#Hydrological Study Required
Explore tagged Tumblr posts
Text
Dhalbhumgarh Airport Project Faces Hurdles as Forest Clearance Returned for Revisions
The Ministry of Environment, Forest and Climate Change requests more details on Dhalbhumgarh Airport’s forest clearance proposal, clarifying the site isn’t within the Elephant Corridor. The Ministry of Environment, Forest and Climate Change (MoEF&CC) has returned the Dhalbhumgarh Airport project’s forest clearance proposal to the Jharkhand government, seeking additional information and…
#compensatory afforestation#Dhalbhumgarh Airport Project#Elephant Corridor Impact#Environmental Impact Assessment#Forest Clearance Delay#human-elephant conflict#Hydrological Study Required#Jharkhand development#MoEF&CC Environmental Review#Singhbhum Elephant Reserve
0 notes
Text
"In response to last year’s record-breaking heat due to El Niño and impacts from climate change, Indigenous Zenù farmers in Colombia are trying to revive the cultivation of traditional climate-resilient seeds and agroecology systems.
One traditional farming system combines farming with fishing: locals fish during the rainy season when water levels are high, and farm during the dry season on the fertile soils left by the receding water.
Locals and ecologists say conflicts over land with surrounding plantation owners, cattle ranchers and mines are also worsening the impacts of the climate crisis.
To protect their land, the Zenù reserve, which is today surrounded by monoculture plantations, was in 2005 declared the first Colombian territory free from GMOs.
...
In the Zenù reserve, issues with the weather, climate or soil are spread by word of mouth between farmers, or on La Positiva 103.0, a community agroecology radio station. And what’s been on every farmer’s mind is last year’s record-breaking heat and droughts. Both of these were charged by the twin impacts of climate change and a newly developing El Niño, a naturally occurring warmer period that last occurred here in 2016, say climate scientists.
Experts from Colombia’s Institute of Hydrology, Meteorology and Environmental Studies say the impacts of El Niño will be felt in Colombia until April 2024, adding to farmers’ concerns. Other scientists forecast June to August may be even hotter than 2023, and the next five years could be the hottest on record. On Jan. 24, President Gustavo Petro said he will declare wildfires a natural disaster, following an increase in forest fires that scientists attribute to the effects of El Niño.
In the face of these changes, Zenù farmers are trying to revive traditional agricultural practices like ancestral seed conservation and a unique agroecology system.
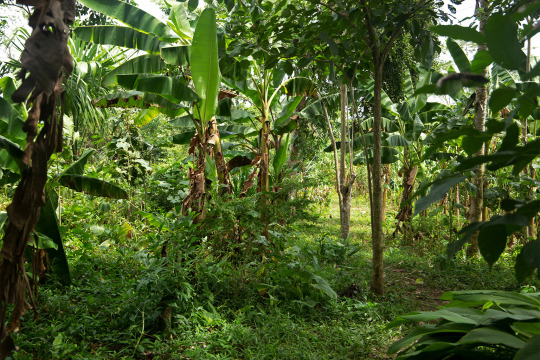
Pictured: Remberto Gil’s house is surrounded by an agroforestry system where turkeys and other animals graze under fruit trees such as maracuyá (Passiflora edulis), papaya (Carica papaya) and banana (Musa acuminata colla). Medicinal herbs like toronjil (Melissa officinalis) and tres bolas (Leonotis nepetifolia), and bushes like ají (Capsicum baccatum), yam and frijol diablito (beans) are part of the undergrowth. Image by Monica Pelliccia for Mongabay.
“Climate change is scary due to the possibility of food scarcity,” says Rodrigo Hernandez, a local authority with the Santa Isabel community. “Our ancestral seeds offer a solution as more resistant to climate change.”
Based on their experience, farmers say their ancestral seed varieties are more resistant to high temperatures compared to the imported varieties and cultivars they currently use. These ancestral varieties have adapted to the region’s ecosystem and require less water, they tell Mongabay. According to a report by local organization Grupo Semillas and development foundation SWISSAID, indigenous corn varieties like blaquito are more resistant to the heat, cariaco tolerates drought easily, and negrito is very resistant to high temperatures.
The Zenù diet still incorporates the traditional diversity of seeds, plant varieties and animals they consume, though they too are threatened by climate change: from fish recipes made from bocachico (Prochilodus magdalenae), and reptiles like the babilla or spectacled caiman (Caiman crocodilus), to different corn varieties to prepare arepas (cornmeal cakes), liquor, cheeses and soups.
“The most important challenge we have now is to save ancient species and involve new generations in ancestral practice,” says Sonia Rocha Marquez, a professor of social sciences at Sinù University in the city of Montería.
...[Despite] land scarcity, Negrete says communities are developing important projects to protect their traditional food systems. Farmers and seed custodians, like Gil, are working with the Association of Organic Agriculture and Livestock Producers (ASPROAL) and their Communitarian Seed House (Casa Comunitaria de Semillas Criollas y Nativas)...
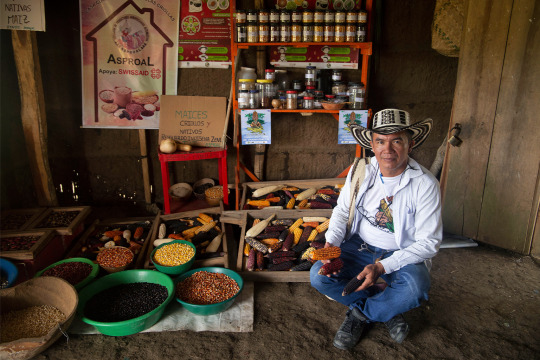
Pictured: Remberto Gil is a seed guardian and farmer who works at the Communitarian Seed House, where the ASPROL association stores 32 seeds of rare or almost extinct species. Image by Monica Pelliccia for Mongabay.
Located near Gil’s house, the seed bank hosts a rainbow of 12 corn varieties, from glistening black to blue to light pink to purple and even white. There are also jars of seeds for local varieties of beans, eggplants, pumpkins and aromatic herbs, some stored in refrigerators. All are ancient varieties shared between local families.
Outside the seed bank is a terrace where chickens and turkeys graze under an agroforestry system for farmers to emulate: local varieties of passion fruit, papaya and banana trees grow above bushes of ají peppers and beans. Traditional medicinal herbs like toronjil or lemon balm (Melissa officinalis) form part of the undergrowth.
Today, 25 families are involved in sharing, storing and commercializing the seeds of 32 rare or almost-extinct varieties.
“When I was a kid, my father brought me to the farm to participate in recovering the land,” says Nilvadys Arrieta, 56, a farmer member of ASPROAL. “Now, I still act with the same collective thinking that moves what we are doing.”
“Working together helps us to save, share more seeds, and sell at fair price [while] avoiding intermediaries and increasing families’ incomes,” Gil says. “Last year, we sold 8 million seeds to organic restaurants in Bogotà and Medellín.”
So far, the 80% of the farmers families living in the Zenù reserve participate in both the agroecology and seed revival projects, he adds."
-via Mongabay, February 6, 2024
#indigenous#ecology#agroforestry#agriculture#traditional food systems#traditional medicine#sustainable agriculture#zenu#indigenous peoples#farming#colombia#indigenous land#traditional knowledge#seeds#corn#sustainability#botany#plant biology#good news#hope#climate action#climate change#climate resilience#agroecology#food sovereignty
1K notes
·
View notes
Text
Yesterday, I posted a summary of the study and report described in this Grist article. We get more details (if we're interested) here. Additionally, to me, the recommendations to proceed more holistically with the numerous global issues dealing with biodiversity and the climate are critically important. I've been repeating this mantra for several years, and will continue to do so. Deal with the environment = solve the climate crisis.
Excerpt from this story from Grist:
As global temperatures rise from the burning of fossil fuels, researchers and policymakers have proposed solutions like installing renewable energy, replacing gasoline-powered cars with electric ones, and developing technology to suck carbon out of the air. But these policies often address climate change in isolation — without regard for other pressing issues like a decline in biodiversity, the contamination of freshwater sources, and the pollution of agricultural soils.
A new report released Tuesday by the United Nations’ expert panel on biodiversity makes the case for a different approach based on addressing the “nexus” between two or more out of five essential issue areas: climate change, biodiversity, food, human health, and water. Such an approach is not only more likely to help the world meet various U.N. targets on biodiversity, sustainable development, and climate mitigation; it’s also more cost-effective.
“We have to move decisions and actions beyond single-issue silos,” said Paula Harrison, a professor of land and water modeling at the U.K. Centre for Ecology and Hydrology and a co-chair of the report, in a statement. Other scientific reports have studied the interlinkages between two or three of these issues, but she told reporters on Tuesday that this latest report is the “most ambitious” to date.
