#light microscopy awards
Explore tagged Tumblr posts
Text

Nervous system of a juvenile sea star (Patiria miniata) about 1 cm wide. Labeled with an antibody against acetylated tubulin after optical clearing, and captured using a color-coded Z-projection.
By Laurent Formery (USA).
Light Microscopy Awards
#laurent formery#photographer#united states#light microscopy awards#micro photography#juvenile sea star#patiria miniata#nature
100 notes
·
View notes
Text

What is this, you ask? Why, of course, it's the nervous system of a juvenile sea star (Patiria miniata) about 1 cm wide. Labeled with an antibody against acetylated tubulin after optical clearing, and captured using a color-coded Z-projection.
No, but seriously folks, check out the link above for more amazing miniature beauty.
8 notes
·
View notes
Text

Global Winner, Laurent Formery (USA).
A look at the inner workings of a sea star won the Global Image of the Year Scientific Light Microscopy Award. The contest is organised by Evident and Olympus, since 2017, the contest has been an incredible way to highlight the artistry and scientific value of light microscopy.
“This is a fantastic feeling,” Formery shared. “Winning the global award feels like an incredible achievement and shows I made progress. I love microscopy and can spend a huge amount of time in front of our confocal microscope, but the very nice samples that I am lucky to work with really make the difference. I work with marine invertebrates, in particular echinoderms (sea stars, sea urchins, and their kind). They are beautiful animals, with a fascinating and aesthetically pleasing fivefold symmetry that is unlike anything else in the animal kingdom.
I’m happy that taking images of them helps communicate how much beauty we have in our oceans, and why it is important to know more about them and protect them.”
Courtesy:My Modern Met
#communication #awareness #natural #light #microscopy #photography
8 notes
·
View notes
Text

Fluorescence
Fluorescence is the emission of light by a substance that has absorbed light or electromagnetic radiation, typically visible light or UV. This phenomenon occurs when electrons in a molecule absorb energy, become excited to a higher energy state, and then release energy as they return to the ground state. Fluorescence is widely applied in scientific research, imaging, and diagnostics, including fluorescence microscopy, flow cytometry, and fluorescent labeling in biomolecular studies.
International Chemistry Scientist Awards
Website: chemistryscientists.org
Contact us: [email protected]
Nominate now: https://chemistryscientists.org/award-nomination/?ecategory=Awards&rcategory=Awardee
#sciencefather#researchawards#Professor,#Lecturer,#Scientist,#Scholar,#Researcher #Fluorescence #PhotonEmission #MolecularImaging #FluorescentLabeling #UVLight #Spectroscopy #ScientificResearch #BiomolecularAnalysis #FluorescenceMicroscopy #FlowCytometry #LightScience #QuantumMechanics #Bioimaging #PhysicsInBiology
👉 Don’t forget to like, share, and subscribe for more exciting content!
Get Connected Here: =============
Blogger : https://www.blogger.com/blog/post/edit/6961521080043227535/467226973388921229
Twitter : https://x.com/chemistryS79687
Pinterest : https://in.pinterest.com/chemistryaward/
Instagram: https://www.instagram.com/alishaaishu01/
Youtube : https://www.youtube.com/channel/UCAD_pDvz3ZHqv_3hf-N0taQ
0 notes
Text
Fwd: Graduate position: LundU.MicrobialEvolutionAnaerobicLife
Begin forwarded message: > From: [email protected] > Subject: Graduate position: LundU.MicrobialEvolutionAnaerobicLife > Date: 28 March 2024 at 04:42:54 GMT > To: [email protected] > > > Dear all, > > We’re looking for a PhD student to join our team at Lund University > (Sweden) to study how microbial eukaryotes - ‘protists' - live > without oxygen. The position is set to start in the Autumn of 2024, > but is flexible per agreement. > > Details about the position and how to apply (including > specific instructions for the cover letter) can be found here: > https://ift.tt/0rSAewJ. > > THE PROJECT > Oxygen-depleted environments are more than just “anoxic dead zones” > – they are home to a diverse array of life, including eukaryotic > microbes. This PhD project aims to explore the mitochondrial biology in > anaerobic