#Microbial Fuel Cells
Explore tagged Tumblr posts
Text
Microbial Fuel Cells: Generating Clean Energy with Biotechnology
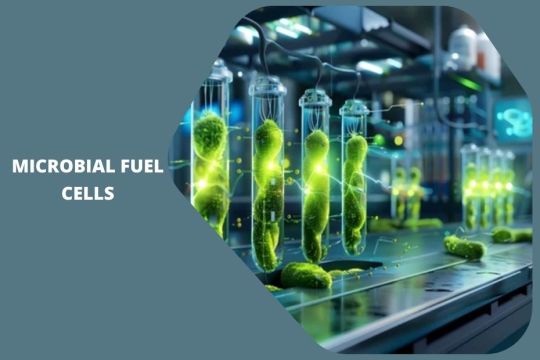
Microbial fuel cells (MFCs) are a remarkable innovation that brings together microbiology and technology to create clean, renewable energy. These systems generate electricity by utilizing the natural metabolic processes of microorganisms. MFCs are a promising solution for energy generation and hold potential for wastewater treatment and environmental restoration. Over the years, the progress in MFC technology has shown how sustainable energy and biotechnology can come together to address some of the world’s pressing challenges. This article explores the inner workings of microbial fuel cells, their applications, advantages, challenges, and future prospects.
What Are Microbial Fuel Cells?
At their core, microbial fuel cells are bio-electrochemical devices that convert chemical energy stored in organic matter into electrical energy through the activity of bacteria. These systems rely on microorganisms to break down organic material, releasing electrons as a byproduct of their metabolic activity. These electrons are then captured and directed to generate an electric current. The process involves an anode chamber where bacteria metabolize organic matter in an anaerobic environment, releasing electrons and protons. The electrons are transferred through an external circuit to the cathode, generating electricity, while protons pass through a proton exchange membrane to complete the reaction.
MFCs effectively mimic natural processes to transform waste into energy. By harnessing bacteria to process organic materials, they provide a sustainable and eco-friendly energy source. Their versatility allows for a wide range of applications, including energy generation, pollution management, and water purification.
How Microbial Fuel Cells Work
Microbial fuel cells rely on a few key components that work together to enable electricity generation. The first is the anode chamber, where microorganisms break down organic matter in an oxygen-free environment. This chamber is crucial because it fosters the growth and activity of bacteria that release electrons during their metabolic processes. These electrons travel through an external circuit, creating a flow of electricity before reaching the cathode.
The cathode chamber is where the final step of the reaction takes place. Here, electrons, protons, and oxygen come together, usually forming water as the end product. The separation between the anode and cathode chambers is maintained by a proton exchange membrane, which selectively allows protons to pass through while keeping the chambers chemically distinct. This design is essential for maintaining anaerobic conditions in the anode chamber and ensuring the system operates efficiently.
Applications of Microbial Fuel Cells
Microbial fuel cells offer a variety of applications, making them a versatile tool in both environmental management and energy production. One of their most notable uses is in wastewater treatment. By integrating MFCs into treatment facilities, organic pollutants can be broken down while simultaneously generating electricity, providing a dual benefit. This approach reduces the energy costs associated with traditional wastewater treatment methods while addressing environmental concerns.
Another significant application is bioremediation. MFCs can be used to clean up environments contaminated with hydrocarbons, heavy metals, or other pollutants. The bacteria in these systems are capable of breaking down harmful substances, contributing to the restoration of ecosystems. Additionally, MFCs are being explored in desalination, where they assist in removing salt from seawater. This offers an energy-efficient method for producing freshwater in areas facing water scarcity.
In addition to these applications, MFCs serve as biosensors. They can detect microbial activity or the presence of specific pollutants in water or soil. These sensors provide real-time data that can be critical for environmental monitoring and decision-making.
Advantages of Microbial Fuel Cells
The advantages of microbial fuel cells make them an attractive solution for clean energy and environmental management. One of their most notable benefits is the ability to generate renewable energy. Unlike fossil fuels, MFCs rely on organic materials as their energy source, reducing dependency on non-renewable resources and lowering greenhouse gas emissions.
MFCs also contribute to waste reduction. By converting organic waste into electricity, they address two significant issues simultaneously: energy generation and waste management. This makes them particularly valuable in industries that produce large amounts of organic waste, such as agriculture and food processing.
