#injection mold trial
Explore tagged Tumblr posts
Text
Unlocking Innovation: Exploring Two-Shot Molding and the Power of 2K Molds
Two-shot molding and 2K molding refer to advanced injection molding processes used in the manufacturing of plastic components. Here's a brief explanation of each:
In summary, two-shot molding and 2K molding are advanced techniques that offer increased design flexibility, cost savings, and improved product functionality by allowing the integration of different materials or colors into a single molded part.
#injection mold surface finish#injection mold tooling cost#2k mold#injection mold trial#molding#insert overmolding#plastic injection molding cost#injection molding#injection mold prototype#two shot mold
0 notes
Text
HEADCANON; On The Spider PT 1/?
After the death of Ben Parker and the hospitalization of May Parker due to a massive heart attack, Peter primarily spent his time in the care of Anna Watson.
May's hospitalization prolonged from 2002 into 2003 with Peter befriending Harry Osborn at the public school, though it is most likely Harry will attend a private school. despite this, they become fast friends.
Peter spend the first portion of 2003 with Harry, running all around OsCorp, with the main rule being to stay on the top two floors of the building as lower levels were sectioned off for research, development, and factory work which made the building self sustainable and vital to both federal and military research. The ground and first floor levels were open to student research and scholarships which made OsCorp a major asset and prime employer within New York City.
A top secret project had been commissioned within OsCorp, starting back after the first major success within the company: building Baxtor Tower in 1974. Baxtor Tower displayed not only the capability to be adaptable to the Fantastic Four's (a group of scientists from NASA as well as active members of the military) newly acquired powers, but also maintained the astronomical levels of radiation and prevented further spread of their conditions.
The project was to analyze the phenomenon of acquired superhuman abilities. OsCorp was given what little remained of the aborted 'Captain America' project and were allowed blood samples of the Fantastic Four (without informing them of the project) with the intent to make a serum that could be issued to the American Armed forces.
The Ideal soldier would not need repeated injections or continued recruitment into the service. The ideal soldier could be molded and bred into the position, with different genetics generating different powers, the best of which would breed further more perfect beings. Beings that could be controlled, slaughtered, and mass produced.
Leading this project was Sergeant William Stryker, an activist in the anti-mutant movement and driving force into mutant studies, particularly the X-gene, and was overseeing the production at OsCorp as an extension of the Weapon Plus Program. Alongside him was Dr. Miles Warren, a prolific geneticist and a biochemistry professor at Empire State University who was renowned for his cloning capabilities of various animals which furthered genetic studies.
Warren's participation within the program was primarily to avoid jail time as he had been caught hoarding genetic samples of his students, particularly those who were minors with varying degrees of clones in varying states of growth in violation of several ethical principles (Respect for autonomy, Nonmaleficence, Merit and integrity, etc).
The research had gone on for years with varying breakthroughs and setbacks. The most prominent, by the time of 2003, had to do with maintaining a sterile environment as trials of varying serums, radiology, gases, etc made retrials costly and difficult to sterilize.
Because of this, a lone spider began to build its web in a radiograph generator and survived the experiment.
it wasn't until the spider had escaped, free within the vents of the building that the webbing was found, having affected the test subject mortally. The spider however, as researchers feared, was nowhere to be seen.
The spider made its way to the top floor where Peter and Harry were playing.
it dropped down onto Peter, and bit him. The bug was cast off, and forgotten about, with the bite simply itching and Peter scratching at the spot, further spreading the venom into his body.
Peter returned home to Anna Watson and became sick the next day, suffering a fever of 110 degrees, with nausea, and lethargy. he recovered within 24 hours and soon began to develop powers at eleven years old.
it wouldn't be until he turned fourteen that he would have any semblance of mastery over them. he has told no one of this development, especially his disabled Aunt May, who suffered with a heart condition and was most decidedly anti-mutant and anti-super-ablest.
he could only remember what his Uncle had once said: with Great Power comes Great responsibility.
2 notes
·
View notes
Note
I wonder what would happen if you took all the viruses, t/g/veronica/c/urobuoros, a plagas, and maybe the mold and infected someone with all of them. Probably be messy but interesting.
"Well,
I have the Progenitor Virus - which is a unique strain of the T-Virus, Uroboros, and a prototype virus.
Las Plagas was the base for Uroboros, and from what I have gathered the mold was the base for the T-Virus.
The T-Veronica was completely wiped out and not further samples exist. So there's no possible way of including it in this concept outright.
That being said if you injected them all at once the results would be fatal.
It would overwhelm the body cause the subject immense pain followed by mutation and death. I doubt any sort of trial would be successful. Each one of those strains has a very high fatality rate on it's own - combining them would result in a 0% survival rate. Even if the test subject was a perfect adapter the stress of the mutations occurring all at once would likely kill them anyways.
Think of it like having a bad flu, plus measles, plus meningitis - all at once.