eukaryotes, shedding light on how their metabolism and organelle > function have evolved to thrive in oxygen-free environments. The focus > will be on the electron transport chain and other electron transferring > strategies like hydrogen generation. We will investigate the functional > diversity of anaerobic respiratory chains in eukaryotes and explore the > evolutionary mechanisms that contribute to this adaptations including > lateral gene transfer and neofunctionalization. Additional projects > related to environmental anaerobic eukaryotes are also available. > > THE ORGANISMS > We study unicellular eukaryotes – or ‘protists’ – that often have > peculiar biology compared to what our textbooks teach us. This project > will centre on protists from the Breviatae and Amoebozoa phyla that have > taken different evolutionary trajectories towards anaerobic life. Instead > of depositing electrons onto oxygen, these protists can make hydrogen > gas or succinate which can be consumed by prokaryotic organisms in a > form of metabolic co-operation known as ‘syntrophy’. Your task will > be to help us follow the electrons and understand the basic cell biology > of these protists. > > RESEARCH METHODS > This project will use cell biological methods, including super-resolution > microscopy, proteomics, and metabolomics of quinone molecules. Moreover, > there is potential to develop expertise in bioinformatic methods such > as transcriptomics, comparative genomics, and phylogenetics as well as > environmental microbiology. > > SUPERVISION > The successful candidate will join the Molecular Cell Biology Division > in the Biology Department at Lund University, under the supervision of > Dr. Courtney Stairs, an expert in eukaryotic microbiology. The project > will also be co-supervised by Dr. Olivier van Aken, a leading researcher > in mitochondrial biology. This project is part of the European Research > Council ‘TANGO2’ StG project, awarded to Dr. Stairs. > > Best wishes, > Courtney > > > Courtney Stairs > Biträdande Universitets Lektor > > Lund University > Department of Biology > https://ift.tt/062OPMV > > > > > Courtney Stairs
0 notes
Text
MIT community members honored with 2024 Franklin Institute Awards
New Post has been published on https://thedigitalinsider.com/mit-community-members-honored-with-2024-franklin-institute-awards/
MIT community members honored with 2024 Franklin Institute Awards
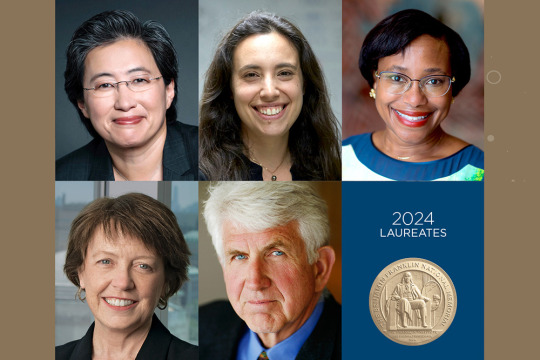
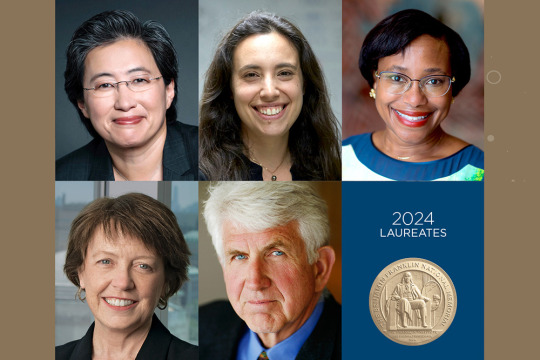
The Franklin Institute recently announced its 2024 cohort of award winners, as part of its bicentennial celebration. Since its inception, the Franklin Institute Awards Program has honored the most influential scientists, engineers, and inventors who have significantly advanced science and technology. It is one of the oldest comprehensive science awards in the world.
The 2024 honorees include Institute Professor and Vice Provost for Faculty Paula T. Hammond ’84 PhD ’93; Associate Professor Gabriela S. Schlau-Cohen; Research Affiliate Robert Metcalfe ’69; Mary Boyce SM ’84, PhD ’87; and Lisa Su ’90, SM ’91, PhD ’94. All 2024 Franklin Institute Award Laureates will be celebrated in a ceremony on April 18 at the Benjamin Franklin National Memorial of the Franklin Institute.
Paula Hammond was awarded the 2024 Benjamin Franklin Medal in Chemistry, one of the oldest comprehensive science awards in the world. The award cites her “innovative methods to create novel materials one molecular layer at a time and for applying these materials to areas ranging from drug delivery to energy storage.” Hammond’s techniques for creating thin polymer films and other materials using layer-by-layer assembly is groundbreaking. They can be used to build polymers with highly controlled architectures by alternately exposing a surface to positively and negatively charged particles. Materials can then be designed for many different applications, including drug delivery, regenerative medicine, noninvasive imaging, and battery technologies. Hammond is the recipient of MIT’s 2023-24 Killian Award and, in 2021, was named an Institute Professor, MIT’s highest faculty honor. Hammond is one of only 25 people who have been elected to all three U.S. National Academies — Engineering, Science, and Medicine.
