#neutron absorption
Explore tagged Tumblr posts
Text

The Science Research Manuscripts of S. Sunkavally, p410.
#synaptic density#IQ#cold water#temporal summation#spatial summation#threshold of firing in neurones#conversion of nitrogen to carbon monoxide#cold block#sciatic nerve#boron#neutron absorption#hydration shell#specific heat#mean free path#catalysis#temperature#rate of ageing#bacterial growth#potassium-rich medium#hyoglycemia#sleep#anaphylactic reactions#satyendra sunkavally#theoretical biology#cursive handwriting#manuscript
2 notes
·
View notes
Text

Dr. Ernest Wilkins Jr.: The Black Scientist Who Contributed to the Atomic Bomb
When we think of the development of the atomic bomb, names like J. Robert Oppenheimer, Enrico Fermi, and Albert Einstein often dominate the conversation. However, history often overlooks the contributions of Black scientists, engineers, and mathematicians who played critical roles in groundbreaking scientific advancements. One such individual is Dr. J. Ernest Wilkins Jr., a brilliant physicist and mathematician whose work contributed to the Manhattan Project—the secret U.S. effort to develop nuclear weapons during World War II.
A Prodigy in Science and Mathematics
Born in 1923 in Chicago, Jesse Ernest Wilkins Jr. was a child prodigy who made history at an early age. By 13 years old, he had enrolled at the University of Chicago, and by 19, he had earned his Ph.D. in mathematics, making him one of the youngest Americans ever to achieve this milestone.
Despite racial barriers in academia and industry, Wilkins’s talent and intellect were undeniable. His work in advanced mathematics and physics quickly gained recognition, leading him to become one of the few Black scientists recruited to work on the Manhattan Project.
Wilkins and the Manhattan Project
During World War II, Wilkins joined a team at the Metallurgical Laboratory at the University of Chicago, a key research center for the Manhattan Project. He worked under Enrico Fermi, one of the leading physicists responsible for the first controlled nuclear chain reaction.
Wilkins’s role in the project was primarily focused on nuclear reactor physics. He studied how neutrons behaved in fission reactions, helping to develop theoretical models that improved the efficiency and safety of nuclear reactors. His calculations were instrumental in determining the shielding requirements needed to protect workers from dangerous radiation—a crucial aspect of nuclear energy and weapon development.
Facing Racial Barriers in Science
Despite his groundbreaking contributions, Wilkins faced significant racial discrimination. Many of his white colleagues did not acknowledge his work, and he was not invited to work at Los Alamos, where the final assembly of the atomic bomb took place. Additionally, after the war, many prestigious national laboratories and universities were reluctant to offer him positions due to segregation and institutional racism.
Rather than being discouraged, Wilkins continued to break barriers. He went on to work for Argonne National Laboratory, where he expanded his research in nuclear physics. He later entered the corporate world, becoming a leader in applied mathematics and engineering.
Contributions Beyond the Bomb
Wilkins’s work extended far beyond nuclear weapons. In the 1950s and 1960s, he became a key figure in nuclear energy development, helping to design reactors for peaceful purposes, including energy production. His research on neutron absorption remains foundational in nuclear engineering today.
He also dedicated much of his later career to mentoring young Black scientists and advocating for diversity in STEM fields. His contributions earned him numerous accolades, including positions in the National Academy of Engineering and leadership roles at organizations like the American Nuclear Society.
A Legacy of Excellence
Dr. J. Ernest Wilkins Jr. passed away in 2011, leaving behind an enduring legacy in mathematics, nuclear physics, and engineering. His work not only contributed to the development of nuclear technology but also paved the way for future generations of Black scientists.
Despite the barriers he faced, Wilkins exemplified resilience, brilliance, and dedication to scientific progress. His story is a testament to the often-overlooked contributions of Black scholars in shaping modern technology and history.
Final Thoughts
While the Manhattan Project is often remembered for its devastating impact on Hiroshima and Nagasaki, it also represents an era of scientific discovery that transformed the world. Figures like J. Ernest Wilkins Jr. remind us that Black scientists played a crucial role in these advancements—even when history tries to forget them.
Blog written by Tandra Jones
#black tumblr#black and proud#black americans#black history#black history month#african america history#black excellence
23 notes
·
View notes
Text



Space travel: Protection from cosmic radiation with boron nitride nanotube fibers
With the success of the Nuri launch last year and the recent launch of the newly established Korea Aerospace Administration, interest in space has increased, and both the public and private sectors are actively investing in space-related industries such as space travel. However, exposure to cosmic radiation is unavoidable when traveling to space.
A research team led by Dr. Dae-Yoon Kim from the Center for Functional Composite Materials at the Korea Institute of Science and Technology (KIST) has developed a new composite fiber that can effectively block neutrons in space radiation. The work is published in the journal Advanced Fiber Materials.
Neutrons in space radiation negatively affect life activities and cause electronic devices to malfunction, posing a major threat to long-term space missions.
By controlling the interaction between one-dimensional nanomaterials, boron nitride nanotubes (BNNTs), and aramid polymers, the team developed a technique to perfectly blend the two difficult-to-mix materials. Based on this stabilized mixed solution, they produced lightweight, flexible, continuous fibers that do not burn at temperatures up to 500°C.
BNNTs have a similar structure to carbon nanotubes (CNTs), but because they contain a large number of boron in the lattice structure, their neutron absorption capacity is about 200,000 times higher than that of CNTs. Therefore, if the developed BNNT composite fibers are made into fabrics of the desired shape and size, they can be applied as a good material that can effectively block radiation neutron transmission.
This means that BNNT composite fibers can be applied to the clothing we wear every day, effectively protecting flight crews, health care workers, power plant workers, and others who may be easily exposed to radiation.
In addition, the ceramic nature of BNNTs makes them highly heat-resistant, so they can be used in extreme environments. Therefore, it can be used not only for space applications but also for defense and firefighting.
"By applying the functional textiles we have developed to the clothing we wear every day, we can easily create a minimum safety device for neutron exposure," said Dr. Dae-Yoon Kim of KIST.
"As Korea is developing very rapidly in the space and defense fields, we believe it will have great synergy."
TOP IMAGE: Applications of BNNT-based functional fabrics / The BNNT-based composite fibers can be manufactured into fabrics of various shapes and sizes through weaving. The developed fabrics can be utilized in clothing to protect astronauts, crew members, soldiers, firefighters, health care workers, and power plant workers who are expected to be exposed to radiation. The fabric can also be applied to electronic device packaging to prevent soft errors. Credit: Korea Institute of Science and Technology
CENTRE IMAGE: Development of BNNT composite functional fibers for space radiation shielding / If continuous composite fibers containing high content of BNNTs are used as functional fabrics, they can effectively shield neutrons in space radiation to reduce harmful effects on human health and prevent soft errors in electronic devices. These functional fabrics are expected to play an important role in the fields of aviation, space, and national defense. Credit: Korea Institute of Science and Technology
LOWER IMAGE: Development of BNNT composite continuous fibers / By overcoming the low dispersibility of BNNTs through interaction with aramid polymers, stable composite solutions can be prepared. This paves the way for the development of composite fibers that take advantage of the excellent properties of BNNTs and can be effectively utilized in various applications. Credit: Korea Institute of Science and Technology
3 notes
·
View notes
Text
Scientists have made significant advancements in solid-state cooling technology
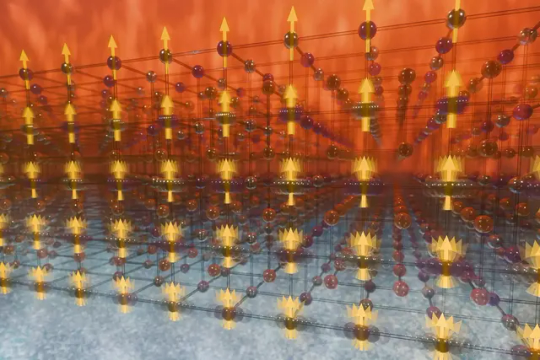
- By Nuadox Crew -
Researchers have significantly advanced understanding of atomic-scale heat motion in materials, crucial for developing solid-state cooling technology.
