#Biological and Biomedical Materials
Explore tagged Tumblr posts
Text
Imagine a person on the ground guiding an airborne drone that harnesses its energy from a laser beam, eliminating the need for carrying a bulky onboard battery. That is the vision of a group of University of Colorado at Boulder scientists from the Hayward Research Group. In a new study, the Department of Chemical and Biological Engineering researchers have developed a novel and resilient photomechanical material that can transform light energy into mechanical work without heat or electricity, offering innovative possibilities for energy-efficient, wireless and remotely controlled systems. Its wide-ranging potential spans across diverse industries, including robotics, aerospace and biomedical devices.
Continue Reading.
149 notes
·
View notes
Note
Hal. Do you get bullied by the other more mutated McCoys?👁️👁️
"Bullied? Well, it's not like they're pushing me in the sandbox and stealing my lollipop like we're schoolchildren. They also don't tend to accost me with childish taunts...No, they ignore me."
"It's not like I'm the only McCoy who's not grown blue fur (or any other color fur for that matter)... but I'm definitely in the minority."
"It mainly lies in the fact that I'm not a biologist, a chemist, an MD, a geneticist, a biomedical engineer...or a fucking aerospace engineer. I don't have a wall full of BS degrees. I have dual BAs in Fashion Design Technology: Menswear and Womenswear from London College of Fashion. "
"I haven't saved the world with a cure for deadly viruses, or halted deadly microbots from destroying people from the inside out, or stopped alien race from taking over earth...and when they...we...are a prideful bunch, being a well-known fashion designer does not rank high in terms of achievements in their eyes."
"But they don't know that I dabble in biological and chemical engineering to create many of my designs. I have created new biosynthetic materials... I just choose to hire a small team of scientists to properly test and patent them. Being a scientist would peak the interest of certain parties that I don't want knocking on my door... I left that life long ago."
"They also don't know the experience of watching a nine-year-old girl cry with joy when she finally puts on a set of clothes that her skin won't melt off of her body. Or the man who was unable to touch anyone because of the strong electrical current running through his body suddenly be able to hug his family for the first time in decades without wearing rubber gloves...or fire departments worldwide now relying on thinner, less cumbersome material that has the same/better protections as their old, heavy layers which allows them to save lives more easily? I could go on..."
"The point is, they view me as beneath them because they don't even bother to see past the optical lenses of the microscope jammed against their eyes."
#the mccoyverse#hank mccoy#ask us anything!#beast xmen#Most McCoy's do not take him seriously even though he's no less intelligent#but he also uses their underestimations to his advantage
3 notes
·
View notes
Text
Operation YAN
C/w: Imagination station, woo. Fake humans, mentions of government, unhealthy behavior, mentions of murder, mentions of pedophilia, mentions of homophobia, mentions of other sexualities, mentions the word "love" a lot, mentions sacrilegious things (making fun of religion, kind of? All in good fun, of course), no beta we die like men
A/n: So I was thinking–I know, such a dangerous occupation–but I was thinking, I want to write a universe much like that one genshin doll au (good lord, why is that when I can't find what I'm looking for until I'm not looking for it???) or any hypotheticals. It's probably already done before but I still wanna write it. Masterlist
It started with an idea.
Jesus (pronounced hay-SOOS)–Yes, that was the name of the man who changed the world–Alfaro was listening to a good friend of his going on and on about his ex-girlfriend, who left him for various reasons.
His friend clicked his tongue. “Man, if only I wasn't such a dumbass… But then I wouldn't be such a dumbass if she wasn't such a dumb bi–”
A light bulb lit up in Jesus's head. What if I were to biomedically engineer the perfect woman for my friend? he thought.
It sounds unrealistic how this idea came about, but to be fair, this rendition was passed down for generations.
Jesus had a biomedical degree sitting on the back burner for several years now since he couldn't find any work. AI had already taken over most of the available jobs. For Jesus and his friend, the last time they saw a human worker in a fast food restaurant or a construction worker was probably when they were in middle school. Jesus was only able to pay for the ridiculously expensive tuition from a lottery scholarship, and he had to work twice as hard as any human in history just to graduate since most of his classmates were AI bots (why AI felt the need to subject their own to school life is beyond anyone's understanding).
Anyway, back to the point. He decided to make use of his biomedical degree and scrounge up all kinds of materials to make his idea happen, from flasks to dials to an incubator. This process included gathering a few samples from his friend, such as hair, saliva, blood, urine, and genital fluids.
“Bro, that's gay.”
“Dude, don't you want the perfect woman though?”
His friend clicked his tongue. “Shit. Fine. Just… just give me a several minutes.”
It only took a minute for Jesus to get a semen sample, but that's digressing from the story.
Anyway, it took several years for Jesus to make it happen, but it happened. The perfect woman, based on his friend’s preferences, was born.
Jesus almost didn't give her up to his friend because he felt like he was giving up his daughter to a fiend. He valued his friendship, yes, but he had to admit his friend was such a dumbass when it came to women.
But miraculously, his friend became a changed man after meeting this perfect woman. Overnight, Jesus's friend became a devoted, and loyal charmer who also became the perfect husband to his wife and father to his children.
Why did this work, one may ask?
Well, Jesus had taken into account biological and sexual compatibilities when he was constructing the perfect woman for his best friend. First, he was able to somehow alter his friend's DNA, so their future children wouldn't inherit any dysfunctional genes that would shorten their lifespan or quality of life. This also eliminated the idea of incest, despite this perfect woman being constructed utilizing his friend's DNA, since Jesus had to make many, many, many adjustments to his friend's sperm to change it into a viable egg. It would've been far easier if Jesus could have secured an egg sample from a willing woman, but the idea of his friend copulating with what is essentially his female self was far better than… well, a ��daughter”. Leave it to Jesus to look out for his friend. 👍
Jesus was not initially an ambitious man, but his friend would brag about his love life to anyone who would listen. This led to Jesus gaining attention, both good and bad attention. There was a point where Jesus had to give birth to several perfect women for a notorious gang who threatened to kill his loved ones.
It was easier this time to grow a woman in a lab, since he already had the knowledge. However, the same thing that happened to his friend happened again to these gang members. These vicious beasts became the most upstanding citizens he had ever seen after they were given their own perfect woman. It was like the power of love performs miracles.
That's when it started the flame of his ambition, and he began to seek out all of the resources and connections he could to continue performing these miracles. The government caught on and decided to collaborate with Jesus in order to combat the world's falling population numbers.
And so, Operation YAN was launched.
The initial batch targeted young, straight men who displayed too much maiden-less behavior to get and keep a lady–much like Jesus's friend. Instead of being upfront about the whole process, the government decided to plant Jesus's women into places these men would most likely frequent, such as adjacent houses making them neighbors.
Most of the women were kind of similar, which may be a result of the targeted men being similar. Friendly, loving, affectionate–so affectionate since they were born to love that these biologically engineered women were codenamed “Your Affectionate Neighbor” aka YAN.
Of course, success was expected and received. However, it may have worked too well…
These biologically engineered women were born to love, but humans are very complex creatures. Not only because these women were born literally days old as adults instead of growing up like natural human women, but because they were constructed to love only their target. Their target, of course, fell in love with them truly but they have their own lives too, whereas these YANs don’t. And the idea of their target leaving them or paying more attention to someone else was far too much for them to handle, that there became cases where these YANs would mercilessly kill anyone they perceived as love rivals.
Since most of these victims tended to be other women, Operation YAN extended to producing male YANs for single straight women in order to combat these jealousy allegations. Eventually this operation expanded their production to include producing YANs for homosexual, bisexual, asexual, etc people since apparently these YANs get jealous way too easily when it comes to meeting a person who is single. Love comes in all shapes and sizes, so having a platonic YAN by your side is better protection than not having one! 😀
Nowadays, you can even have a YAN that grows up with you–Pardon? That branch was discontinued due to general discomfort, pedophilic allegations and child murders? Of course, of course. Apologies, folks. Due to potential abuse of these YANs (whether you consider them human or not) and various ethical reasons, you must be an adult at the legal age of 25 to receive your very own YAN.
