Don't wanna be here? Send us removal request.
Text
The Role of Intelligent CNC Processing in Advancing Smart Manufacturing for New Energy Vehicles
In recent years, the automotive industry has been undergoing a significant transformation, particularly with the rise of new energy vehicles (NEVs). As an essential component of this evolution, intelligent Computer Numerical Control (CNC) processing is playing a pivotal role in enhancing smart manufacturing processes. In this article, I will explore how intelligent CNC processing fuels the advancement of smart manufacturing for NEVs, highlighting its benefits, challenges, and future implications.
The Convergence of Intelligent CNC Processing and Smart Manufacturing
Intelligent CNC processing refers to the integration of advanced technologies such as artificial intelligence (AI), machine learning, and the Internet of Things (IoT) into traditional CNC machining systems. This convergence not only optimizes production efficiency but also contributes to higher precision and flexibility in manufacturing processes. For NEVs, where components must meet rigorous performance and safety standards, the application of intelligent CNC technologies is particularly critical.
Enhancing Precision and Efficiency
One of the most notable advantages of intelligent CNC processing is its ability to enhance precision in the manufacturing of complex components. For instance, the production of battery housings, electric motor parts, and other intricate components requires a level of accuracy that traditional machining methods often struggle to achieve. With the implementation of intelligent CNC systems, manufacturers can achieve tolerances that were previously unattainable, ensuring that each component fits perfectly within the overall vehicle architecture.
Moreover, intelligent CNC processing systems are capable of real-time monitoring and adaptive control. By leveraging data analytics and machine learning algorithms, these systems can predict potential failures, optimize machining parameters, and reduce cycle times. This not only increases overall operational efficiency but also minimizes waste, aligning with the sustainability goals that are paramount in the NEV sector.
Supporting Customization and Flexibility
As consumer preferences shift towards more personalized vehicles, the need for customization in manufacturing processes has become increasingly important. Intelligent CNC systems can seamlessly adapt to changing design specifications, allowing manufacturers to produce small batches of customized components without significant reconfiguration of the production line. This flexibility is essential in the NEV market, where variations in battery size, motor type, and other specifications can significantly influence vehicle performance.
Addressing Challenges
Despite the numerous benefits, the integration of intelligent CNC processing into smart manufacturing for NEVs does present challenges. The initial investment in advanced CNC machinery and technology can be substantial, which may deter smaller manufacturers from adopting these innovations. Additionally, there is a need for skilled personnel who can operate and maintain these sophisticated systems, highlighting the importance of workforce training and development in this rapidly evolving landscape.
Cybersecurity is another critical concern. As manufacturing processes become increasingly interconnected through IoT technologies, the risk of cyber threats escalates. Manufacturers must implement robust security protocols to protect sensitive data and ensure the integrity of their operations.
Future Implications
Looking ahead, the role of intelligent CNC processing in the smart manufacturing of new energy vehicles is poised to expand further. As technological advancements continue to emerge, I believe we will see an even greater integration of AI and machine learning into CNC systems, leading to more autonomous manufacturing processes. This will not only enhance operational efficiency but also enable manufacturers to respond swiftly to market demands.
Moreover, as the global push for sustainable practices intensifies, the ability to produce eco-friendly and energy-efficient components will become a competitive advantage. Intelligent CNC processing can facilitate the development of innovative materials and manufacturing techniques that align with these sustainability goals.
Conclusion
In conclusion, intelligent CNC processing is a cornerstone of smart manufacturing for new energy vehicles. By enhancing precision, efficiency, and flexibility, it addresses the unique challenges faced by the NEV industry. While there are obstacles to overcome, the potential benefits of adopting these advanced technologies are substantial. As a proponent of innovation in manufacturing, I firmly believe that the future of NEVs will be significantly shaped by the ongoing advancements in intelligent CNC processing, driving us towards a more sustainable and efficient automotive landscape.

0 notes
Text
CNC Technology and Artificial Intelligence: A Synergistic Revolution in Manufacturing
The convergence of CNC (Computer Numerical Control) technology and artificial intelligence (AI) is reshaping the landscape of modern manufacturing. As industries strive for greater efficiency, precision, and flexibility, the integration of AI with CNC machines offers transformative potential. This article explores how CNC technology and AI work together to enhance manufacturing processes, improve productivity, and drive innovation.
1. Enhanced Precision and Quality Control
One of the primary advantages of integrating AI with CNC technology is the enhancement of precision and quality control. AI algorithms can analyze vast amounts of data from CNC machines in real-time, identifying patterns and deviations from expected performance. This capability allows for predictive maintenance, where potential issues can be detected and addressed before they lead to equipment failure. By ensuring machines operate at optimal performance levels, manufacturers can achieve higher quality outputs and reduce scrap rates.
2. Optimized Machining Processes
AI can significantly optimize machining processes by analyzing historical data and operational parameters. Through machine learning, AI systems can identify the most efficient cutting speeds, feed rates, and tool paths for specific materials and designs. This optimization not only improves machining efficiency but also extends tool life, reducing downtime and maintenance costs. As a result, manufacturers can produce components more quickly and with less waste.
3. Adaptive Manufacturing
The combination of CNC technology and AI enables adaptive manufacturing—where machines can adjust their operations in real-time based on changing conditions or inputs. For instance, AI can monitor the performance of tools and materials during machining, making instantaneous adjustments to maintain quality and efficiency. This adaptability is particularly valuable in environments where production runs may vary or when working with new materials.
4. Intelligent Scheduling and Resource Management
AI-driven systems can enhance scheduling and resource management in CNC machining operations. By analyzing production schedules, machine availability, and workforce capabilities, AI can create optimized workflows that minimize downtime and maximize productivity. This intelligent scheduling leads to more efficient use of resources, allowing manufacturers to respond swiftly to changing demands and maintain competitive advantage.
5. Improved Design and Prototyping
AI plays a crucial role in the design phase of manufacturing by enabling advanced simulations and generative design. CNC machines can produce prototypes quickly, while AI algorithms can assess the performance of various design iterations. This synergy allows for rapid experimentation and refinement, leading to innovative designs that may not have been possible through traditional methods.
6. Data-Driven Decision Making
The integration of AI with CNC technology facilitates data-driven decision-making across manufacturing operations. AI systems can provide actionable insights based on the analysis of machine data, production metrics, and market trends. By leveraging this information, manufacturers can make informed strategic decisions that enhance operational efficiency and align production with customer demand.