The new report was the result of three years of work of the Intergovernmental Science-Policy Platform on Biodiversity and Ecosystem Services, or IPBES, an expert body that’s analogous to the United Nations’ Intergovernmental Panel on Climate Change, which periodically assesses the state of the science on global warming.
The report centers on biodiversity — that’s the IPBES’s remit, after all — describing how the variety of life on Earth is “essential to our very existence.” But it goes out of its way to show how rapidly accelerating biodiversity loss is both contributing to and being exacerbated by other crises. Climate change, for instance, is making some habitats inhospitable to their erstwhile animal populations, while the loss of those populations can have impacts on freshwater availability and carbon storage. The five interlinking issues were selected by representatives of the 147 IPBES’s member countries.
Meanwhile, solutions that focus on just one issue may have detrimental effects on other elements. Pete Smith, a professor of soils and global change at the University of Aberdeen in the United Kingdom, gave the example of bioenergy with carbon capture and storage, or BECCS, a climate solution in which crops are grown to draw CO2 out of the air and then burned to generate energy. The resulting greenhouse gas emissions are captured and stored in rock formations, with the aim of removing them from the carbon cycle permanently.
The problem, Smith said, is that to implement this process on a large scale would require vast tracts of land that might otherwise have been used to grow food crops — so BECCS can unintentionally harm food security. Devoting land to single-variety crops can also use up lots of water and jeopardize biodiversity.
10 notes
·
View notes
Text
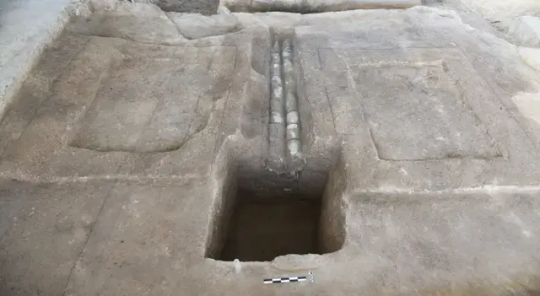
4,000-Year-Old Ceramic Drainage System Discovered in China
The people of Pingliangtai built and operated the system without any help from a central state government.
China’s Longshan period which lasted from about 2600 to 2000 BCE is best known for its sophisticated pottery shapes, but their sophisticated plumbing is getting some well-deserved attention. A team of archaeologists found the oldest known ceramic water pipes in China, demonstrating that locals were capable of major feats of engineering without a centralized state government. The findings are described in a study published August 14 in the journal Nature Water.
The newly unearthed network of ceramic water pipes and drainage ditches were found at the ancient walled city of Pingliangtai, located in what is now the Huaiyang District of Zhoukou City in central China. The town was home to roughly 500 people during neolithic times and had protective walls and a surrounding moat. It sits on the Upper Huai River Plain on the vast Huanghuaihai Plain, and the climate 4,000 years ago saw large seasonal climate shifts. Summer monsoons could dump a foot and a half of rain on the region every month.

With all this rain, it was critical for the region to manage floodwaters. The people of Pingliangtai appear to have built and operated a two-tier drainage system to help mitigate the rainy season’s excessive rainfall. Simple but coordinated lines of drainage ditches ran parallel to the rows of houses to divert water from the residential area to a series of ceramic water pipes that carried the water into the surrounding moat, and away from the village.
The team says that this network of pipes shows that the community cooperated with one another to build and maintain this drainage system.
“The discovery of this ceramic water pipe network is remarkable because the people of Pingliangtai were able to build and maintain this advanced water management system with stone age tools and without the organization of a central power structure,” study co-author and University College London archaeologist Yijie Zhuang said in a statement. “This system would have required a significant level of community-wide planning and coordination, and it was all done communally.”
The network is made of interconnecting individual segments which run along roads and walls that divert rainwater. According to the team, it shows an advanced level of central planning and is the oldest complete system discovered in China to date.
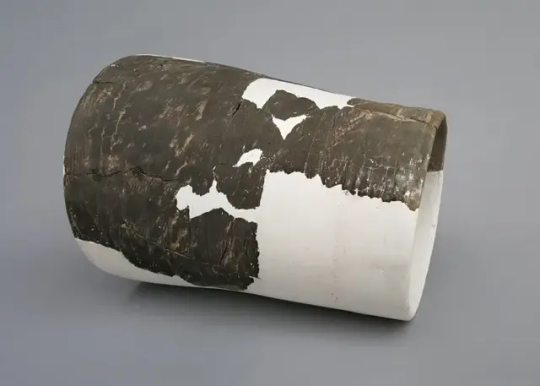
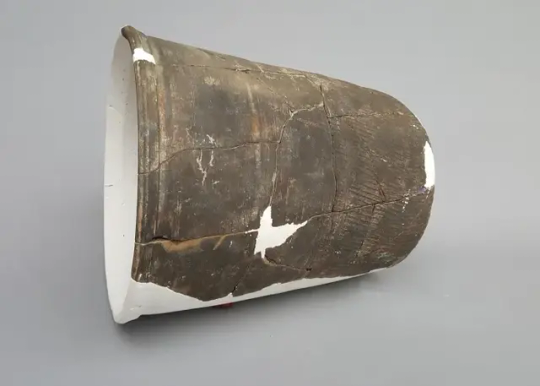
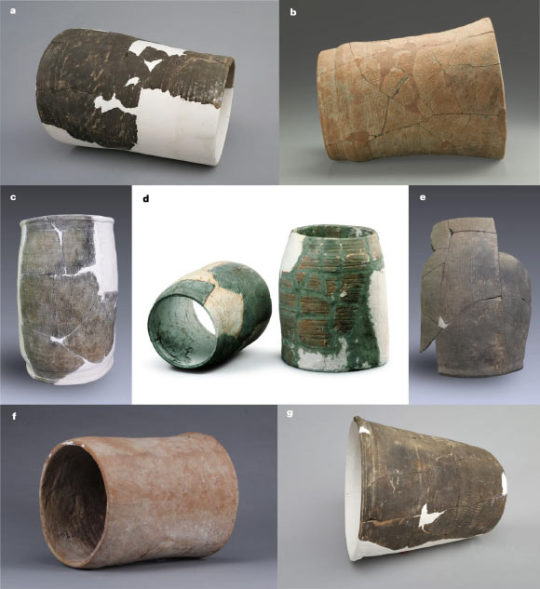
The team was also surprised by this find because the Pingliangtai settlement shows little evidence of a social hierarchy. The homes within it were uniformly small and there aren’t any signs of social stratification or significant inequality amongst the population. Digs at the town’s cemetery also didn’t reveal any evidence of a social hierarchy in burials the way excavations at other nearby towns have.
The level of complexity that these pipes demonstrate also undermines some earlier understanding of archaeological finds that believe only a centralized state power could organize and provide the resources for such a complex water management system. Other ancient societies that used advanced water systems tended to have a stronger, more centralized government, but Pingliangtai shows that that centralized power was possibly not always needed.
“Pingliangtai is an extraordinary site. The network of water pipes shows an advanced understanding of engineering and hydrology that was previously only thought possible in more hierarchical societies,” study co-author and Peking University archaeologist Hai Zhang said in a statement.
The ceramic water pipes also show an advanced level of technology for this period in time. Like with Longshan pottery, there was some variety of decoration and styles, but each pipe segment was about 7.8 and 11.8 inches in diameter and about 11.8 to 15.7 inches long. Multiple segments were slotted into one another to transport the water over long distances.
According to the study, the team can’t say specifically how the labor to build this infrastructure was organized and divided. A similar level of communal coordination would also have been necessary to build the earthen walls and moat that surround Pingliangtai.
By Laura Baisas.
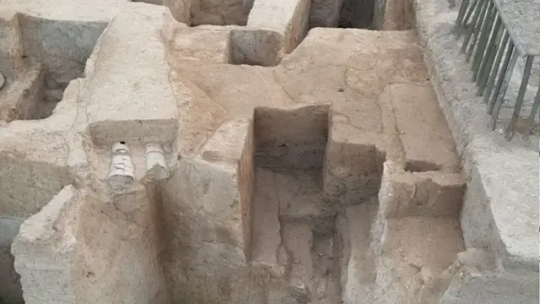
#4000-Year-Old Ceramic Drainage System Discovered in China#ancient city of Pingliangtai#Zhoukou City#ancient artifacts#archeology#archeolgst#history#history news#ancient history#ancient culture#ancient civilizations#ancient china#chinese history
36 notes
·
View notes
Text
Unit 5 Blog Post: Citizen Science and Conservation Practices
Happy thanksgiving everyone!
Given that this week’s blog prompt is open, I wanted to share some thoughts inspired by our course content so far.