Why 25? That's because you can only receive one YAN in your lifetime! And it is very important that the details and preferences you fill out on your paperwork are very, very thought out.
Speaking of which, if you want to get your own YAN today, log into your personal tablet and fill out the required electronic work. Here is a preview:
You must be 25 and older to be legible to receive your very own YAN.
You must sign and print your first name, middle name (if applicable), and surname in all of the indicated boxes, to ensure your informed consent. You must also write down your Social Security number and your permanent address in all of the indicated boxes.
You must completely fill out your personality quiz to the best of your ability.
You must completely fill out your ideal type to the best of your ability.
You will be required to be fingerprinted and photographed for recognition purposes.
You will be required to supply a blood sample, a hair sample, a saliva sample, a urine sample, and a discharge sample from your genitals (if applicable–if you do not have genitals, then you do not need to provide this particular sample). We will have licensed doctors provided for you if need be.
Failure to complete all of the above properly will result in the negation of this application.
Finally, once you place your application, there are no refunds.
Allow us to repeat: ATTENTION!! THERE ARE NO TAKEBACKS. RETURNS ARE IMPOSSIBLE. YOUR YAN WAS CREATED JUST FOR YOU, THEREFORE IT IS IMPOSSIBLE TO REUSE SAID YAN FOR ANY OTHER PURPOSE EXCEPT TO LOVE YOU. IF YOU ARE UNHAPPY WITH YOUR YAN, PLEASE MAKE THE BEST OF IT. THERE ARE MANY SOURCES AVAILABLE, INCLUDING THERAPY, ONLINE VIDEOS, AND PETS. WE ARE NOT RESPONSIBLE FOR YOUR IRRESPONSIBILITY.
Thank you and have a wonderful life with your YAN. In Jesus, we trust. 😊
#random thoughts#sacrilegious thoughts#crackfic#yandere#imagines#yandere imagines#deuxcherise imagines
5 notes
·
View notes
Text
A specialized ink hardens when exposed to focused ultrasound waves, transforming into biologically compatible structures
Date: December 7, 2023
Source: Duke University
Summary: Engineers have developed a bio-compatible ink that solidifies into different 3D shapes and structures by absorbing ultrasound waves. Because the material responds to sound waves rather than light, the ink can be used in deep tissues for biomedical purposes ranging from bone healing to heart valve repair.
2 notes
·
View notes
Text
Unlocking the secrets of natural materials
New Post has been published on https://thedigitalinsider.com/unlocking-the-secrets-of-natural-materials/
Unlocking the secrets of natural materials
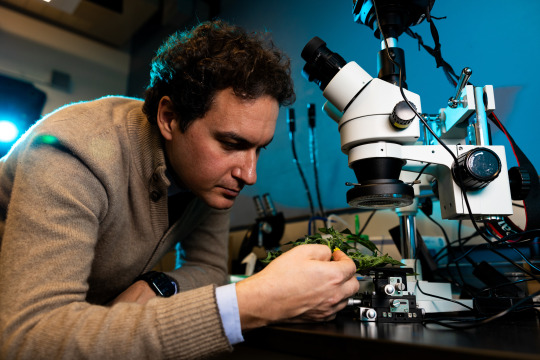
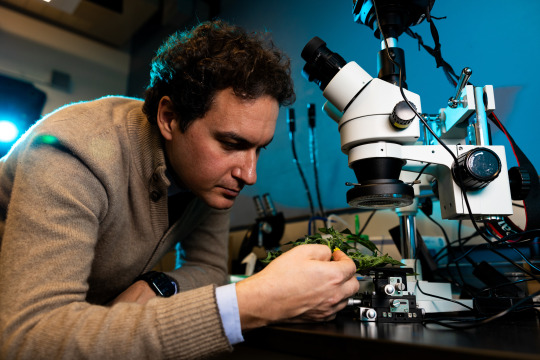
Growing up in Milan, Benedetto Marelli liked figuring out how things worked. He repaired broken devices simply to have the opportunity to take them apart and put them together again. Also, from a young age, he had a strong desire to make a positive impact on the world. Enrolling at the Polytechnic University of Milan, he chose to study engineering.
“Engineering seemed like the right fit to fulfill my passions at the intersection of discovering how the world works, together with understanding the rules of nature and harnessing this knowledge to create something new that could positively impact our society,” says Marelli, MIT’s Paul M. Cook Career Development Associate Professor of Civil and Environmental Engineering.
Marelli decided to focus on biomedical engineering, which at the time was the closest thing available to biological engineering. “I liked the idea of pursuing studies that provided me a background to engineer life,” in order to improve human health and agriculture, he says.
Marelli went on to earn a PhD in materials science and engineering at McGill University and then worked in Tufts University’s biomaterials Silklab as a postdoc. After his postdoc, Marelli was drawn to MIT’s Department of Civil and Environmental in large part because of the work of Markus Buehler, MIT’s McAfee Professor of Engineering, who studies how to design new materials by understanding the architecture of natural ones.
“This resonated with my training and idea of using nature’s building blocks to build a more sustainable society,” Marelli says. “It was a big leap forward for me to go from biomedical engineering to civil and environmental engineering. It meant completely changing my community, understanding what I could teach and how to mentor students in a new engineering branch. As Markus is working with silk to study how to engineer better materials, this made me see a clear connection with what I was doing and what I could be doing. I consider him one of my mentors here at MIT and was fortunate to end up collaborating with him.”
Marelli’s research is aimed at mitigating several pressing global problems, he says.
“Boosting food production to provide food security to an ever-increasing population, soil restoration, decreasing the environmental impact of fertilizers, and addressing stressors coming from climate change are societal challenges that need the development of rapidly scalable and deployable technologies,” he says.
Marelli and his fellow researchers have developed coatings derived from natural silk that extend the shelf life of food, deliver biofertilizers to seeds planted in salty, unproductive soils, and allow seeds to establish healthier plants and increase crop yield in drought-stricken lands. The technologies have performed well in field tests being conducted in Morocco in collaboration with the Mohammed VI Polytechnic University in Ben Guerir, according to Marelli, and offer much potential.
“I believe that with this technology, together with the common efforts shared by the MIT PIs participating in the Climate Grand Challenge on Revolutionizing Agriculture, we have a real opportunity to positively impact planetary health and find new solutions that work in both rural settings and highly modernized agricultural fields,” says Marelli, who recently earned tenure.
As a researcher and entrepreneur with about 20 patents to his name and awards including a National Science Foundation CAREER award, the Presidential Early Career Award for Scientists and Engineers award, and the Ole Madsen Mentoring Award, Marelli says that in general his insights into structural proteins — and how to use that understanding to manufacture advanced materials at multiple scales — are among his proudest achievements.
More specifically, Marelli cites one of his breakthroughs involving a strawberry. Having dipped the berry in an odorless, tasteless edible silk suspension as part of a cooking contest held in his postdoctoral lab, he accidentally left it on his bench, only to find a week or so later that it had been well-preserved.
“The coating of the strawberry to increase its shelf life is difficult to beat when it comes to inspiring people that natural polymers can serve as technical materials that can positively impact our society” by lessening food waste and the need for energy-intensive refrigerated shipping, Marelli says.
When Marelli won the BioInnovation Institute and Science Prize for Innovation in 2022, he told the journal Science that he thinks students should be encouraged to choose an entrepreneurial path. He acknowledged the steepness of the learning curve of being an entrepreneur but also pointed out how the impact of research can be exponentially increased.
He expanded on this idea more recently.
“I believe an increasing number of academics and graduate students should try to get their hands ‘dirty’ with entrepreneurial efforts. We live in a time where academics are called to have a tangible impact on our society, and translating what we study in our labs is clearly a good way to employ our students and enhance the global effort to develop new technology that can make our society more sustainable and equitable,” Marelli says.
Referring to a spinoff company, Mori, that grew out of the coated strawberry discovery and that develops silk-based products to preserve a wide range of perishable foods, Marelli says he finds it very satisfying to know that Mori has a product on the market that came out of his research efforts — and that 80 people are working to translate the discovery from “lab to fork.”