Another advantage is their environmental friendliness. MFCs produce minimal emissions and often contribute to environmental restoration efforts. For instance, when used in wastewater treatment, they clean the water and produce energy as a byproduct. Furthermore, their scalability allows them to be adapted for various applications, from small-scale biosensors to large industrial systems.
Challenges Facing Microbial Fuel Cells
Despite their potential, microbial fuel cells face several challenges that limit their widespread adoption. One of the primary issues is their relatively low power output. Compared to conventional energy sources, MFCs generate significantly less electricity, making them unsuitable for applications requiring high energy demands.
The cost of materials is another hurdle. The components of MFCs, including electrodes and membranes, are often made from expensive materials that increase the overall system cost. This presents a significant barrier to large-scale implementation, especially in resource-limited settings.
Scalability is another area where MFCs face difficulties. While they work effectively in small, controlled environments, scaling them up for industrial applications poses technical and economic challenges. The microbial efficiency, which is influenced by environmental conditions and the type of bacteria used, also affects the performance and reliability of these systems.
Future Prospects for Microbial Fuel Cells
The future of microbial fuel cells looks promising, with ongoing research and innovation aimed at addressing current limitations. Researchers are exploring cost-effective alternatives to traditional electrode materials and developing more efficient microbial communities to enhance performance. Advancements in system design, such as stacked MFCs, offer the potential to increase power output and scalability.
Integrating MFCs with other renewable energy technologies, such as solar and wind, could further expand their applications. For instance, hybrid systems could be developed to combine the strengths of multiple energy sources, making them suitable for diverse environments and needs. Additionally, expanding the use of MFCs in remote areas and off-grid communities could provide sustainable energy solutions where traditional infrastructure is unavailable.
Key Applications of Microbial Fuel Cells
Wastewater treatment: Break down pollutants while generating electricity.
Bioremediation: Clean up contaminants like heavy metals and hydrocarbons.
Desalination: Remove salt from seawater efficiently.
Biosensing: Detect pollutants and monitor microbial activities in real-time.
In Conclusion
Microbial fuel cells are a groundbreaking innovation at the intersection of biotechnology and sustainable energy. They hold immense potential not just for clean energy generation but also for addressing critical environmental challenges like waste management and pollution. While challenges such as low power output and scalability remain, ongoing research continues to refine and enhance these systems. With their ability to transform organic waste into electricity, MFCs exemplify the power of harnessing biology for technological solutions. By continuing to innovate and integrate this technology, we can move closer to a future where energy is clean, renewable, and accessible for all.
0 notes
Link
1 note
·
View note
Text
Microbial Fuel Cell Market Size, Share & Industry Analysis, By Type (Mediated, Unmediated), By End-User (Residential & Commercial, Industrial, Transportation, Military, Utilities, Others) and Regional Forecast, 2022-2029
0 notes
Link
#market research future#microbial fuel cells company#microbial fuel cell industry#microbial fuel cell analysis#microbial fuel cell report
0 notes
Text
I'm fascinated by microbiomes, the communities of bacteria, unicellular fungi, and other tiny living beings that populate various parts of animal bodies. We literally can't function without them; our gut micriobiome, for example, is crucial to our ability to digest food.
It's long been thought that the brain was kept sterile by the blood-brain barrier. However, this recent study shows that fish have a brain microbiome, so it's not entirely out of the realm of possibility we might, too. It's tough to study, because accessing the brain opens up the risk of outside contamination confounding the results of the study.
But if it is true, the implications could be huge. There's already a growing body of evidence that our gut microbiome has a significant impact on our mental health and mood through what's known as the Gut-Brain Axis. This is a series of connections between these parts of the body that includes the nervous, endocrine, immune, and other systems. Given there's about one microbial cell for every human cell in our bodies, it wouldn't be at all surprising that our microbial communities have a bigger influence on our lives than we think.
Because it's quite dangerous to study a living human brain, it will be difficult to determine whether we do have cranial microbiomes. But I'll be keeping my eye out for any further news along these lines.