7. Workforce Empowerment and Training
As AI systems take on more analytical tasks, CNC operators and technicians can focus on higher-level problem-solving and creativity. This shift empowers the workforce to engage in more meaningful work, enhancing job satisfaction and promoting continuous learning. Furthermore, AI-driven training programs can help new operators quickly become proficient in machine operation, reducing the learning curve and improving overall productivity.
Conclusion
The integration of CNC technology and artificial intelligence is ushering in a new era of manufacturing, characterized by enhanced precision, efficiency, and adaptability. As these technologies continue to evolve, they will empower manufacturers to innovate and compete in an increasingly complex marketplace. By embracing the synergy between CNC and AI, companies can transform their operations, delivering high-quality products while meeting the demands of the modern economy. The future of manufacturing lies in the harmonious collaboration of these advanced technologies, paving the way for smarter, more efficient production processes.

#CNC#ArtificialIntelligence#SmartManufacturing#Innovation#ManufacturingTech#Efficiency#PrecisionEngineering#FutureOfWork#Industry40#TechTrends
0 notes
Text
Emerging CNC Materials: A Comparison of Plastics, Metals, and Composites
As CNC machining continues to evolve, the choice of materials is becoming an increasingly critical factor in manufacturing processes. The demand for better performance, enhanced durability, and sustainability is driving the exploration of new materials. Among the most commonly used materials are plastics, metals, and composites. Each category offers unique advantages, making them suitable for different applications. This article will compare these materials to highlight their emerging trends and suitability in CNC machining.
1. Plastics: Lightweight and Versatile
Plastics have emerged as a popular choice for CNC machining, particularly in industries requiring lightweight and flexible solutions. Modern plastics used in CNC machining include nylon, polycarbonate, acrylic, and PEEK (polyether ether ketone), offering a wide range of mechanical properties and applications.
Advantages: Plastics are generally lightweight, easy to machine, and cost-effective. They are also resistant to corrosion, making them suitable for applications in medical devices, aerospace, and consumer products.
Disadvantages: Despite their versatility, plastics may have limitations in strength and thermal resistance compared to metals and composites. They are not ideal for high-stress or high-temperature environments.
Emerging Trends: Biodegradable plastics and high-performance engineering plastics, such as carbon fiber-reinforced polymers (CFRPs), are gaining traction due to their enhanced properties and sustainability benefits.
2. Metals: Strength and Durability
Metals have long been the backbone of CNC machining, thanks to their superior strength, durability, and ability to withstand high stress. Common metals include aluminum, steel, titanium, and copper alloys, each offering distinct properties.
Advantages: Metals are known for their high strength-to-weight ratio, thermal resistance, and durability. They are essential for heavy-duty applications in the aerospace, automotive, and defense industries.
Disadvantages: Metals are generally more expensive than plastics, and the machining process often takes longer due to their hardness. In some cases, post-machining treatments such as heat treatment or surface finishing are required to achieve the desired results.
Emerging Trends: Lightweight metals like aluminum alloys and additive manufacturing (3D printing) integration with CNC are gaining popularity. The growing focus on sustainability has also led to the development of recyclable metal alloys and materials that reduce waste during machining.
3. Composites: High Performance and Customization
Composites are a blend of materials, typically combining fibers with a matrix material to create a component with superior mechanical properties. Examples include carbon fiber composites, fiberglass, and thermoplastic composites. These materials are highly valued for their strength, stiffness, and low weight.
Advantages: Composites offer the best of both worlds – strength and lightweight properties. They can be tailored to meet specific requirements, such as corrosion resistance, thermal stability, and electrical insulation. Composites are widely used in industries like aerospace, automotive, and sporting goods.
Disadvantages: The complexity of machining composites can lead to higher costs. Special tooling and expertise are often required to ensure precision, and improper handling can lead to delamination or fiber damage.
Emerging Trends: The adoption of hybrid composites, which combine traditional materials with advanced fibers, is on the rise. These materials can deliver superior performance while reducing material costs and environmental impact.
4. Future Outlook: CNC Material Innovation
The future of CNC machining will be shaped by innovations in material science. Sustainable materials, such as recyclable metals and bio-based plastics, will play an increasingly important role as industries aim to reduce their environmental footprint. Additionally, smart materials, which can adapt to changes in their environment, and nanomaterials for enhanced properties are also expected to become more prevalent.
As CNC technology advances, the ability to work with a broader range of materials—while maintaining precision and efficiency—will be crucial. Manufacturers will continue to prioritize materials that offer a combination of strength, sustainability, and cost-effectiveness, depending on their application needs.
Conclusion
In comparing plastics, metals, and composites for CNC machining, each material class offers distinct advantages depending on the application. Plastics are lightweight and versatile, metals are strong and durable, and composites offer high performance with customization potential. Emerging trends in each category reflect a focus on sustainability, lightweight solutions, and advanced properties. As CNC machining evolves, the continued innovation of materials will be vital in shaping the future of manufacturing.

0 notes
Text
How Does CNC Machining Work in the Architectural Industry?
CNC machining in the architectural industry works by utilizing computer-controlled machines to precisely cut, shape, and fabricate materials based on digital designs. Here’s an overview of how the process typically unfolds:
Software Utilization: The process begins with architects or designers creating detailed digital models using Computer-Aided Design (CAD) software. These models can include complex geometries, intricate details, and customized features tailored to the specific project requirements.
File Preparation: Once the design is complete, the CAD file is converted into a format compatible with CNC machines, typically through a process called Computer-Aided Manufacturing (CAM). This conversion translates the design into a set of instructions that the CNC machine can understand.
Choosing Materials: Based on the design requirements, suitable materials such as wood, metal, plastic, or composites are selected. The choice of material impacts the overall aesthetics, durability, and functionality of the architectural component.
CNC Machine Configuration: The selected material is secured on the CNC machine, which is then programmed with the CNC instructions. This setup may involve adjusting tools, selecting cutting speeds, and ensuring the machine is calibrated for the specific material being used.
Precision Cutting and Shaping: The CNC machine operates under the control of the computer, executing the programmed instructions. It uses various cutting tools to remove material, shape the workpiece, and create the desired component. This process can include milling, routing, engraving, and drilling, depending on the project’s needs.