If you even survived through the night you'd be unlikely to walk away from that. It's stressful on the body to battle one viral infection - let alone several. "

1 note
·
View note
Video
youtube
The masterful design of the two-liter plastic soda bottle
Apr 11, 2025 Bill explains how the two-liter plastic soda bottle begins as a plastic tube, called a preform, which is heated and inflated with air in a bottle-shaped mold. He explains how the stretching of the preform creates a crystalline regions in the bottle's plastic (polyethylene terephthalate) that create a bottle with great strength, low permeability to carbon dioxide, but which is also lightweight—some 35 times lighter than a glass bottle of the same size. Bill explains key features of the bottles design, including: why the bottle looks like it does, why the neck has gaps in its threads, and how the tamper-proof ring works. He also discusses "hot-fill bottles" used for sports drinks and plastic juice bottles, noting the panels molded into the bottles to accommodate temperature changes. Lastly, he discusses briefly the recycling of PET bottles, although noting that about 75% of the 500 billion PET bottles manufactured annually end up in landfills or are incinerated.
Video summary 00:04 Preform Plastic soda bottles begin as preforms (small tubes) created by injection molding. This preform is heated slightly, then inflated to create a bottle. (See Bill's video on injection molding: • Plastic Injection Molding ) 00:31 Inflation in a mold In manufacturing the preform is inflated in a mold in two steps — by a blast of air at 100 PSI initially, then a blast at 580 PSI — to form the bottle in a fraction of a second. 01:13 Cost of air The energy to generate the compressed air is the largest cost after the plastic itself. 01:31 Stretching strengths plastic A plastic soda bottle is strong enough that it will neither burst nor deform when dropped from two meters onto a concrete floor 02:19 Pressure from carbonation The bottle must also be strong to contain the carbon dioxide dissolved in the soda, which exerts a pressure of about 70 psi/4.8 bar. 02:37 Strength created by stretching the plastic A plastic soda bottle is made from PET, polyethylene terephthalate. When the bottle is stretched small crystalline regions are formed, which are oriented in the two directions of stretch: along the axial and hoop directions. This orientation of the crystalline regions (about 25% of bottle's wall) increases the strength of the plastic. 03:36 Other plastics too weak The plastic used in a soda bottle is strong enough to keep the carbonation of the soda from distorting it, unlike a plastic milk jug, which is made from a different type of plastic (high density polyethylene). 03:58 Barrier to permeation of CO2 The stretch induced crystallization reduces carbon dioxide leakage: a 2 liter plastic soda bottle loses 15% of its carbonation in six weeks. Although this isn't as good as a glass bottle, it's good enough. 04:40 Shape of the bottle The bottle has a hemispherical shape at the top so the pressure is distributed evenly on the wall. Bottles from the 1970s had hemispherical bottoms, which were covered with a plastic cap so the bottle could sit on the table. 05:20 Petaloid bottom To get rid of the cap, manufacturers invented a "petaloid" bottom — meaning like a flower. This petaloid bottom provides stability while maintaining strength. 05:36 Neck of the bottle The bottle neck features specific engineering elements: a lip for the mold, a beveled lip for the tamper-proof ring, and discontinuous threads to release pressure. Engineers have reduced the neck size to save plastic (about 2g per bottle). 06:59 Tests on neck To engineer a new neck for the bottle engineers used trial and error. They subjected the neck to many tests. 07:39 Hot fill bottles "Hot-fill" bottles are designed for beverages requiring higher fill temperatures. They have special panels to accommodate contraction and expansion. 09:39 Patent disputes Patent disputes in the 1990s delayed implementation of the petaloid and hot-fill bottle bottom designs. 11:07 Recycling & disposal Approximately 500 billion PET bottles are manufactured yearly; about 75% end up in landfills or are incinerated. Yet, PET is recyclable and can be converted into fibers for fabrics used in sports jerseys and car upholstery. 12:03 End credits & blooper
1 note
·
View note
Text
Why Internal Lubricants Matter in PVC Processing – Industry Secrets Uncovered

The plastic manufacturing sector operates with polymers twisted by steel under pressure and heat, but small adjustments lead to significant effects. The influence of internal lubricants in PVC manufacturing operations exceeds the acclaim of other manufacturing elements despite their low public recognition. A deep exploration follows to prove how a PVC lubricant, including its internal form, functions as an essential unappreciated player throughout your entire production process.
Understanding the Science Behind Internal Lubricants and Their Role in PVC Formulations
PVC as a natural polymer, exhibits behaviour equivalent to that of a maintenance-intensive being in response to strong shearing forces. Processing machines function at high temperatures where friction develops from invisible to recognizable enemy status. Internal lubricants perform as microscopic peacekeepers to support PVC materials in various manufacturing situations. Internal substances enter the matrix structure to adjust molecular activity while decreasing internal drag without moving toward outer layers. Internal lubricants differ from external agents since internal agents penetrate deep inside structures. They modify inter-particle interactions. These additives perform like facilitators because they enhance chain movement and optimize melt flow, and preserve thermal stability with mechanical performance. Modern PVC lubricants that demonstrate value usually employ this fundamental principle.
The Untold Benefits of Using Internal Lubricants in Rigid PVC Applications
Rigid PVC compounds demand more control. Whether extruding profiles, calendaring sheets or injection molding fittings, thermal and mechanical stability becomes key. An internal lubricant PVC formulation influences processing ease in several ways. First, it fine-tunes the melt viscosity. This helps maintain smooth flow through dies and molds without causing scorch or build-up.Second, the right PVC lubricant reduces torque load on machinery. This doesn’t just preserve equipment—it saves money, cuts downtime and extends service cycles. Furthermore, internal agents aid in better pigment dispersion, granting finished products cleaner tones and uniform appearances. You wouldn’t want blotchy piping or color-bleeding wall panels, right?Also, critical—dimensional accuracy. Internal lubricants help avoid unwanted swelling or shrinkage, keeping tolerances within tight margins. Precision like this separates high-grade production from cheap knock-offs.