While watching Washington Wachira’s TED Talk "For the Love of Birds," I began reflecting on the role of citizen science. Apps like iNaturalist, which is widely used in Guelph, offer a powerful tool to connect people with nature by allowing users to log observations. However, they also inadvertently filter participation.
For instance, I’ve spoken with older individuals who possess immense knowledge of local flora and fauna but do not engage with these apps. Their insights are invaluable, yet their observations remain undocumented in digital platforms. This raises a concern: Are we excluding certain demographics from contributing to citizen science simply because of a technological barrier?
This issue highlights the need to design more inclusive citizen science initiatives. If older generations or non-tech-savvy individuals struggle to access these platforms, we may miss crucial knowledge. Digital platforms should be complemented with physical or analog extensions—perhaps logbooks or community-led observation notebooks that can be collected and digitized by volunteers.
During my time in Kenya, I witnessed how citizens live in harmony with their natural environment. Kenya’s incredible biodiversity, which spans savannahs, tropical forests, deserts, and highlands, surpasses that of Canada. Yet, despite this richness, much of the local knowledge remains undocumented in apps or digital tools. Conservation in Kenya requires more than just technological solutions—it depends on community engagement and biocultural conservation. One of my professors, Carol Muriuki, a conservationist with the National Environment Management Authority (NEMA), shared insights that transformed my understanding of conservation. She emphasized that conservation cannot follow a “one-size-fits-all” approach. Community stewardship and biocultural conservation is crucial for designing conservation initiatives that have a lasting positive impact. Instead of crafting a conservation plan that looks good on paper but is not feasible in the real world. Each initiative must account for ecological, economic, and cultural realities.
A compelling example is the Lake Naivasha region, where rising water levels—likely caused by climate change—are displacing communities that rely on the lake for food and income. As Carol explained, simply forcing people to relocate isn’t a viable solution. Instead, NEMA is working on a more holistic approach, such as restructuring hydrological infrastructure, planting mangrove trees, and compensating displaced families. This approach integrates the needs of both people and the environment, exemplifying how inclusive conservation practices can lead to sustainable outcomes.

Figure 1. Blurry view of Lake Naivasha from the campground in Kenya (Griffiths, 2024)
I see Carol’s work as a model for future conservation efforts, where citizen science plays a central role in shaping projects rather than just being a tool for data collection. For citizen science to be effective, it must evolve beyond passive contributions. It should foster continuous dialogue between scientists and the public, ensuring citizens actively participate in research and conservation initiatives. This approach could help address the issue of bias in scientific sampling. Scientists often focus on charismatic species—those that are easy to observe or already have a wealth of knowledge available from past studies. As opposed to cryptic or under-studied species. In contrast, citizen observations tend to be more exploratory, as participants are not constrained by preconceptions about which species are significant, enriching scientific understanding in unexpected ways.
One of the biggest takeaways from this course is the realization that academic science offers only a narrow lens through which to engage with nature. As students, it’s easy to become trapped within the confines of scientific rigor and overlook the many other ways people connect with the natural world. Yet, through this course, I’ve learned that storytelling, art, and lived experiences are equally powerful tools for interpreting the environment. For example, conservation is as much about understanding community needs as it is about protecting ecosystems. Similarly, citizen science is not just about data—it’s about fostering a deeper relationship between people and nature.
Ultimately, effective conservation requires both emotional and intellectual engagement. Successful initiatives depend on integrating scientific knowledge with community stewardship. Similarly, citizen science can only reach its full potential when it invites participation from all walks of life—from scientists, to tech-savvy citizens, and those more comfortable with traditional forms of engagement. As I reflect on what we’ve covered so far, I believe we are just beginning to scratch the surface of how we can engage an audience with nature. The challenge lies in finding new ways to connect with both people and the environment—whether through technology, community dialogue, or personal storytelling.
2 notes
·
View notes
Text
Sustainable Natural Resource Management: Forests, Woodlands, and Wetlands
Abstract
Forest and wetlands are fragile communities, when human activities precede uncontrolled their roles are lost. Objective was to investigate the role of forest and wetlands as water catchment areas in Zimbabwe. Christmas Pass forest woodland and wetland in Mutare was the study site. Sample of 196 people, selected through stratified random sampling and simple random sampling, then purposive sampling for 20 key informants. In-depth interview, key informant interviews, focus group discussion, and observation was conducted. Study revealed that both forest and wetlands are important in the hydrological cycle. Noted that there are several adverse impacts brought by anthropogenic activities. Observed that water was an essential factor in sustainable forest management, and forests are crucial for regulating the water cycle. Forest woodlands and wetlands are under a huge threat for extinction, as anthropogenic activities continue to impact negatively on these areas. Forest woodlands and wetlands are a major water catchment area and there is need for catchment basin management plan for as to rejuvenate the river flow downstream. Recommended the need for best management practices (BMPs) as they are proactive and often voluntary practical methods or practices used during forest management to achieve goals related to water quality, silviculture, wildlife and biodiversity, aesthetics, and/or recreation. Noted that the sustainable management of the forest woodlands requires participatory approach of all stakeholders through capacity building and empowerment. Above all, there was need for the catchment basin to balance its role of provision of human needs and the ecosystem services.

Introduction
The deforestation involves conversion of forest land to agriculture land, or residential resettlement. Worldwide the most concentrated deforestation occurs in tropical rainforests. About 31% of Earth's land surface is covered by forests. Between 15 million to 18 million hectares of forest, an area the size of Belgium, are destroyed every year, on average 2,400 trees are cut down each minute (IUFRO, 2007). FAO (2013) indicated that only 4 billion hectares of forest are left. The world has lost one-third of its forest, an area twice the size of the United States. This is despite the fact that forest and wetland are major catchment area for water, which need to be used by the human beings.
The above degradation of the wetland and forest has significantly affected the hydrological cycle. FAO (2013) suggested that water is a scarce commodity as it availability, accessibility, adequate and safety heavily depends on climate conditions, weather and sustainable management of the water catchment basins. The first and key step in providing safe water is the selection of the best available sources of water. The best sources of safe water is found in well protected catchment area that includes forest woodlands and wetlands. In general ground water is better protected water that the surface water, the ground water is usually found in the forest or wetlands as springs (Bonan, 2008).Catchment protection is the second step in providing safe water and where, for whatever reason, source choice is limited it presents a key opportunity to minimise pathogen contamination. A catchment is an area where water is collected by the natural landscape. Imagine cupping a person’s hands in a downpour of rain and collecting water in them (FAO, 2013). The forest woodlands and wetlands are a very important water catchment basin. In most parts of Zimbabwe, it is being evident that the management of water catchment basin depends largely on the institutional setting as well as policy orientation of different communities.
Naturally, human beings, animals, birds and forests depend largely on each other and without proper management systems human beings will overrule the natural communities. This naturally creates tension between natural resources, including woodlands, wetlands, animals and birds since the demand and the need for these natural resources will increase (FAO, 2013). This has led to degradation of the forest woodlands and the wetlands. Wetlands and forest woodlands are fragile communities and when human activities precede uncontrolled, function and roles of the wetland and forest woodland as a water catchment source and species richness will be lost. According to Bredemeier (2002), anthropogenic activities affect the health of our water catchments this is through deforestation of the forest woodlands, and settlement and farming in the wetlands just to mention a few.
Humans often equate forest and wetlands with wasteland, a place to be drained, filled in, burnt off and re-purposed. In fact, FAO (2013) studies show that 64% of the world’s wetlands have disappeared since 1900. Measured against 1700, an estimated 87% have been lost. There has been serious deforestation, clearance, clearcutting, or clearing is the removal of a forest or stand of trees the Christmas Pass forest woodland and wetland that is then converted to non-forest use.
Water has become a scarce commodity in the study area as the water catchment areas are drying up. FAO (2013) indicated that the forest woodlands and wetlands are being cleared for the purpose of timber harvesting, resettlement and farming. Therefore the study area is not spared, this has led to woodlands and wetlands around the study area losing their original status of being a water catchment basin, loss of flora and fauna species used to be seen in the forest and wetland area as there is no water to drinking. The rivers network are dried up and no water is flowing downstream. This then means that Zimbabwe has not been spared, from the adverse impacts of land degradation desertification, and drought. FAO (2013) indicated that it is estimated that 10% of land’ soils are under high risk of erosion due to the nature of soils, which are sodic. The soils break into fine particles and tunnel subsequently collapsing and forming gullies (FAO, 2013). Some of the reason for land degradation especially taking the form of desertification, deforestation, overgrazing, salinization, or soil erosion, land degradation can be caused by unsustainable land management practices, such as deforestation, soil nutrient mining and biophysical factors, such as the natural topography of an area or its rainfall, wind, and temperature.