#microbiome#microbes#microbiology#health#mental health#gut health#mental illness#brains#science#scicomm#nature#animals#wildlife#holobiont
131 notes
·
View notes
Text
Doing some brushing up studies before big plane maintenance exam #2 in a few days, and this section on corrosion flipped the Transformer fic/world building switch in my head👀👀
Might be a big reason why some Cybertronians are squeamish of organic organisms. Corrosion's not something a being made of metal would take lightly.
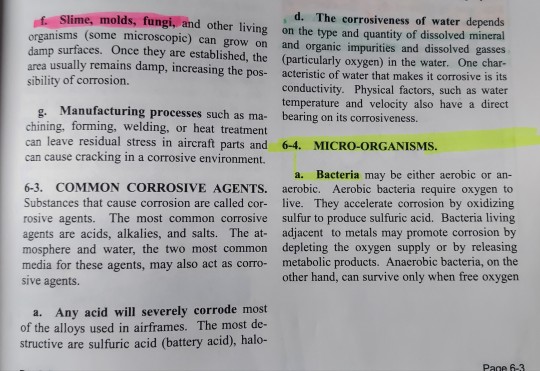
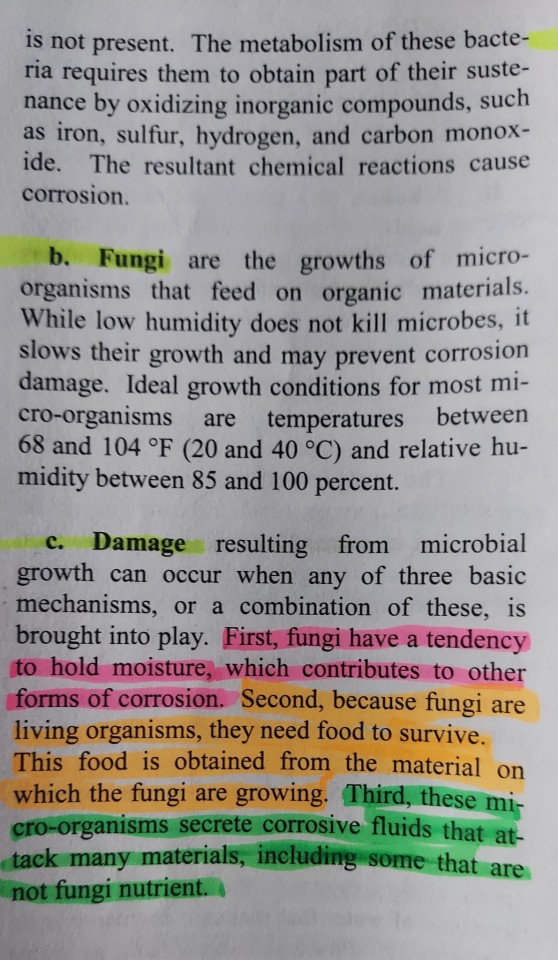
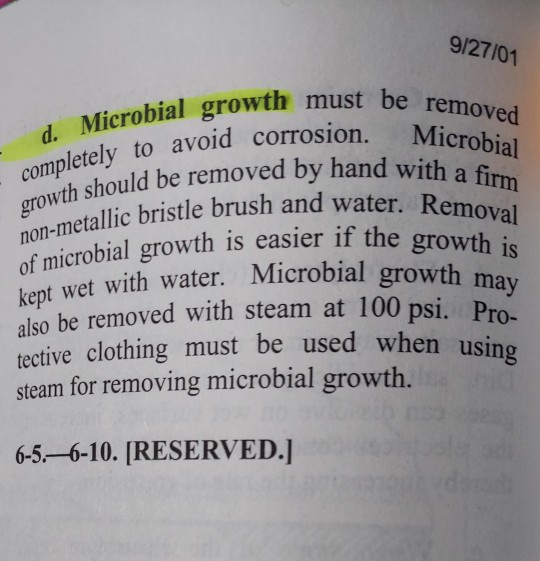
Also in aviation we have to keep an eye out for microbial critters in fuels too, jet fuel especially. There's a type of microbe that loves to live in jet fuel (it's got a high viscosity & holds water too easily). They collect with water at the bottoms of warm fuel tanks and can clog fuel filters, sumps, sensors, fuel lines, pumps, engines, everything- in addition to corroding everything metal in their path. Rubber seals and gaskets or rubber fuel tank cells don't fare too well either, the acids they produce can deteriorate those as well.