Avoiding Common Pitfalls in PVC Production by Leveraging Internal Lubricants Properly
Problems often arise when there's too much of a good thing. Overdosing an internal lubricant for PVC might lead to plasticization issues. You get unpredictable flow, sticky behaviours or even delamination in layered structures. Likewise, underdosing sacrifices flow stability and promotes high shear stress, which could degrade resin prematurely.Compatibility matters too. Not every internal additive meshes well with every polymer grade. A mismatch creates phase separation or migration, where lubricants ooze out and disrupt downstream performance. Thus, a finely balanced selection process becomes vital.Processors must consider processing temperature, shear rates, die geometry and the physical properties expected from end-products. Choosing an appropriate lubricant PVC blend requires trial runs, data scrutiny and perhaps even gut instinct built from years on the factory floor.
How Internal Lubricants Outperform Traditional External Solutions in PVC Operations
External lubricants serve well in demolding and surface smoothness, but they’re transient by nature. Heat and time draw them to the surface, where they either evaporate, smear or leave residues. Internal lubricants stick around longer. They interact chemically or physically with the polymer chains, ensuring consistent flow behaviour across long production cycles.This deeper interaction is vital for products that require enduring strength, thermal resistance or clarity. For example, transparent sheets or pressurized piping rely on this molecular harmony. Only an internal lubricant for PVC ensures that balance remains unshaken.Moreover, internal agents won’t interfere with secondary operations—welding, printing, coating—all of which could be compromised by surface-active additives. That seamless compatibility makes them a smarter choice in complex manufacturing chains.
Too often overshadowed by flashy fillers or stabilizers, internal lubricants form the invisible backbone of efficient PVC processing. These agents don’t shout, yet their impact echoes through every inch of pipe, panel or profile churned out of an extruder. Understanding how and why a PVC lubricant works beneath the surface gives manufacturers a hidden edge—a stealthy advantage in a competitive market.Mastering the right use of lubricant PVC technologies doesn’t just solve problems. It elevates products, safeguards equipment and secures long-term performance. That’s a secret worth uncovering.
0 notes
Text
Drain, Seal, Protect: Your Step-by-Step Wet Basement Fix Guide
A wet basement isn’t just a nuisance—it can quickly turn into a costly headache. From mold growth to foundation damage, water intrusion in your basement can threaten both your home’s value and your family's health. The good news? With the right approach, you can fix it—and prevent it from coming back. Here's your step-by-step wet basement repair guide to help you drain, seal, and protect your home.
Step 1: Drain the Water
The first priority is getting rid of any standing water. Use a wet/dry vacuum or a sump pump if flooding is significant. Be sure to wear protective gear and ventilate the area if mold is present.
Once the water is removed, run fans and dehumidifiers to fully dry out the space. The faster you act, the less damage moisture can cause.
Step 2: Identify the Source
Before jumping into repairs, figure out where the water is coming from. Common causes include:
Cracks in foundation walls or floors
Poor exterior grading directing water toward the home
Clogged or damaged gutters and downspouts
Failing sump pump systems
A professional inspection will provide a clearer picture and help you avoid trial-and-error fixes.
Step 3: Seal and Waterproof
After identifying the issue, it’s time for repairs. Depending on the problem, your solution may include:
Sealing foundation cracks with epoxy or polyurethane injections
Installing or upgrading a sump pump system
Adding interior or exterior waterproofing membranes
Replacing or installing proper drainage (e.g., weeping tiles)
A comprehensive wet basement repair strategy tackles both the symptoms and the source.
Step 4: Protect for the Future
Preventative maintenance is key. Ensure gutters are cleaned regularly, downspouts extend at least 6 feet away from your foundation, and your yard is properly graded. Test your sump pump system annually and consider a backup battery in case of power outages.
Conclusion: Fix It Right the First Time
A wet basement can quickly spiral into a major issue if left unchecked. Acting fast and following the right steps—drain, seal, protect—can save you money, protect your investment, and ensure your home stays safe and dry. If you're unsure where to begin or need long-term solutions, trust the team at Basement Waterproofing System for expert wet basement repair services across the GTA
0 notes
Text
Why Design for Injection Molding (DfIM) Is Critical for High-Quality Plastic Parts
Imagine investing months into designing a groundbreaking plastic component—only to discover during production that it warps, cracks, or simply can’t be molded efficiently. This nightmare scenario is all too common when Design for Injection Molding (DfIM) is overlooked. In industries ranging from medical devices to automotive manufacturing, achieving high-quality plastic parts isn’t just about aesthetics or functionality—it’s about ensuring every step of the process, from design to production, aligns seamlessly.
Design for Injection Molding (DfIM) is the secret weapon that transforms good ideas into great products. By integrating principles like plastic part design optimization and leveraging expert injection mold design services, DfIM ensures your parts are manufacturable, cost-effective, and built to last. In this in-depth guide, we’ll explore why DfIM is indispensable, how it applies to specialized fields like DFM for medical devices, and why partnering with a design and manufacturing company—such as Aprios—can make all the difference. Let’s get started.
What Is Design for Injection Molding (DfIM)?