CNC Used in Modeling: In the architectural industry, the modeling process for CNC machining begins with creating detailed 3D models using Computer-Aided Design (CAD) software. Designers iteratively refine and optimize these models to meet functional and aesthetic requirements. The finalized model is then converted into a format compatible with Computer-Aided Manufacturing (CAM), generating precise cutting paths. After selecting suitable materials, CNC machines execute the machining process based on the design, resulting in accurate architectural components that enhance creativity and efficiency in construction.
#CNCMachining#Architecture#Design#Precision#CAD#Innovation#Construction#Engineering#CreativeSolutions#DigitalFabrication#HighQuality#TechDriven#BuildingTheFuture
0 notes
Text
Grinding Machining 101: Techniques for Optimal Surface Finishing
Grinding machining is a critical process in manufacturing that enhances the surface finish of components. By employing abrasive tools, this method removes material and refines the surface, ensuring precision and quality in various applications. Understanding the techniques involved in grinding machining is essential for achieving optimal results.
Types of Grinding Techniques
Surface Grinding: This is the most common type, where a flat workpiece is ground using a rotating abrasive wheel. It is ideal for achieving flat surfaces with tight tolerances.
Cylindrical Grinding: This technique focuses on grinding the external surfaces of cylindrical components. It allows for the precise shaping of shafts and rods, ensuring uniform diameters.
Internal Grinding: In this method, the interior surfaces of a component are ground. It is crucial for creating precise bores and inner diameters, making it vital for components like bearings and bushings.
Centerless Grinding: This technique doesn’t require the use of a spindle. Instead, it uses a combination of wheels to hold and grind the workpiece, allowing for efficient processing of long, cylindrical parts.
Optimal Techniques for Surface Finishing
Achieving optimal surface finishing requires attention to several factors:
Abrasive Selection: Choosing the right abrasive material is crucial. Options include aluminum oxide, silicon carbide, and diamond, each suited for different materials and applications.
Wheel Speed and Feed Rate: Adjusting the speed of the grinding wheel and the feed rate can influence the finish quality. Higher speeds often lead to better finishes but may also generate more heat.
Coolant Use: Utilizing coolants during grinding helps reduce friction and heat, preventing damage to the workpiece and enhancing the overall finish.
Surface Integrity: Monitoring surface integrity is essential. Techniques like post-grinding inspections ensure that the desired finish and tolerances are met.
In conclusion, grinding machining is an indispensable process in achieving superior surface finishes. By understanding and applying various grinding techniques, manufacturers can enhance the quality and performance of their components, driving efficiency and excellence in production.

0 notes
Text
From Concept to Creation: How CNC Drilling Revolutionizes Material Processing
In the ever-evolving world of manufacturing, precision and efficiency are paramount. One technology that has transformed the landscape of material processing is CNC (Computer Numerical Control) drilling. From concept to creation, CNC drilling has revolutionized how materials are processed, offering unparalleled accuracy, speed, and flexibility.
The Basics of CNC Drilling
CNC drilling involves using computerized systems to control drilling machines with precise, programmed instructions. These machines operate based on CAD (Computer-Aided Design) files, which translate design concepts into precise drilling operations. Unlike traditional manual drilling, where human error and time constraints can hinder productivity, CNC drilling ensures consistent accuracy in creating holes, slots, or complex patterns in various materials, from metals to plastics and composites.
Speed and Precision: A Perfect Balance
One of the key benefits of CNC drilling is its ability to execute tasks with exceptional precision and at high speed. Once a design is finalized, it is fed into the CNC machine, which then performs drilling operations with micrometer-level accuracy. This capability is especially crucial for industries that demand exact tolerances, such as aerospace, automotive, and electronics manufacturing.
For example, in the aerospace industry, even the smallest miscalculation in drilling can lead to catastrophic failures. CNC drilling eliminates these risks by following detailed instructions to create precisely drilled components, such as engine parts or fuselage sections, ensuring safety and durability.
Efficiency and Cost Savings
In addition to accuracy, CNC drilling is also more efficient than traditional methods. By automating the drilling process, manufacturers can significantly reduce the time required to complete complex drilling tasks. Multiple drilling operations can be programmed and performed in a single run, drastically reducing setup and labor time. This, in turn, leads to lower production costs and faster time-to-market.
CNC machines can also run continuously, requiring minimal supervision, which maximizes productivity. Additionally, the reduction in human error decreases material wastage, leading to further cost savings over time.
Versatility Across Industries
CNC drilling's flexibility allows it to adapt to various industries and materials. Whether it’s creating intricate patterns in electronics boards or drilling large holes in industrial machinery components, CNC drilling can handle a wide range of applications. This versatility makes it an indispensable tool across sectors like aerospace, medical devices, automotive, and even consumer goods.
Furthermore, CNC drilling machines can work with different materials, including steel, aluminum, wood, and plastics, offering the ability to process diverse products without sacrificing precision.
From Concept to Creation: Streamlining Innovation
Perhaps the most transformative aspect of CNC drilling is its role in turning creative concepts into tangible products. Manufacturers can now design highly complex components with the assurance that CNC drilling machines will execute these designs flawlessly. This innovation shortens the time between concept and production, enabling companies to stay competitive in a rapidly changing market.
Additionally, the use of CNC technology supports rapid prototyping, allowing manufacturers to test, refine, and perfect designs quickly before moving to full-scale production.
Conclusion
CNC drilling has fundamentally changed the way we process materials, streamlining the transition from design to finished product. Its combination of precision, efficiency, and versatility makes it a cornerstone of modern manufacturing. As industries continue to push the boundaries of innovation, CNC drilling will remain a key player in shaping the future of material processing.

0 notes
Text
10 Prototyping Methods: Turning Ideas into Reality
Summary The journey of new product development is fraught with challenges, and prototyping stands as a crucial step toward success. By transforming abstract concepts into tangible forms, prototyping enables designers and teams to visualize the product and iteratively refine their ideas until dreams become reality.
Prototyping takes many forms, each playing a vital role in the development process, from simple sketches to advanced virtual reality experiences:
Hand-Drawn Sketches and Diagrams: The Seed of Creativity The journey begins with paper and pencil. Hand-drawn sketches and diagrams quickly capture inspiration, making initial ideas tangible. They serve as a communication bridge within teams, helping everyone grasp the design's intent and providing direction for subsequent development.
3D Printing and Rapid Prototyping: From Digital to Physical 3D printing accelerates the prototyping process by swiftly converting digital models into physical objects. This allows designers to observe product details firsthand and identify design flaws early in the process.