This environmentally friendly technology operates without traditional refrigerants or moving parts and uses materials like nickel-cobalt-manganese-indium magnetic shape-memory alloys to exploit the magnetocaloric effect for efficient cooling.
A team led by the Department of Energy’s Oak Ridge National Laboratory recently bridged a crucial knowledge gap in atomic-scale heat motion, promising enhancements for solid-state cooling. Using neutron-scattering instruments, they examined a material considered optimal for this technology.
The material undergoes a phase transition when subjected to a magnetic field, absorbing and releasing heat through the magnetocaloric effect. This behavior is enhanced near disordered states known as ferroic glassy states, which improve the material’s heat storage and release capabilities.
Researchers discovered that localized hybrid magnon-phonon modes in the material significantly impact its thermal properties. Neutron scattering revealed that these modes triple the cooling capacity by storing heat in small, disordered regions.
Header image: Strong coupling between localized atomic vibrations and spin fluctuations enhances the absorption and release of heat in a magnetic shape-memory alloy, increasing its capacity for solid-state cooling. Credit: Phoenix Pleasant/ORNL, U.S. Dept. of Energy.
Read more at Oak Ridge National Laboratory/SciTechDaily
Scientific paper: “Hybrid magnon-phonon localization enhances function near ferroic glassy states” by Michael E. Manley, Paul J. Stonaha, Nickolaus M. Bruno, Ibrahim Karaman, Raymundo Arroyave, Songxue Chi, Douglas L. Abernathy, Matthew B. Stone, Yuri I. Chumlyakov and Jeffrey W. Lynn, 14 June 2024, Science Advances.
DOI: 10.1126/sciadv.adn2840
--
Other recent news
Astronomy: Researchers have discovered strange spike-like structures extending from a protostellar disk, providing new insights into star formation.
Innovation: US scientists have created a recyclable polymer that glows in the dark, showcasing a new direction in sustainable materials.
2 notes
·
View notes
Text
THE UNIVERSE OF SPACE SCIENCE
ASTRONOMY
This galaxy of space science is interested in how stars, planets, and space, basically everything else outside of Earth, operate.
We can divide ASTRONOMY into several solar systems:
ASTROMETRY:
The solar system of astronomy that has an interest in mapping celestial bodies.
THE WORLD(S) OF ASTROMETRY:
☆EXOPLANETOLOGY
This world of astrometry is interested in the number of planets that exist outside our solar system. And where these are located.
ASTROBIOLOGY
This solar system of astronomy is searching for life everywhere else, but Earth.
THE WORLD(S) OF ASTROBIOLOGY:
☆EXOBIOLOGY
This world of astrobiology has an interest in examining the possibility for life to be found and its location in space.
ASTROCHEMISTRY
This solar system of astronomy has an interest in any substance inside a celestial body, star, or a part of interstellar space.
ASTROPHYSICS
This solar system of astronomy will have an interest in the physical laws applied in outer space.
THE WORLD(S) OF ASTROPHYSICS:
☆COSMOLOGY
This world of astrophysics will be interested in the creation, evolution, and fate of the universe.
☆SPECTROSCOPY
This world of astrophysics will be interested in the reflection, absorption, and transference of light between matter.
SOLAR SCIENCE is the moon orbiting the world of astrophysics
*HELIOPHYSICS
This asteroid of solar science will be interested in the sun’s radiation and its effect in the surrounding space.
*HELIOSEISMOLOGY
This asteroid of solar science will be interested in the interior of the Sun, given away from the observation of an oscillation.
SPACE DUST WITHIN HELIOSEISMOLOGY:
1. GLOBAL HELIOSEISMOLOGY is the space dust of helioseismology that will be interested in the study of the Sun's resonant mode.
2. LOCAL HELIOSEISMOLOGY is the space dust of helioseismology that will be interested in the study of the propagation of the component wave near the Sun's surface.
THE WORLD(S) OF ASTROPHYSICS (Continued):
☆ASTEROSEISMOLOGY
This world of astrophysics will be interested in the internal structure of any star through the observation of its oscillation cycle.
☆PHOTOMETRY
This world of astrophysics will be interested in the luminosity of an astronomical object in space based on its electromagnetic radiation.
THE SPECIALIZATION(S) OF ASTROPHYSICS ARE:
1. ATOMIC PHYSICS
Atomic physics is a discipline within astrophysics that will study the atomic structure and the interaction between separate atoms.
2. NUCLEAR PHYSICS
Nuclear physics is a discipline within astrophysics that will study a proton and a neutron at the center of an atom and the interactions that hold them together in a space just a few femtometres across.
3. CONDENSED-MATTER
The study of CONDENSED MATTER is a discipline within astrophysics that will focus on the macroscopic and microscopic physical properties of matter, especially the solid and liquid phase that will arise from electromagnetic forces between atoms. More generally, the subject will study the condensed phase of matter: a system of many constituents with strong interactions among them. A more exotic condensed phase will include the superconducting phase exhibited by certain materials at an extremely low cryogenic temperature, the ferromagnetic and antiferromagnetic phase of spins on crystal lattices of atoms, and the Bose–Einstein condensate found in below freezing atomic systems.
4. PLASMA
The study of PLASMA is a discipline within astrophysics that will examine almost all of the observable matter in the universe found in the plasma state. Formed at high temperature, plasma consists of freely moving ions and free electrons. It is often called the “fourth state of matter” because its unique physical properties distinguish it from a solid, liquid, and gas. Plasma densities and temperatures vary widely, from the cold gases of interstellar space to the extraordinarily hot, dense cores of stars. Plasma densities range from those in a high vacuum with only a few particles inside a volume of 1 cubic centimeter to 1,000 times the density of a solid.
5. SUPER-FLUIDITY
The study of SUPER-FLUIDITY is a discipline within astrophysics that will examine the characteristic property of a fluid with zero viscosity, which could flow without any loss of kinetic energy.
6. GENERAL RELATIVITY
The study of GENERAL RELATIVITY is a discipline within astrophysics that will examine GRAVITY, a fundamental force in the universe. Gravity does define macroscopic behavior, which will describe large-scale physical phenomena. General relativity does, however, follow from Einstein’s principle of equivalence: on a local scale. It is impossible to distinguish between a physical effect due to gravity and those due to acceleration. Gravity is regarded as a geometric phenomenon that could arise from the curvature of space-time.
7. QUANTUM-FIELD THEORY
8. STRING THEORY
ASTROGEOLOGY
This solar system of astronomy will have an interest in the study of rocks, terrain, and material in space.
THE WORLD(S) OF ASTROGEOLOGY:
☆EXOGEOLOGY
This world of astrogeology will study how geology would relate to celestial bodies like moons, asteroids, meteorites, and comets.
☆SELENOGRAPHY
This world of astrogeology will study how any physical feature on the moon formed, such as the lunar maria, craters, and the range of mountains.
4 notes
·
View notes
Text
Hafnium Metal Prices: Market Analysis, Trend, Graph, News and Forecast
Hafnium Metal Prices a rare and valuable element used primarily in the nuclear, aerospace, and electronics industries, has witnessed dynamic price movements in recent years due to shifting supply chains, rising demand, and limited production sources. As a byproduct of zirconium refining, the global hafnium market is inherently constrained by the production of its parent mineral, making the supply chain highly susceptible to disruptions. This scarcity, combined with growing industrial applications, has contributed to the fluctuating price trends observed in the hafnium metal market.