((Excuse my highlighting scribbling method of studying.. it helps me break up the text blobs :'3 ))
191 notes
·
View notes
Text
Scientists may have figured out why a potent greenhouse gas is rising. The answer is scary. (Washington Post)
Excerpt from this Washington Post story:
Almost two decades ago, the atmosphere’s levels of methane — a dangerous greenhouse gas that is over 80 times as potent as carbon dioxide in the short term — started to climb. And climb.
Methane concentrations, which had been stable for years, soared by 5 or 6 parts per billion every year from 2007 onward. Then, in 2020, the growth rate nearly doubled.
Scientists were baffled — and concerned. Methane is the big question mark hanging over the world’s climate estimates; although it breaks down in the atmosphere much faster than carbon dioxide, it is so powerful that higher-than-expected methane levels could shift the world toward much higher temperatures.
But now, a study sheds light on what’s driving record methane emissions. The culprits, scientists believe, are microbes — the tiny organisms that live in cows’ stomachs, agricultural fields and wetlands. And that could mean a dangerous feedback loop — in which these emissions cause warming that releases even more greenhouse gases — is already underway.
“The changes that we saw in the last couple of years — and even since 2007 — are microbial,” said Sylvia Michel, lead author of the paper published last month in the Proceedings of the National Academy of Sciences. “Wetlands, if they are getting warmer and wetter, maybe they’re producing more methane than they used to.”
It’s difficult for scientists to identify all the sources of methane in the world. It comes from leaking oil and gas operations, from cows belching, from landfills and marshes, and from thawing permafrost in the Arctic. When methane emissions increase, finding the cause is like solving a complicated algebra problem with too many unknowns.
And it’s a problem that will determine the fate of the climate.
For a time, scientists thought that soaring methane emissions stemmed from the growth in the use of natural gas, which is largely methane. Leaks from drilling or from pipelines can leach the greenhouse gas into the atmosphere.
But the new paper points to microbes as the biggest source of the methane spike. Michel and her co-authors analyzed samples of methane, or CH4, from 22 sites around the globe at a Colorado laboratory. Then they measured the “heaviness” of that methane — specifically, how many of the molecules had a heavier isotope of carbon in them, known as C13.
Different sources of methane give off different carbon signatures. Methane produced by microbes — mostly single-celled organisms known as archaea, which live in cow stomachs, wetlands and agricultural fields — tends to be “lighter,” or have fewer C13 atoms. Methane from fossil fuels, on the other hand, is heavier, with more C13 atoms.
As the amount of methane has risen in the atmosphere over the past 15 years, it’s also gotten lighter and lighter. The scientists used a model to analyze those changes and found that only large increases in microbial emissions could explain both the rising methane and its changing weight.
6 notes
·
View notes
Text
When it comes to making fuel from plants, the first step has always been the hardest -- breaking down the plant matter. A new study finds that introducing a simple, renewable chemical to the pretreatment step can finally make next-generation biofuel production both cost-effective and carbon neutral. For biofuels to compete with petroleum, biorefinery operations must be designed to better utilize lignin. Lignin is one of the main components of plant cell walls. It provides plants with greater structural integrity and resiliency from microbial attacks. However, these natural properties of lignin also make it difficult to extract and utilize from the plant matter, also known as biomass. "Lignin utilization is the gateway to making what you want out of biomass in the most economical and environmentally friendly way possible," said UC Riverside Associate Research Professor Charles Cai. "Designing a process that can better utilize both the lignin and sugars found in biomass is one of the most exciting technical challenges in this field."
Read more.
21 notes
·
View notes
Note
Photosynthesis is a system of biological processes by which photosynthetic organisms, such as most plants, algae, and cyanobacteria, convert light energy, typically from sunlight, into the chemical energy necessary to fuel their metabolism. Photosynthesis usually refers to oxygenic photosynthesis, a process that produces oxygen. Photosynthetic organisms store the chemical energy so produced within intracellular organic compounds (compounds containing carbon) like sugars, glycogen, cellulose and starches. To use this stored chemical energy, an organism's cells metabolize the organic compounds through cellular respiration. Photosynthesis plays a critical role in producing and maintaining the oxygen content of the Earth's atmosphere, and it supplies most of the biological energy necessary for complex life on Earth.