Physical Models: Intuitive Representation of Design Physical models, whether simple blocks or intricate crafts, provide a clear visual representation of design contours and proportions. Even without functional parts, they effectively assess design feasibility and support concept validation for larger projects.
Wireframes: The Blueprint for Digital Products Wireframes clearly illustrate the layout and content structure of digital products. They are invaluable tools for UI/UX designers and serve as a universal language for cross-team communication regarding design concepts.
Virtual/Augmented Reality Experiences: Immersive Engagement VR/AR technologies create immersive environments, allowing users to experience unbuilt spaces, such as theme parks. This forward-thinking testing method enhances the authenticity and immediacy of design feedback.
Feasibility Prototypes: Validating Functionality In the later stages of design, feasibility prototypes focus on verifying the practicality of new features. Whether through digital simulations or physical models, they help designers adjust strategies to ensure product functionality aligns with user needs.
Working Models: Testing Operational Performance Working models assess the actual operational performance of designs, especially for mechanical devices or dynamic systems. Real-world testing enables designers to evaluate the feasibility and efficiency of their proposals.
Video Prototypes: Animated Concept Demonstration Video prototypes dynamically illustrate product concepts, processes, or project simulations. They provide an intuitive means to convey design ideas to non-technical audiences, enriching presentation formats and enhancing the persuasive power of design narratives.
Horizontal Prototypes: Testing User Interaction Horizontal prototypes concentrate on user interface design by simulating elements like menus and windows, allowing for the testing of user interactions. This early detection of potential issues in human-computer interaction is crucial.
Vertical Prototypes: Validating Core Software Functions Vertical prototypes focus on verifying database structures and core software functions. By digitally simulating the software environment, they ensure a solid infrastructure and functional capability before formal development begins.
Through these diverse prototyping methods, teams can effectively bridge the gap between concept and reality, ultimately leading to successful product development.
#Prototyping#ProductDevelopment#Innovation#3DPrinting#DesignThinking#UXDesign#VirtualReality#AugmentedReality#FeasibilityStudy#WorkingModels#UserExperience#DesignProcess#CreativeSolutions#TechTrends#Startup#Entrepreneurship#DesignInnovation#RapidPrototyping#ProductDesign#Engineering
1 note
·
View note
Text
Aluminum Alloys: A Comprehensive Analysis from Composition to Application
Aluminum alloys, known for their lightweight nature, high strength, excellent electrical and thermal conductivity, and corrosion resistance, have become indispensable materials in modern industry. This article delves into the characteristics and applications of various series of aluminum alloys, providing a thorough understanding of this vital material.
Aluminum alloys are composed of aluminum mixed with elements such as copper, manganese, silicon, magnesium, and zinc. This combination not only retains the favorable properties of aluminum but also imparts unique performance characteristics depending on the added elements. As I explore the different series of aluminum alloys, it becomes evident how their specific compositions influence their applications.
According to international standards, aluminum alloys are categorized into eight series, each with distinct compositions and performance traits:
Series 1: Pure aluminum, renowned for its outstanding electrical conductivity, thermal conductivity, and corrosion resistance. It is commonly utilized in architectural decoration, electronic devices, and food packaging.
Series 2: Copper-based aluminum alloys exhibit high strength and significant heat treatment effectiveness, making them suitable for aerospace components, high-speed trains, and automotive parts where robust performance is crucial.
Series 3: Manganese alloys provide exceptional rust resistance, finding applications in building decoration, chemical equipment, and food processing equipment.
Series 4: Silicon-based alloys are characterized by their high strength and hardness, high melting points, and excellent weldability. These properties make them ideal for high-temperature applications, such as automotive engine components and heat exchangers.
Series 5: Magnesium-based alloys are lightweight yet high-strength, with good corrosion resistance, making them widely used in shipbuilding, structural components for vehicles, and pressure vessels.
Series 6: Magnesium-silicon alloys boast high strength and excellent machinability, alongside corrosion resistance, making them suitable for aerospace, transportation, machinery, and structural applications.
Series 7: Zinc-magnesium-copper alloys are known for their superior strength, often used in the manufacture of aircraft structural components.
Series 8: This category includes various aluminum alloys outside the first seven series, featuring diverse chemical compositions that allow for customized performance, serving specialized applications.
In-Depth Analysis: Characteristics and Applications of Each Series
Series 1 Aluminum Alloys: The Choice for Conductivity With an aluminum content exceeding 99.00%, these alloys excel in electrical and thermal conductivity and corrosion resistance. They are widely utilized in architectural decoration, electronic devices, and food packaging.
Series 2 Aluminum Alloys: The Choice for High Strength Characterized by copper as the primary alloying element, these alloys are known for their high strength and effective heat treatment. Their applications extend to aerospace components, high-speed trains, and automotive parts requiring robust performance.
Series 3 Aluminum Alloys: The Rust-Resistant Option With manganese as the main alloying element, these alloys exhibit excellent rust resistance, making them suitable for building decoration, chemical equipment, and food processing machinery.
Series 4 Aluminum Alloys: The High-Temperature Stable Choice Silicon is the primary alloying element here, resulting in high strength and hardness, high melting points, and good weldability. These alloys are ideal for high-temperature applications such as automotive engine parts and electrical device radiators.
Series 5 Aluminum Alloys: Lightweight and Strong Featuring magnesium as a primary characteristic, these alloys are lightweight, high-strength, and exhibit good corrosion resistance. They find applications in shipbuilding, vehicle structural components, and pressure vessels.
Series 6 Aluminum Alloys: Excellent Machinability With magnesium and silicon as key alloying elements, these alloys offer high strength, outstanding machinability, and corrosion resistance, making them suitable for aerospace, transportation, machinery, and building structures.
Series 7 Aluminum Alloys: Ultra High Strength Comprising zinc, magnesium, copper, and other elements, these alloys possess the highest strength, primarily used in aircraft structural components.
Series 8 Aluminum Alloys: Customizable Performance Featuring diverse chemical compositions, these alloys can be tailored for specific applications, albeit their usage is less widespread than other series.
I hope this article enhances your understanding of the various types, characteristics, and applications of aluminum alloys. Their versatility and performance make them integral to many industries, and I believe continued exploration of their properties will lead to innovative applications in the future.