The global demand for hafnium metal has surged due to its unique properties such as high thermal stability, excellent corrosion resistance, and neutron absorption capabilities. These characteristics make hafnium essential in nuclear reactors, particularly in control rods, where its ability to absorb neutrons without becoming radioactive enhances operational safety. With increasing investments in nuclear energy across countries like China, India, and Russia, the requirement for hafnium has expanded significantly, putting upward pressure on prices. At the same time, the aerospace industry has become a major end-user, utilizing hafnium alloys in jet engines and spacecraft components where high temperature resistance is critical. The dual demand from both nuclear and aerospace sectors continues to push the global market towards a tighter supply-demand balance.
Geopolitical factors also play a substantial role in shaping hafnium metal prices. Most of the world's zirconium—and hence hafnium—is mined and processed in a handful of countries, such as Australia, South Africa, and China. Any disruption in mining operations, policy changes, or export restrictions from these regions can quickly impact global supply. For example, stricter environmental regulations in China have periodically reduced zirconium production, thereby indirectly impacting hafnium availability. In turn, such constraints cause spot market prices to spike, especially when demand from industrial users remains robust. This volatility creates uncertainty for manufacturers relying on stable raw material costs, prompting some to explore long-term supply contracts or alternative sourcing strategies.
Get Real time Prices for Hafnium Metal: https://www.chemanalyst.com/Pricing-data/hafnium-metal-1598
The hafnium metal market is also influenced by advancements in technology, particularly in the semiconductor industry. As chip manufacturers push the limits of miniaturization and performance, hafnium-based high-k dielectrics have become essential for improving transistor efficiency and reducing leakage current. This technological shift, driven by the global push for faster and more energy-efficient electronic devices, has amplified the demand for ultra-pure hafnium compounds. In response, producers have been forced to enhance refining capabilities, which adds to production costs and can translate to higher market prices. The intersection of technological innovation and limited raw material supply reinforces the premium nature of hafnium in high-tech applications.
Another critical factor impacting hafnium prices is the growing emphasis on strategic materials and supply chain security. Governments and corporations around the world have started recognizing the strategic importance of rare metals like hafnium, leading to increased stockpiling and investment in domestic production. For instance, the United States has categorized hafnium as a critical mineral, prompting efforts to secure local sources and reduce dependence on foreign supply. Such initiatives, while aimed at stabilizing long-term access, can introduce short-term market distortions as players compete to secure limited volumes. These dynamics often result in speculative buying and elevated prices in the open market.
Market speculation and investment trends also contribute to price fluctuations in the hafnium sector. As with many rare and strategic metals, investor interest can drive short-term price increases, especially during periods of global economic uncertainty or heightened geopolitical tensions. When traders anticipate future supply bottlenecks or spikes in industrial demand, they may buy up available inventory, reducing immediate supply and driving prices upward. This speculative behavior is particularly pronounced in illiquid markets like hafnium, where trading volumes are relatively low and pricing transparency is limited. As a result, even small shifts in buying behavior can lead to significant price changes.
Environmental and sustainability concerns are increasingly shaping the hafnium supply chain, influencing both production practices and regulatory oversight. The extraction and refining of zirconium ores must now meet stricter environmental standards in many regions, which affects the cost and feasibility of hafnium recovery. Companies are investing in cleaner technologies and more efficient processes to meet these requirements, and while these innovations are vital for long-term sustainability, they can also contribute to increased operating costs. These added costs often filter through to the end product, impacting the overall price structure of hafnium metal in global markets.
The ongoing shift toward clean energy and advanced manufacturing is expected to keep hafnium prices elevated in the foreseeable future. As global economies prioritize low-carbon technologies, demand for nuclear power is set to rise, bolstering the need for hafnium-based materials. Similarly, the rise of electric vehicles, quantum computing, and next-generation electronics will likely sustain demand for hafnium in high-performance applications. With limited production expansion and long lead times for new capacity development, the market is likely to remain supply-constrained, maintaining firm price levels or even driving further appreciation depending on the pace of demand growth.
In conclusion, the hafnium metal market is influenced by a complex web of factors including limited supply, expanding industrial demand, geopolitical risks, environmental regulations, and technological evolution. These dynamics create a volatile pricing environment where costs can rise swiftly in response to minor disruptions or shifts in demand. As the strategic importance of hafnium continues to grow, stakeholders across the supply chain must remain agile and informed, leveraging market insights to manage risk and seize emerging opportunities in this high-value sector.
Get Real time Prices for Hafnium Metal: https://www.chemanalyst.com/Pricing-data/hafnium-metal-1598
Our Blog:
Paints and Coatings Solvents Prices: https://www.chemanalyst.com/Industry-data/paints-and-coatings-solvents-3
Synthetic Rubber Prices: https://www.chemanalyst.com/Industry-data/synthetic-rubber-4
Contact Us:
ChemAnalyst
GmbH - S-01, 2.floor, Subbelrather Straße,
15a Cologne, 50823, Germany
Call: +49-221-6505-8833
Email: [email protected]
Website: https://www.chemanalyst.com
#Hafnium Metal Pricing#Hafnium Metal News#Hafnium Metal Price Monitor#India#united kingdom#united states#Germany#business#research#chemicals#Technology#Market Research#Canada#Japan#China
0 notes
Text
Vanadium's Role in the Renewable Energy Revolution: Market Outlook
Vanadium Market Growth & Trends
The global Vanadium Market size was estimated at USD 2.73 billion in 2023 and is estimated to grow at a CAGR of 3.0% from 2024 to 2030. Growing crude steel production on account of demand from construction, automotive, machinery, and transportation industries is expected to fuel the demand for vanadium over the coming years.
Vanadium is primarily used as an alloy in steel manufacturing, where it increases the strength, toughness, and wear resistance of steel. The global demand for high-strength steel in the construction, automotive, and aerospace sectors is on the rise, driving the demand for vanadium. As countries and companies invest in infrastructure and manufacturing, demand for vanadium is expected to observe growth. For instance, as reported by the World Steel Association, global crude steel production reached 1,892.6 million tons in 2023 from 1,878.6 million tons in 2019.
Vanadium is a key component in vanadium redox flow batteries (VRFBs), which are used for energy storage systems. Owing to shifts toward renewable energy sources, the demand for efficient, large-scale energy storage solutions is increasing. VRFBs are particularly suited for grid storage due to their scalability, long lifespan, and ability to discharge over a long period, making vanadium an essential material for the emerging clean energy economy. This shift represents a significant growth driver for the vanadium market as energy storage technologies become increasingly vital.
The nuclear energy sector represents a significant opportunity for vanadium products. In nuclear energy, vanadium alloys are highly valuable due to their low neutron-absorption characteristics and high temperature and corrosion resistance. These properties make vanadium-based alloys ideal for use in nuclear reactors, particularly in the construction of pressure vessels and structural components. The ability of vanadium alloys to withstand the harsh operating environments inside a nuclear reactor without significant degradation extends the service life of these components, enhancing the overall safety and efficiency of nuclear power plants.
Curious about the Vanadium Market? Download your FREE sample copy now and get a sneak peek into the latest insights and trends.
Vanadium Market Report Highlights
Steel held the largest revenue share of over 90% in 2023. In superconducting magnets, vanadium plays a crucial role, especially in the form of Vanadium Gallium (V3Ga) superconductors.
Asia Pacific held over 62% revenue share of the global vanadium market. North America vanadium market is primarily driven by a strong focus on the aerospace and defense industry, which requires high-strength steel alloys.