Source : Sustainable Natural Resource Management: Forests, Woodlands, and Wetlands | InformatoveBD
2 notes
·
View notes
Text
Brazil’s Cerrado, the second largest biome after the Amazon, could lose almost 34% of its water by 2050
The Cerrado, a vast tropical savanna in Brazil and the country’s second largest biome after the Amazon rainforest, could lose 33.9% of its river flows by 2050, if the pace of agricultural exploitation remains at current levels. This volume of water corresponds to the flow of eight Nile rivers in Egypt.
The Cerrado occupies 22% of the Brazilian territory, in the center-east region, including the capital Brasília. The alert was made by Instituto Cerrados (Cerrados Institute), an NGO that works to protect the environment. They analyzed 81 river basins in the region, between 1985 and 2022, and discovered that there was a decrease in flow by 88% due to the growth of agriculture.
The study indicates that the cultivation of soybeans, corn and cotton, as well as livestock, have influenced the water cycle of the Cerrado. Also, according to the study, changes in land use resulting from deforestation caused a reduction in water flow in 56% of cases. The other 44% is associated with climate change.
“Deforestation for large-scale agriculture, which requires intense irrigation, changes the hydrological cycle in a way that reduces the flow of rivers,” said the founder and director of Cerrados Institute, Yuri Botelho Salmona.
Continue reading.
#brazil#politics#brazilian politics#environmentalism#cerrado#mod nise da silveira#image description in alt
14 notes
·
View notes
Text
Understanding Idaho Mineral Remoteness Evaluations

Idaho’s expansive landscape contains numerous natural resources, but not every location is suitable for mining or mineral exploration. This is why Idaho mineral remoteness evaluations are so critical. These studies assess the possibility of valuable minerals being present in certain areas, guiding informed choices about land use and development.
What Are Mineral Remoteness Evaluations?
Mineral remoteness evaluations identify whether an area contains significant mineral resources or is unlikely to hold such deposits. These evaluations play a pivotal role in balancing resource extraction with environmental preservation. Completing these studies helps ensure compliance with state and federal laws while protecting Idaho’s distinctive ecosystems.
Why Are These Evaluations Important?
In Idaho, these studies provide several benefits, such as:
Promoting Sustainable Development: Evaluations pinpoint areas unsuitable for mining to encourage responsible land planning.
Reducing Environmental Damage: By avoiding remote zones for exploration, evaluations safeguard delicate ecosystems.
Maintaining Compliance: Abiding by environmental standards is essential for any undertaking.
To learn more, visit our mineral remoteness evaluations page.
Why Environmental Assessments Matter in Idaho
Environmental assessments in Idaho form a vital part of mineral remoteness studies. These evaluations provide a comprehensive look at a project’s environmental impact. From soil analysis to water resource management, an Idaho environmental firm addresses every detail thoroughly.
How Environmental Assessments Add Value to Mineral Studies
Environmental assessments support mineral remoteness evaluations by ensuring:
Detailed Data Collection: Experts analyze soil, rock formations, and water systems to evaluate geological potential.
Regulatory Compliance: Comprehensive reports aid in meeting legal requirements.
Sustainable Decision-Making: Holistic evaluations encourage environmentally conscious planning.
Selecting an Idaho Environmental Firm
Choosing a reputable environmental firm in Idaho is key to obtaining accurate evaluations. An experienced team understands the complexities of local regulations and geological studies, providing actionable insights.
Qualities of a Dependable Idaho Environmental Firm
Extensive Expertise: Look for firms with a proven track record in Idaho environmental services.
In-Depth Knowledge: A team with expertise in geology, hydrology, and environmental science is invaluable.
Regional Understanding: Familiarity with Idaho’s terrain ensures precise evaluations.
Our firm tailors its services to meet your specific needs. Whether you require mineral remoteness evaluations or environmental assessments, we’re here to help.
Steps in Mineral Remoteness Evaluations
Mineral remoteness evaluations involve several stages to deliver dependable results. Here’s an outline of the process:
1. Initial Site Review
Specialists begin by examining the site thoroughly. This includes reviewing geological maps, historical records, and available documentation. This foundational step ensures accurate assessments.
2. On-Site Investigations
On-site investigations gather data about geological structures and potential mineral presence. These field studies are vital for developing comprehensive evaluation reports.
3. Data Analysis and Reporting
Once field data is collected, experts use advanced methods to analyze the findings. The final report outlines the site’s mineral potential and environmental considerations.
Benefits of These Evaluations
Encourages Responsible Development
Identifying areas unfit for mining prevents unnecessary disruptions to Idaho’s natural regions.
Simplifies Regulatory Adherence
Complying with state and federal regulations is crucial for any project. Mineral remoteness evaluations make this process easier.
Protects Vulnerable Ecosystems
Idaho’s ecosystems are diverse and sensitive. Conducting proper evaluations helps preserve these environments for future generations.
Why Partner With Our Idaho Environmental Services?
As a reliable Idaho environmental firm, we provide customized evaluations designed to suit your needs. Our team focuses on:
Accuracy: Delivering precise and actionable information.
Efficiency: Completing projects promptly and within budget.
Sustainability: Assisting clients in making eco-friendly choices.
Conclusion
Mineral remoteness evaluations are vital for fostering sustainable development and protecting Idaho’s environment. Working with a skilled environmental firm ensures comprehensive assessments, regulatory adherence, and responsible land use.
Ready to start? Visit our mineral remoteness evaluations page to learn more about how we can support your projects.
0 notes
Text
A Comprehensive Analysis of the SSC JE Exam Syllabus
Introduction to SSC JE Examination
A route to esteemed engineering positions in government agencies is the Staff Selection Commission Junior Engineer (SSC JE) test. This test evaluates a candidate's technical proficiency and general aptitude and is intended for civil, electrical, and mechanical engineers. The first step to success is comprehending the syllabus. In order to help candidates succeed, this handbook offers a thorough breakdown.
Overview of SSC JE
The SSC JE exam consists of two papers:
Paper 1: Computer-based, covering General Intelligence and Reasoning, General Awareness, and Engineering-specific topics.
Paper 2: Written, focusing on engineering discipline specifics.
Importance of Understanding the Syllabus
A thorough comprehension of the curriculum guarantees targeted preparation, minimizes time waste, and optimizes scoring opportunities. Candidates can use it to prioritize topics according to their abilities and discover important areas.
Exam Patterns
The SSC JE exam pattern includes:
Paper 1: 200 marks (General Intelligence & Reasoning, General Awareness, and Engineering Discipline).
Paper 2: 300 marks (Engineering-specific written exam).
Total Time: 2 hours for each paper.
General Intelligence and Reasoning Syllabus
This section tests logical reasoning and problem-solving skills.
Key Topics Covered
Analogies and Similarities
Spatial Orientation
Visual Memory
Arithmetical Reasoning
Coding and Decoding
Syllogism
Tips for Mastering Logical Reasoning
Practice puzzles daily.
Focus on weak areas using targeted practice.
Use reasoning books like R.S. Aggarwal's "A Modern Approach to Verbal & Non-Verbal Reasoning."
Recommended Resources
Online reasoning quizzes
SSC-specific preparation apps
General Awareness Syllabus
This section evaluates a candidate's knowledge of current affairs and static GK.
Static General Knowledge
History and Geography
Indian Polity and Constitution
Economy and Development
Current Affairs
National and International News
Science and Technology Developments
Sports and Awards
Environmental Studies Overview
Topics like biodiversity, climate change, and sustainable development are often included.
SSC JE Civil Engineering Syllabus
Civil engineering is a major discipline in SSC JE.
Structural Engineering Topics
Theory of Structures
RCC Design
Steel Design
Hydraulics and Water Resources Engineering
Fluid Mechanics
Hydrology
Irrigation Systems
Soil Mechanics
Soil Properties
Compaction and Consolidation
Bearing Capacity
SSC JE Electrical Engineering Syllabus
Electrical engineering covers both theoretical and practical aspects.
Basic Electrical Engineering Concepts
DC and AC Circuits
Electrical Machines
Circuit Theory
Power Systems
Transmission and Distribution
Power Generation Methods
Protective Relays
Electronics and Communication
Basics of Semiconductor Devices
Digital Electronics
Microprocessors
SSC JE Mechanical Engineering Syllabus
Mechanical engineering topics require a strong grasp of core concepts.
Thermodynamics
Laws of Thermodynamics
IC Engines
Refrigeration and Air Conditioning
Manufacturing Processes
Casting and Forging
Metal Cutting
Welding Techniques
Machine Design
Design of Gears
Shafts and Bearings
Mechanical Vibrations
Exam Preparation Tips for SSC JE
Preparation requires a systematic approach.