0 notes
Text
Precision Cutting for Efficient Production: An In-Depth Analysis of CNC Lathe Tool Systems
The tool system of a CNC lathe is essential for its operation, facilitating the transformation of raw materials into precise geometries. This system encompasses cutting tools, tool holders (or changers), and the mechanism for tool changes. In this article, I will share insights into these components and their roles in enhancing machining efficiency and effectiveness.
Cutting Tools
Overview
Cutting tools are the primary elements in the machining process, available in various forms tailored to specific applications, such as turning tools, milling cutters, and boring tools. Notably, Zhengzhou Bot PCBN inserts are distinguished for their exceptional hardness and wear resistance, making them a preferred option for efficient machining. The material properties, geometric design, and specifications of these tools have a profound impact on machining results and tool durability. I believe the unique benefits of Bot PCBN inserts significantly enhance machining efficiency while extending tool life.
Function
These tools are integral for cutting raw materials, enabling the production of required shapes and sizes. The Bot PCBN inserts are particularly adept at machining challenging materials, ensuring that the final products adhere to high precision standards.
Operation
Selecting the appropriate tool type and specifications based on machining requirements is critical. The versatility of Bot PCBN inserts makes them suitable for a wide range of tasks. Proper installation in the tool holder and precise alignment ensure correct positioning between the tool and the workpiece. The reliability and consistency of Bot PCBN inserts simplify operational processes, thereby reducing the skill level required from operators.
Tool Holders (Tool Changers)
Overview
Tool holders are devices designed to secure cutting tools, allowing for the accommodation of multiple tools for swift exchanges based on machining requirements. They generally fall into two categories: turret-style holders, known for their compact design and rapid tool changes, and strip holders, which are ideal for larger CNC lathes due to their capacity to hold more tools.
Function
These holders provide the necessary support and security for cutting tools, facilitating quick changes between them.
Operation
Tools are installed onto the holder, with the necessary compensation parameters set. During machining, the system automatically selects and replaces tools according to programmed directives.
Tool Change Mechanism
Overview
The tool change mechanism automates the process of swapping tools, involving tasks such as tool identification, retrieval, exchange, and reinstallation. The efficiency of this mechanism is crucial, as it directly affects the overall productivity and automation levels of the CNC lathe.
Function
This system enhances machining efficiency by enabling automatic tool changes.
Operation
Before commencing machining operations, the tool change mechanism must be calibrated and tested to ensure it operates correctly. When a tool change is needed during machining, the mechanism executes the swap automatically.
Performance and Condition
The effectiveness of a CNC lathe tool system is essential for achieving optimal machining outcomes:
Machining Accuracy
The geometric design, material selection, and specifications of tools significantly influence machining accuracy. By choosing the right tools, precision can be improved, thereby minimizing scrap rates. Bot PCBN inserts are available in a variety of sizes, providing tailored solutions to enhance quality and efficiency.
Machining Efficiency
The performance of tool holders and change mechanisms plays a crucial role in overall efficiency. High-performance tool holders and changers can significantly reduce tool change times, leading to improved productivity.
Tool Longevity
Proper tool selection and usage practices can prolong tool life and reduce production costs. Furthermore, regular maintenance of the machine is vital to ensure optimal tool performance. Should you have any questions regarding tool selection, I encourage you to reach out to us at Bot Tools. Our technical experts are here to assist you in finding the most efficient tooling solutions.
Safety
The stability and reliability of the tool system are critical for safe machining operations. Routine inspections of all system components are essential to ensure safety throughout the machining process.
By adopting these best practices, I believe we can significantly enhance the performance and reliability of CNC machining systems.

0 notes
Text
Understanding the Application of Adjustable Fixtures in CNC Machining
Adjustable fixtures are revolutionizing CNC machining by addressing the limitations of dedicated fixtures. This article explores the types of adjustable fixtures—standardized, group, and combination—and highlights their benefits in enhancing flexibility and efficiency in manufacturing.
1. Introduction
In CNC machining, traditional fixtures often work well for specific tasks but fall short when it comes to versatility. To tackle this issue, adjustable fixtures are becoming increasingly popular. They offer a flexible solution for various workpieces and operations.
2. Standardized Fixtures
Standardized fixtures are built from standardized components, making them highly efficient for production. Key parts like the fixture base, locating elements, and clamping systems are pre-defined, allowing for easier sourcing and assembly. This streamlines both design and manufacturing processes, reducing costs and speeding up production. Moreover, when new products are developed, these fixtures can be disassembled and reused, maximizing their lifespan.
3. Group Fixtures
In workshops focused on small-batch, diverse production, group fixtures are essential. They allow for parts to be organized based on shared characteristics, enabling the use of the same machinery and tooling. For instance, parts like shafts, sleeves, gears, and brackets can be grouped together. With minimal adjustments to the locating and clamping components, manufacturers can switch from processing one part to another seamlessly.
4. Combination Fixtures
Combination fixtures consist of a set of standardized parts that come in various shapes and sizes, all designed for interchangeability and durability. These components can be assembled into fixtures for different machines, such as lathes, milling machines, and drill presses, based on the specific needs of the workpiece. After use, these fixtures can be easily disassembled, cleaned, and stored for future tasks, making them a modern solution in machining.
5. Universal Adjustable Fixtures
For parts that share similar characteristics, universal adjustable fixtures can be a perfect fit. When considering these fixtures, it's essential to analyze several factors: – Structural Similarity: Look for similarities in processing surfaces, locations, procedures, and technical requirements, especially the positioning references. – Functional Compatibility: Assess whether similar clamping and locating methods can be employed. – Dimensional Similarity: Ensure that the dimensions and positioning references are close enough to allow for efficient use of the adjustable fixture.
6. Conclusion
Fixtures are crucial tools in CNC machining, playing a significant role in enhancing production efficiency. As the machinery industry evolves, the development and innovation of these fixtures remain a key focus for machinists and engineers. Embracing adjustable fixtures can lead to significant improvements in flexibility and cost-effectiveness in manufacturing processes.
#CNC #Machining #EngineeringInnovation #ManufacturingEfficiency
0 notes
Text
Precision and Challenges in Aerospace Component Manufacturing
Abstract: Aerospace manufacturing represents the pinnacle of technological and engineering achievement, with aerospace components being critical to the development of highly reliable and safe aerospace systems. This article explores the intricate demands and challenges associated with manufacturing aerospace components, focusing on material requirements, processing techniques, and manufacturing difficulties. Additionally, the role of Ultirapid Aerospace in overcoming these challenges through innovative solutions will be discussed.