Vanadium Market Segmentation
Grand View Research has segmented the global vanadium market report on the basis of application, and region.
Application Outlook (Volume, Kilotons; Revenue, USD Million, 2018 - 2030)
Steel
Non-Ferrous Alloys
Chemicals
Energy Storage
Regional Outlook (Volume, Kilotons; Revenue, USD Million, 2018 - 2030)
Europe
Germany
UK
Russia
North America
U.S.
Canada
Mexico
Asia Pacific
China
India
Japan
South Korea
Central & South America
Brazil
Middle East & Africa
Download your FREE sample PDF copy of the Vanadium Market today and explore key data and trends.
0 notes
Text
Radiation Safety in Industrial Hygiene Nature has it that all living things depend on a certain type of radiation to survive. This is evident in many ways for instance we can see because our eyes sense and become aware of the radiation in the form of light; then there is infrared radiation that allows is to keep ourselves warm in the cold weather, radiation is used for cooking, whether it is on the stove or in the microwave. Radiowaves are used for long distance communication by using sound or picture; and ultraviolet radiation is used for medical treatment or for putting on a good suntan. Even though some forms of radiation can travel long distances, it can be stopped by employing the correct absorbers: starlight can transgress galaxies but then using a piece of paper it can also be stopped; radiowaves are also capable of travelling huge distances but can be absorbed by metal like substances. Just as light travels in straight lines, so does ionising radiation til it is absorbed by materials. It was back in the twentieth century, that "ionising radiation" was discovered. Ionising radiation is found everywhere and supports our natural background radiation. It is also found in many sources such as the sun, outer-space, the rocks and soil beneath our feet, the buildings we inhabit, the air we breathe, the food and drink we consume, and even in our bodies. Types and sources of ionising radiation Inclusion to X-rays, are three types of ionising radiation called alpha, beta and gamma. Alpha rays also called the helium nuclei can be stopped by paper, beta rays also known as high-speed electrons are stopped less easily, and gamma rays need lead or concrete to stop them. It is a known fact that since one cannot glow in the dark due to light similarly, a chest X-ray will not make one radioactive, therefore, ionising radiations will not make one radioactive. This is because in industries in a reactor there are billions of free nuclear projectiles called neutrons, which on absorption into any material cause it to become radioactive. This leads to the material producing its own radiation. A person's annual radiation dosage originates from the decay of natural radioactivity because the presence of bricks and mortar escalates the concentration of a radioactive gas called radon. Radon is produced naturally from the radioactive decay of uranium and thorium, found in rocks, soil, bricks, mortar, tiles and concrete. Plunging ventilation to conserve energy in turn causes an increase in the radon concentration in the air we breathe. Another way to increase radon concentration is by using bore water, especially in hot showers. Other sources of smaller dosages of everyday radiation come from cosmic rays and from the natural radioactivity found in the consumption of our food and drink. The man-made sources of radiation are from the use of medical technology, such as the usage of X-rays for radiography and tomography, and radioactivity in nuclear medicine. According to a study, a person absorbs more than 2000 microsieverts a year of natural background radiation. Other sources of additional doses depend on the medical use of radiation according to a person's medical history. Dental X-rays account for one-tenth the annual background while multiple X-rays in combination with a barium enema, accounts for a huge percentage of the annual background radiation. Radiation doses are the highest in cancer therapy fro cancer patients.. Some people get more radiation than others since study shows that cosmic rays are different for different latitudes, height above sea-level, and with sun activity: on top of a mountain, the radiation dose is higher than on the ground. Also rocks and beach sand are more radioactive than other parts of the earth. Radioactive substances affects our food and drink, olives and brazil nuts are two food items that receive more radioactivity in comparison to others. There are many industries that produce and release radioactivity into the environment, this is especially the case with coal-burning plants, and to some extent, the fertiliser, mining and building industries. Other sources of radiation exposure are: older luminescent clocks and watches, compasses, exit signs, certain paints and pigments, dental porcelain, fire alarms, smoke detectors, television sets, normal operations of the nuclear power industry, and the use of radionuclides in industry, agriculture and the environment. Still with so many sources, the human race has continued to survive in this radioactive environment. It's next to impossible to avoid the sources of natural radiation in our everyday life but we can take precautions to maintain distance with the local sources of radiation and also use distance, time and radiation shielding to protect ourselves. The less contact we have with such sources the lesser will be the dosage, however if it is necessary to use a source then it is mandatory one minimises the time spent near it, and in case of the source emitting strong radiation it is a must that one uses adequate shielding between the source and themselves. The effect of radiation on the body Ionising radiation does not accumulate in our body, but science proves that the radiation effects are evident from exposure to large amounts of radiation, as in sunburns from too much exposure to strong sunlight. Radiation carries energy that has a damaging effect on the living cells of living things and can either kill them or change their structure and function to inhibit correct functioning but this would take large doses to kill a good number of cells to cause death. Radiation dose would have to be several thousand times bigger than the dose received annually from the environment to cause death. Death would occur if the person were exposed more over a year. For example, exposure to sunlight over a year gives one a suntan, but one-day exposure of sunbaking could cause death by sunstroke. Over a period of time, the body has the ability to repair small damage caused by radiation, but small dose can become more serious. There are two kinds of radiation damage: damage to any of the cells of our body, putting one at risk, and can even damage our reproductive cells putting future generations at risk. There are many different somatic effects but the long-term effect of radiation is caused by cancer. High exposure to radiation puts a person at a 40:1000000 chance of getting a cancer from a dose of radiation equal to one year of natural background. This means that lifetime dose of natural background gives one a 1:500 chance of dying from cancer. Detecting radiation Ionising radiation is detected by using a geiger counter. Radiographers, workers in the nuclear industry, and radiation workers are required to wear a film badge or a thermoluminescent dosimeter to record their radiation doses. The International Commission on Radiological Protection suggests that all doses should be kept "as low as reasonably achievable" stating that doses absorbed by radiation workers should not exceed 50,000 microsieverts over the whole one year, while the public should not receive more than one-fiftieth of the workers lifetime average. Standards put by the regulating bodies and how they have affected the workplace. In 1958, the Radiation Protection Act, in New Jersey was implemented. This Act allowed the authority to set standards for the possession, handling, transportation and use of sources of radiation in the State of New Jersey. The Act led to the formation of the New Jersey Commission on Radiation Protection called the Commission to circulate rules and regulations to ban and prevent unnecessary radiation. In adherence with the Act, the Department of Environmental Protection implemented the rules. In the past decades much concern has been shown regarding the adverse human health effects caused by the exposure to electric and magnetic fields from 60 Hertz electric power transmission, sub-transmission, distribution lines and other sources including appliances. The concern arose after a series of epidemiological studies, showed that children with cancer, such as, leukemia, lymphoma and brain cancer are likely to live longer in housing with higher magnetic field exposures of the electrical distribution wires, measured magnetic fields and magnetic fields. Also studies indicate that workers with high magnetic field exposures, as indicated from job classifications and magnetic field measurements, indicate higher rates of cancer. Radiation is however not responsible for the assumed mechanism of carcinogenesis caused by the exposure to magnetic fields and this is not found with much evidence since there is not much experimental evidence to assist the epidemiologic findings. Possible reasons for the lack of enough information are due to the wrong explanations for the increased rates of cancer usage of inappropriate exposure measures. It will take a number of years before the issue is solved scientifically, and from a biologically perspective to show that a certain measure of exposure can be linked to exposure and disease. To reduce radiation, the commission has installed new and modified electric power transmission lines for three reasons. First of all, either it is believed that these lines do not yet exist or if they do exist than these lines should be proposed for modification. By changing the wire configuration, or the phasing or other line characteristics to lower the resultant magnetic field will cause minor changes in the limited economic costs for the utilities or ratepayers. The Commission did this so that limited cost increments would cut down the warranted potential hazard of magnetic field exposures. Those areas with the highest magnetic fields, receive large doses of