“Knowing that the technology can move the needle in crises such as food waste and food-related environmental impact is the highest reward of all,” he says.
Marelli says he tells students who are seeking solutions to extremely complicated problems to come up with one solution, “however crazy it might be,” and then do an extensive literature review to see what other researchers have done and whether “there is any hint that points toward developing their solution.”
“Once we understand the feasibility, I typically work with them to simplify it as much as we can, and then to break down the problem in small parts that are addressable in series and/or in parallel,” Marelli says.
That process of discovery is ongoing. Asked which of his technologies will have the greatest impact on the world, Marelli says, “I’d like to think it’s the ones that still need to be discovered.”
#2022#advanced materials#agriculture#architecture#background#Bioinspiration#Biological engineering#Building#career#career development#challenge#Civil and environmental engineering#climate#climate change#coatings#Collaboration#Community#cooking#Design#development#devices#energy#Engineer#engineering#engineers#Environmental#environmental impact#Faculty#Food#food production
2 notes
·
View notes
Text
Graphene-Based Biomedical Applications Market: Analysis and Forecast, 2025-2035

The graphene-based biomedical applications market is experiencing significant growth, driven by advancements in nanotechnology and increasing demand for innovative medical solutions. Graphene's exceptional properties, such as high electrical conductivity, mechanical strength, and biocompatibility, make it an ideal candidate for various biomedical applications. Key areas of focus include the development of flexible biosensors, drug delivery systems, and neural interfaces. However, challenges such as high production costs, scalability issues, and regulatory hurdles remain. Despite these obstacles, ongoing research and strategic collaborations are expected to accelerate the commercialization of graphene-based biomedical products, offering promising prospects for the healthcare industry.
Market Segmentation
1. By Application
Drug Delivery Systems (One of the prominent application segments)
Biosensors and Diagnostic Devices
Imaging and Diagnostics Platforms
Tissue Engineering and Regenerative Medicine
Antibacterial and Antimicrobial Agents
Cancer Therapeutics
Gene and Cell Therapy
2. By End-User
Hospitals and Clinics
Pharmaceutical and Biotechnology Companies (Estimated market leader in terms of end-user segment)
Research and Academic Institutions
Diagnostic Laboratories
Medical Device Manufacturers
3. By Product
Base Graphene Materials
Pristine Graphene / Single-layer Graphene
Few-layer Graphene
Graphene Oxide (GO)
Reduced Graphene Oxide (rGO)
Graphene Quantum Dots (GQDs)
Functionalized Graphene (Estimated market leader in terms of product segment)
4. By Region
North America: U.S., Canada, Mexico
Europe: Germany, France, Italy, U.K., Rest-of-Europe
Asia-Pacific: Australia, China, Japan, South Korea, India
Rest-of-the-World: South America, Middle East, Africa
Asia-Pacific is anticipated to gain traction in terms of production, owing to the continuous growth in the adoption and the presence of key manufacturers in the region.
Demand Drivers
Enhanced Biocompatibility of Graphene: Because of their exceptional biocompatibility, graphene and its derivatives can be seamlessly integrated into biological systems without triggering severe toxicity or immunological reactions. Their use in tissue engineering, drug delivery, and diagnostic devices is fueled by this characteristic.
Advancements in Nanotechnology Enhancing Graphene Functionality: The manufacturing, functionalization, and manipulation of graphene materials have improved due to ongoing advancements in nanotechnology, which have also increased the materials' surface area, electrical conductivity, and chemical reactivity. These improvements increase their performance and usefulness in biomedical applications such as imaging, therapies, and biosensors.
Download TOC for this report. Click Here!
Lean more about Advance Material and Chemical Vertical. Click Here!
Conclusion
The graphene-based biomedical applications market is poised for significant growth, driven by graphene’s superior biocompatibility and the rapid advancements in nanotechnology that enhance its functionality. Major end customers, including pharmaceutical corporations and research institutions, are supporting the growing adoption of key application areas such drug delivery systems, biosensors, and cancer treatments. Because of technical advancements and expanding manufacturing capacities, the functionalized graphene product segment and the Asia-Pacific region are anticipated to drive market expansion. In the near future, graphene's special qualities promise to transform biomedical technologies and provide more efficient, accurate, and customized healthcare solutions as issues like scalability and regulatory barriers are resolved.
#Graphene-Based Biomedical Applications Market#Graphene-Based Biomedical Applications Industry#Graphene-Based Biomedical Applications Report#Advance material#chemical
0 notes
Text
Microscopy Market Trends and Innovations Driving Growth Across Healthcare and Scientific Research Fields
The microscopy market has experienced significant growth over recent years, driven by advancements in technology and expanding applications across various industries. Microscopy, the technique of using microscopes to view objects that are not visible to the naked eye, is a critical tool in scientific research, healthcare, materials science, and many other fields. As technological innovations continue to evolve, the microscopy market is poised to expand even further, presenting new opportunities and challenges.

One of the primary factors fueling the growth of the microscopy market is the increasing demand for high-resolution imaging techniques in biomedical research. Researchers rely on advanced microscopy tools to observe cells, tissues, and microorganisms in greater detail, enabling breakthroughs in understanding diseases and developing new treatments. For instance, fluorescence microscopy and confocal microscopy have revolutionized biological research by providing three-dimensional images and precise localization of molecules within cells.
Healthcare is another major driver of the microscopy market. Pathologists and medical researchers use microscopes for diagnostic purposes, including cancer detection and infectious disease identification. With the rising prevalence of chronic diseases and the demand for personalized medicine, there is a growing need for more sophisticated and accurate microscopy techniques. Digital pathology, which combines microscopy with digital imaging and artificial intelligence (AI), is gaining traction as it allows remote diagnostics and automated image analysis, improving efficiency and accuracy.
The materials science sector also heavily relies on microscopy for analyzing the structural properties of metals, polymers, ceramics, and composites. Electron microscopy, including scanning electron microscopy (SEM) and transmission electron microscopy (TEM), is extensively used to study material composition and surface morphology at the nanoscale. This has significant implications for industries such as aerospace, automotive, electronics, and nanotechnology, where understanding material properties is crucial for innovation and quality control.
Technological advancements continue to be a cornerstone of growth in the microscopy market. The development of super-resolution microscopy techniques, such as stimulated emission depletion (STED) microscopy and structured illumination microscopy (SIM), has pushed the boundaries of optical resolution beyond the traditional limits set by light wavelength. These innovations allow scientists to observe molecular interactions and cellular structures with unprecedented clarity.
Another emerging trend is the integration of microscopy with digital technologies, such as AI, machine learning, and big data analytics. These integrations facilitate automated image processing, pattern recognition, and predictive analysis, significantly enhancing the speed and accuracy of microscopy-based research and diagnostics. Portable and smartphone-based microscopes are also becoming popular, especially in remote or resource-limited settings, expanding access to microscopy tools worldwide.
Geographically, the microscopy market is witnessing robust growth in North America and Europe due to well-established research infrastructure, high healthcare expenditure, and strong government funding. Asia-Pacific is emerging as a key region with rapid industrialization, increasing investments in research and development, and growing demand from pharmaceutical and biotechnology companies. The expanding middle class and improving healthcare infrastructure in countries like China and India contribute to market growth in this region.
Despite the promising prospects, the microscopy market faces challenges. The high cost of advanced microscopy equipment and the need for skilled operators can limit adoption, especially in smaller laboratories or in developing countries. Additionally, maintaining and calibrating sophisticated microscopes requires specialized knowledge and resources. Data management and storage are becoming critical issues as microscopy generates large volumes of high-resolution images that require efficient handling and analysis.
Companies in the microscopy market are focusing on product innovation, strategic collaborations, and expanding their global footprint to stay competitive. Major players are investing in research to develop more user-friendly, compact, and affordable microscopy solutions. Furthermore, collaborations between academia, healthcare institutions, and industry are accelerating the translation of microscopy technologies into practical applications.
