 January 2020 from https://phys.org/news/2020-01-chromatin-d-forests-cells.html
This document is subject to copyright. Apart from any fair dealing for the purpose of private study or research, no part may be reproduced without the written permission. The content is provided for information purposes only.
#Biology
0 notes