radiation from the electric power transmission line. This implies that the greater the magnetic field the greater the risk, however controlling the electric power transmission lines will restrict the exposure for those exposed to the highest fields, and also at greatest risk. The Commission recognizes that this way the population will become a small number of those exposed, and form that part of the society that is not affected by the health hazards. It is known that there are other short-term, controllable, everyday exposures from electric power transmission lines, found in appliances, but radiation emission is not so lethal from them. Ionizing Radiation Radiation is defined as the movement of energy in free space. Radioactivity is the activity of some unstable elements to decay and release radiation. Ionizing radiation contains enough energy to release electrons from atoms causing certain chemical properties of the substance it interacts with to change. Ionizing radiation is found in alpha particles, beta particles, gamma rays, x-rays and cosmic rays. In chemistry it is known that when a nuclide decays, the left over substance will never possess the same chemical element as the original element had. After a certain point, the decay product will not be able to decay further due to stability. But if the decay product is still unstable it can undergo another radioactive decay later on. This is called half-life, which is defined as the time taken for half of a given amount of a nuclide to undergone radioactive decay. Contamination If contact is made with radioactive substances, it leads to contamination and the spread of radioactive material, however it is also true that simply contact with radioactive material will not make non-active substances radioactive. Materials become 'radioactive' when they are contaminated by radioactive material by keeping them in close contact with such substances. An example of this is when a patient irradiated in the beam of an X-ray machine does not come out of the x-ray room being radioactive. However, a worker in the same room, in close contact with radioactive dust may have clothes or skin that has been contaminated or in the danger of inhaling radioactive material. This is why the person needs to undergo decontamination to lessen his radiation inhalation and consequently protect others from radiation. The risks involved in radiation have been studied in depth by the study of groups of people exposed to known amounts of radiation using their own personal experiences, such as, atomic bomb survivors or radiotherapy patients, and also by the study of biological and cellular experiments. Radiation can cause two sorts of harmful effects on health because of radiation damage to tissues. Stochastic Effects These effects simply increase the risk of cancers and hereditary diseases over many years or decades after the first radiation dose. The risk is primarily proportional to the dose. To illustrate this, the best case is of radioiodine, which causes radiation exposure in a particular pattern of cancer in children its papillary thyroid carcinoma, which is very different from the natural incidence pattern. Despite the information available, the truth is that cancers caused by radiation cannot be differentiated from those caused by natural means. The long-term cancer risk is estimated to be 5% per Sievert of whole body effective dose. This means that an additional radiation dose of 5 mSv would add an additional 0.025% to a person's 25% risk of dying from cancer. This would make their total cancer death risk 25.025%. Risk factors for non-fatal cancer and hereditary effects have also been estimated and are used for the purposes of radiological protection. Overall the risks of hereditary effects are judged to be substantially less than those of cancer. Deterministic Effects These effects take place when a threshold dose is reached but they really show the effects when very high doses of radiation are taken causing radiation sickness and radiation burns. The symptoms start after exposure and the greater the radiation dose is, the more severe the symptoms are. Symptom Whole Body or Localized Exposure Minimum Dose for Detection Detectable Chromosome Damage Without Symptoms Whole Body 0.1 Gy Radiation Sickness Whole Body 1 Gy Death From Radiation Sickness Whole Body 3 Gy (without medical treatment) & 5 Gy (with medical treatment) Skin Reddening Localized 5 Gy Skin Burn Localised 10 Gy Source: LONDON HEALTH OBSERVATORY - Health In London, published: 18 November 2003] Other than direct effects on the body, psychological effects may also take place in the person exposed to severe radiation and it has nothing to do with the radiation dose. The causes can be due to the way an incident is taken care of affecting the person's psychological side in an incident. Radiation Exposure Monitoring Radiation Exposure number of different organizations are making wide environmental measurements around the world, including local authorities and Government regulatory bodies. They research includes air measurements to look for radioactive plumes and regular monitoring of ionizing radiation in food crops as contained in the FSA report on radioactivity in food and the environment under Food standards. Industries using radioactive materials are strictly monitored by the HSE, Nuclear Installations Inspectorate and, in also by the local authorities. Workers at risk of high exposure to radiation are required to undergo personal monitoring of radiation dose. The Central Index of Dose Information is the Health & Safety Executive's national database of occupational exposures to ionizing radiation. Radioactive sources and discharges into the environment have to be allowed under the Radioactive Substances Act (1993) by the Environment Agency. Heath and safety bodies from the department of health take care of and control medical radiation in the patient exposed. Businesses using radioactive resources must have a 'radiation protection adviser' such as a physicist, who keeps a check on the equipment and procedures. Radiation Accidents On an average, there is one major radiation accident every year in some part of the world. What is more common in England is accidents associated with lost source's. Lost sources are found in the scrap metal industry. Scrap metal yards have radiation monitors to detect any unknown radiation in the delivery received. The nuclear industry and many other resources of radioactive sources are used in an enormous range of industrial processes such as, industrial radiography, thickness gauges, smoke alarms, medical diagnosis and treatment. This is why the number of accidents related to radiation is relatively high in the UK. The UK National Arrangements for Incidents involving Radioactivity scheme, employs a system that sends a response to the police in the UK if they suspect an uncontrolled radiation source. Types Of Radiation Accident There are 4 types of possible accident. Criticality accidents. This is where a nuclear chain reaction occurs when not intended and radiation is released to the environment. An example is the accident in Tokai-Mura, Japan in 1999. A mixture of enriched uranium in nitric acid, which exceeded the critical mass, was created by mistake and a nuclear chain reaction occurred in a research laboratory giving very large doses to two people. A detonated nuclear weapon spreads fission products over a wide area because of a nuclear chain reaction. Reactor accidents. A working reactor allows a nuclear chain reaction to occur in controlled circumstances. If the structure that contains the reactor is breached, or the nuclear reaction goes out of control, then fission products can be released into the environment. The accident at Chernobyl in 1986 is the best recent example. Fires dispersing a plume of radioactive material. Here there is no nuclear chain reaction, but a plume disperses the radioactivity from a fire. If a nuclear weapon were involved in an accidental fire or conventional explosion, then plutonium from the weapon would disperse in this way. This happened in Palomares, Spain in 1966 when a B52 plane, carrying nuclear missiles crashed. This is not the same as detonation of the weapon. Lost sources. The most harmful known lost source accident to date occurred in Goiania, Brazil in 1987. A radiotherapy source was broken open and radioactivity scattered widely within a city by hand-to-hand contact and cross contamination of the environment. Thousands of people were contaminated. Source: LONDON HEALTH OBSERVATORY - Health In London, published: 18 November 2003] Environmental health effects are similar on workers in the industry and public from ionizing radiation and perhaps from air pollution. Ionizing radiation has been under the limelight after the testing of nuclear weapons. This has been able to create compelling awareness in the nuclear power industry concerning radiation hazards. Radioactivity is measurable and its effects are understood better than those of other hazards with delayed effects, such as, all chemical cancer-inducing substances. Radiation is classified as a weak carcinogen The food industry uses radiation The food industry too uses radiation in the production, quality assurance, and sterilization of products. Radiation is increasingly used for the production of synthetic materials and natural rubber products. The radiation is used for polymerization without the bad side effects of heat processing. Radiation is also used to preserve cold pasteurize food and sterilize medical products. This is the most cheapest way to destroy bacteria and viruses in products produced for human consumption for people with not so healthy immune systems. Radiation has many uses, but it should be used cautiously to prevent the calamities that go hand in hand with its usage in industry and elsewhere. Read the full article
0 notes
Text
Zirconium Target | High Purity & Thermal Stability
Zirconium Target are essential in skinny-film coating, semiconductor, and optical packages. Known for his or her high corrosion resistance, thermal stability, and occasional neutron absorption, they're broadly utilized in sputtering and PVD techniques. Their sturdiness and overall performance in extreme environments make them perfect for high-tech and industrial production programs.