Creating a Study Plan
Allocate time based on topic weightage.
Include breaks to avoid burnout.
Time Management Strategies
Prioritize difficult topics.
Use timers during practice sessions.
Practice Through Mock Tests
Mock tests simulate exam conditions and help identify weaknesses.
Subject-Wise Weightage in SSC JE Examination
Knowing the weightage helps in prioritizing topics.
Civil Engineering Weightage
Structural Analysis: 25%
Hydraulics: 20%
Electrical Engineering Weightage
Circuit Theory: 30%
Power Systems: 25%
Mechanical Engineering Weightage
Thermodynamics: 35%
Manufacturing: 30%
Best Books and Study Materials for SSC JE
Choosing the right resources can make a difference.
Subject-Specific Books
Civil: "Strength of Materials" by B.C. Punmia
Electrical: "Electrical Technology" by H. Cotton
Mechanical: "Theory of Machines" by S.S. Rattan
Online Resources and Mock Tests
Websites offering free test series
Official SSC materials
Coaching Institutes Recommendations
Institutes like NextEngineer provide tailored coaching for SSC JE aspirants.
Importance of Mock Tests and Previous Year Papers
Mock tests and previous year papers offer invaluable insights.
Why Mock Tests Are Crucial
Build speed and accuracy.
Familiarize with the exam pattern.
Analyzing Previous Year Questions
Identify repeating trends and important topics.
Developing Exam Strategies
Learn time management and question prioritization.
Tips to Tackle Time Management During the Exam
Effective time management is critical.
Allocating Time for Each Section
Spend no more than 30 minutes on General Awareness.
Reserve the majority of time for technical sections.
Strategies for Speed and Accuracy
Skip time-consuming questions.
Double-check calculations.
Avoiding Negative Marking
Attempt only those questions you are confident about.
SSC JE Exam Eligibility and Application Process
Understanding eligibility is the first step.
Educational Qualifications
Diploma or degree in engineering.
Discipline-specific qualifications.
Age Criteria
General: 30 years (varies for reserved categories).
Step-by-Step Application Guide
Visit the official SSC website.
Fill out the application form.
Pay the application fee.
How to Stay Motivated During SSC JE Preparation
Motivation keeps the preparation journey smooth.
Setting Realistic Goals
Break your preparation into achievable milestones.
Overcoming Challenges
Seek help for difficult topics.
Stay positive and persistent.
Maintaining Mental Health
Take regular breaks.
Practice mindfulness or meditation.
Frequently Asked Questions about SSC JE Syllabus
1. What is the SSC JE exam pattern?
The exam includes two papers: a computer-based test (Paper 1) and a descriptive paper (Paper 2).
2. Are there any changes in the SSC JE syllabus?
The syllabus remains consistent; refer to the official SSC notification for updates.
3. How to prepare for the technical section?
Focus on core engineering concepts and practice previous year questions.
4. Is coaching necessary for SSC JE?
While self-study can suffice, coaching offers structured guidance and expert tips.
5. How to manage time during the SSC JE exam?
Prioritize high-scoring sections and avoid spending too much time on one question.
6. What are the best books for SSC JE preparation?
Refer to the books mentioned in the "Best Books and Study Materials" section above.
Conclusion and Next Steps
The SSC JE examination is an excellent opportunity for engineering graduates to secure a government job. With a clear understanding of the syllabus and a disciplined preparation strategy, success is within reach. Start your preparation today and aim high!
0 notes
Text
Beginner's Guide to Key Subjects in Civil Engineering for GATE Aspirants: Tips from the Best Gate Coaching Centre in Kerala
Preparing for the GATE Civil Engineering exam can be a daunting task, especially for beginners. To excel, it’s crucial to have a strong foundation in the key subjects and the right guidance. Opting for Gate Civil Online Coaching ensures flexibility while offering expert mentorship. In this blog, we will explore the core subjects that every GATE Civil Engineering aspirant should master and how the Best Gate Coaching Centre in Kerala can help you achieve your goals.
1. Importance of Structural Engineering in GATE Preparation
Structural Engineering forms the backbone of civil engineering and is a high-scoring subject in GATE. Topics like bending moment, shear force, and structural analysis are crucial.
Why focus on it? It constitutes a significant portion of the GATE syllabus and tests your ability to solve practical problems.
How can online coaching help? Platforms offering Gate Civil Online Coaching provide recorded lectures, live sessions, and problem-solving practice, helping you grasp these concepts effectively.
Expert Tip: The Best Gate Coaching Centre in Kerala often includes mock tests specifically tailored for structural engineering to boost your confidence.
2. Mastering Geotechnical Engineering for Conceptual Clarity
Geotechnical Engineering deals with soil mechanics and foundation engineering, subjects often considered tricky.
Why is it important? Questions from this subject are both theoretical and numerical, requiring a clear understanding.
Role of coaching: Online platforms offer interactive tools like animations to explain soil behavior under different conditions, a feature provided by the top centers like the Best Gate Coaching Centre in Kerala.
Preparation Tip: Regular quizzes available through Gate Civil Online Coaching help reinforce concepts and improve your speed.
3. Understanding Environmental Engineering for Scoring High
Environmental Engineering is one of the most straightforward and scoring subjects for GATE aspirants.
Key topics: Water treatment, air pollution, and solid waste management.
Coaching benefits: Through Gate Civil Online Coaching, aspirants gain access to concise notes, which are indispensable for last-minute revisions.
Real-world application: The Best Gate Coaching Centre in Kerala ensures students not only excel in exams but also understand practical applications, enhancing their problem-solving skills.
4. Transportation Engineering: An Essential Scoring Subject
Transportation Engineering is another critical subject for GATE aspirants. It includes topics like highway design, traffic engineering, and pavement materials.
Why focus here? Its practical relevance and straightforward nature make it a scoring area.
How coaching aids: Online platforms provide practice sets and case studies, helping aspirants tackle a variety of questions efficiently.
Pro Strategy: Enrolling in the Best Gate Coaching Centre in Kerala ensures access to resources like solved examples and interactive classes, making learning easier.
5. Revising Hydraulics and Water Resources Engineering
Hydraulics and Water Resources Engineering often intimidate students due to the numerical component.
Key areas to focus on: Fluid mechanics, open channel flow, and hydrology.
How online coaching helps: With Gate Civil Online Coaching, aspirants can revisit difficult topics anytime through recorded sessions.
Boosting Confidence: Mock tests offered by the Best Gate Coaching Centre in Kerala include a variety of numerical problems, helping students improve their problem-solving speed.
Conclusion
Preparing for GATE Civil Engineering requires a strategic approach and a strong grasp of fundamental subjects. With the support of Gate Civil Online Coaching, you can achieve your dreams by gaining access to structured content, expert guidance, and flexible learning schedules. Additionally, choosing the Best Gate Coaching Centre in Kerala ensures you’re equipped with top-notch resources and mentorship to tackle the exam with confidence. Start your preparation today, focus on the key subjects, and unlock the doors to a successful career in civil engineering!
0 notes
Text
Commercial Land Capability Assessments: A Comprehensive Guide
When planning a construction project, especially in rural or semi-urban areas, one of the most critical steps is ensuring the land’s suitability for septic systems and other infrastructure. Victorian Septic Land Capability Assessments (VSLA) provides professional services to evaluate and determine the capacity of land to handle wastewater systems effectively. This blog delves into the details of Commercial Land Capability Assessments, their importance, and what you need to know about them.

What is a Land Capability Assessment?
A Land Capability Assessment (LCA) is an evaluation process that determines the suitability of a specific piece of land for a proposed use, such as the installation of septic systems or construction of commercial properties. It involves analyzing soil, topography, vegetation, and climate to ensure the land can handle wastewater safely without causing environmental harm.
At VSLA, our experts specialize in providing accurate, data-driven assessments tailored to meet regulatory requirements and ensure environmental sustainability.
Why Are Land Capability Assessments Important for Commercial Projects?
Regulatory Compliance: Authorities often mandate LCAs before approving building permits. These assessments ensure your project adheres to local environmental and construction standards.
Environmental Protection: Poorly planned land use can lead to soil degradation, water pollution, and ecosystem damage. LCAs help mitigate these risks by ensuring the land is suitable for its intended purpose.
Project Feasibility: A comprehensive LCA provides insights into the potential challenges of the land, such as drainage issues or soil instability, helping developers make informed decisions.
Cost Efficiency: Identifying potential land limitations early in the planning phase can save significant costs by avoiding redesigns or regulatory penalties.