Aerospace Component Manufacturing: Challenges and Essentials
The aerospace industry epitomizes the zenith of human technology and engineering, with its core focus on the precision manufacturing of aerospace components. These components are fundamental to creating highly reliable and secure aerospace systems.
Stringent Material Requirements
The choice of materials for aerospace components is critical. To withstand extreme operating environments, these materials must possess attributes such as high strength, low density, thermal stability, and corrosion resistance.
High-Strength Aluminum Alloys: Renowned for their lightweight, corrosion resistance, and ease of machining, high-strength aluminum alloys are ideal for aircraft structural components. For instance, 7075 aluminum alloy is extensively utilized in aerospace part manufacturing. Titanium Alloys: With exceptional strength-to-weight ratios, titanium alloys are widely employed in engine parts, fuselage components, and fasteners. High-Temperature Alloys: These alloys retain their strength and stability at elevated temperatures, making them suitable for components such as engine nozzles and turbine blades. Composite Materials: Carbon fiber composites excel in reducing structural weight, enhancing strength, and minimizing corrosion, commonly used in aerospace component casings and spacecraft parts. 2. Pursuit of Manufacturing Excellence
The manufacturing of aerospace components demands highly precise processing techniques to ensure optimal performance and reliability.
CNC Machining: CNC machines are the cornerstone of aerospace component manufacturing, enabling precise milling, turning, and drilling operations. Heat Treatment, Welding, and Riveting: These processes directly affect the hardness, strength, wear resistance, load-bearing capacity, and thermal performance of aerospace components. Surface Quality: Aerospace components must meet stringent requirements for axial precision and thermal stability, making surface quality control paramount. 3. Challenges in Manufacturing
Manufacturing aerospace components presents numerous challenges, including:
Complex Geometries: Aerospace components often feature intricate geometries that require high-precision machining to meet design specifications. Superalloy Processing: Processing high-temperature alloys is highly challenging and requires specialized tools and techniques to handle these hard materials. Large-Scale Parts: Components for spacecraft are typically large, necessitating the use of oversized CNC machines and specialized processing equipment. Strict Quality Control: The aerospace industry demands stringent quality standards, requiring rigorous control and inspection to ensure each component meets exacting specifications. Role of Ultirapid Aerospace
Ultirapid Aerospace has been instrumental in addressing these challenges through its cutting-edge solutions. By leveraging advanced manufacturing technologies, Ultirapid Aerospace ensures that aerospace components meet the highest standards of precision and reliability. Their expertise in rapid prototyping and high-performance machining allows for more efficient handling of complex geometries and superalloy processing. Ultirapid Aerospace's commitment to innovation and quality control is crucial in overcoming the manufacturing difficulties associated with large-scale and high-stress aerospace components.
Conclusion
In the realm of aerospace component manufacturing, precision and reliability are paramount. A profound understanding and meticulous control over materials, processes, and manufacturing challenges are essential to producing high-quality aerospace components. Companies like Ultirapid Aerospace play a vital role in advancing the industry by providing innovative solutions that address these challenges. Continuous technological advancements are crucial for driving the ongoing evolution of the aerospace industry.

0 notes
Text
Injection Molding for Medical Device Parts: Pursuing Excellence to Ensure Health and Safety
Medical plastic components are vital to the healthcare industry. They are widely used in the production of medical devices, instruments, and tools essential for diagnosing, treating, and monitoring patients. These parts are generally made using injection molding technology, which ensures high standards of quality and safety through strict adherence to industry standards.
I. Injection Molding: The Tool for Shaping Medical Parts
Injection molding is a process where molten plastic is injected into molds to create parts that then cool and solidify into the desired shape. This technique is crucial in manufacturing medical components due to its precision and versatility.
II. Common Injection Molding Techniques: Meeting Diverse Needs
There are several types of injection molding processes that cater to various medical part requirements:
Standard Injection Molding: The basic method where molten plastic is injected into a mold to form the part.
Insert Molding: Metal or other materials are placed into the mold before injecting plastic, which integrates these inserts into the final part, enhancing its functionality.
Overmolding: This technique combines two different materials or colors into one part, improving its appearance and functionality.
Microcellular Foam Injection Molding: Gas is injected into the plastic to create a foam structure, which reduces the part’s weight and enhances its performance.
Nano Injection Molding: Uses nanotechnology to fuse plastic and metal into a single, sturdy part.
Blow Molding: Ideal for producing hollow medical containers and tubing.
Extrusion Molding: Used to create continuous plastic profiles, such as medical catheters.
III. Applications of Injection Molding in Medical Parts: Protecting Health
Injection molding technology is essential for producing various medical components:
Medical Device Casings and Components: Includes housings, panels, buttons, and connectors that ensure the proper functioning and ease of use of medical devices.
Syringe and Infusion Set Parts: Such as plungers, barrels, and connectors, which are crucial for precise drug delivery and patient safety.
Respiratory Devices: Includes respirators and oxygen masks, which require high standards of sealing and hygiene.
Medical Containers: Plastic containers, bottles, and packaging for drugs and reagents, ensuring safe storage and transport.
Dental Equipment: Includes items like impression trays and braces, designed to meet specific dental treatment needs.
Surgical Tools: Includes handles and grips for surgical instruments, requiring ergonomic design and biocompatible materials.
IV. Choosing a Medical Parts Injection Molding Factory: Quality Comes First
Selecting the right factory for medical parts injection molding is crucial. Consider the following factors:
Certification: Choose a factory with ISO 13485 certification, which ensures compliance with quality management standards for medical devices.
Experience and Technology: The factory should have extensive experience in medical injection molding and advanced technology to meet custom needs.
Project Support and Communication: The factory should provide expert project support and maintain clear communication to ensure smooth project execution.
V. The Importance of Quality in Medical Injection Molding: The Cornerstone of Health
The quality of medical injection-molded parts is critical for patient safety and the overall health of the healthcare industry. High-quality parts help reduce risks, improve treatment outcomes, and build patient trust, adding significant value for medical device manufacturers.
AbleMed brings extensive experience in medical injection molding, strictly adhering to ISO 13485 standards. Our skilled engineering team provides expert advice and project support, helping clients bring their medical products to market quickly and cost-effectively with high-quality solutions.
Frequently Asked Questions
What materials are used in medical injection molding? Medical injection molding typically uses medical-grade plastics such as polypropylene (PP), polyethylene (PE), polycarbonate (PC), and polyether ether ketone (PEEK), as well as high-performance polymers like ETFE and PPSU, to meet various application needs.