0 notes
Text
The Chapter of Preaching Tao, Article 6: The Unified Field of the Universe
Xuefeng
December 30, 2005
(Translation edited by Qinyou)
The term "universe" encompasses all infinite space (宇) and all infinite time (宙). Thus, the universe is a composite of material and nonmaterial components, constituted by infinite space and infinite time.
The "unified field of the universe" refers to the laws that universally apply to all times and spaces—essentially, "universal laws" that hold true everywhere.
Establishing a unified field of the universe has been the dream and pursuit of scientists. They envision using a simple, clear formula to describe this unified field theory—a formula that could be called the ultimate formula, applicable to all fields.
It is said that Einstein spent thirty years of his later life attempting to find this formula, but in the end, he was left lamenting and empty-handed. Modern scientists, whether globally renowned or obscure, have all employed various methods in their quest to solve the problem of the unified field of the universe, hoping to achieve the glory of solving this problem, akin in significance to the Goldbach conjecture.
The promising prospect is alluring, but it remains as elusive as a mirage—seemingly near, yet far. After reviewing the history and paths they have taken, I found that they have made a fundamental directional error.
First, let us consider the scientists' perspectives. Their views are uniformly focused on the material world, completely ignoring the nonmaterial world. It's like admiring a beautiful woman's smooth, tender skin and feeling the breath of love, while entirely overlooking the myriad charms and graces of her inner world. Thus, scientists are not masters of love in the unified field of the universe but are like jaded veterans lingering in the red-light district.
Scientists believe that there are four fundamental forces in the universe: magnetic force, gravitational force, strong nuclear force, and weak nuclear force. They think that if a formula or theory could seamlessly incorporate these four forces, a unified field of the universe could be established, allowing them to effortlessly enjoy the mysteries of the universe and marvel at its wonders.
They are deeply intoxicated by their own fantasies and find it difficult to extricate themselves. Not to mention establishing a unified field of the universe—they are currently unable to unify even these four forces with a single formula. Even if they were one day to unify them, it would be akin to Tang Monk encountering a false Thunder Sound Temple on his journey to the West, rather than the true Thunder Sound Temple where the Ancestral Buddha Tathagata resides.
How many fundamental forces are there in the universe?
Answer: There are eight—magnetic force, gravitational force, strong force, weak force, structural force, repulsive force, conscious force, and spiritual force.
Here is a brief description of these eight forces:
l Magnetic Force: The interaction between charged particles.
l Gravitational Force: The force of attraction between masses, caused by the curvature of spacetime due to mass and motion.
l Strong Force: The force that binds quarks together in protons and neutrons and binds protons and neutrons together in atomic nuclei.
l Weak Force: The force that acts on all matter particles but not on force-carrying particles.
l Structural Force: The force generated by the structure of material and nonmaterial components, with each structure having its unique energy absorption force.
l Repulsive Force: The force that causes like charges or poles to repel each other.
l Conscious Force: A force similar to the brain's ability to make the bladder and urethra start working when one wants to urinate.
l Spiritual Force: The force that causes mutual perception between things.
I have said that scientists cannot establish a unified field theory of the universe primarily because they cannot measure or calculate the latter four forces. Even though these four forces cannot be measured or calculated, we cannot deny or ignore their existence. Since they exist, and you cannot incorporate them into a single formula or theoretical system, you cannot establish a unified field of the universe. This is the fundamental reason why Einstein's efforts were in vain.
At this point, many "scientists" might mock Xuefeng. Go ahead and mock! Laugh to your heart's content! Let's see who will laugh last.
So, can the unified field of the universe not be established? No, it has already been established.
This is—the Tao of the Greatest Creator, also known as the holographic system, composed of the three elements of the universe: consciousness, structure, and energy.
The universe is holographic, and the Tao of the Greatest Creator encompasses the eight forces mentioned above, incorporating them wonderfully into a unified system.
Scientists have focused their attention only on the material world, ignoring the nonmaterial world. But the universe is composed of both material and nonmaterial components, existing in infinite time and infinite space. How can a unified field of the universe be established if the nonmaterial world is ignored?
This is Lifechanyuan's unified field theory of the universe.
The remaining task is to work on the "Tao."
Why is this called "The Chapter of Preaching Tao"? This should now be clear.

0 notes
Text

TSRNOSS, p 572.
#surfactant#paraffin#absorption of radiation#gamma radiation#albedo#RBE#deuterium oxide#lithium#neutron absorption#cosmic radiation#tuberculosis#eskimoes#thiamine deficiency#psychosis#redwoods#typhus#enzymes#rotational energy#hemoglobin#diffusion rate of hemoglobin#infrared radiation
0 notes
Text
The Gadolinium Market is projected to grow from USD 5,833.3 million in 2024 to USD 9,020.4 million by 2032, reflecting a compound annual growth rate (CAGR) of 5.60%.Gadolinium, a rare earth element with the symbol Gd and atomic number 64, plays a critical role in various high-tech and industrial applications. This silvery-white metal is unique due to its magnetic properties and ability to absorb neutrons, making it indispensable in several sectors, from medical imaging to nuclear reactors. The gadolinium market has been witnessing significant growth, driven by increasing demand in these key areas.
Browse the full report at https://www.credenceresearch.com/report/contrast-media-market
Medical Imaging: A Major Driver
One of the primary applications of gadolinium is in medical imaging, particularly in magnetic resonance imaging (MRI). Gadolinium-based contrast agents (GBCAs) are used to enhance the quality of MRI scans. These agents improve the visibility of blood vessels, tumors, and other structures, aiding in accurate diagnosis and treatment planning. The rising prevalence of chronic diseases and the growing emphasis on early diagnosis are propelling the demand for MRIs, thereby boosting the gadolinium market. According to a report by Grand View Research, the global MRI market is expected to reach USD 9.8 billion by 2025, indicating a sustained demand for GBCAs.
Nuclear Reactors: A Key Application
Gadolinium's neutron absorption properties make it an essential component in nuclear reactors. It is used as a burnable poison in nuclear fuel rods, helping to control the fission process and extend the life of the reactor. As the world looks towards cleaner energy sources, nuclear power remains a vital part of the energy mix. Countries like China and India are investing heavily in nuclear energy to meet their growing power needs, thereby driving the demand for gadolinium. The World Nuclear Association projects that nuclear power capacity will increase significantly over the next few decades, providing a stable growth outlook for the gadolinium market.
Electronics and Magnetics
Gadolinium is also used in various electronic applications due to its magnetic properties. It is used in the manufacturing of gadolinium gallium garnet (GGG), which is a substrate for microwave and optical components. Additionally, gadolinium is used in the production of magneto-optical recording devices and as a component in various high-temperature superconductors. The expanding electronics industry, particularly in Asia-Pacific, is expected to drive the demand for gadolinium in this segment.
Supply Chain and Market Dynamics
The supply of gadolinium, like other rare earth elements, is dominated by China, which controls over 70% of the global production. This concentration of supply poses a risk to the global market, as geopolitical tensions and trade policies can impact availability and prices. Efforts are being made to diversify the supply chain, with countries like the United States, Australia, and Canada exploring their rare earth reserves and investing in mining projects. These initiatives aim to reduce dependence on China and ensure a stable supply of gadolinium.