Commercial Land Capability Assessments
Commercial properties, such as office buildings, factories, or retail spaces, often require robust septic and wastewater management systems. This makes LCAs particularly crucial for such projects.
Key aspects evaluated in a Commercial Land Capability Assessment include:
Soil Permeability: Determines how well the soil absorbs and filters water.
Slope Analysis: Steep slopes can cause erosion and water runoff issues.
Hydrology: Examines surface and groundwater flows to prevent water contamination.
Vegetation Coverage: Analyzes existing vegetation that might impact water retention and soil stability.
VSLA's assessments are tailored to address the unique needs of commercial projects, ensuring a balance between development and environmental stewardship.
Steps Involved in Conducting an LCA
Initial Site Visit and Analysis: Our team inspects the site to collect data on soil composition, vegetation, and topography.
Soil Testing: Laboratory testing is conducted to determine soil properties such as texture, permeability, and nutrient levels.
Hydrological Study: We evaluate surface and groundwater conditions to ensure sustainable wastewater management.
Report Preparation: The findings are compiled into a detailed report, which includes recommendations for septic design, land use, and necessary precautions.
Submission and Approval: The report is submitted to the relevant authorities to obtain necessary permits for your project.
What Makes Victorian Septic Land Capability Assessments Unique?
At VSLA, we prioritize precision, efficiency, and customer satisfaction. Here's why our clients trust us:
Experienced Professionals: Our team of experts has extensive knowledge of local environmental regulations and land assessment techniques.
State-of-the-Art Equipment: We use advanced tools to ensure accurate data collection and analysis.
Tailored Solutions: Every land parcel is unique. We provide customized recommendations based on the specific needs of your project.
Timely Delivery: We understand the importance of timelines in commercial projects and ensure prompt delivery of our reports.
Common Challenges in Commercial LCAs and How to Overcome Them
Inadequate Soil Quality: If soil lacks the necessary permeability for septic systems, consider solutions like engineered fill or advanced treatment systems.
High Water Table: A high water table can pose risks for septic installation. Mitigation strategies include raised systems or alternative drainage solutions.
Steep Slopes: Terracing or retaining walls can help manage erosion and stabilize the site.
Regulatory Hurdles: With VSLA’s in-depth understanding of Victorian regulations, we ensure your assessment meets all legal requirements, minimizing approval delays.
Understanding the Costs of Land Capability Assessments
The cost of a Land Capability Assessments varies depending on factors such as:

Size of the land parcel
Complexity of the site
Type of project (e.g., commercial, residential)
Additional testing requirements
While LCAs represent an upfront investment, they save significant costs in the long term by preventing issues like system failures or environmental fines.
Tips for Developers: Preparing for an LCA
Share Detailed Project Plans: Provide comprehensive information about your intended development to help the assessor focus on relevant aspects.
Clear the Site: Remove debris or overgrown vegetation to facilitate accurate testing.
Engage Professionals Early: Hiring VSLA early in the planning process ensures timely identification of potential land limitations.
Conclusion
Victorian Septic Land Capability Assessments is your trusted partner in ensuring the success of your commercial projects. Our thorough evaluations not only ensure compliance with local regulations but also safeguard the environment and the long-term viability of your development.
Whether you’re planning a new office, retail outlet, or industrial facility, our expertise in Commercial Land Capability Assessments provides the foundation for informed decision-making. Contact us today to learn more about our services and how we can help your project succeed.
0 notes
Text
Understanding Land Capability Assessment (LCA) Services and Their Costs
Land Capability Assessments (LCA) play a pivotal role in ensuring sustainable land use and environmental compliance. Whether you're developing a property or planning agricultural activities, understanding LCA services and their associated costs is crucial for informed decision-making. This blog explores what LCA services entail, why they are essential, and what factors influence their costs.

What is a Land Capability Assessment (LCA)?
A Land Capability Assessment Cost is a scientific evaluation that determines the suitability of land for specific uses, such as residential development, farming, or wastewater management. It involves analyzing soil quality, topography, vegetation, and water resources to assess the land's capacity to support proposed activities without causing environmental harm.
LCA is particularly vital for properties in rural or semi-urban areas where proper sewage treatment, stormwater management, or agricultural viability must be ensured.
Why Are LCA Services Essential?
1. Environmental Compliance
Many local councils and regulatory bodies require an LCA as part of development applications. These assessments ensure that your project aligns with environmental sustainability standards.
2. Sustainable Land Use
An LCA helps avoid soil erosion, water contamination, and loss of biodiversity. It ensures that the land is utilized without compromising its ecological balance.
3. Optimized Development
By identifying the land's capabilities and limitations, LCA services provide insights into the best practices for construction, agriculture, or other activities.
4. Long-term Cost Savings
Identifying potential risks early on can save developers and property owners from costly rectifications in the future.
Key Components of LCA Services
LCA services involve a multidisciplinary approach, combining expertise from environmental science, hydrology, and engineering. Here's what typically goes into an LCA:
1. Soil Testing
Analyzing soil composition, texture, and permeability.
Assessing its ability to absorb and treat wastewater.
2. Topographical Analysis
Studying the land’s slope and elevation to determine drainage patterns.
3. Hydrological Assessment
Identifying water sources, groundwater levels, and drainage systems.
4. Vegetation Survey
Evaluating existing flora and fauna to understand the ecological impact.
5. Risk Analysis
Identifying risks such as erosion, flooding, or salinity that may affect the land’s usability.
6. Recommendations
Providing solutions for septic system installation, irrigation management, or other necessary modifications.
Factors Influencing Land Capability Assessment Cost
The cost of an LCA varies depending on several factors:
1. Property Size
Larger properties require more extensive surveys, soil sampling, and analysis, leading to higher costs.
2. Land Complexity
Land with diverse topography or challenging environmental conditions may necessitate detailed studies, increasing the cost.
3. Purpose of Assessment
An LCA for residential development may differ in scope and detail compared to one for agricultural or industrial use, impacting the cost.
4. Regulatory Requirements
Local council guidelines and environmental regulations often dictate the level of detail required, influencing assessment costs.
5. Expertise and Equipment
Hiring highly skilled professionals and using advanced equipment can lead to more accurate results but may also increase expenses.
6. Report Customization
Tailored reports with specific recommendations or additional data may add to the overall cost.
Typical Cost Range for LCA Services
While costs can vary widely, here's a general breakdown of what to expect:
Basic LCA Services: $1,500 - $3,000
Comprehensive LCA Services: $3,500 - $6,000
Highly Complex Assessments: $6,000+
It’s important to request detailed quotes from service providers to understand what’s included in the price.
Choosing the Right LCA Services Provider
Selecting the right provider is crucial for ensuring accurate and actionable results. Here are some tips:
1. Check Credentials
Look for qualified environmental consultants with experience in LCA.
2. Review Past Projects
Ask for references or case studies to evaluate the provider’s expertise.
3. Compare Costs
While cost is a factor, prioritize quality over price to avoid incomplete or inaccurate assessments.
4. Ensure Regulatory Compliance
Verify that the provider is familiar with local council and state regulations.
Benefits of Investing in High-Quality LCA Services
Although the upfront cost of LCA Services might seem significant, the long-term benefits outweigh the expenses:

Reduced Environmental Impact: LCA ensures that your project minimizes harm to the surrounding environment.
Improved Property Value: Proper land use planning can enhance the value and functionality of your property.
Regulatory Approvals: A thorough LCA report facilitates smoother approval processes with local authorities.
Informed Decision-Making: With detailed insights into land capabilities, you can make strategic and sustainable choices.
How Septic Land Capability Assessment Can Help
At Septic Land Capability Assessment, we specialize in providing reliable and cost-effective LCA services tailored to your project needs. Our team of experts combines cutting-edge technology with years of experience to deliver comprehensive reports that meet local regulations and exceed expectations.
Why Choose Us?
Accurate Assessments: We use advanced soil testing methods and GIS tools for precise results.
Affordable Pricing: Transparent pricing structures with no hidden costs.
Timely Delivery: We understand the importance of meeting deadlines and ensure prompt service.
Customer Support: Our team is always available to answer your queries and guide you through the process.
Conclusion
A Land Capability Assessment is an indispensable step in sustainable land management and development. By understanding the scope of LCA services and their costs, you can plan your projects effectively while adhering to environmental regulations. Investing in high-quality LCA services not only ensures compliance but also protects the long-term value and usability of your land.
Contact Septic Land Capability Assessment today to learn more about our LCA services and get a customized quote for your project. Let’s work together to create sustainable and thriving developments!