Gabriela Schlau-Cohen earned the Benjamin Franklin NextGen Award for “illuminating the fundamental chemical processes that protect plants from sun damage, uncovering novel approaches to increasing crop yields.” Schlau-Cohen combines tools from chemistry, optics, biology, and microscopy to develop new approaches to probe dynamics. Her group focuses on dynamics in membrane proteins, particularly photosynthetic light-harvesting systems that are of interest for sustainable energy applications. Following a postdoc at Stanford University, Schlau-Cohen joined the Department of Chemistry faculty in 2015. She earned a bachelor’s degree in chemical physics from Brown University in 2003 followed by a PhD in chemistry at the University of California at Berkeley.
Robert Metcalfe ’69, a research affiliate of the MIT Computer Science and Artificial Intelligence Laboratory and MIT Corporation life member emeritus, won the Benjamin Franklin Medal in Electrical Engineering for “his pioneering role in the design, development, and commercialization of Ethernet, an interface for networking and file sharing between computers.” MetCalfe is a graduate of MIT’s Department of Electrical Engineering and Computer Science (EECS) and is a former president of the MIT Alumni Association.
Mary Boyce SM ’84, PhD ’87 won the Benjamin Franklin Medal in Mechanical Engineering for “transformative contributions to our understanding of the physical behavior of polymers, materials made of long chains of molecules, leading to innovative product development of rubber and other soft materials.” A longtime MIT faculty member and former head of MIT’s Department of Mechanical Engineering, Boyce is currently a professor of mechanical engineering and provost emerita of Columbia University.
Lisa Su ’90, SM ’91, PhD ’94, a graduate of MIT’s Department of EECS and the current president, CEO, and chair of AMD, won the Bower Award for Business Leadership for “her transformational leadership of AMD, a leader in high-performance and adaptive computing and one of the fastest growing semiconductor companies in the world.”
#2023#2024#Alumni/ae#amd#applications#artificial#Artificial Intelligence#Awards#honors and fellowships#battery#Behavior#Benjamin Franklin#Biology#Business#Business leadership#CEO#chemical#Chemical engineering#chemistry#Community#Companies#comprehensive#computer#Computer Science#Computer Science and Artificial Intelligence Laboratory (CSAIL)#computers#computing#Design#development#drug
0 notes
Text
Zoom-In on Talent: How Microscopy is Revolutionizing the World of Life Science Recruiter

Let’s play with this idea: have you ever imagined a parallel universe where your job as a life sciences recruiter hinges upon your ability to appreciate the striking beauty of a mouse brain slice under a microscope? Sounds peculiar, doesn’t it? Well, the reality might not be as far-fetched as it seems. Know everything here with advice of the best life science recruiter.
Microscopy, the fascinating science of magnified wonders, is redefining the rules of the game in life sciences recruiter. The ability to study the minutest details of the natural world is not just inspiring awe, but it’s also creating a ripple effect in the world of recruitment. In this post, we’ll dive into the nucleus of this curious phenomenon. Let’s begin, shall we?
Life Sciences Recruiters Embrace Microscopy
Microscopy and life science recruiter share a relationship as old as time, but the concept of integrating microscopy into the recruitment process is relatively new. Life sciences recruiters are starting to realize that a candidate’s prowess in microscopy can serve as a litmus test for various skills such as precision, patience, and understanding of biological complexities.
When a candidate showcases an intricate, beautifully crafted slice of a mouse brain under a microscope, it sends a clear message about their mastery of detail, thoroughness, and technical expertise. Not to mention, their ability to remain patient through the painstaking process of preparing, staining, and imaging.
The allure of the microscopic world is not just for the scientific community anymore, it’s steadily capturing the imagination of life sciences recruiters. Know all about data management in life sciences.
The Microscopy Prize and Its Unexpected Impact
A microscopy competition might appear, at first glance, to be just another scientific contest. But, the recent Olympus Global Image of the Year Award sent ripples through the life sciences recruitment sector.
Ainara Pintor’s striking image of an immunostained mouse-brain slice, dubbed “Neurogarden,” not only showcased her scientific expertise but also her creativity and artistry. Her win emphasized the importance of unique, talented individuals who can merge scientific rigor with artistic vision. That’s the perfect combination that life sciences recruiters are actively seeking. The contest opened up new horizons for recruiters, enabling them to scout for talent in places they hadn’t considered before.