Environmental and Regulatory Concerns
The extraction and processing of gadolinium, like other rare earth elements, have significant environmental impacts. Mining activities can lead to soil and water contamination, and the separation process generates harmful byproducts. Increasing regulatory scrutiny and the push for sustainable practices are encouraging companies to adopt cleaner technologies and reduce their environmental footprint. Recycling of gadolinium from electronic waste is also gaining attention as a potential way to meet demand while minimizing environmental impact.
Market Outlook
The gadolinium market is poised for steady growth, driven by its diverse applications and increasing demand in key sectors. The medical imaging segment will continue to be a major driver, supported by advancements in healthcare and the growing need for diagnostic procedures. The nuclear energy sector offers significant growth potential, particularly with the global shift towards cleaner energy sources. The electronics industry will also contribute to market expansion, given the ongoing technological advancements and increasing adoption of high-tech devices.
However, the market faces challenges related to supply chain concentration and environmental concerns. Addressing these issues through supply diversification, sustainable practices, and recycling initiatives will be crucial for the long-term growth and stability of the gadolinium market. As industries continue to evolve and new applications for gadolinium emerge, the market is set to play a vital role in the future of technology and energy.
Key Player Analysis
Bracco Imaging S.p.A.
GE Healthcare
Bayer AG
Daiichi Sankyo Company, Limited
Guerbet Group
Mallinckrodt Pharmaceuticals
Taejoon Pharm Co., Ltd.
J.B. Chemicals & Pharmaceuticals Ltd.
Spago Nanomedical AB
Isologic Innovative Radiopharmaceuticals
Segments:
Based on Product Type:
Acetate
Acetylacetonate
Oxide
Nitrate
Chloride
Others
Based on Application:
Electronics
Medical Equipment
Imaging Agents
Nuclear Reactors
Others
Based on the Geography:
North America
US
Canada
Mexico
Europe
Germany
France
UK
Italy
Spain
Rest of Europe
Asia Pacific
China
Japan
India
South Korea
South-east Asia
Rest of Asia Pacific
Latin America
Brazil
Argentina
Rest of Latin America
Middle East & Africa
GCC Countries
South Africa
Rest of the Middle East and Africa
Browse the full report at https://www.credenceresearch.com/report/contrast-media-market
About Us:
Credence Research is committed to employee well-being and productivity. Following the COVID-19 pandemic, we have implemented a permanent work-from-home policy for all employees.
Contact:
Credence Research
Please contact us at +91 6232 49 3207
Email: [email protected]
0 notes
Text
E009: Radiation Protection- Final
Radiation is the shedding of extra energy from a radioisotope or radionuclide. It emits energy in the form of waves or particles. Protection against radiation aims to reduce unnecessary exposure and to minimize the harmful effects of ionizing radiation. There 4 different types of ionizing radiation: Gamma, Alpha, Beta, and Neutrons. Major sources of exposure can include natural occurrences; cosmic, cosmogenic, terrestrial and technology modified sources; radiation therapy or radiology imaging. Both have potential to cause internal contamination through specific biological pathways. This includes absorption through open cuts and wounds, ingestion, and inhalation. In comparison, external contamination comes from the skin, surfaces, and clothing. Radiation damage to tissue and/or organs depends on the dose of radiation received, or the absorbed dose which is expressed in a unit called the gray (Gy). The potential damage from an absorbed dose depends on the type of radiation and the sensitivity of the different tissues and organs. Damage done by ionizing radiation is at a cell or subcellular level and can be repairable. When used appropriately, ionizing radiation has many beneficial applications, including uses in medicine, industry, agriculture, and research.
0 notes
Text
It’s difficult to understand why anyone would ever say that small nuclear reactors would lead to the production of less waste, and yet we have seen the claim made, repeatedly. For a given reactor type, the smaller the core, the greater the loss of neutrons by leakage. This means that the initial fuel charge must have a greater proportion of fissile material, and less of it is consumed before the operating reactivity margin falls too low and it must be replaced.
This study, however, doesn’t make a great deal of sense. The authors concentrate on two factors which are both probably irrelevant. The first is neutron activation of steel — specifically the steel of the reactor pressure vessel. The first reason that this is surprising is that the main constitutents of steel, iron and carbon, do not generally become transformed into radioactive isotopes by interaction with neutrons, and especially not long-lived, energetic radioisotopes. About the only substance commonly found in steel that does become so activated is cobalt, and so that element is typically excluded from reactor construction. (There is also some possibility of neutron absorption in molybdenum to form technetium.) Since the half-life of cobalt-60 is less than 6 years, irradiated stainless steels and other nickel alloys containing traces of cobalt can, if necessary, be held for 60 years for the activity to decay, before being mixed with other scrap steel.
Now, neutron collisions move atoms out of their places in the crystal lattice of a solid material. This happens much more often than the absorption of neutrons to create new (and sometimes radioactive) nuclei. As a result, inside the typical reactor pressure vessel you will find something called a “thermal shield”. This is a steel liner, which is under no structural load, so that changes in its mechanical properties as a result of such displacements, known as “neutron embrittlement”, don’t hurt anything. In other words, its whole function is to stop neutrons from getting to the pressure vessel (which is frequently lined with stainless steel, which in turn may contain traces of cobalt). And since this thermal shield is constructed of materials which do not become strongly and long-lastingly radioactive under neutron bombardment, it can be treated as normal scrap steel after a moderate cooling-off period.
The second factor they consider is radiotoxicity of plutonium in the fuel wastes. This, it seems to us, reflects a fundamental misunderstanding of the role of the small reactor. The large nuclear power reactor is very economical in meeting the energy needs of large cities. In the absence of anti-nuclear political pressure, the demand for such reactors tends to be strong. While there are many potential applications for small reactors, relatively few of them are so economically or technically compelling that they are likely to be pursued, absent a strong commitment to shifting the overall energy supply towards fission.
A heavily-nuclear energy economy requires a closed, regenerative nuclear fuel cycle. In other words, small reactors are not likely to account for more than a very small amount of the nuclear fuel consumed (and thus the fuel waste produced) unless discharged fuel is going to reprocessing plants and into breeder reactors, not to geological repositories for disposal. Therefore the question of “disposing of plutonium” from such small reactors is probably irrelevant.
#nuclear waste#atomic power to the people#small reactors#total nuclearization#deep decarbonization#closed fuel cycle#the plutonium economy
1 note
·
View note
Text
Ever wondered What Are Industrial Application Of Customized Molybdenum
Ever wondered about the industrial applications of customized molybdenum? Here's a glimpse:
Aerospace: Customized molybdenum components are used in aerospace applications due to their high strength, heat resistance, and ability to withstand extreme environments. They are utilized in aircraft engines, structural components, and aerospace fasteners.
Medical: In the medical industry, customized molybdenum parts are employed in various medical devices and equipment. They offer biocompatibility, corrosion resistance, and sterilization capabilities, making them ideal for applications such as surgical instruments, implants, and diagnostic tools.
Energy: Molybdenum is crucial in energy-related applications, particularly in nuclear power generation. Customized molybdenum components are used in reactor cores, fuel rods, and other critical components due to their high melting point and excellent neutron absorption properties.
Electronics: Molybdenum finds applications in the electronics industry, where its high thermal conductivity and low thermal expansion coefficient are beneficial. Customized molybdenum parts are used in semiconductor manufacturing, vacuum deposition systems, and electrical contacts.
Defense: Customized molybdenum components play a vital role in defense applications, including military vehicles, aircraft, and naval vessels. They offer durability, corrosion resistance, and high-temperature performance, meeting the stringent requirements of defense systems.