At its core, Design for Injection Molding (DfIM) is a strategic approach within the broader discipline of Design for Manufacturing (DFM). It focuses on tailoring plastic part designs specifically for the injection molding process—one of the most versatile and widely used methods for producing precise, repeatable components. DfIM isn’t just about making a part look good on paper; it’s about anticipating how that design will behave when molten plastic is injected into a mold under high pressure.
The process begins with understanding the unique demands of injection molding: material flow, cooling rates, and mold release. Whether you’re crafting a simple consumer product or a complex component requiring DFM for medical devices, DfIM ensures your design is optimized for production. This might mean adjusting wall thickness, adding draft angles, or rethinking feature placement—all part of plastic part design optimization.
Industries like healthcare, automotive, and electronics rely on DfIM to bridge the gap between creativity and practicality. By working with plastic injection mold design services, companies can avoid costly redesigns and bring their products to market faster. In short, DfIM is the foundation of efficient, high-quality plastic manufacturing.
Why DfIM Is Critical for High-Quality Plastic Parts
Injection molding is a high-stakes game. With pressures reaching thousands of PSI and cycle times measured in seconds, there’s little room for error. A poorly designed part can lead to defects like sink marks, warping, or incomplete fills—issues that compromise both quality and profitability. Here’s why Design for Injection Molding (DfIM) is essential for delivering high-quality plastic parts:
Defect Prevention: Uniform wall thickness is a cornerstone of DfIM. Uneven walls can cause uneven cooling, leading to stresses that distort the final part. Proper draft angles—slight tapers on vertical surfaces—make ejection easier and reduce surface imperfections. These details, often refined through injection mold design services, are what separate a flawless part from a flawed one.
Material Efficiency: Choosing the right resin is only half the battle; DfIM ensures the design maximizes that material’s potential. For instance, a part with excessive thickness wastes material and increases cooling time, while a thin, optimized design—crafted with design for manufacturing solutions—balances strength and efficiency.
Cost Savings: Every iteration of a mold or design tweak costs time and money. By incorporating DFM services early, DfIM minimizes trial-and-error, reduces scrap rates, and shortens production timelines. The result? Lower costs without sacrificing quality.
Consider a real-world example: a manufacturer designing a plastic housing for an electronic device. Without DfIM, they might overlook shrinkage rates, leading to parts that don’t fit their assemblies. With DfIM, they’d account for this upfront, ensuring a perfect fit every time. Across industries, high-quality plastic parts owe their success to this meticulous approach.
DfIM in Action – Key Principles and Best Practices
To truly harness the power of Design for Injection Molding (DfIM), designers must adhere to a set of proven principles. These aren’t just guidelines—they’re the building blocks of manufacturability. Here’s a closer look at the essentials, along with practical applications:
Avoid Sharp Corners and Undercuts: Sharp edges disrupt resin flow and create stress concentrations that can crack under load. Rounding corners improves mold filling and strengthens the part. Similarly, undercuts—features that trap the part in the mold—complicate ejection. Injection mold design services can suggest alternatives, like side actions or redesigns, to keep things simple.
Optimize Ribs and Bosses: Ribs add strength without excessive material, but they must be thin (typically 50-60% of wall thickness) to avoid sink marks. Bosses, used for screws or inserts, need proper spacing and support. These tweaks, part of plastic part design optimization, ensure durability without overcomplicating the mold.
Incorporate Proper Tolerances: Injection molding requires tighter tolerances than, say, 3D printing. DfIM aligns tolerances with the process’s capabilities—typically ±0.005 inches for precision parts. Collaborating with plastic injection mold design services ensures these specs are realistic and achievable.
Take a plastic gear as an example. A DfIM-optimized design might feature rounded tooth profiles, consistent thickness, and a slight draft for easy release—making it both functional and mold-friendly. These best practices, refined through design for manufacturing solutions, turn concepts into production-ready realities.
DfIM for Specialized Applications (e.g., Medical Devices)
When precision and reliability are non-negotiable, DFM for medical devices elevates DfIM to a whole new level. Medical components—like syringe barrels, catheter housings, or implant casings—must meet stringent regulatory standards (e.g., FDA or ISO 13485) while performing flawlessly in life-or-death scenarios.
Here, DfIM goes beyond basic manufacturability. It considers biocompatibility (ensuring materials won’t harm patients), sterilization compatibility, and microscopic tolerances. For instance, a poorly designed mold draft could leave burrs on a surgical tool, posing a contamination risk. DFM development services address these challenges by integrating regulatory insights into the design phase.
A practical case: designing a plastic inhaler component. DfIM ensures airtight seals and smooth surfaces for patient safety, while design for manufacturing services validate the design against medical standards. This specialized focus makes DfIM indispensable for industries where failure isn’t an option.
How Design and Manufacturing Companies Support DfIM
Bringing a plastic part from sketch to shelf is no small feat, and that’s where a design and manufacturing company shines. Companies like Aprios offer a suite of DFM services that amplify DfIM’s impact:
Plastic Injection Mold Design Services: Crafting molds tailored to your part’s geometry, material, and production volume. A well-designed mold can handle millions of cycles without wear.
Prototyping and Testing: Before committing to full production, prototyping—often via 3D printing or soft tooling—lets you validate DfIM principles. Adjustments here save headaches later.
DFM Analysis: Using simulations and expertise, injection mold design services identify potential issues—like resin flow bottlenecks or cooling inefficiencies—early in the process.