0 notes
Text
How to Choose the Right Flood Risk Assessment Consultant for Your Needs
In an era where climate change has heightened the risks of flooding, the need for professional guidance in assessing these risks has become paramount. Flood risk assessment consultants play a crucial role in helping property owners understand their vulnerabilities and develop strategies to mitigate potential flooding impacts. However, selecting the right consultant can be a daunting task. This article provides a comprehensive guide on how to choose the right flood risk assessment consultant tailored to your specific needs.
Understanding the Role of Flood Risk Assessment Consultants
Before delving into the selection process, it is essential to comprehend what flood risk assessment consultants do. These professionals specialize in evaluating the likelihood of flooding in a given area and the potential consequences for properties and infrastructure. Their expertise encompasses a range of services, including data collection, site evaluations, hydrological modeling, and the development of mitigation strategies.
A well-qualified consultant will not only assess the current flood risks but also consider future scenarios influenced by climate change and urban development. They provide property owners with critical insights that inform decision-making, ensuring that investments are safeguarded against potential flood damage.
Identifying Your Specific Needs
The first step in choosing the right consultant is to identify your specific needs. Are you a residential property owner concerned about potential flooding? Or perhaps you are part of a commercial development project that requires a comprehensive flood risk assessment? Understanding your unique situation will help narrow down the pool of consultants.
Additionally, consider the scope of the assessment you require. Some consultants specialize in specific areas, such as residential properties, commercial developments, or infrastructure projects. Knowing what type of expertise you need will guide your search for the right professional.
Evaluating Qualifications and Experience
Once you have a clear understanding of your needs, the next step is to evaluate the qualifications and experience of potential flood risk assessment consultants. Look for professionals who hold relevant certifications and accreditations in environmental science, civil engineering, or hydrology. These qualifications indicate a level of expertise and a commitment to industry standards.
Experience is another critical factor. A consultant with a proven track record in conducting flood risk assessments in similar contexts will likely be more adept at identifying potential issues and providing effective solutions. Review case studies or client testimonials to gauge their success in previous projects. This will help you ascertain whether they have the necessary experience to address your specific concerns.
Assessing Methodologies and Tools
Flood risk assessment consultants utilize various methodologies and tools to conduct their evaluations. It is essential to inquire about the approaches they employ and the technologies they use for data collection and analysis. A reputable consultant should be familiar with modern hydrological modeling techniques and geographic information systems (GIS) that enhance the accuracy of flood risk assessments.
Understanding their methodologies will also give you insight into how thorough and comprehensive their assessments will be. Ask potential consultants to explain their process in detail, including how they gather data, assess vulnerabilities, and develop mitigation strategies. This transparency reflects their professionalism and commitment to delivering high-quality services.
Communication and Collaboration
Effective communication is crucial when working with a flood risk assessment consultant. You will want to ensure that the consultant is approachable and willing to engage in an open dialogue. A consultant who takes the time to listen to your concerns and answer your questions demonstrates a commitment to your project and an understanding of its unique challenges.
Additionally, consider how the consultant collaborates with other stakeholders, such as local governments, engineers, and environmental specialists. A consultant who fosters collaboration can provide a more comprehensive assessment and better integrate their findings into broader flood risk management strategies.
Understanding Costs and Deliverables
Cost is an inevitable consideration when selecting a flood risk assessment consultant. It is essential to obtain detailed quotes from multiple consultants to understand the pricing structure. Keep in mind that the lowest price may not always equate to the best value. Instead, focus on the quality of services offered, the consultant's experience, and the comprehensiveness of their assessments.
Moreover, clarify what deliverables you can expect from the consultant. A thorough flood risk assessment should culminate in a detailed report outlining the findings, potential risks, and recommended mitigation measures. Ensure that the consultant provides a clear timeline for the assessment process and outlines key milestones along the way.
Seeking Recommendations and Conducting Interviews
When in doubt, seeking recommendations from trusted sources can be invaluable. Reach out to colleagues, industry peers, or local organizations involved in environmental management. Their experiences and insights may lead you to reputable flood risk assessment consultants who have successfully served similar needs.
Once you have a shortlist of potential consultants, conduct interviews to gauge their fit for your project. Prepare a list of questions that cover their experience, methodologies, and approach to communication. This interaction will allow you to assess their professionalism and determine whether they align with your expectations.
Trusting Your Instincts
While qualifications, experience, and methodologies are essential factors in choosing the right consultant, trusting your instincts is equally important. A strong rapport and a sense of confidence in the consultant's abilities will contribute to a successful working relationship. If you feel comfortable and confident in their capabilities, it is likely a sign that you have found the right fit.
Conclusion: Making an Informed Choice
Choosing the right flood risk assessment consultant is a crucial step in safeguarding your property against potential flood risks. By understanding the role of consultants, identifying your specific needs, evaluating qualifications, and assessing communication, you can make an informed choice that aligns with your goals.
For those navigating this complex process, partnering with experienced professionals can make all the difference. Consulting with specialists in flood risk assessments can provide the guidance needed to protect your investments and ensure safety. As you consider your options, remember that expertise and collaboration are key to a successful assessment and effective flood risk management.
#flood risk assessment#flood risk assessments#flood risk assessment consultants#flood risk assessment planning
0 notes
Text
All this pavement has direct environmental consequences.
One is simply the cost of producing it all: the production of cement is responsible for almost 10 percent of global greenhouse gas emissions, and lots and garages are part of that total, as is all the infrastructure required to serve the sprawl.
A second is the loss of natural land to suburban development. America lost 460,000 acres of wetlands, for example, every year in the 1950s and ’60s, and 290,000 acres a year in the ’70s and ’80s. This transition is associated with steep declines in animal density, especially of birds and bugs. This is happening even in the oldest and slowest-growing parts of the country: Massachusetts, for example, developed more land in the last five decades of the twentieth century than in the three hundred years prior to 1950.
A third is the urban heat island effect. Pavements and roofs absorb energy from the heat-generating elements of urban life, such as buildings and vehicles, which means that cities both warm up faster and cool down slower than natural areas.
A fourth is flooding. A city is, among other things, a complex new hydrological zone, where water collects and flows in unpredictable ways. In sprawling Houston, fifty years of growing faster than any city in America have sealed a Belgium-sized section of Texas grassland beneath asphalt, concrete, and lawn. Flat as a tile and nearly as resistant to water, Houston is the epicenter of the urban flooding epidemic in the United States. When the stronger and more frequent rainstorms caused by climate change pass over this heavily populated region just inland from the Gulf of Mexico, the water has nowhere to go. Various studies have estimated that “impervious” cover such as parking lots may increase runoff and flooding by up to a factor of ten. In the two decades preceding Hurricane Harvey, greater Houston grew by 2.7 million people—the equivalent of adding an entire city of Chicago to the metro area in just twenty years, mostly on former prairie, farmland, and forest. Approximately 770 square miles were developed in this time, with about half of the land becoming impervious. From 1996 to 2011, impervious surface in Harris County increased by a quarter, and from 1992 to 2010, the area lost almost a third of its wetlands—about sixteen thousand acres. In Brays Bayou, one of the city’s major creeks, rainfall has increased by 26 percent over the past forty years—but runoff is up 204 percent. Runoff has tripled because the region has paved its way into an unmapped, manmade floodplain. Subdivisions built upstream are causing hundred-year-old houses downstream to flood for the first time. It’s a problem that goes far beyond Houston. Cities like Chicago and Saint Louis have dug huge underground tunnels to hold all the rain. In Philadelphia and Seattle, homeowners have adopted rain barrels and cultivated native plants. Everywhere, architects have installed green roofs and city planners have replaced the desiccated pits of street trees with reedy green swales. Even the engineers of parking lots are doing their best, adapting to stormy weather with porous materials like gravel or crushed clay.
A fifth consequence of all this pavement is water pollution. Outside of cities, runoff from roads and parking lots often goes straight into lakes and streams. In the northern part of the country, in the winter and spring, this runoff is contaminated with road salt. In the summer, heated by the smoldering blacktop, it can drain ten to twenty degrees hotter than it fell. In all seasons, it is contaminated by pollutants such as motor oil, rubber dust from tires, animal droppings, pesticides, air pollution residue, and heavy metals. Jurisdictions are frantically trying to slow this phenomenon; by 2020 they had created more than 1,800 stormwater utilities in the United States that try to treat or divert runoff away from sensitive habitats.
A sixth is groundwater absorption, the flip side of flooding. The largest stormwater utility of all is funded by a “driveway tax” in sunny Los Angeles, of all places, which in 2021 put a 2.5-cent annual levy on every square foot of pavement. It is not enough money to encourage greener building practices at scale—but will raise enough across the county to build infrastructure to keep the city’s precious rainstorms from being flushed down its concrete river directly into the Pacific Ocean. That will keep the beaches clean. But it will also, crucially, preserve millions of gallons of stormwater that could be used for nonpotable uses like watering lawns and keeping saltwater out of underground aquifers. During difficult times, it could be filtered and put back into the pipes.