For customized molybdenum components tailored to your specific marine applications, trust Marine Techq, a leading provider of high-quality marine engineering solutions. With Marine Techq's expertise and commitment to excellence, you can be assured of reliable and customized molybdenum components that meet the rigorous demands of the marine industry.
0 notes
Text
November 11, 1930: Patent Granted For Einstein-Szilard Refrigerator
Refrigeration Patent
Albert Einstein is best known to the general public for devising the world’s most famous equation: E=mc2. But his contributions to physics extend over a broad range of topics, including Brownian motion, the photoelectric effect, special and general relativity, and stimulated emission, which led to the development of the laser. Less well known, even among physicists, is his work with Leo Szilard to develop an energy efficient absorption refrigerator with no moving parts.
Szilard was born in Budapest, Hungary in 1898, the son of a civil engineer, and served in the Austro-Hungarian Army during World War I. After the war, he returned to university, studying physics under Einstein and Max Planck, among others. His dissertation was in thermodynamics, and in 1929 he published a seminal paper, “On the Lessening of Entropy in a Thermodynamic System by Interference of an Intelligent Being”–part of an ongoing attempt by physicists to better understand the “Maxwell’s Demon” thought experiment first proposed by James Clerk Maxwell in the 19th century.
Szilard had a knack for invention, applying for patents for an x-ray sensitive cell and improvements to mercury vapor lamps while still a young scientist. He also filed patents for an electron microscope, as well as the linear accelerator and the cyclotron, all of which have helped revolutionize physics research. Szilard’s most important contribution to 20th century physics was the neutron chain reaction, first conceived in 1933. In 1955, he and Enrico Fermi received a joint patent on the first nuclear reactor.
Einstein wasn’t a stranger to the patent process, either, having worked as a patent clerk in Bern as a young man. He later received a patent with a German engineer named Rudolf Goldschmidt in 1934 for a working prototype of a hearing aid. A singer of Einstein’s acquaintance who suffered hearing loss provided the inspiration for the invention.
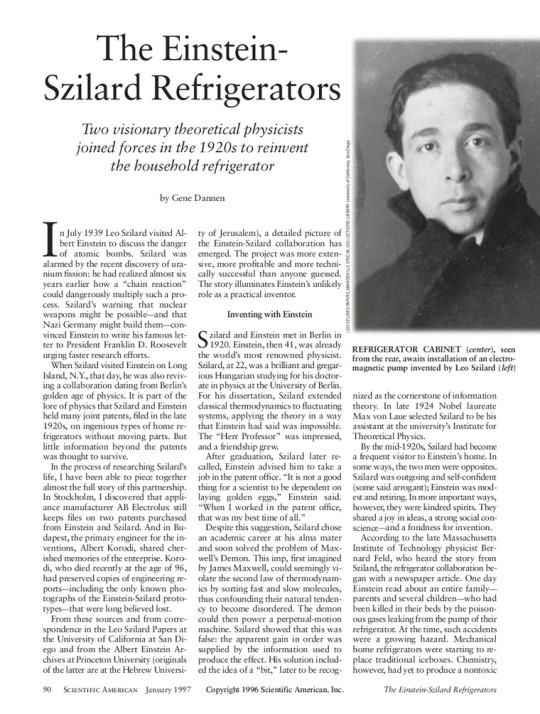
When they met, Einstein was already a world-famous physicist, thanks to his work on relativity, while Szilard was just starting out, as a graduate assistant at the University of Berlin. The impetus for the two men’s collaboration on a refrigerator occurred in 1926, when newspapers reported the tragic death of an entire family in Berlin, due to toxic gas fumes that leaked throughout the house while they slept, the result of a broken refrigerator seal. Such leaks were occurring with alarming frequency as more people replaced traditional ice boxes with modern mechanical refrigerators which relied on poisonous gases like methyl chloride, ammonia, and sulfur dioxide as refrigerants.
Einstein was deeply affected by the tragedy, and told Szilard that there must be a better design than the mechanical compressors and toxic gases used in the modern refrigerator. Together they set out to find one. They focused their attention on absorption refrigerators, in which a heat source–in that time, a natural gas flame–is used to drive the absorption process and release coolant from a chemical solution. An earlier version of this technology had been introduced in 1922 by Swiss inventors, and Szilard found a way to improve on their design, drawing on his expertise in thermodynamics. His heat source drove a combination of gases and liquids through three interconnected circuits.
One of the components they designed for their refrigerator was the Einstein-Szilard electromagnetic pump, which had no moving parts, relying instead on generating an electromagnetic field by running alternating current through coils. The field moved a liquid metal, and the metal, in turn, served as a piston and compressed a refrigerant. The rest of the process worked much like today’s conventional refrigerators.
Einstein and Szilard needed an engineer to help them design a working prototype, and they found one in Albert Korodi, who first met Szilard when both were engineering students at the Budapest Technical University, and were neighbors and good friends when both later moved to Berlin.
The German company A.E.G. agreed to develop the pump technology, and hired Korodi as a full-time engineer. But the device was noisy due to cavitation as the liquid metal passed through the pump. One contemporary researcher said it “howled like a jackal,” although Korodi claimed it sounded more like rushing water. Korodi reduced the noise significantly by varying the voltage and increasing the number of coils in the pump. Another challenge was the choice of liquid metal. Mercury wasn’t sufficiently conductive, so the pump used a potassium-sodium alloy instead, which required a special sealed system because it is so chemically reactive.
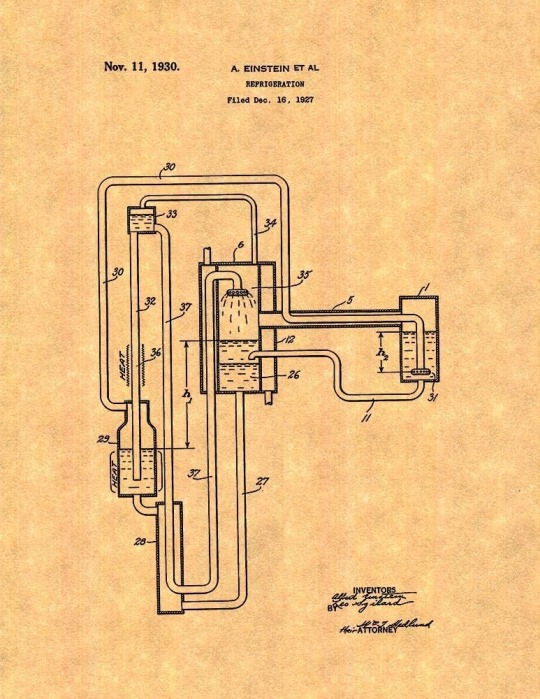
Despite filing more than 45 patent applications in six different countries, none of Einstein and Szilard’s alternative designs for refrigerators ever became a consumer product, although several were licensed, thereby providing a tidy bit of extra income for the scientists over the years. And the Einstein/Szilard pump proved useful for cooling breeder reactors. The prototypes were not energy efficient, and the Great Depression hit many potential manufacturers hard. But it was the introduction of a new non-toxic refrigerant, freon, in 1930 that spelled doom for the Einstein/Szilard refrigerator.
Interest in their designs has revived in recent years, fueled by environmental concerns over climate change and the impact of freon and other chlorofluorocarbons on the ozone layer, as well as the need to find alternative energy sources. In 2008, a team at Oxford University built a prototype as part of a project to develop more robust appliances, and a former graduate student at Georgia Tech, Andy Delano, also built a prototype of one of Einstein and Szilard’s designs. Yet another team at Cambridge University is experimenting with cooling via magnetic fields. Perhaps this invention won’t revolutionize the world, but in its own small way, it might help spare the planet–more than 70 years after Einstein and Szilard first conceived of it.
0 notes