Some bacteria also perform anoxygenic photosynthesis, which uses bacteriochlorophyll to split hydrogen sulfide as a reductant instead of water, producing sulfur instead of oxygen. Archaea such as Halobacterium also perform a type of non-carbon-fixing anoxygenic photosynthesis, where the simpler photopigment retinal and its microbial rhodopsin derivatives are used to absorb green light and power proton pumps to directly synthesize adenosine triphosphate (ATP), the "energy currency" of cells. Such archaeal photosynthesis might have been the earliest form of photosynthesis that evolved on Earth, as far back as the Paleoarchean, preceding that of cyanobacteria (see Purple Earth hypothesis).
While the details may differ between species, the process always begins when light energy is absorbed by the reaction centers, proteins that contain photosynthetic pigments or chromophores. In plants, these proteins are chlorophylls (a porphyrin derivative that absorbs the red and blue spectrums of light, thus reflecting green) held inside chloroplasts, abundant in leaf cells. In bacteria they are embedded in the plasma membrane. In these light-dependent reactions, some energy is used to strip electrons from suitable substances, such as water, producing oxygen gas. The hydrogen freed by the splitting of water is used in the creation of two important molecules that participate in energetic processes: reduced nicotinamide adenine dinucleotide phosphate (NADPH) and ATP.
In plants, algae, and cyanobacteria, sugars are synthesized by a subsequent sequence of light-independent reactions called the Calvin cycle. In this process, atmospheric carbon dioxide is incorporated into already existing organic compounds, such as ribulose bisphosphate (RuBP). Using the ATP and NADPH produced by the light-dependent reactions, the resulting compounds are then reduced and removed to form further carbohydrates, such as glucose. In other bacteria, different mechanisms like the reverse Krebs cycle are used to achieve the same end.
The first photosynthetic organisms probably evolved early in the evolutionary history of life using reducing agents such as hydrogen or hydrogen sulfide, rather than water, as sources of electrons. Cyanobacteria appeared later; the excess oxygen they produced contributed directly to the oxygenation of the Earth, which rendered the evolution of complex life possible. The average rate of energy captured by global photosynthesis is approximately 130 terawatts, which is about eight times the total power consumption of human civilization. Photosynthetic organisms also convert around 100–115 billion tons (91–104 Pg petagrams, or a billion metric tons), of carbon into biomass per year. Photosynthesis was discovered in 1779 by Jan Ingenhousz. He showed that plants need light, not just air, soil, and water.
Photosynthesis is vital for climate processes, as it captures carbon dioxide from the air and binds it into plants, harvested produce and soil. Cereals alone are estimated to bind 3,825 Tg or 3.825 Pg of carbon dioxide every year, i.e. 3.825 billion metric tons.

That reminds me of the Krebs cycle, which creates ATP instead of using it. I am learning just how much lifeforms rely on each other to survive. Destroying one could cause many others to crumble. Interesting.
(OOC: Sorry, but I do not understand plants very well at all. I like anatomy of animals, humans, and bugs more).
3 notes
·
View notes
Note
Photosynthesis is a system of biological processes by which photosynthetic organisms, such as most plants, algae, and cyanobacteria, convert light energy, typically from sunlight, into the chemical energy necessary to fuel their metabolism. Photosynthesis usually refers to oxygenic photosynthesis, a process that produces oxygen. Photosynthetic organisms store the chemical energy so produced within intracellular organic compounds (compounds containing carbon) like sugars, glycogen, cellulose and starches. To use this stored chemical energy, an organism's cells metabolize the organic compounds through cellular respiration. Photosynthesis plays a critical role in producing and maintaining the oxygen content of the Earth's atmosphere, and it supplies most of the biological energy necessary for complex life on Earth.
Some bacteria also perform anoxygenic photosynthesis, which uses bacteriochlorophyll to split hydrogen sulfide as a reductant instead of water, producing sulfur instead of oxygen. Archaea such as Halobacterium also perform a type of non-carbon-fixing anoxygenic photosynthesis, where the simpler photopigment retinal and its microbial rhodopsin derivatives are used to absorb green light and power proton pumps to directly synthesize adenosine triphosphate (ATP), the "energy currency" of cells. Such archaeal photosynthesis might have been the earliest form of photosynthesis that evolved on Earth, as far back as the Paleoarchean, preceding that of cyanobacteria (see Purple Earth hypothesis).