For example, a company developing a plastic automotive bracket might turn to design for manufacturing solutions to refine rib placement and test mold durability. The result is a part that’s lighter, stronger, and cheaper to produce. With expert support, DfIM becomes a competitive edge.
Common DfIM Mistakes to Avoid
Even seasoned engineers can trip over DfIM pitfalls without proper guidance. Here are the most common mistakes—and how DFM services can help you steer clear:
Ignoring Shrinkage Rates: Different plastics shrink at different rates (e.g., ABS at 0.5-0.8%, nylon at 1-2%). Failing to adjust for this distorts dimensions. Plastic injection mold design services calculate shrinkage upfront.
Overcomplicating Designs: Adding unnecessary features—like intricate textures or redundant supports—increases mold complexity and cost. Simplify with plastic part design optimization.
Skipping DFM Reviews: Rushing to production without a design for manufacturing services review risks missing flaws—like inadequate venting—that cause defects.
Picture a phone case with an overly elaborate logo. Without DfIM, it might stick in the mold or show burn marks from trapped air. A quick DFM check could streamline the design, saving thousands in rework. Prevention, powered by expertise, is always cheaper than correction.
Conclusion
Design for Injection Molding (DfIM) isn’t just a step in the process—it’s the backbone of producing high-quality plastic parts that meet performance goals and budget constraints. From DFM for medical devices to everyday consumer goods, DfIM ensures your designs are manufacturable, efficient, and built to impress. By embracing plastic part design optimization, avoiding common pitfalls, and tapping into design for manufacturing solutions, you’re setting your project up for success.
At Aprios, we specialize in turning your ideas into reality with expert plastic injection mold design services and comprehensive DFM development services. Don’t leave quality to chance—contact us today to discuss your next project. Let’s collaborate to create something extraordinary, from concept to completion.
#Design for Injection Molding (DfIM)#DFM for Medical Devices#Plastic Part Design Optimization#design and manufacturing company#plastic injection mold design services#injection mold design services#dfm services#design for manufacturing solutions#dfm development services#design for manufacturing services
0 notes
Text
Medical Device Manufacturers: Driving Innovation in Healthcare

The Importance of Medical Device Manufacturing
Medical device manufacturers play a crucial role in the healthcare industry by developing and producing life-saving equipment and tools. These manufacturers create devices ranging from surgical instruments and diagnostic machines to implantable medical devices and wearables. The accuracy, reliability, and compliance of these products are essential for patient safety and effective treatment. As the demand for advanced healthcare solutions continues to grow, medical device manufacturing remains at the forefront of technological advancements.
Precision and Compliance in Manufacturing
Manufacturing medical devices demand strict compliance with international standards like ISO 13485 and FDA guidelines. Precision is paramount, as even the smallest defect can compromise patient safety. Advanced manufacturing techniques, including CNC machining, injection molding, and 3D printing, help achieve the required accuracy and consistency. Additionally, manufacturers must ensure rigorous quality control processes, including testing and validation, to guarantee that products meet industry standards.
Materials and Technologies in Medical Devices
Medical device manufacturers utilize a variety of materials, including biocompatible metals, plastics, and ceramics, to produce durable and safe medical equipment. Titanium, stainless steel, and medical-grade polymers are commonly used in implants and surgical instruments. Cutting-edge technologies such as artificial intelligence (AI), robotics, and nanotechnology are also being integrated into medical devices, leading to more effective treatments and improved patient outcomes. These advancements enable the production of highly sophisticated devices, such as robotic-assisted surgical systems and smart diagnostic tools.
Challenges in the Medical Device Industry
Despite significant advancements, medical device manufacturers face several challenges, including stringent regulatory requirements, high production costs, and the need for continuous innovation. The approval process for new devices can be lengthy and complex, requiring extensive testing and clinical trials. Additionally, manufacturers must address supply chain disruptions, cybersecurity threats, and the rising demand for personalized medical solutions. Overcoming these challenges requires investment in research, development, and collaboration with healthcare professionals.
The Future of Medical Device Manufacturing
Emerging technologies and an increased focus on patient-centric care are driving the future of medical device manufacturing. Innovations such as wearable health monitors, remote patient monitoring systems, and AI-driven diagnostics are revolutionizing the industry. Additionally, advancements in additive manufacturing and bioprinting hold the potential to create customized implants and prosthetics tailored to individual patient needs. As technology continues to evolve, medical device manufacturers will play a pivotal role in shaping the future of healthcare.
Conclusion
Medical device manufacturers are essential in advancing healthcare by producing high-quality, innovative, and life-saving medical equipment. Through precision engineering, compliance with regulations, and technological integration, they contribute to better patient care and improved medical outcomes. As the industry continues to grow, embracing new technologies and overcoming challenges will be crucial in ensuring that medical device manufacturing remains a key driver of healthcare innovation.
0 notes
Text

The manufacturing process of injection molds involves several steps. Here is a general outline of the process:
1. Design Phase
Requirements Analysis: Confirm specifications, functions, and material requirements of the product.
CAD Design: Use computer-aided design software (such as SolidWorks, AutoCAD, etc.) to create detailed designs, including 3D models and 2D engineering drawings.
2. Material Selection
Mold Steel: Choose suitable mold materials based on product characteristics, commonly used materials include P20, H13, S7, among others.
Surface Treatment: Consider treatments for wear resistance and corrosion resistance, such as nitriding or chrome plating.
3. Machining Stage
Rough Machining: Use CNC machines for initial cutting to form the basic shape of the mold.