Going Beyond Borders and Disciplines
The power of microscopy knows no bounds, and this has significant implications for life sciences recruiters. With the globalization of science, talent can spring from any corner of the world, and the recent Olympus contest serves as an apt testament to this fact.
From Howard Vindin’s intriguing autofluorescence image of a mouse embryo that fetched him the Asia-Pacific regional prize to Alan Prescott’s arresting image of a mouse’s frozen head that won the European award, life sciences recruiters are learning that talent is a global phenomenon. Their talent-seeking endeavors are no longer restricted by geographical or disciplinary boundaries, paving the way for a diverse, multi-faceted talent pool.
Unearthing the Hidden Gems in Microscopy
Not all talent receives the limelight it deserves. This is as true in the world of microscopy as it is in the recruitment field. Honorable mentions in the Olympus contest, although they did not take center stage, presented a plethora of unique subjects. From photonic crystals in insect scales to desert locust wings, these beautiful images shed light on the lesser-explored avenues of microscopy.
Life sciences recruiters see these honorable mentions as uncut diamonds, brimming with potential. They understand that these unsung heroes of the microscopic world can bring fresh perspectives and skills to their organizations, spurring innovation and progress.
Evolving Requirements in Life Sciences Recruitment
Life sciences recruiters are increasingly looking for candidates who can blend their artistic sensibility with scientific prowess. An engaging microscopic image, as life sciences recruiters understand, is not just a result of technique but also of aesthetics.
Factors like composition, lighting, exposure, and post-processing are all part of the package. Candidates who can master this blend are fast becoming the most sought-after professionals in the life sciences domain.To know more about this contact us today.
The Future of Microscopy in Life Sciences Recruitment
The future holds exciting possibilities. As the field of microscopy evolves, so too will the demands and expectations of life sciences recruiters. The ability to produce striking microscopic images will no longer be a bonus but a prerequisite. Life sciences recruiters will increasingly look for individuals who can push the boundaries of what’s possible with a microscope, ultimately transforming the landscape of life sciences recruitment.
Conclusion
As the arena of life sciences recruitment rapidly evolves, microscopy is proving to be a game-changer. It’s not just about exploring the unknown world of tiny wonders but about unearthing the rich potential within life sciences professionals. Life sciences recruiters, equipped with their new microscope-aided perspective, are poised to bring in a new wave of talent that will push the boundaries of research and innovation with Agile Search.
So, next time you look at an impressive microscopic image, remember, it might just be the ticket to an exciting opportunity in the world of life sciences. Life sciences recruiters, it seems, are now playing a whole new ball game, under a microscope.
Website : https://agilesearchinc.com/sb/revolutionizing-life-science-recruitment-with-microscopy/
0 notes
Text
0 notes
Text
Advanced Light Microscopy Symposium at CNSI.
Join us for the first annual Lightfest with the Advanced Light Microscopy Symposium at CNSI. A celebration of UNESCO's International Day of Light 2023, this event is held on May 16th in honor of Theodore Maiman, who fired the first laser right here in southern California on May 16th 1960. This one-day symposium will feature invited talks from a selection of light microscopy users, poster presentations and awards, an image contest, and vendor booths. This event is free and open to anyone, we hope to see you there!
Lightfest! Advanced Light Microscopy Symposium 2023.Date: 16/05/2023 - 16/05/2023, Location: Los Angeles, USA
#Advanced Light#international day of light#16 may#microscopy#symposium#Theodore Maiman#first laser#Lightfest#UCLA#UCLA Health#United States#California NanoSystems Institute
0 notes
Photo

The Future of Quantum Biology
by Adriana Marais , Betony Adams , Andrew K. Ringsmuth , Marco Ferretti , J. Michael Gruber , Ruud Hendrikx , Maria Schuld , Samuel L. Smith , Ilya Sinayskiy , Tjaart P. J. Krüger , Francesco Petruccione and Rienk van Grondelle Published:14 November 2018
Abstract
Biological systems are dynamical, constantly exchanging energy and matter with the environment in order to maintain the non-equilibrium state synonymous with living. Developments in observational techniques have allowed us to study biological dynamics on increasingly small scales. Such studies have revealed evidence of quantum mechanical effects, which cannot be accounted for by classical physics, in a range of biological processes. Quantum biology is the study of such processes, and here we provide an outline of the current state of the field, as well as insights into future directions.
