Don't wanna be here? Send us removal request.
Photo
When cavitation bubbles collapse, they can produce temperatures well over 2,000 Kelvin. Since cavitation near a surface can be so destructive, researchers have long wondered whether the high temperatures inside the bubble can be transmitted to nearby surfaces. A new set of numerical simulations provides some insight into that process. The researchers found that collapsing cavitation bubbles raised nearby wall temperatures in two ways: bubbles that were further away sent shock waves that heated the material, and nearby bubbles could contact the surface itself as they collapsed.
Heat transfer requires time, however; this is part of why quickly dunking your hand in liquid nitrogen and pulling it out likely won’t damage you. (Still, we don’t recommend it.) The cavitation bubbles could only transmit these high temperatures for less than 1 microsecond, which means that most materials won’t actually heat up to their melting temperature. The researchers did conclude, however, that softer materials exposed to frequent bubble collapses could show localized melting under the barrage. (Image credit: L. Krum; research credit: S. Beig et al.)
154 notes
·
View notes
Photo

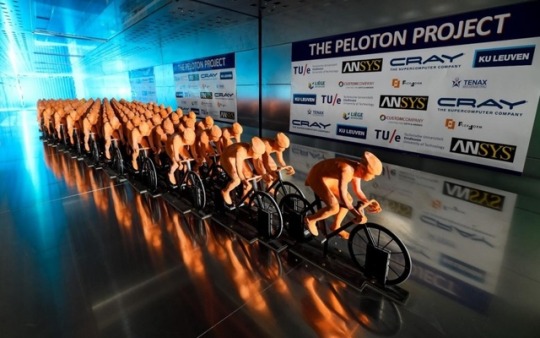

It’s well-known by professional cyclists that sitting in the middle of the peloton requires little effort to overcome aerodynamic drag, but now, for the first time, there’s a scientific study to back that up. Researchers built their own quarter-scale peloton of 121 riders to investigate the aerodynamic effect of cycling in such a large group versus riding solo. Through wind tunnel studies and numerical simulation, they found that riders deep in the peloton can experience as little as 5-10% of the aerodynamic drag of a solo cyclist.
Tactically, this means teams should aim to position their protected leader or sprinter mid-way in the pack, where they’ll receive lots of shelter without risking one of the crashes common near the back of the peloton. It also suggests that teams wanting to isolate another team’s leader should try to push them toward the outer edges of the peloton rather than letting them sit in the middle. It will be interesting to see whether pro teams shift their race strategies at all with these numbers in hand.
Of course, this study considers only a pure headwind. But other groups are looking at the effects of side winds on cyclists. (Image credit: J. Miranda; image and research credit: B. Blocken et al.; submitted by 1307phaezr)
381 notes
·
View notes
Video
vimeo
“Volumes” is an experimental art film by Maxim Zhestkov using physics-based particle animation. Waves and unseen forces send billions of color-changing particles aloft in the film. The motions – especially the way the particles seem to tear themselves – are reminiscent of a complex fluid, like yogurt. These substances have both liquid-like (viscous) and solid-like (elastic) properties depending on the forces they experience. Zhestkov’s particles are similar; they move like a fluid but tear more like a solid.
I particularly like the sequence beginning at 1:30. The upwelling of particles leaves behind a lower layer that looks like a snapshot of convection in a planetary mantle while the upper layer resembles the clash of ocean waves. The whole film is quite mesmerizing. Check it out! (Video and image credit: M. Zhestkov; GIFs via Colossal)
870 notes
·
View notes
Photo
Theo Jansen’s New Strandbeest Roams the Beach Like an Undulating Caterpillar
4K notes
·
View notes
Photo
Nearly every lab has a magnetic stirrer for mixing fluids, but this ubiquitous tool still holds some surprises, like its ability to unexpectedly levitate. Magnetic stirrers consist of two main parts, a driving magnet that creates a rotating magnetic field, and a bar magnet – commonly referred to as the flea – that is submerged in the fluid to be stirred. When the driver’s rotating field is active, the flea will spin at the bottom of its container, keeping its magnetic field in sync with the driver.
But if you place the flea in a viscous enough fluid, the drag forces on the flea can pull it out of sync with the driver’s field. Above a certain speed, the flea will jump so that its field repulses the driver’s. That makes the flea levitate as it spins. Depending on the interplay of viscous and magnetic forces, that spin can be unstable (left) or stable (right). The researchers suggest that this peculiar behavior could help artificial swimmers propel themselves or lead to new methods for measuring fluid viscosity. (Image and research credit: K. Baldwin et al.; via APS; submitted by Kam-Yung Soh)
442 notes
·
View notes
Photo