0 notes
Text
The Backbone of Water Supply: Unveiling the Science of Canal and Dam Surveys
Often water is referred to as the essence of life, so it is also the backbone of agriculture, industry, and daily living. As populations grow and climate change alters precipitation patterns, the demand for reliable water supply systems has never been more critical. At the heart of effective water supply management lies the intricate science of canal and dam surveys. In this blog, we will explore the importance of these surveys, the methodologies involved, and how Dolphin Engineers play a pivotal role in ensuring sustainable water management.
Understanding Canal and Dam Surveys
Canal and dam surveys are comprehensive assessments conducted to evaluate the design, construction, and maintenance of water conveyance and storage systems. These surveys provide essential data that informs engineers, planners, and policymakers about the current state of water infrastructure and its capacity to meet future demands.
Why Are Canal and Dam Surveys Important?
Resource Management: Accurate surveys help in understanding water availability, distribution, and storage capacities. It is very crucial to have effective resource management, especially in regions that are prone to drought or flooding.
Safety Assessments: Dams are critical structures that must be regularly assessed to ensure they can withstand environmental stresses. Surveys help identify potential weaknesses and inform necessary reinforcements.
Environmental Impact: Surveys assess the ecological impact of water infrastructure on surrounding ecosystems. This information is vital for sustainable development practices that balance human needs with environmental preservation.
Regulatory Compliance: Governments and regulatory bodies often require detailed surveys to ensure compliance with safety and environmental regulations. This ensures that water supply systems operate within legal frameworks and adhere to safety standards.
The Survey Process: Techniques and Technologies
Canal and dam surveys involve a combination of traditional surveying techniques and modern technology.
Here are some of the key methods to apply:
1. Topographic Surveys:
Using total stations and GPS technology, engineers create detailed topographic maps of the land surrounding canals and dams. This data is crucial for understanding the terrain and planning construction or maintenance activities.
2. Hydrological Studies:
Hydrological surveys assess water flow, quality, and availability. They involve collecting data on rainfall, evaporation, and river flow rates to predict how much water can be stored or diverted.
3. Geotechnical Investigations:
Understanding the soil and rock composition is vital for dam stability. Geotechnical surveys involve drilling and sampling to analyze the ground conditions where dams and canals will be constructed or maintained.
4. Remote Sensing and Drones:
Advancements in drone technology and remote sensing allow for aerial surveys that can cover vast areas quickly and efficiently. This technology provides high-resolution images and data that can be analyzed for various purposes, including vegetation mapping and land use analysis.
5. Structural Assessments:
Regular inspections of existing structures are essential to identify wear and tear. Engineers use various tools, including ultrasonic testing and structural health monitoring systems, to evaluate the integrity of dams and canals.
Dolphin Engineers: Pioneering Water Management Solutions
At Dolphin Engineers, we recognize the critical role that canal and dam surveys play in sustainable water management. Our team of experienced engineers and surveyors employs cutting-edge technology and innovative methodologies to ensure that water infrastructure is resilient, efficient, and environmentally responsible.
Our Commitment to Excellence
Expertise: With years of experience in the field, our team is equipped to handle projects of any scale, from small irrigation canals to large-scale dam constructions.
Sustainability: We prioritize sustainable practices in all our projects, ensuring that our water management solutions benefit both communities and the environment.
Collaboration: We work closely with local governments, communities, and stakeholders to develop tailored solutions that meet specific water supply needs.
Conclusion
The science of canal and dam surveys is vital to ensuring a reliable and sustainable water supply for future generations. As we face increasing challenges related to water scarcity and climate change, the role of engineers and surveyors in this field becomes even more critical. At Dolphin Engineers, we are committed to advancing water management practices through innovative surveying techniques and a deep understanding of the complexities involved. Together, we can build a more sustainable future, one survey at a time.
For more information on our services and how we can assist with your water management projects, visit us at https://www.dolphinengineers.com/.
#WaterManagement#SustainableWater#CanalSurvey#DamSurvey#Hydrology#WaterEngineering#InfrastructureSafety#EnvironmentalSustainability#ResourceManagement#ClimateResilience#DolphinEngineers#HydrologicalStudies#GeotechnicalSurvey#RemoteSensing#StructuralIntegrity#DroneTechnology#WaterResources#SustainableFuture#CivilEngineering#WaterSupply
0 notes
Text
Electrical Resistivity Testing Company in India Epitome
When it comes to understanding the subsurface properties of soil and rock formations, Electrical Resistivity Testing is a widely used and highly effective geophysical method. In India, where diverse geological conditions exist, this method plays a crucial role in various sectors, such as construction, mining, environmental studies, and groundwater exploration. Epitome, a leading Electrical Resistivity Testing Company in India, provides reliable and precise resistivity testing services to meet the complex needs of industries across the country.

What is Electrical Resistivity Testing? Electrical Resistivity Testing is a method used to determine the subsurface characteristics by measuring the resistance of the ground to the flow of electrical current. It is a non-invasive and cost-effective way to analyze the composition and structure of the earth beneath the surface, offering valuable data for geotechnical, environmental, and hydrological investigations.
In simple terms, different soil and rock types have varying abilities to conduct electricity. By injecting a controlled electrical current into the ground and measuring the resulting voltage, experts can infer the resistivity of the materials below. This resistivity can then be used to map out the subsurface, identifying materials such as clay, sand, rock, or water-bearing formations.
Applications of Electrical Resistivity Testing Groundwater Exploration: Identifying potential aquifers and underground water sources is essential in regions with limited water supply. Electrical resistivity testing helps locate water-bearing formations, making it a crucial tool for water resource management.
Geotechnical Investigations: Construction projects require detailed information about the subsurface before foundation work begins. Resistivity testing can detect soil types, bedrock, and voids, ensuring that the construction site is suitable for building.
Environmental Studies: This testing method is used to assess contamination in soils and groundwater, aiding environmental scientists in identifying polluted zones and providing data for remediation efforts.
Mining: In mineral exploration, electrical resistivity testing can locate mineral deposits and assess the quality of underground resources, helping mining companies make informed decisions about where to dig.
Archaeological Investigations: The technique is also applied in archaeology to detect buried structures or artifacts without the need for excavation.
Why Choose Epitome for Electrical Resistivity Testing? As one of India’s premier geotechnical service providers, Epitome brings years of experience and expertise in conducting electrical resistivity tests across various industries. Here’s why Epitome stands out:
Cutting-Edge Technology: Epitome uses advanced equipment and state-of-the-art technology to conduct accurate and reliable resistivity surveys, ensuring precise results.
Experienced Team: The company boasts a team of highly qualified geophysicists and engineers who have extensive experience in interpreting resistivity data, allowing them to provide insights that are crucial for decision-making.
Customized Solutions: Epitome understands that no two projects are the same. The company offers tailor-made resistivity testing solutions to meet the specific needs of each client, whether for groundwater detection, construction, or environmental assessments.
Nationwide Service: Epitome operates across India, providing services to a wide range of sectors, from infrastructure development to agriculture. No matter where your project is located, Epitome can deliver the required expertise.
Sustainability: With a focus on environmental responsibility, Epitome ensures that all testing methods are performed with minimal impact on the surrounding ecosystem, making it a preferred choice for companies committed to sustainability.
How Does the Process Work? Epitome’s electrical resistivity testing process is efficient and straightforward:
Site Evaluation: The first step involves assessing the site to determine the best approach for conducting the test.
Electrode Placement: Electrodes are placed in the ground in a predefined array to introduce and measure electrical currents.
Data Collection: Electrical current is injected into the ground, and the resulting voltage differences are measured to gather data on subsurface resistivity.
Data Interpretation: The data is then analyzed to create a resistivity profile of the subsurface, identifying any anomalies or points of interest.
Report Generation: Epitome provides clients with detailed reports, including graphical representations of the resistivity data and expert interpretations to aid in planning and decision-making.
Conclusion Whether you are embarking on a large infrastructure project, seeking groundwater resources, or conducting an environmental study, electrical resistivity testing provides vital data about the subsurface. With Epitome’s proven track record and expert team, you can trust that you’ll receive accurate, dependable results that inform and enhance your project.
More Info : https://epitomegs.com Contact : +91-96756 94400
#epitomegs#ElectricalResistivityImaginginIndia#ElectricalResistivityTomographyCompanyinIndia#TomographySurveyinIndia#ERTsurveycompanyinIndia#ERTsurveyinIndia#ElectricalResistivityTestingCompanyinIndia#ERTsurvey#ElectricalResistivityImaging#ElectricalResistivityTomography
0 notes