In conclusion, the microscopy market is on a robust growth trajectory fueled by technological advancements, expanding applications in healthcare and materials science, and increasing global demand for high-resolution imaging. While challenges such as cost and technical complexity exist, ongoing innovations and the integration of digital technologies are likely to overcome these barriers. As microscopy continues to evolve, it will remain an indispensable tool driving scientific discovery and innovation across multiple disciplines.
#MicroscopyMarket#MicroscopyTechnology#BiomedicalResearch#HealthcareInnovation#MaterialScience#ScientificResearch
0 notes
Text
Future Outlook for Chitosan Market Indicates Strong Demand in Bio-Based Product Industries

The global chitosan market has garnered significant attention over recent years, driven by growing environmental concerns, increasing demand for sustainable materials, and expanding applications across multiple industries. Chitosan, a natural polysaccharide derived from chitin the second most abundant natural polymer found in crustacean shells has proven to be an exceptional biopolymer with wide-ranging functionalities. Its biodegradability, biocompatibility, and non-toxic nature have made it a preferred choice for industries including pharmaceuticals, agriculture, water treatment, food packaging, and cosmetics.
This article offers a comprehensive overview of the chitosan market, outlining its current status, key drivers, challenges, regional dynamics, and future outlook.
Understanding Chitosan and Its Significance
Chitosan is primarily produced by deacetylation of chitin, which is sourced mainly from shrimp, crab, and lobster shells byproducts of the seafood processing industry. This conversion yields a biopolymer with remarkable properties such as film-forming ability, antimicrobial activity, and the capacity to interact with various biological molecules. These unique attributes enable its use in diverse applications ranging from wound healing to agricultural bio-stimulants.
The rising global focus on reducing reliance on synthetic chemicals and plastics has further enhanced the importance of chitosan as an eco-friendly and sustainable material. This trend aligns with the increasing regulatory push for greener alternatives, accelerating the adoption of chitosan worldwide.
Market Size and Growth Trajectory
The global chitosan market is currently valued at approximately USD 8 billion and is projected to grow at a compound annual growth rate (CAGR) of around 10% over the next five to seven years. This growth is largely driven by the expanding demand in pharmaceutical, agriculture, and water treatment sectors, alongside emerging applications in food packaging and cosmetics.
Advances in biotechnology and processing technologies are enabling manufacturers to improve chitosan’s quality and functionality, broadening its scope across high-value industries such as healthcare and environmental management. The market’s growth trajectory is expected to maintain momentum as research continues to unlock new applications and production methods.
Key Market Drivers
Several factors are catalyzing the growth of the chitosan market:
Sustainability Imperatives: Increasing environmental regulations aimed at reducing plastic pollution and chemical usage promote the adoption of biodegradable polymers like chitosan.
Biomedical Applications: Chitosan’s biocompatibility and antimicrobial properties make it highly desirable in wound care, drug delivery systems, and tissue engineering, where demand continues to rise due to aging populations and chronic diseases.
Agricultural Use: The push toward sustainable farming has expanded the use of chitosan as a natural pesticide and bio-stimulant, helping improve crop yields while minimizing chemical residues.
Water Treatment Needs: Chitosan serves as an effective, natural flocculant for removing heavy metals and organic contaminants from wastewater, making it valuable in meeting increasingly strict environmental standards.
Food Industry Trends: With consumers seeking natural preservatives and eco-friendly packaging, chitosan-based edible coatings and films have gained popularity for extending shelf life and reducing plastic waste.
Cosmetic Industry Demand: The shift toward clean beauty and natural ingredients has driven cosmetic manufacturers to incorporate chitosan for its moisturizing and antimicrobial benefits.
Market Segmentation
The chitosan market can be segmented by source, application, and form:
Source: While the majority of chitosan is derived from crustacean shells, fungal-based chitosan is emerging as a promising alternative due to its allergen-free nature and consistent supply unaffected by seafood industry fluctuations.
Application: Key applications include pharmaceuticals and biomedical products, agriculture, water treatment, food and beverage, and cosmetics. Among these, pharmaceuticals and water treatment currently dominate demand.
Form: Chitosan is available in powder, film, and solution forms, catering to diverse industrial requirements. Powdered chitosan remains the most widely used due to its versatility and ease of handling.
Regional Market Dynamics
Asia-Pacific leads the global chitosan market, accounting for the largest share, thanks to its vast seafood industry providing abundant raw materials and growing end-use sectors. Countries such as China, India, Japan, and South Korea are significant producers and consumers. Regional government initiatives promoting sustainability and bio-based materials further support market expansion.
North America and Europe are mature markets characterized by stringent regulatory environments and strong emphasis on innovation. These regions focus heavily on advanced biomedical and environmental applications, supported by substantial R&D investments.
Emerging markets in Latin America, the Middle East, and Africa present promising opportunities, driven by rising environmental concerns and increasing regulatory frameworks encouraging sustainable practices.
Challenges Facing the Market
Despite robust growth prospects, the chitosan market faces several challenges:
Raw Material Supply Variability: Dependence on crustacean shells ties production to the seafood industry, which can be affected by seasonal and environmental factors.
Cost and Production Complexity: Traditional chemical extraction methods are energy-intensive and generate hazardous waste, raising costs and environmental concerns.
Regulatory Barriers: Variations in global regulations for food, pharmaceutical, and cosmetic applications can impede market entry and product approval.
To mitigate these issues, innovations in enzymatic extraction and fungal-derived chitosan production are gaining traction, promising to reduce environmental impact and stabilize supply chains.
Future Outlook
The outlook for the chitosan market remains positive, with continuous innovation and growing awareness driving expanded adoption. Research into nanotechnology and functionalization is creating new biomedical and industrial applications, while efforts to improve production efficiency and sustainability are making chitosan more accessible.
As governments and industries intensify their commitment to sustainability, chitosan’s role as a biodegradable, versatile material is expected to strengthen, opening up new growth avenues across diverse sectors.
Conclusion
Chitosan’s unique properties and eco-friendly profile have positioned it as a key player in the global transition toward sustainable materials. Its diverse applications, coupled with rising environmental and health concerns, underpin the market’s steady growth.
Though challenges such as raw material dependency and production costs persist, ongoing technological advancements and alternative sourcing methods provide promising solutions. Understanding the evolving landscape of the chitosan market is essential for stakeholders looking to capitalize on the opportunities presented by this dynamic and expanding industry.
0 notes
Text
Engineering Masters Courses in Ireland for International Students
A lot of international students now choose Ireland for its great engineering programs and educational opportunities. Unlike other programs, Irish engineering master’s courses focus on honing students’ knowledge and skills for practical and industrial use through research, innovation and strong partnerships. Thanks to innovative infrastructure, busy tech centers and partnerships with worldwide firms, Canada offers superb conditions for developing engineers.
This blog looks at the leading engineering master’s courses available to international students in Ireland, focusing on their details, leading universities, admission steps, expenses, scholarships, and career benefits.
Why Study Engineering in Ireland?
Before diving into the courses, let’s look at why Ireland is an ideal choice for pursuing a master's degree in engineering:
1. Globally Recognized Universities
Many of the top global universities are found in Ireland, including Trinity College Dublin, University College Dublin and University College Cork.
2. Industry-Oriented Curriculum
Engineering courses in Ireland are created in step with what the industries require, so students can find work quickly after graduation.
3. Post-Study Work Opportunities
After joining a master's program in Ireland, non-EU students can apply for a two-year stay-back visa and use their time to gain important job experience.
4. English-Taught Programs
All master’s programs in engineering are taught in English, making it easier for international students to adapt and succeed.
5. Tech and Engineering Hub
Ireland hosts major multinational companies like Google, Intel, Pfizer, Medtronic, and Apple, providing graduates with excellent career opportunities in engineering, technology, and R&D.
Popular Engineering Master’s Courses in Ireland
Ireland offers a diverse selection of engineering master’s courses. Here are some of the most in-demand specialisations:
1. Mechanical Engineering
Covers areas such as thermodynamics, materials, control systems, and design. Graduates can work in manufacturing, energy, aerospace, and more.