While the details may differ between species, the process always begins when light energy is absorbed by the reaction centers, proteins that contain photosynthetic pigments or chromophores. In plants, these proteins are chlorophylls (a porphyrin derivative that absorbs the red and blue spectrums of light, thus reflecting green) held inside chloroplasts, abundant in leaf cells. In bacteria they are embedded in the plasma membrane. In these light-dependent reactions, some energy is used to strip electrons from suitable substances, such as water, producing oxygen gas. The hydrogen freed by the splitting of water is used in the creation of two important molecules that participate in energetic processes: reduced nicotinamide adenine dinucleotide phosphate (NADPH) and ATP.
In plants, algae, and cyanobacteria, sugars are synthesized by a subsequent sequence of light-independent reactions called the Calvin cycle. In this process, atmospheric carbon dioxide is incorporated into already existing organic compounds, such as ribulose bisphosphate (RuBP). Using the ATP and NADPH produced by the light-dependent reactions, the resulting compounds are then reduced and removed to form further carbohydrates, such as glucose. In other bacteria, different mechanisms like the reverse Krebs cycle are used to achieve the same end.
The first photosynthetic organisms probably evolved early in the evolutionary history of life using reducing agents such as hydrogen or hydrogen sulfide, rather than water, as sources of electrons. Cyanobacteria appeared later; the excess oxygen they produced contributed directly to the oxygenation of the Earth, which rendered the evolution of complex life possible. The average rate of energy captured by global photosynthesis is approximately 130 terawatts, which is about eight times the total power consumption of human civilization. Photosynthetic organisms also convert around 100–115 billion tons (91–104 Pg petagrams, or a billion metric tons), of carbon into biomass per year. Photosynthesis was discovered in 1779 by Jan Ingenhousz. He showed that plants need light, not just air, soil, and water.
Photosynthesis is vital for climate processes, as it captures carbon dioxide from the air and binds it into plants, harvested produce and soil. Cereals alone are estimated to bind 3,825 Tg or 3.825 Pg of carbon dioxide every year, i.e. 3.825 billion metric tons.
Why are we suddenly in a science lesson? Its interesting nontheless though!
4 notes
·
View notes
Text

Electric Bacteria: Harnessing Nature's Microscopic Power Plants for a Sustainable Future
Electric bacteria, or electrogenic microbes, are fascinating organisms capable of generating electricity as part of their natural metabolic processes. Found in diverse environments such as soil, freshwater, and even the human gut, these bacteria can convert organic compounds into electrical energy. This unique ability is primarily observed in species like Shewanella and Geobacter, which use conductive proteins to transfer electrons to external electrodes, functioning like microscopic power plants.
Shewanella oneidensis and Geobacter sulfurreducens are notable examples. These bacteria can form biofilms on electrodes, creating a microbial fuel cell that generates electricity. This phenomenon is not just a scientific curiosity but has practical applications. For instance, microbial fuel cells can be used in wastewater treatment plants to both clean water and generate electricity simultaneously. Additionally, electrogenic bacteria are being explored for bioremediation, helping to clean up polluted environments by breaking down contaminants and converting them into less harmful substances.
The potential of electric bacteria extends to sustainable energy solutions. By harnessing their natural abilities, researchers aim to develop innovative technologies that offer renewable energy sources. The intersection of microbiology and energy technology could lead to breakthroughs that address some of the world's pressing environmental challenges.
References:
Lovley, D. R. (2012). Electromicrobiology. Annual Review of Microbiology, 66, 391-409.
Nealson, K. H., & Rowe, A. R. (2016). Electromicrobiology: Realities, grand challenges, goals and predictions. Microbial Biotechnology, 9(5), 595-600.
Logan, B. E. (2009). Exoelectrogenic bacteria that power microbial fuel cells. Nature Reviews Microbiology, 7(5), 375-381.