Finishing Machining: Further refine the mold to improve precision and surface finish.
Heat Treatment: Apply heat treatment to the mold steel to enhance hardness and durability.
4. Assembly and Testing
Assembly: Assemble various components of the mold, including cavities, cores, and guide pillars.
Testing: Conduct trial molding to check forming effects and fit, making adjustments as necessary.
5. Finalization and Maintenance
Inspection: Perform a comprehensive inspection of the mold to ensure it meets design specifications.
Maintenance: Establish a maintenance plan to prolong the lifespan of the mold.
Considerations
Cost Control: Consider costs during the design and manufacturing processes to ensure economic efficiency.
Technology Updates: Continuously update equipment and processes with technological advancements to improve production efficiency and product quality.
Through these steps, injection molds are completed and put into use.
1 note
·
View note
Text
Precision Molding Excellence: Exploring 2K Injection Molding and Two-Shot Injection Molding Companies
Introduction:
In the realm of injection molding, companies specializing in 2K injection molding and two-shot injection molding play a crucial role in delivering complex and precise components. This article explores the capabilities and advantages offered by these specialized manufacturing processes, highlighting leading companies that excel in 2K injection molding and two-shot injection molding.
Part 1: 2K Injection Molding Companies
Part 2: Two-Shot Injection Molding Companies
Conclusion:
Companies specializing in 2K injection molding and two shot injection molding companies are at the forefront of delivering precision, versatility, and innovation in the injection molding industry. By understanding the unique capabilities of these processes and recognizing the expertise of leading companies, manufacturers can make informed choices to meet the demands of complex component production. This comprehensive guide serves as a valuable resource for those seeking excellence in 2K injection molding and two-shot injection molding solutions.
#injection mold tooling cost#injection mold surface finish#2k mold#injection mold trial#molding#plastic injection molding cost#insert overmolding#injection molding#two shot mold#injection mold prototype
0 notes
Text
How to Improve the Quality of Plastic Injection Molds?
Do you want to make high-quality plastic parts with injection molding? Curious about how mold quality impacts the final product? What can you do to ensure your molds give the best results? Let's check out the factors to improve the quality of your plastic injection molds.
Plastic injection moulding is a key method used to manufacture everything from little plastic parts to significant components. High-quality moulds are important for achieving the greatest outcomes. The quality of the mould has a direct influence on the finished product, from its appearance to its strength and durability. Here are some practical methods you may take to boost the quality of plastic injection moulds.
1. Choose the Right Material
The quality of your mould begins with the material you employ to create it. The mould material should be strong and resistant to damage, especially if you're making a big number of pieces. Hardened steel, stainless steel, and aluminium are examples of commonly used materials. Steel is more costly, but it has more durability and can generate higher-quality parts over time.
2. Design with Precision
A well-designed mould is necessary to deliver high-quality results. Pay particular attention to design elements like wall thickness, cooling channels, and draft angles. Proper design ensures that the plastic flows smoothly throughout the mould, lowering the possibility of flaws such as warping or partial filling. Use modern CAD (Computer-Aided Design) equipment to generate accurate and detailed designs.
3. Use High-Quality Injection Machines
The quality of the injection moulding machine has an important impact on the overall quality of the mould. Ensure that your machines are properly maintained and calibrated. Machines with upgraded controls can deliver more constant pressure and temperature, which are critical for making high-quality moulds.
4. Implement Rigorous Quality Control
Quality control is essential at every stage of the injection molding process. Regularly inspect molds for wear and tear and check the finished products for any defects. Using tools like Coordinate Measuring Machines (CMM) can help in accurately measuring parts and ensuring they meet specifications.
5.Optimize the Cooling Process
Cooling is a critical phase in injection molding that affects the quality of the final product. Uneven or inadequate cooling can lead to defects such as warping or shrinkage. To optimize cooling, ensure that the cooling channels in the mold are well-designed and that the coolant flows efficiently throughout the mold. Using conformal cooling techniques, where cooling channels are designed to follow the shape of the part, can further enhance the cooling efficiency.
6. Use Proper Mold Maintenance
Regular maintenance of molds is key to extending their life and ensuring consistent quality. Clean the mold regularly to remove any residues or buildup. Also, inspect the mold components like ejector pins and slides to ensure they are functioning properly. Lubricate moving parts to prevent wear and replace any worn-out components promptly.
7. Control Injection Parameters
The parameters used during the injection process, such as temperature, pressure, and injection speed, significantly affect the quality of the molded parts. Fine-tuning these parameters can help reduce defects and improve overall product quality. Consider conducting trials to determine the optimal settings for your specific mold and material.
To improve the quality of plastic injection molds, use the right materials, design them accurately, use good machines, and maintain strict quality control. Regular maintenance and having skilled workers also help in making high-quality molds. By paying attention to these factors, you can produce parts that meet or exceed your quality expectations.
If you would like to discuss your requisites with one of our experts. You can submit you files here and our team will contact you as soon as possible.
0 notes
Text
Forming Processes for Ceramic Substrates
Ceramic substrates are generally ultra-thin sheets below 1mm or even around 0.3mm. Forming and sintering are both key difficulties in preparation. The flatness, surface roughness, and dimensional stability of ceramic substrates are key factors that affect the subsequent preparation of copper cladding and circuit etching of the substrate. This places high demands on the forming process of ceramic substrates. At the same time, mass production also requires the forming method to have high production efficiency and low cost.