Top Universities Offering This Course:
University College Dublin (UCD)
University of Limerick (UL)
Cork Institute of Technology
2. Civil and Structural Engineering
Focuses on construction, environmental engineering, and infrastructure development. Ireland’s growing infrastructure sector creates high demand for civil engineers.
Top Universities Offering This Course:
Trinity College Dublin
National University of Ireland Galway (University of Galway)
Dublin Institute of Technology (TU Dublin)
3. Electrical and Electronic Engineering
Covers advanced topics such as embedded systems, power electronics, digital communication, and circuit design.
Top Universities Offering This Course:
University College Dublin (UCD)
Dublin City University (DCU)
Maynooth University
4. Computer and Software Engineering
Ideal for students interested in AI, cybersecurity, data analytics, and software systems. Given Ireland's booming tech industry, this is a highly sought-after course.
Top Universities Offering This Course:
Trinity College Dublin
University College Cork (UCC)
Technological University of the Shannon (TUS)
5. Biomedical Engineering
Combines engineering principles with biological sciences to develop healthcare technologies. Ireland has a strong medical device and pharma sector.
Top Universities Offering This Course:
University of Galway
University of Limerick
University College Dublin
6. Chemical and Process Engineering
Focuses on process design, manufacturing, energy systems, and material science. Suited for careers in pharma, energy, and food industries.
Top Universities Offering This Course:
University College Dublin
University College Cork
Dublin City University
Top Universities in Ireland for Engineering Master’s Courses
Here are some of the best universities offering engineering master’s degrees in Ireland:
1. Trinity College Dublin (TCD)
Ranked among the top 100 universities globally.
Offers diverse specialisations in mechanical, computer, and biomedical engineering.
Strong links with industry and research centers.
2. University College Dublin (UCD)
One of Ireland’s leading research-intensive universities.
Offers high-quality engineering programs with modern facilities.
Known for strong industry collaborations.
3. University of Galway
Renowned for civil and biomedical engineering programs.
Excellent placement and research opportunities.
Located in a vibrant student city with tech and pharma hubs.
4. University College Cork (UCC)
Offers accredited engineering master’s programs.
Strong research output and partnerships with global companies.
Focus on sustainability and green energy engineering.
5. University of Limerick (UL)
Offers practice-based learning and co-op placements.
Recognized for mechanical and biomedical engineering.
Close ties with local and international industries.
Admission Requirements for International Students
While specific requirements vary by institution and course, here are the general criteria for admission:
Bachelor’s Degree: A relevant undergraduate degree in engineering or a related field.
Academic Transcripts: Certified copies of previous academic records.
English Language Proficiency:
IELTS: Minimum 6.5 overall (with no band less than 6.0)
TOEFL iBT: 90+
PTE Academic: 63+
Statement of Purpose (SOP): A well-written essay explaining your academic background, goals, and reasons for choosing the course.
Letters of Recommendation: Usually 2 academic or professional references.
Resume/CV: Detailing your academic and professional experience.
Some universities may require interviews or entrance exams depending on the program.
Tuition Fees for Engineering Master’s Programs in Ireland
The tuition fees for engineering master’s programs in Ireland vary based on the university and course. On average, international students can expect:
€13,000 – €25,000 per year
Additional costs may include student registration fees, health insurance, and lab fees.
Cost of Living in Ireland for International Students
The average cost of living in Ireland ranges between €10,000 and €15,000 per year, depending on your lifestyle and location. Major expenses include:
Accommodation: €400 – €800/month
Food & Utilities: €200 – €300/month
Transport: €50 – €100/month
Books & Supplies: €75 – €100/month
Miscellaneous: €100 – €200/month
Students are allowed to work part-time (up to 20 hours/week during term and 40 hours/week during holidays), which can help cover living costs.
Career Opportunities After Graduation
Engineering graduates in Ireland enjoy strong employability thanks to the country's thriving industrial sectors. With the 2-year stay-back option (Third Level Graduate Scheme), students can search for jobs and apply for work permits or permanent residency.
Key Industries Hiring Engineers:
Information Technology and Software
Civil Infrastructure and Construction
Biomedical and Healthcare Devices
Renewable Energy
Pharmaceuticals and Chemical Engineering
Manufacturing and Automation
Popular Job Roles:
Mechanical Engineer
Civil/Structural Engineer
Software Developer/Engineer
Biomedical Engineer
Process Engineer
Electrical/Electronics Engineer
Data Engineer/Analyst
Average starting salaries for engineering graduates in Ireland range from €30,000 to €45,000, depending on the specialisation and company.
How Cliftons Study Abroad Can Help You
At Cliftons Study Abroad, we make your journey smoother with personalized support from start to finish. Our services include:
Free one-on-one counselling
Course and university selection
Application and admission guidance
Visa documentation and interview prep
Scholarship assistance
Educational loan guidance
Post-landing support in Ireland
Our expert team works closely with top universities in Ireland to ensure that you secure the best engineering program that suits your interests and career goals.
Final Thoughts
Earning a master’s degree in engineering in Ireland gives you the chance to expand your knowledge, build your career and create personal benefits. Because of its strong education, industry links, friendly atmosphere and many work options, Ireland is favored by international engineering students.
If you have an interest in mechanical systems, civil infrastructure, biomedical technologies or the newest software, you’ll discover a relevant program in Ireland.
Ready to take the next step? Get in touch with Cliftons Study Abroad today and let us help you make your study abroad dreams a reality!
#study abroad#study in uk#study abroad consultants#study in germany#study in australia#study in ireland#study blog
0 notes
Text
From Traditional Braiding Methods to Additive Manufacturing for Fabricating Mckibben Artificial Muscles
From Traditional Braiding Methods to Additive Manufacturing for Fabricating Mckibben Artificial Muscles in Biomedical Journal of Scientific & Technical Research
McKibben artificial muscles are among the most pragmatic accessible artificial muscles due to their similar performance to biological muscles, braided sleeve is an essential part of Mckibben artificial muscles. The main task of this part after activation the muscle is to transform the generated radius force inside the inner tube into the length direction force. The methods used to design, materials used in manufacturing and geometry of the braided sleeve directly affect the performance of this part during the actuation. This review gives a brief overview of the manufacturing trend of these materials and also provides some future directions.
For more articles in Journals on Biomedical Sciences click here bjstr
Follow on Twitter : https://twitter.com/Biomedres01/ Follow on Blogger :https://biomedres01.blogspot.com/ Like Our Pins On : https://www.pinterest.com/biomedres/
#Medical and Medicinal Journal#Biomedical Journal Impact Factor#Biomedical Open Access Journals#journal of biomedical research and reviews#open access journals of biomedical science
1 note
·
View note
Text

Pong prodigy: Hydrogel material shows unexpected learning abilities
In a study published22 August in Cell Reports Physical Science, a team led by Dr. Yoshikatsu Hayashi demonstrated that a simple hydrogel—a type of soft, flexible material—can learn to play the simple 1970s computer game "Pong." The hydrogel, interfaced with a computer simulation of the classic game via a custom-built multi-electrode array, showed improved performance over time. Dr. Hayashi, a biomedical engineer at the University of Reading's School of Biological Sciences, said, "Our research shows that even very simple materials can exhibit complex, adaptive behaviors typically associated with living systems or sophisticated AI. "This opens up exciting possibilities for developing new types of 'smart' materials that can learn and adapt to their environment."
Read more.
11 notes
·
View notes
Text
Nonpolymeric Organic Nanomaterials Market Size, Demand & Supply, Regional and Competitive Analysis 2025–2031
Definition
Nonpolymeric Organic Nanomaterials are nanoscale materials composed of organic molecules that do not include polymeric chains. These nanomaterials often consist of carbon-based compounds, small organic molecules, and natural organic substances. Their unique properties—such as high surface area, biocompatibility, and tunable chemical functionality—make them suitable for diverse applications including drug delivery, biosensing, catalysis, electronics, and environmental remediation.
Unlike their polymer-based counterparts, nonpolymeric organic nanomaterials offer enhanced flexibility in molecular design, enabling the development of highly specialized nanostructures. They are especially useful in precision medicine, sustainable energy, and high-performance materials.