5 notes
·
View notes
Text
youtube
In 2052 Six Months Before the Apocalypse by In 20xx Futurism People are scared the world will end when the Moon Canons Project is shut down. A couple move to an Xtian Nationalist state to care for an elderly mother and soon face fanatics and local terrorist. A woman comes to town for revenge on people who killed her family. Moon canon project - Canons on the moon that shoot dust to create cloud trails, intended to reduce sunlight and combat climate change. Shut down unexpectedly. Autonomous planes - Used to ferry residents out of Springfield airport after schools close. Luggage bots - Suitcases that can walk and follow their owners on legs. Survive-all pod - A refrigerator-sized pod filled with equipment, walks on legs, provides power, water, etc. An essential survival tool. AR glasses - Provide 360 degree vision, night vision, infrared, and other enhanced senses. Allow accessing the internet through AR. Mechanical stomach - Produces nutritional bars from plant matter. An off-grid food source. Atmospheric water harvester - Condenses moisture from the air into drinkable water. Useful in arid environments. Century battery - Provides constant power for over 100 years through microbial fuel cell technology. Powers the survive-all pod. Medusa - An AI that can infect devices and create shadow servers to enable internet access off the grid. DNA eraser - Chemical that destroys DNA, used to cover tracks. The Tuckers use it when the main character escapes in a jeep. Cop bots - Robots used as autonomous police in Springfield. Brought back online to search for the fugitive. Many of the characters in this project appear in future episodes. Using storytelling to place you in a time period, this series takes you, year by year, into the future. From 2040 to 2195. If you like emerging tech, eco-tech, futurism, perma-culture, apocalyptic survival scenarios, and disruptive science, sit back and enjoy short stories that showcase my research into how the future may play out. This is Episode 47 of the podcast "In 20xx Scifi and Futurism." The companion site is https://in20xx.com where you can find a timeline of the future, descriptions of future development, and printed fiction. These are works of fiction. Characters and groups are made-up and influenced by current events but not reporting facts about people or groups in the real world. Copyright © Leon Horn 2021. All rights reserved. Check out the latest episode of In 20xx Scifi and Futurism! Listen to the full podcast here https://ift.tt/Jl1ODGb (video made with https://ift.tt/f3e1h62) via YouTube https://youtu.be/nI8INTJMmTo
#emerging tech#eco-tech#apocalypse#survival#disruptive#science#climate#future#short#solarpunk#post-apocalyptic#predictions#futurology#futurism#scifi#sci-fi#technology#tech#black mirror#Youtube
2 notes
·
View notes
Text
Microbial Fuel Cell Market Size, Share & Industry Analysis, By Type (Mediated, Unmediated), By End-User (Residential & Commercial, Industrial, Transportation, Military, Utilities, Others) and Regional Forecast, 2022-2029
0 notes
Link
#market research future#microbial fuel cells company#microbial fuel cell industry#microbial fuel cell analysis#microbial fuel cell report
0 notes
Text
Biotech Ingredients Market Analysis: Key Challenges and Opportunities
Growing Adoption of Sustainable and Innovative Solutions Drives Growth in the Biotech Ingredients Market.

The Biotech Ingredients Market size was valued at USD 2.2 billion in 2023 and is expected to reach USD 4.3 billion by 2032 and grow at a CAGR of 7.8% over the forecast period 2024-2032.
The Biotech Ingredients Market is experiencing rapid growth as industries increasingly shift toward sustainable, high-performance, and bio-based alternatives. Biotech ingredients, derived from microbial fermentation, plant cell culture, and enzyme technology, are revolutionizing sectors such as cosmetics, pharmaceuticals, food & beverages, and personal care. With a strong emphasis on eco-friendliness, reduced carbon footprint, and enhanced efficacy, biotech ingredients are gaining widespread adoption across the globe.
Key Players in the Biotech Ingredients Market
BASF SE (Lecithin, Enzymes)
Cargill, Incorporated (Soy Protein, Lactic Acid)
DuPont de Nemours, Inc. (Proteins, Enzymes)
Evonik Industries AG (Amino Acids, Biopolymers)
Genomatica, Inc. (Bio-BDO, Bio-1,4-Butanediol)
DSM (Dutch State Mines) (Amino Acids, Enzymes)
Novozymes A/S (Cellulases, Amylases)
Roche Holding AG (Biopharmaceuticals, Diagnostic Reagents)
SABIC (Saudi Basic Industries Corporation) (Biodegradable Polymers, Bio-based Chemicals)
Syngenta AG (Biofungicides, Bioinsecticides)
These companies are investing heavily in biotechnology, fermentation processes, and sustainable ingredient development to meet the rising consumer demand for natural and clean-label products.