1. Tape casting process
Tape casting is currently the most important method for forming thin films or sheets.
The basic process of ceramic substrate tape casting is preparing tape casting slurry, vacuum degassing, tape casting, sintering, etc. Tape casting has the dual advantages of high production efficiency and ultra-thinness, but due to the low density of the green body, it is easy to deform when preparing large-sized substrates. The powder forming process combining tape casting and isostatic pressing is used to increase the density of the ceramic substrate tape casting green body, further, reduce the shrinkage of the green body during sintering, and help obtain large-sized high thermal conductivity ceramic substrates.
2. Dry pressing
Dry pressing is to put powder into a mold and apply pressure through a press to press the powder into a green body of a certain shape. The dry pressing process is simple, suitable for mass production, and has a short cycle. The green body has high density, relatively precise size, small firing shrinkage, high mechanical strength of the ceramic parts, and good electrical properties. Dry pressing is suitable for pressing round and thin sheet products. The product has high density and the flatness of the substrate is easy to ensure, but it is difficult to prepare ultra-thin substrates.
3. Gel injection molding
Gel injection molding is to disperse ceramic powder in an aqueous solution or non-aqueous solution containing organic monomers and cross-linking agents to prepare a concentrated suspension with low viscosity and high solid volume fraction (>50vol%), then add initiators and catalysts, inject the suspension into the mold, and initiate the polymerization of organic monomers under certain temperature conditions, resulting in in-situ solidification molding. After low-temperature drying, a green body with high strength and machinability is obtained. Gel injection molding is suitable for the molding of ceramic substrates with a thickness of 0.6 to 10 mm, but the degree of automation and molding efficiency is relatively low. In mass production, the slurry's gravity is generally used as a power source to inject into the mold. The process has high requirements on slurry fluidity, flow mode of injection into the mold, gel time control, green body drying uniformity, etc., and quantitative control is difficult. There are currently few examples of mass production of gel injection molding for substrate molding.
4. Additive Manufacturing 3D Printing
3D printing gets rid of the limitations of molds on traditional molding. In today's increasingly competitive market, 3D printing can achieve frequent product trials and modifications, which has incomparable advantages over conventional processing methods.
0 notes
Text
Precision Injection Molding: Features and Advantages
Precision Injection Molding: Features and Advantages
The precision injection molding is a process of manufacturing that focuses on accuracy when making plastic components. It is necessary for industries with delicate pieces like electronics, automotive, medical devices and consumer goods among others. Below are some key points about precision injection molding:Get more news about plastic injection mold,you can vist our website!
High dimensional accuracy: The strictest tolerance levels can be achieved by using precision injection molding hence parts would have minimum deviation from their ideal sizes which must fit well with other components such as those found in automotive applications or medical devices.
Complex geometries: There are no limits to what shape you want to make out of your product since this method allows for creation of any form however complicated it may seem so long as all details are included during designing stage; without doubt other techniques cannot produce such results even if they were applied together in one process or separately after each another because none has ability to do so alone.
Uniform quality: Through carrying out many trials then settling on the best one ensures that all parts manufactured within wide production runs look alike i.e., same design, color, size etc.; therefore customers will always get identical goods whenever they place orders for large quantities thereby reducing chances of getting defective items due to wrong specifications provided by client which would have led into low reliability rate among users.
Material flexibility: A lot of thermoplastics can be used here while high performance engineering resins fall under these too; thus it becomes possible for manufacturers selecting most suitable materials basing on what is needed by a particular application vis-à-vis strength requirement flexi needs chemical resistances temperature resistance balance.
Productivity and cost-effectiveness: It might be expensive at first setting up things but once everything has been put in place then mass production takes over making each unit cheaper hence more money saved during manufacturing process compared with other methods where only few units could have been produced within same period leading higher per item cost plus longer hours used .
Surface finish/looks: This method ensures that articles made have smooth surfaces which do not need further polishing after being removed from moulds; this feature is important especially when dealing with consumer goods because people buy items depending on how attractive they look like.
0 notes
Video
youtube
2 component toothpaste tube flip cap mold
Bi injection technology can be applicated to the toothpaste tube packaging of the caps. By this way, no need to do the heat seal of the aluminized paper on the tube bottle mouth part. Now we YUEYI did trialed on the 2k shampoo cap mold, and achieved the good result. China 2k mold maker, offer 2 component toothpaste cap mold, bicolors HDPE tube bottle cover in mold close, multi shot toothpaste topper mold, rotary mold squeezer bottle flips mold.
Web: www.yueyimold.com WhatsApp& WeChat: +86 183 5761 6586 Mail: [email protected]
#china mold#2 component mold#bi material mold#two color toothpaste cap mold#2k hdpe tube bottle cover mold#multi shot toothpaste topper mold#double squeezer bottle flips mold
0 notes
Text
Bulk Moulding Compound Revolutionizes Manufacturing Processes
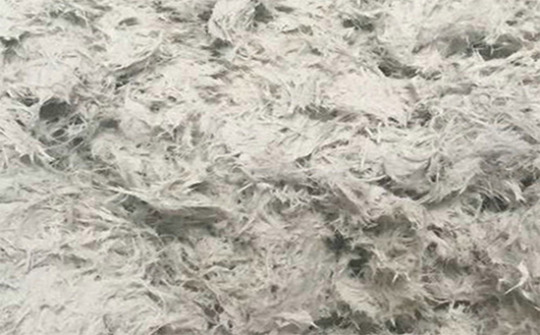
Key Developments
Precision Mold Design: Manufacturers are investing in state-of-the-art mold design software and advanced machining technologies to create intricate and high-precision molds for BMC components. These molds ensure uniform material distribution, minimized defects, and enhanced surface finish, meeting the stringent requirements of modern applications.