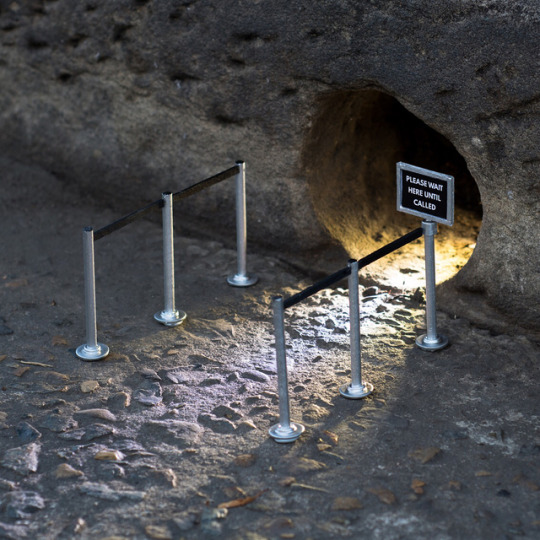
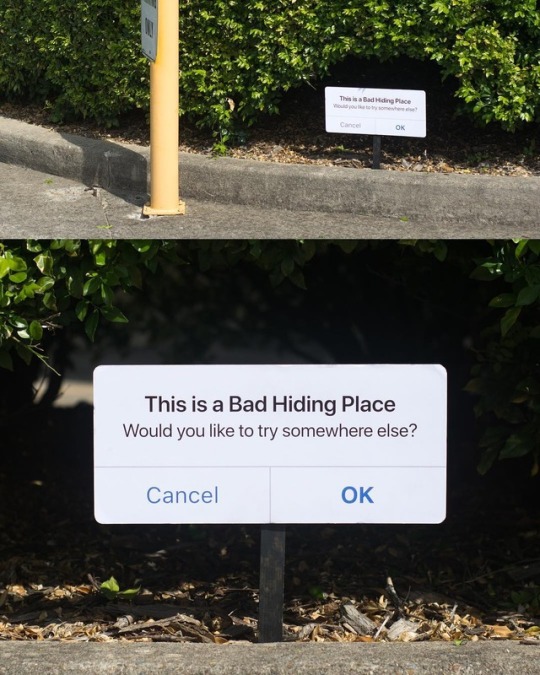
New Miniature Warning Signs and Other Humorous and Unexpected Interventions by Michael Pederson
5K notes
·
View notes
Video
376 notes
·
View notes
Video
youtube
Earlier this fall, I attempted my first corn maze. It didn’t work out very well. Early on I unknowingly cut through an area meant to be impassable and thus ended up missing the majority of the maze. Soap, as it turns out, is a much better maze-solver, taking nary a false turn as it heads inexorably to the exit. The secret to soap’s maze-solving prowess is the Marangoni effect.
Soap has a lower surface tension than the milk that makes up the maze, which causes an imbalance in the forces at the surface of the liquid. That imbalance causes a flow in the direction of higher surface tension; in other words, it tends to pull the soap molecules in the direction of the highest milk concentration. But that explains why the soap moves, not how it knows the right path to take. It turns out that there’s another factor at work. Balancing gravitational forces and surface tension forces shows that the soap tends to spread toward the path with the largest surface area ahead. That’s the maze exit, so Marangoni forces pull the soap right to the way out! (Video credit: F. Temprano-Coleto et al.; research credit: F. Peaudecerf et al.)
ETA: Based on the latest research results, gravity may play less of a role than originally thought. Instead, it seems as though the soap chooses its path in part through pre-existing background levels of surfactant. As the dye advances, it compresses the background surfactant, decreasing the local surface tension until it is in equilibrium with dyed area. Because longer paths take longer to reach that equilibrium, the dye spreads preferentially toward the largest surface area.
393 notes
·
View notes
Video
youtube
Pascal’s Law tells us that pressure in a fluid depends on the height and density of the fluid. This is something that you’ve experienced firsthand if you’ve ever tried to dive in deep water. The deeper into the water you swim, the greater the pressure you feel, especially in your ears. Go deep enough and the pressure difference between your inner ear and the water becomes outright painful.
In the video demonstration above, you’ll see how a tall, thin tube containing only 1 liter of water is able to shatter a 50-liter container of water. Not only does this show just how powerful height is in creating pressure in a fluid, but it shows how a fluid can be used to transmit pressure over a distance – one of the fundamental principles of hydraulics! (Video credit: K. Visnjic et al.; submitted by Frederik B.)
696 notes
·
View notes
Photo

Designs by Marcus Martinez
Marcus Martinez aka @isopoly is a young, freelance graphic designer from Harbor City,CA. He creates gifs using Cinema 4D, Adobe After Effects, Adobe Illustrator and Photoshop. Marcus was spotted by Tumblr radar 12 times!
Stay up to date with isopoly’s work by follow him on Twitter and Tumblr. via
Spice up your timeline by following us on Facebook!
posted by Margaret
2K notes
·
View notes
Note
What are some of your favorite architecture, art and design blogs?
Too many!
Here are some of my favorite art and design blogs:
@really-shit
@burningstandard
@turecepcja
@irakalan
@myampgoesto11
@lustik
@crossconnectmag
@travelingcolors
@definitelydope
@shamefullyinspired
@worclip
806 notes
·
View notes
Photo
Earth’s oceans are a complex and dynamic environment, but fortunately, we can simulate some of their physics on a smaller scale in the laboratory. The time series of images above show how fresh and salty waters mix. On the right side of the image is fresh water with its top layer dyed green. On the left is salty water dyed pink. Initially, the fresh water spreads horizontally toward the salty region in a smooth and laminar fashion. As the fresh water picks up salt, it gets denser and starts sinking, ultimately forming a turbulent plume that will push all the way back across the tank. For more images, check out the full poster. (Image credit: P. Passaggia et al.)
347 notes
·
View notes
Photo
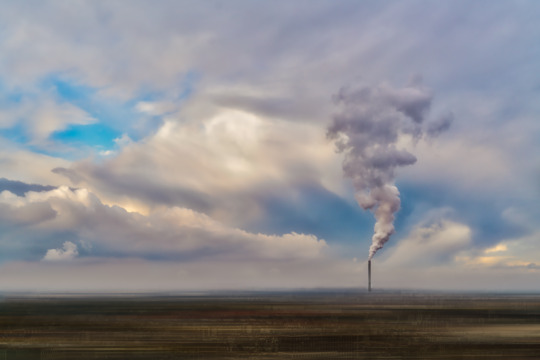
First Impressions
This smokestack was one of my first impressions of Amsterdam as I took a cab to the hotel. I shared the ride with another traveller who asked our driver about it. Slightly annoyed, the driver replied that it was obviously a power plant. Perhaps a lot of people ask the same question upon first arrival. (more)
140 notes
·
View notes