Market Size
📎 Download a Sample Report PDF https://www.24chemicalresearch.com/download-sample/292794/nonpolymeric-organic-nanomaterials-market
As of 2023, the global Nonpolymeric Organic Nanomaterials market was valued at USD 482 million and is projected to reach USD 764 million by 2031, expanding at a CAGR of 5.9% over the forecast period. The increasing use of organic nanomaterials in biomedical applications, green technologies, and electronics has significantly boosted demand.
Growth Projections and Trends
The market is expected to experience robust growth due to the rise in nanotechnology adoption across industries such as pharmaceuticals, semiconductors, and environmental engineering. The surge in demand for low-toxicity, biodegradable, and sustainable nanomaterials is particularly fueling the uptake of nonpolymeric organic types.
Key trends include:
Integration into targeted drug delivery systems.
Application in nano-biosensors for disease diagnostics.
Use in green catalysis and environmental cleanup processes.
Demand from flexible electronics and solar energy harvesting devices.
Market Dynamics
📈 Drivers
Rise in Biomedical Applications: These nanomaterials are increasingly used for drug delivery, gene therapy, and diagnostic imaging due to their biocompatibility and customizable surface chemistry.
Environmental Sustainability Focus: Favorable regulations and industry shifts toward eco-friendly nanomaterials.
Advancement in Nanofabrication Technologies: Innovations in bottom-up synthesis and molecular self-assembly are enhancing production scalability.
📉 Restraints
High R&D Costs: Developing new materials and applications requires significant investment in research infrastructure.
Limited Commercialization: Despite potential, many innovations are still in lab-scale testing or pilot programs.
💡 Opportunities
Growth in Precision Medicine: Nanomaterials tailored for specific biological targets.
Emerging Applications in Renewable Energy: Including light-harvesting nanostructures and organic photovoltaic cells.
Government and Private Funding for Nanotech: Particularly in Asia-Pacific and Europe.
⚠️ Challenges
Regulatory Hurdles: Standardization, toxicity testing, and compliance requirements remain a challenge.
Scalability of Production: Transitioning from lab-scale synthesis to industrial production can be complex and expensive.
Regional Analysis
🌎 North America
Dominates the market due to a strong biotech sector, nanotech R&D initiatives, and government support. The U.S. is a key contributor, with significant investment in healthcare applications.
🌍 Europe
Holds a considerable share owing to stringent environmental regulations encouraging the adoption of green nanomaterials, especially in Germany, France, and the UK.
🌏 Asia-Pacific
Expected to register the fastest growth rate due to growing industrialization and innovation hubs in countries like China, Japan, and South Korea. Government initiatives and increased funding in nanosciences are key drivers.
🌎 Latin America & MEA
Emerging regions with increasing investment in nanotechnology research and potential for adoption in agriculture, healthcare, and environmental sectors.
Competitor Analysis
Key Market Players
ACS Materials LLC
Nanocomposix Inc.
Merck KGaA
US Research Nanomaterials Inc.
Strem Chemicals Inc.
These companies are focusing on:
Expanding nanomaterial portfolios.
Collaboration with research institutes.
Technological advancements in synthesis techniques.
Market Segmentation
By Application:
Biomedical and Healthcare (Drug delivery, Imaging)
Electronics and Photonics
Catalysis
Environmental Remediation
Others
By Type:
Fullerenes and Derivatives
Carbon Dots
Nanocrystals
Organic Nanotubes
Others
FAQs
1. What is the current market size of Nonpolymeric Organic Nanomaterials? The global market is valued at USD 482 million in 2023 and is projected to reach USD 764 million by 2031.
2. Which industries are the major consumers of these nanomaterials? Key industries include biomedical, electronics, energy, and environmental remediation.
3. What are the major drivers for market growth? The main drivers are demand for sustainable materials, biomedical advancements, and increasing applications in green energy technologies.
4. Who are the leading players in the market? Major companies include ACS Materials, Nanocomposix, Merck KGaA, US Research Nanomaterials, and Strem Chemicals.
5. Which region is expected to witness the fastest growth? The Asia-Pacific region is projected to grow at the highest CAGR due to government funding and industrial expansion.
📘 Get The Complete Report & TOC https://www.24chemicalresearch.com/reports/292794/nonpolymeric-organic-nanomaterials-market-2024-2031-899
🔗 Follow Us on LinkedIn https://www.linkedin.com/company/24chemicalresearch
0 notes
Text
North America Ultra-Low Temperature Freezer Market Global Trends, Statistics, Size, Share, Regional Analysis by Key Players (2019-2027)
The North America ultra-low temperature freezer market is expected to reach US$ 313.19 Mn in 2027 from US$ 213.42 Mn in 2018. The market is anticipated to grow with a CAGR of 4.7% from 2019-2027.
North America Ultra-Low Temperature Freezer Market Introduction
Indispensable for the storage of critical biological materials such as viruses, bacteria, eukaryotic cells, blood, and semen, Ultra-Low Temperature freezers are specialized equipment. They are widely used in key sectors including blood banks, hospitals, epidemic control services, research organizations, and biomedical engineering companies. A major factor contributing to market growth is the increasing demand for blood and blood-derived products. However, the considerable expense associated with purchasing ultra-low temperature freezers is anticipated to hinder market expansion.
Download our Sample PDF Report
@ https://www.businessmarketinsights.com/sample/TIPRE00012985
North America Ultra-Low Temperature Freezer Strategic Insights
Strategic insights into the North America Ultra-Low Temperature Freezer market deliver data-driven analyses of the current industry environment. This includes identifying present market trends, profiling major industry players, and detailing specific regional market characteristics. These insights offer practical recommendations, enabling readers to gain a competitive edge by discovering unexploited market segments or developing unique value propositions. By effectively utilizing data analytics, these insights assist industry participants, whether investors, manufacturers, or other stakeholders, in foreseeing upcoming market shifts. Maintaining a forward-looking perspective is crucial, aiding stakeholders in predicting market evolutions and strategically positioning themselves for sustained success within this dynamic North American market. Ultimately, robust strategic insights equip readers to make well-grounded decisions that enhance profitability and support the achievement of their business objectives within the sector.
North America Ultra-Low Temperature Freezer Regional Insights
The geographic scope of the North America Ultra-Low Temperature Freezer market delineates the precise territories in which businesses operate and compete. A deep understanding of local differences, such as the diverse preferences of consumers (for instance, the need for specific electrical plug types or the required duration of battery backup systems), varying economic climates, and differing regulatory frameworks, is vital for customizing strategies to particular markets. Businesses can expand their market reach by identifying underserved areas or adapting their product offerings to align with local requirements. A focused market approach facilitates more efficient resource management, the implementation of targeted marketing initiatives, and improved competitive positioning relative to local players, ultimately fostering growth within those specific target regions.
NORTH AMERICA ULTRA-LOW TEMPERATURE FREEZER MARKET SEGMENTATION
North America Ultra-Low Temperature Freezer Market: By Type
Upright ULT Freezers
Chest ULT Freezers
North America Ultra-Low Temperature Freezer Market: By End-User
Bio-Banks
Hospital
Pharmaceutical and Biotechnology Companies
Academic and Research Institute
North America Ultra-Low Temperature Freezer Market: By Geography
• North America
US
Canada
Mexico
North America Ultra-Low Temperature Freezer Market: Company Profiles
Helmer Scientific Inc.
Eppendorf AG
THERMO FISHER SCIENTIFIC INC.
Arctiko
Haier Biomedical
About Us:
Business Market Insights is a market research platform that provides subscription service for industry and company reports. Our research team has extensive professional expertise in domains such as Electronics & Semiconductor; Aerospace & Defense; Automotive & Transportation; Energy & Power; Healthcare; Manufacturing & Construction; Food & Beverages; Chemicals & Materials; and Technology, Media, & Telecommunications
#North America Ultra-Low Temperature Freezer Market#North America Ultra-Low Temperature Freezer Market Trends#North America Ultra-Low Temperature Freezer Market Statistics
0 notes
Text
Why Biomedical Waste Bags Are Critical for Healthcare Safety
In the world of healthcare, safety isn't just a priority—it’s a necessity. From the instruments used in surgeries to the gloves worn by nurses, everything that comes in contact with patients has to be carefully managed. One often overlooked but absolutely essential component in this chain of safety is the biomedical waste bag. These simple-looking bags are the frontline defense in preventing the spread of infection, contamination, and environmental hazards. In this blog, we’ll dive deep into why biomedical waste bags are critical for healthcare safety and how they’re more than just trash bags in a hospital.