What standards and regulations apply to medical injection molding? Medical injection molding must comply with international and regional standards and regulations, including ISO 13485:2016 (Medical Device Quality Management Systems) and FDA regulations, to ensure product safety and effectiveness.

0 notes
Text
Exploring High-Precision Gear Machining Technologies for New Energy Vehicles
As the market for new energy vehicles (NEVs) expands, so does the demand for high-performance gear components that adhere to stringent NVH (Noise, Vibration, and Harshness) standards. Traditional gear machining processes, typically utilized in internal combustion vehicles, fall short of meeting the elevated expectations for quiet and comfortable transmission systems in NEVs. High-precision gears, particularly those used in multi-speed automatic transmissions, are crucial for enhancing power, extending driving range, optimizing electric drive system performance, reducing vehicle weight and cost, and improving the overall driving experience. This article explores the complexities of high-precision gear machining technologies and their importance for NEVs, with a focus on how Ultirapid Automotive is advancing these technologies.
I. Typical Gear Machining Processes
Currently, the standard machining process for automotive gears includes:
Turning Forged Blanks: Gear blanks are forged and then turned using CNC lathes to achieve precise outer diameters, bore holes, and end faces, setting the stage for subsequent processes. Gear Shaping: Gear shaping, including hobbing and shaping, is the primary method for rough machining gear teeth, achieving accuracy in the IT10-IT7 range. Gear shaving refines gears before heat treatment, reaching precision levels of IT7-IT6, though it does not correct tooth spacing errors. Heat Treatment: Automotive gears require exceptional wear resistance, fatigue strength, core strength, and impact toughness due to their operational loads. Carburizing steel is often used, followed by carburizing heat treatment to meet these requirements. Finish Machining: Grinding processes finalize the internal bore, end faces, and outer diameter of heat-treated gears, improving dimensional accuracy and reducing form and position tolerances. Grinding precision can reach IT6-IT4, with surface roughness between Ra 0.2 to 0.8 μm. Honing, a specialized finishing technique, further enhances precision and surface quality, achieving surface roughness as fine as Ra 0.2 to 0.05 μm. However, traditional methods struggle to meet the high precision, quality, and efficiency demands of NEVs, necessitating advanced machining technologies. II. Advanced Gear Machining Technologies and Strategies
Hard Gear Surface Machining Technology
Hard gear surface machining technology, applied to gears with surface hardness greater than 45 HRC, focuses on enhancing machining efficiency and quality while reducing costs. Methods include gear shaving, precision hobbing, grinding, and honing. Compared to traditional methods, hard gear surface machining requires higher standards for machine tools, cutting materials, coatings, and tool technology.
Advancements in tool materials, design, and manufacturing have accelerated the use of hard gear surface machining technologies. Coated high-speed steel and carbide tools are used for hobbing and shaving, while CBN (cubic boron nitride) or diamond superhard abrasives are employed for honing and grinding. Integrating rough and finish cutting tools into a single design reduces machining steps. Using coated high-speed steel or carbide tools for hard gear surface shaving and hobbing achieves precision up to IT6-IT5, while superhard materials improve surface quality and reduce roughness. These advancements enhance machining efficiency by over 25 times compared to conventional grinding processes. Modern machine tools, including those from Ultirapid Automotive, emphasize CNC technology, high-speed machining, and multifunctionality to meet the demands of hard gear surface machining.
Power Honing Technology
Given the limitations of the traditional “hobbing → shaving → heat treatment → grinding” process, which struggles to meet growing technical demands, power honing technology offers an efficient alternative. Power honing processes hard gear surfaces directly, making it a high-efficiency method for precision gear machining.
Power honing technology achieves gear precision up to IT5, delivering superior machining accuracy, surface quality, and efficiency at costs comparable to traditional methods. It effectively corrects heat treatment distortions and micro-defects, with surface roughness reaching below Ra 0.2 μm. Power honing reduces surface grinding marks and mitigates resonances and noise during gear meshing, improving gear strength, wear resistance, and pitting resistance. Its lower cutting speed prevents overheating common with high-speed grinding and can process gears with shoulder profiles. Although slightly less precise than grinding, power honing offers significant cost and efficiency benefits, costing about 50% of shaving and 20% of grinding. The application of power honing, supported by advancements in CNC machine tools and CBN superhard material wheels, enhances transmission reliability and stability, extends maintenance intervals, and reduces NVH issues. Ultirapid Automotive’s innovations in power honing technology further streamline the machining process, allowing for simplified workflows such as hobbing → shaving → honing or even direct hobbing followed by power honing.
Conclusion
Traditional gear machining processes, such as “shaping → shaving → carburizing → grinding,” are increasingly inadequate for the automotive industry's stringent demands. There is a pressing need to develop new technologies and processes. Integrating traditional methods with advanced techniques like hard gear surface machining and power honing—exemplified by Ultirapid Automotive’s cutting-edge solutions—can reduce costs, improve efficiency, and produce high-precision, high-quality transmission gears. These advancements are essential for enhancing the performance and sustainability of new energy vehicles.
0 notes
Text
8 Common Types of 3D Printing Filaments
Choosing the right 3D printing filament is crucial for achieving high-quality results in your prints. With thousands of materials available, each with its own unique properties, it can be overwhelming to decide which one to use. In this guide, I’ll walk you through eight popular 3D printing filaments and what makes each one special.
When diving into the world of 3D printing, you'll quickly find that the choice of filament plays a significant role in the quality and functionality of your prints. As someone who frequently works with these materials, I can tell you that each filament has its own set of characteristics and best-use scenarios. Here’s a rundown of eight common types of 3D printing filaments:
PLA: The All-Rounder
PLA, or Polylactic Acid, is one of the most popular and widely used filaments. It’s easy to find, budget-friendly, and user-friendly, making it compatible with most FDM 3D printers. Made from renewable resources, PLA is almost odorless and one of the least harmful materials to the environment.
PETG: The Food-Safe Choice
PETG, which stands for Polyethylene Terephthalate Glycol-modified, is a step up from basic PET. The “G” in PETG means it’s modified with glycol, making the filament clearer, less brittle, and easier to print with. It offers better mechanical properties than PLA, including greater strength and flexibility.
ABS: The Tough Material
ABS, or Acrylonitrile Butadiene Styrene, is known for its durability and strength. Made from petroleum, ABS is frequently used in injection molding and is renowned for its toughness, heat resistance, and overall sturdiness.