Automated Molding Processes: Automation has revolutionized BMC molding operations, leading to faster production cycles, reduced labor costs, and improved consistency. Automated systems control material mixing, injection, compression, and curing processes with precision, resulting in superior part quality and increased throughput.
Advanced Material Formulations: Continuous research and development efforts have led to the formulation of BMC materials with enhanced mechanical properties, thermal stability, and flame resistance. Tailored formulations address specific application requirements, such as automotive under-the-hood components, electrical enclosures, and structural parts, expanding the versatility of BMC in diverse industries.
Integration of Sustainable Practices: With growing emphasis on sustainability, manufacturers are exploring eco-friendly additives and recyclable materials to enhance the environmental footprint of BMC products. Sustainable BMC formulations not only reduce waste but also contribute to regulatory compliance and corporate social responsibility initiatives.
Real-time Process Monitoring: Implementation of sensor-based monitoring systems allows real-time tracking of key process parameters during BMC molding. By monitoring variables such as temperature, pressure, and flow rates, manufacturers can identify deviations, optimize settings, and ensure consistent part quality, enhancing overall production efficiency.
Furthermore, collaboration between material suppliers, mold designers, equipment manufacturers, and end-users has fostered a collaborative ecosystem conducive to innovation and knowledge exchange. Through joint research projects, pilot trials, and technology transfer initiatives, stakeholders across the BMC value chain are driving continuous improvement and pushing the boundaries of what is achievable with composite materials.
Looking ahead, the outlook for BMC molding remains promising, with ongoing advancements poised to unlock new applications and markets. As industries embrace lightweight, durable, and cost-effective solutions, BMC stands out as a material of choice, offering unmatched performance and versatility in the ever-evolving landscape of composite materials.
0 notes
Text
Exploring the Art of Streamline Patterns on Vape Device Casings: A Dive into PC/ABS Material and Key Techniques

The aesthetic appeal of vape devices is just as vital as their functionality, with streamline patterns on their casings standing out as a prime example of innovative design. This article delves into the materials and techniques used to create these mesmerizing patterns, focusing on the popular PC/ABS material. 1. Understanding PC/ABS Material PC/ABS, a blend of Polycarbonate (PC) and Acrylonitrile Butadiene Styrene (ABS), is a thermoplastic that harnesses the strengths of both components. This alloy improves the heat resistance and tensile strength over ABS alone while reducing the melt viscosity of PC, easing the manufacturing process and enhancing the final product's low-temperature impact resistance and chemical durability. Widely used in automotive components, home appliance casings, and consumer electronics, PC/ABS is a versatile choice for vape device casings. 2. The Creation of Streamline Effects Also known as the marble-like texture, the streamline effect involves the use of high-melting-point color masterbatches during the extrusion process. These masterbatches, when blended with PC and ABS, flow at different rates due to their differing viscosities, causing the pigments to distribute irregularly and create the distinctive streamline effect. For instance, typical molding temperatures for PC/ABS range from 230-240°C. At these temperatures, standard color masterbatch carriers like PE (Polyethylene) or EVA (Ethylene-vinyl acetate) melt completely, leading to a uniform flow. However, using a high-melting-point carrier, such as PA66 which melts at 260°C, allows the pigments to remain partially unmelted at the molding temperatures, thus enabling adjustments in color depth and distribution by varying the masterbatch concentration and molding parameters. 3. Key Technical Aspects of Streamline Pattern Presentation Streamline patterning can present challenges such as incomplete dispersion of masterbatches, incompatibility between the masterbatch and the base material, and inconsistency in the pattern itself. For vape devices, the variety of possible colors — ranging from 20 to 30 different effects — depends on the specific masterbatch used, which in turn influences the appearance of the streamline pattern. Throughout the injection molding process, factors such as the proportion of masterbatch in the mix, molding temperature, injection parameters, and the position of the gate significantly affect the outcome of the streamline pattern. Here are some critical considerations: - Material Selection: Opt for a base material like ABS or PC/ABS that offers high fluidity and good impact resistance. - Pattern Presentation on Components: The dispersion of streamline masterbatches in the plastic base is random, affecting the pattern's location and orientation. The direction of the streamline pattern aligns with the flow of the plastic melt in the mold, and it cannot be altered by process adjustments alone; changes in the component's wall thickness might help guide the melt flow direction. - Gate Requirements: The gate design should accommodate the flow of streamline masterbatches, with a recommended minimum diameter of 0.8mm to prevent clogging, although increasing the temperature can alleviate some issues. - Process Adjustments: Follow specific guidelines for setting temperatures and be prepared to make multiple trials to determine if temperature adjustments are necessary. The streamline effect not only enhances the visual appeal of vape devices but also showcases the intricate possibilities of modern manufacturing techniques. By understanding and manipulating the properties of PC/ABS and the behavior of color masterbatches, manufacturers can create stunning, unique patterns that elevate the aesthetic value of their products. Read the full article
0 notes