Understanding Biomedical Waste and Its Dangers
Biomedical waste isn’t your regular household trash. It includes anything that has been contaminated with bodily fluids, infectious agents, or hazardous chemicals. Think used syringes, surgical gloves, dressings soaked with blood, discarded medicines, and even lab cultures. Improper disposal of such waste can lead to:
Spread of infectious diseases such as HIV, Hepatitis B and C
Environmental pollution from hazardous substances
Injuries due to sharp objects like needles or scalpels
Risk to waste handlers, cleaners, and even the public
This is where biomedical waste bags come into play.
Purpose of Biomedical Waste Bags in Healthcare Settings
Every hospital, clinic, diagnostic lab, or even veterinary center deals with biohazardous material daily. To safely handle and dispose of it, the healthcare system relies heavily on biomedical waste bags and biohazard waste bag options. These bags are designed specifically to:
Contain and segregate infectious and hazardous waste
Prevent leakage and exposure
Make handling, transportation, and disposal easier
Ensure compliance with safety and environmental regulations
Unlike ordinary trash bags, biohazard bags are color-coded, puncture-resistant, and made of high-density materials to withstand the weight and composition of medical waste.
Color Coding System and Its Significance
The color of a biomedical waste bag isn’t for aesthetics—it’s a crucial part of the waste management system. Each color represents a specific category of waste and directs how it should be treated and disposed of. Here’s a quick rundown:
Red bags – Used for infectious waste like tubing, catheters, and IV sets
Yellow bags – For pathological waste like human tissues, organs, and body parts
Blue/White translucent bags – For glassware and metallic body implants
Black bags – For non-hazardous, general waste
Correct segregation is vital. Mixing up these categories can result in contamination, regulatory violations, and unsafe disposal practices.
Benefits of Using Biomedical Waste Bags
Hospitals and medical centers can’t afford to compromise when it comes to safety. The benefits of using the right biomedical waste bag system include:
Protection for healthcare workers: Prevents accidental contact with infectious or harmful materials
Safety for waste handlers: Minimizes injury risks and exposure during collection and transport
Compliance with legal regulations: Avoids heavy fines and sanctions from health authorities
Environmental safety: Ensures hazardous waste doesn't contaminate soil, air, or water sources
Biohazard Waste Bags: A Deeper Look
Biohazard waste bag types are specifically designed for waste that contains potential biological threats. You’ll typically see these with a universal biohazard symbol printed in bright red or orange, warning anyone who handles it. These bags are:
Leak-proof and tear-resistant
Able to withstand autoclaving (for sterilization before disposal)
Sealable to prevent spillage
Made to be incinerated without releasing toxic fumes
When disposing of these bags, they are often treated with methods like incineration or chemical disinfection, depending on the type of waste and local regulations.
Consequences of Improper Disposal
Failure to use proper biomedical waste bags or mixing biomedical waste with regular garbage can lead to severe consequences, such as:
Legal penalties and fines for healthcare providers
Health hazards to hospital staff, patients, and the general public
Spread of deadly infections in communities
Long-term environmental degradation
And these consequences aren’t theoretical. There have been numerous cases globally where mishandled biohazardous waste caused public health crises and legal action against responsible organizations.
How to Ensure Proper Usage
To ensure safety and compliance, hospitals and medical facilities should follow these best practices:
Train all healthcare staff on waste segregation and disposal
Use only certified biomedical waste bags and bio hazard bags
Always seal and label the bags correctly before transport
Have a dedicated collection and disposal schedule
Audit and monitor waste management procedures regularly
Key Takeaways
Let’s wrap up with the core reasons why biomedical waste bags are a must in healthcare:
They prevent contamination and infection
Support safe handling and disposal of hazardous materials
Comply with medical and environmental regulations
Play a vital role in protecting public health and the environment
While they may seem like a minor part of hospital supplies, biohazard bags are truly unsung heroes in maintaining hygiene and safety standards. Without them, the risks would be catastrophic. Think of them as the first and last line of defense in a world where one small mistake can lead to a big outbreak.
#BiomedicalWaste#BiohazardBags#MedicalWasteDisposal#HealthcareSafety#InfectionControl#WasteManagement#HospitalWaste#BiohazardSafety#SafeDisposal#MedicalWasteManagement#HealthcareWaste#HazardousWaste#SustainableHealthcare#WasteSegregation#ClinicalWaste
0 notes
Text
Graphene-Based Biomedical Applications Market: Industry Overview

The graphene-based biomedical applications market is experiencing significant growth, driven by advancements in nanotechnology and increasing demand for innovative medical solutions. Graphene's exceptional properties, such as high electrical conductivity, mechanical strength, and biocompatibility, make it an ideal candidate for various biomedical applications. Key areas of focus include the development of flexible biosensors, drug delivery systems, and neural interfaces. However, challenges such as high production costs, scalability issues, and regulatory hurdles remain. Despite these obstacles, ongoing research and strategic collaborations are expected to accelerate the commercialization of graphene-based biomedical products, offering promising prospects for the healthcare industry.
What are graphene-based biomedical applications?
Applications harnessing the unique properties of graphene, such as high electrical conductivity, mechanical strength, and biocompatibility, to develop advanced medical technologies. These applications encompass a range of areas, including drug and gene delivery systems, biosensors, bioimaging, tissue engineering, and neural interfaces. Graphene's versatility allows for the creation of multifunctional platforms that can address multiple medical needs simultaneously, offering potential advancements in personalized medicine and minimally invasive therapies. Ongoing research continues to explore and expand the scope of graphene's role in biomedicine.
Frequently Asked Questions (FAQs) About Graphene-Based Biomedical Applications Market:
Is graphene safe for use in the human body?
Graphene shows great potential for biomedical use, but its long-term safety in the human body is still under investigation.
What is the market size and projected growth of graphene biomedical applications?
The graphene-based biomedical applications market is experiencing significant growth, driven by advancements in nanotechnology and increasing demand for innovative medical solutions.
Market Segmentation
1. By Application
Drug Delivery Systems (One of the prominent application segments)
Biosensors and Diagnostic Devices
Imaging and Diagnostics Platforms
Tissue Engineering and Regenerative Medicine
Antibacterial and Antimicrobial Agents
Cancer Therapeutics
Gene and Cell Therapy
2. By End-User
Hospitals and Clinics
Pharmaceutical and Biotechnology Companies (Estimated market leader in terms of end-user segment)
Research and Academic Institutions
Diagnostic Laboratories
Medical Device Manufacturers
3. By Product
Base Graphene Materials
Pristine Graphene / Single-layer Graphene
Few-layer Graphene
Graphene Oxide (GO)
Reduced Graphene Oxide (rGO)
Graphene Quantum Dots (GQDs)
Functionalized Graphene (Estimated market leader in terms of product segment)
Which regions are leading the production and adoption of graphene-based biomedical products?
North America: U.S., Canada, Mexico
Europe: Germany, France, Italy, U.K., Rest-of-Europe
Asia-Pacific: Australia, China, Japan, South Korea, India
Rest-of-the-World: South America, Middle East, Africa
Asia-Pacific is anticipated to gain traction in terms of production, owing to the continuous growth in the adoption and the presence of key manufacturers in the region.
Demand Drivers
Enhanced Biocompatibility of Graphene: Because of their exceptional biocompatibility, graphene and its derivatives can be seamlessly integrated into biological systems without triggering severe toxicity or immunological reactions. Their use in tissue engineering, drug delivery, and diagnostic devices is fueled by this characteristic.
Advancements in Nanotechnology Enhancing Graphene Functionality: The manufacturing, functionalization, and manipulation of graphene materials have improved due to ongoing advancements in nanotechnology, which have also increased the materials' surface area, electrical conductivity, and chemical reactivity. These improvements increase their performance and usefulness in biomedical applications such as imaging, therapies, and biosensors.