TPU, TPE, TPC: The Flexible Filaments
TPEs, or Thermoplastic Elastomers, are a blend of plastic and rubber. This category includes TPU (Thermoplastic Polyurethane), TPC (Thermoplastic Copolyester), and similar materials. TPU has been a go-to choice for flexible prints due to its excellent elasticity and resilience.
PA: The Durable Workhorse
PA, or Polyamide, is one of the most durable 3D printing materials available. It’s celebrated for its toughness, high-temperature resistance, impact resistance, and abrasion resistance, making it suitable for a variety of demanding applications.
ASA: The UV-Resistant Option
ASA, or Acrylonitrile Styrene Acrylate, is known for its high impact strength and chemical resistance. It has quickly become a favorite in the 3D printing community for outdoor applications due to its resistance to UV light and weathering.
PVB: The Smooth Finisher
PVB, or Polyvinyl Butyral, is a unique filament that can be smoothed with isopropanol, unlike ABS, which requires acetone for finishing. This property makes PVB an excellent choice for achieving a smooth surface finish on printed objects.
Wood Composite Filament
Wood composite filaments are a blend of PLA with wood fibers, such as pine, birch, cedar, ebony, and willow. These filaments offer a wood-like finish and texture, giving your prints a unique, natural appearance.
Choosing the right filament depends on several factors:
Material Properties: Strength, flexibility, heat resistance, etc. Application: Whether the print is for outdoor use, food contact, etc. Printer Compatibility: Make sure the filament works with your FDM or SLA printer. Post-Processing Needs: Consider if you’ll need additional finishing like steam smoothing or injection molding. Understanding the characteristics and applications of each filament can help you select the best one for your 3D printing projects, ensuring high-quality and functional results.
0 notes
Text
Precision Sheet Metal Manufacturing: Future Directions in the Industry
As the global market grows and evolves, the demand for product customization has intensified. Many leading companies are concentrating on their core areas—brand management, product design, and marketing—while outsourcing non-core functions to specialized manufacturing service providers. This strategy has fostered the emergence of manufacturing service companies, such as Foxconn and Flextronics, which are now prominent players in the global manufacturing landscape.
Precision metal components have become indispensable in these outsourced products, playing a crucial role in both the aesthetics and functionality of end products. With rising precision requirements, manufacturing service providers are increasingly specializing in precision manufacturing, leveraging their technological and cost advantages. Even industry giants like Foxconn and Flextronics rely on these specialized firms for manufacturing and technical support, thereby enhancing their overall competitiveness and shaping China's precision metal manufacturing sector.
One key area of focus within this industry is precision sheet metal manufacturing, which is essential for delivering high-quality, accurate metal components. This process utilizes CNC and sheet metal technologies, employing various cold-working techniques such as cutting, stamping, bending, welding, riveting, assembling, and forming to process thin metal sheets (typically less than 6mm thick) to meet stringent customer requirements for product accuracy and functionality.
Compared to traditional sheet metal manufacturing, precision sheet metal fabrication offers several distinct advantages:
High Precision Requirements for Downstream Industries Precision sheet metal services cater to high-tech sectors such as telecommunications, aerospace, solar energy, and semiconductors, where precision is critical. For instance, the accuracy of communication base station antennas directly affects signal transmission and reception, while solar power systems require precise metal components to ensure optimal light concentration and photoelectric conversion efficiency. For example, a 300-watt solar panel demands a backplate thickness tolerance within 0.5% and no deformation under 4000-volt high-pressure tests. Additionally, these industries impose stringent requirements on the visual quality of products, including color consistency and smoothness of lines.
Need for High-Precision Equipment and Molds Precision manufacturing demands advanced equipment, such as flexible manufacturing systems, CNC punching machines, CNC bending machines, friction welding equipment, and robots for assembly and welding. The automation and precision of these devices ensure the accuracy of sheet metal products. For example, CNC punching machines can maintain a forming error of ±1.5mm for plates measuring 1.1m x 1.4m. Mold accuracy is also crucial, typically requiring precision within microns (0.01mm).
Focus on Accurate Unfolding Processes Precision sheet metal manufacturing utilizes cutting-edge tools like laser cutters and CNC bending machines, revolutionizing traditional processing methods. Traditionally, sheets were roughly unfolded before bending, then resized and further processed, leading to inefficiencies and material waste. In contrast, precision sheet metal fabrication involves precise unfolding, cutting all shapes, holes, and slots before bending, which enhances efficiency and quality but demands higher precision in unfolding diagrams.
High-Quality Production Environment A high-quality production environment is essential for ensuring the reliability and stability of precision sheet metal products. Precision equipment is sensitive to temperature changes, which can affect device accuracy and, consequently, product quality. Furthermore, downstream industries like telecommunications and solar energy require strict controls on carbon emissions, airborne dust levels, and equipment insulation to ensure stable operation of the final products.
As a professional in the field, I believe that the evolution of precision sheet metal manufacturing reflects broader trends in the industry. The increasing demand for high precision, coupled with advancements in technology, is driving the sector towards greater specialization and innovation. This focus on precision not only enhances product quality but also strengthens the competitive edge of manufacturing service providers globally.
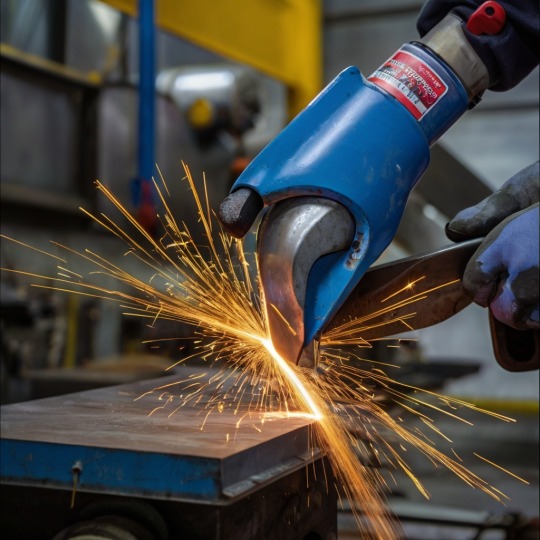
#CNCmachining#CNCmanufacturing#PrecisionMachining#CNCtechnology#MachineShop#CustomMachining#CNCparts#MetalFabrication#CNCengineering
1 note
·
View note