Future Scope and Emerging Trends
The Biotech Ingredients Market is poised for substantial growth, driven by the expanding applications in cosmetics, pharmaceuticals, and food technology. The rising preference for plant-based, cruelty-free, and sustainable alternatives is accelerating demand for biotech-derived fragrances, active skincare compounds, and pharmaceutical excipients.
Advancements in synthetic biology, enzyme engineering, and microbial fermentation are enabling the production of high-purity, effective, and eco-friendly ingredients that replace conventional chemical-based counterparts. Additionally, biotech innovations are helping reduce dependency on petrochemicals and promote circular economy initiatives by utilizing waste-to-value strategies.
Key Market Points:
✅ Growing Demand for Bio-Based & Sustainable Ingredients: Increased focus on eco-friendly, non-toxic, and renewable resources. ✅ Expanding Applications: Biotech ingredients are widely used in cosmetics, fragrances, food flavors, nutraceuticals, and pharmaceuticals. ✅ Advancements in Fermentation & Synthetic Biology: Cutting-edge technology enables the production of high-quality biotech actives. ✅ Rise in Clean Beauty & Green Chemistry: Consumers prefer non-GMO, natural, and biodegradable ingredients. ✅ Regulatory Support & Green Initiatives: Government policies promoting bio-based innovations and sustainability are boosting market growth. ✅ Personalized & Functional Ingredients: The emergence of customized biotech ingredients for targeted skincare and health benefits.
Conclusion
The Biotech Ingredients Market is set for strong expansion, fueled by sustainable innovations, regulatory support, and rising consumer demand for ethical and effective products. Companies focusing on biotech-driven ingredient solutions, fermentation-based production, and clean-label formulations are well-positioned to lead the next phase of industry transformation.
Read Full Report: https://www.snsinsider.com/reports/biotech-ingredients-market-4593
Contact Us:
Jagney Dave — Vice President of Client Engagement
Phone: +1–315 636 4242 (US) | +44- 20 3290 5010 (UK)
#Biotech Ingredients Market#Biotech Ingredients Market Size#Biotech Ingredients Market Share#Biotech Ingredients Market Report#Biotech Ingredients Market Forecast
0 notes
Text
Microbial Fuel Cells: Turning Waste into Energy—The Future is Alive 🔬⚡
Microbial Fuel Cells for Waste Treatment Market is revolutionizing sustainability by converting organic waste into electricity. These bio-electrochemical systems offer an eco-friendly alternative to traditional waste treatment, bridging the gap between waste management and renewable energy generation.
To Request Sample Report: https://www.globalinsightservices.com/request-sample/?id=GIS32726 &utm_source=SnehaPatil&utm_medium=Article
The wastewater treatment segment dominates the market, playing a crucial role in industrial and municipal applications. Bioremediation follows as a rising trend, attracting investment in eco-friendly remediation technologies. North America leads, thanks to advanced infrastructure and strong regulatory support, while Europe, driven by stringent environmental policies, emerges as the second-largest market. The United States and Germany spearhead growth with cutting-edge innovations and sustainability commitments.
By 2028, the market is set to expand rapidly, fueled by technological advancements in microbial systems and a rising global focus on renewable energy. Countries like China and India are also accelerating adoption due to rapid industrialization and increasing waste challenges. Industry pioneers are shaping the future with groundbreaking solutions that enhance waste-to-energy efficiency. As the demand for sustainable waste treatment rises, microbial fuel cells are poised to be a game-changer in the green energy revolution.
#microbialfuelcells #renewableenergy #wastetoelectricity #sustainablewastemanagement #bioelectrochemistry #wastewatertreatment #cleantech #circularenergy #climateaction #greeninnovation #futureofenergy #sustainabledevelopment #carbonneutral #bioremediation #environment #smartwaste #energytransition #greentech #netzero #wastevalorization #nextgentech #cleanpower #sustainablecities #urbaninnovation #industrialwaste #municipalsolutions #renewabletech #wasteconversion #bioenergy #climatechange #smartwastemanagement #ecotechnology #cleanenergysolutions #futuretech #biotech #greenpower #blueeconomy #wasteutilization #carbonreduction #sustainability
0 notes