Download TOC for this report. Click Here!
Lean more about Advance Material and Chemical Vertical. Click Here!
Conclusion
The graphene-based biomedical applications market is poised for significant growth, driven by graphene’s superior biocompatibility and the rapid advancements in nanotechnology that enhance its functionality. Major end customers, including pharmaceutical corporations and research institutions, are supporting the growing adoption of key application areas such drug delivery systems, biosensors, and cancer treatments. Because of technical advancements and expanding manufacturing capacities, the functionalized graphene product segment and the Asia-Pacific region are anticipated to drive market expansion. In the near future, graphene's special qualities promise to transform biomedical technologies and provide more efficient, accurate, and customized healthcare solutions as issues like scalability and regulatory barriers are resolved.
#Graphene-Based Biomedical Applications Market#Graphene-Based Biomedical Applications Industry#Graphene-Based Biomedical Applications Report
0 notes
Text
Microfluidic Devices: Shaping the Future of Lab-on-a-Chip Technology
The global microfluidic devices market is witnessing sustained growth as technological innovations, rising healthcare demands, and the shift toward miniaturized, portable diagnostic solutions continue to drive adoption. Microfluidics—the manipulation of fluids at the sub-millimeter scale—is rapidly transforming the landscape of healthcare, biotechnology, and pharmaceuticals, with applications ranging from lab-on-a-chip devices to high-throughput drug screening platforms.
Industry stakeholders across diagnostics, life sciences, and drug delivery sectors are increasingly investing in microfluidic solutions to enhance sensitivity, reduce reagent consumption, and streamline complex biological workflows. As microfluidic technology evolves, it is becoming a core enabler of next-generation medical diagnostics and personalized therapies.

Technological Advancements Accelerate Adoption
One of the central growth engines of the microfluidic devices market is the rapid advancement in microfabrication techniques. Innovations in soft lithography, 3D printing, and injection molding have enabled the design and production of microfluidic devices with improved precision, scalability, and cost efficiency. These advancements have lowered the entry barrier for new players and facilitated the production of complex, integrated systems for diverse biomedical applications.
Moreover, integration with electronics, sensors, and wireless communication modules is making microfluidic systems more intelligent and versatile. The convergence of microfluidics with fields such as nanotechnology, artificial intelligence, and biosensing has opened up new possibilities in disease detection, drug testing, and therapeutic monitoring.
Rising Demand for Point-of-Care and Home-Based Testing
The post-pandemic shift in diagnostic practices has heightened interest in decentralized and rapid testing. Microfluidic devices are at the forefront of this movement due to their capability to perform accurate and fast assays using minimal sample volumes. Point-of-care testing (POCT) applications—such as infectious disease screening, glucose monitoring, and cardiovascular risk assessment—are increasingly relying on microfluidic platforms for efficiency and portability.
Home-based diagnostic tools powered by microfluidics are gaining prominence, particularly in chronic disease management and preventive healthcare. The scalability and ease of integration into user-friendly formats such as lateral flow assays and wearable sensors are bolstering the adoption of microfluidic technology among both clinicians and consumers.
Expansion in Life Sciences and Drug Development
Beyond diagnostics, microfluidic devices are playing a pivotal role in revolutionizing the drug discovery and development process. Their ability to simulate in vivo environments on a microscale makes them ideal for organ-on-chip, cell culture, and high-throughput screening applications. Pharmaceutical companies are increasingly turning to microfluidic platforms to reduce lead times and improve the accuracy of preclinical testing.
Lab-on-a-chip systems, in particular, are enabling researchers to conduct complex biological and chemical analyses on a single, compact platform. This reduces reagent consumption and speeds up data generation, supporting faster decision-making in research and development pipelines.
Additionally, microfluidic devices are contributing to the growing field of precision medicine by facilitating real-time monitoring and enabling personalized therapeutic interventions based on individual biological responses.
Material Innovation and Manufacturing Trends
The choice of materials in microfluidic device fabrication has a significant impact on functionality, cost, and scalability. While traditional materials like glass and silicon have offered excellent performance in early-stage development, polymers such as polydimethylsiloxane (PDMS), polymethyl methacrylate (PMMA), and cyclic olefin copolymers (COC) are gaining popularity due to their cost-effectiveness, ease of prototyping, and biocompatibility.
The shift toward disposable and low-cost microfluidic devices is influencing material innovation. Manufacturers are focusing on developing hybrid devices that combine the benefits of different materials to optimize performance and manufacturability. Automated production techniques and roll-to-roll manufacturing are being explored to meet the increasing demand and facilitate large-scale deployment.
Regulatory Landscape and Standardization Efforts
The expanding applications of microfluidic devices in clinical and research settings necessitate stringent regulatory oversight and robust quality control. Regulatory authorities across key regions are increasingly developing frameworks for evaluating the safety, effectiveness, and consistency of microfluidic-based diagnostic and therapeutic devices.
Standardization of microfluidic components and interfaces is emerging as a critical focus area, especially in the context of interoperability and modularity. Industry bodies and consortiums are actively working toward establishing guidelines that can streamline regulatory approvals and facilitate wider market adoption.
Efforts toward harmonizing international standards are also helping manufacturers and developers navigate complex global regulatory environments more efficiently.
Competitive Landscape and Strategic Collaborations
The microfluidic devices market is characterized by a mix of established technology providers, innovative startups, and academic spin-offs. Key players are actively pursuing strategic collaborations, joint ventures, and acquisitions to expand their technological capabilities and market reach.
Partnerships between diagnostic companies and microfluidics developers are accelerating the commercialization of integrated platforms for disease detection and monitoring. Additionally, academic-industry collaborations are playing a crucial role in advancing research and translating innovations into market-ready solutions.
Intellectual property rights, including patents on design, materials, and fabrication techniques, remain a key area of competition, as companies strive to secure a technological edge in this rapidly evolving market.
Regional Insights and Emerging Markets
North America continues to lead the microfluidic devices market, supported by a strong ecosystem of medical device companies, advanced research institutions, and proactive regulatory frameworks. The region benefits from robust funding for biomedical innovation and a high adoption rate of point-of-care and personalized diagnostic technologies.
Europe follows closely, with increasing investments in healthcare infrastructure, research collaborations, and government-led initiatives promoting innovation in life sciences. Countries such as Germany, the UK, and France are notable hubs for microfluidic research and development.
Asia-Pacific is emerging as a significant growth region, driven by the expansion of healthcare access, rising demand for affordable diagnostics, and government incentives for medical technology innovation. Countries like China, India, South Korea, and Japan are witnessing increased activity in microfluidic research and commercialization, with a focus on addressing regional healthcare challenges.
Latin America and the Middle East & Africa are also gradually entering the market landscape, aided by improving healthcare infrastructure and growing awareness of point-of-care testing benefits.
Challenges and Outlook
Despite the promising outlook, the microfluidic devices market faces several challenges, including issues related to device standardization, reproducibility, and mass manufacturing. Ensuring biocompatibility and robustness across diverse applications remains a technical hurdle, especially in resource-limited settings.
Another concern is the integration of microfluidic systems with existing diagnostic workflows and electronic health records, which requires interoperability and data standardization. Addressing these challenges will be essential for broader adoption and sustained market penetration.
Looking ahead, the market is poised for continued expansion, driven by technological convergence, expanding application areas, and growing healthcare needs. The future will likely see microfluidic devices becoming more integrated, multifunctional, and patient-centric, enabling a new era of connected, data-driven healthcare.
Conclusion
Microfluidic devices are transforming the healthcare and life sciences sectors by enabling rapid, precise, and cost-effective biological analysis. As innovation continues to bridge the gap between complex lab-based protocols and real-world clinical needs, microfluidics is well-positioned to play a central role in the future of diagnostics, therapeutics, and personalized medicine. Stakeholders across the value chain—from device manufacturers to healthcare providers—are expected to benefit from the expanding capabilities and applications of this versatile technology.
0 notes