1. Introduction
Quantum mechanics is the fundamental theory that describes the properties of subatomic particles, atoms, molecules, molecular assemblies and possibly beyond. Quantum mechanics operates on the nanometre and sub-nanometre scales and is at the basis of fundamental life processes such as photosynthesis, respiration and vision. In quantum mechanics, all objects have wave-like properties, and when they interact, quantum coherence describes the correlations between the physical quantities describing such objects due to this wave-like nature.
In photosynthesis, respiration and vision, the models that have been developed in the past are fundamentally quantum mechanical. They describe energy transfer and electron transfer in a framework based on surface hopping. The dynamics described by these models are often ‘exponential’ and follow from the application of Fermi’s Golden Rule [1,2]. As a consequence of averaging the rate of transfer over a large and quasi-continuous distribution of final states the calculated dynamics no longer display coherences and interference phenomena. In photosynthetic reaction centres and light-harvesting complexes, oscillatory phenomena were observed in numerous studies performed in the 1990s and were typically ascribed to the formation of vibrational or mixed electronic–vibrational wavepackets. The reported detection of the remarkably long-lived (660 fs and longer) electronic quantum coherence during excitation energy transfer in a photosynthetic system revived interest in the role of ‘non-trivial’ quantum mechanics to explain the fundamental life processes of living organisms [3]. However, the idea that quantum phenomena—like coherence—may play a functional role in macroscopic living systems is not new. In 1932, 10 years after quantum physicist Niels Bohr was awarded the Nobel Prize for his work on the atomic structure, he delivered a lecture entitled ‘Light and Life’ at the International Congress on Light Therapy in Copenhagen [4]. This raised the question of whether quantum theory could contribute to a scientific understanding of living systems. In attendance was an intrigued Max Delbrück, a young physicist who later helped to establish the field of molecular biology and won a Nobel Prize in 1969 for his discoveries in genetics [5].
All living systems are made up of molecules, and fundamentally all molecules are described by quantum mechanics. Traditionally, however, the vast separation of scales between systems described by quantum mechanics and those studied in biology, as well as the seemingly different properties of inanimate and animate matter, has maintained some separation between the two bodies of knowledge. Recently, developments in experimental techniques such as ultrafast spectroscopy [6], single molecule spectroscopy [7–11], time-resolved microscopy [12–14] and single particle imaging [15–18] have enabled us to study biological dynamics on increasingly small length and time scales, revealing a variety of processes necessary for the function of the living system that depend on a delicate interplay between quantum and classical physical effects.
Quantum biology is the application of quantum theory to aspects of biology for which classical physics fails to give an accurate description. In spite of this simple definition, there remains debate over the aims and role of the field in the scientific community. This article offers a perspective on where quantum biology stands today, and identifies potential avenues for further progress in the field.
2. What is quantum biology?
Biology, in its current paradigm, has had wide success in applying classical models to living systems. In most cases, subtle quantum effects on (inter)molecular scales do not play a determining role in overall biological function. Here, ‘function’ is a broad concept. For example: How do vision and photosynthesis work on a molecular level and on an ultrafast time scale? How does DNA, with stacked nucleotides separated by about 0.3 nm, deal with UV photons? How does an enzyme catalyse an essential biochemical reaction? How does our brain with neurons organized on a sub-nanometre scale deal with such an amazing amount of information? How do DNA replication and expression work? All these biological functions should, of course, be considered in the context of evolutionary fitness. The differences between a classical approximation and a quantum-mechanical model are generally thought to be negligible in these cases, even though at the basis every process is entirely governed by the laws of quantum mechanics. What happens at the ill-defined border between the quantum and classical regimes? More importantly, are there essential biological functions that ‘appear’ classical but in reality are not? The role of quantum biology is precisely to expose and unravel this connection.
Fundamentally, all matter—animate or inanimate—is quantum mechanical, being constituted of ions, atoms and/or molecules whose equilibrium properties are accurately determined by quantum theory. As a result, it could be claimed that all of biology is quantum mechanical. However, this definition does not address the dynamical nature of biological processes, or the fact that a classical description of intermolecular dynamics seems often sufficient. Quantum biology should, therefore, be defined in terms of the physical ‘correctness’ of the models used and the consistency in the explanatory capabilities of classical versus quantum mechanical models of a particular biological process.
As we investigate biological systems on nanoscales and larger, we find that there exist processes in biological organisms, detailed in this article, for which it is currently thought that a quantum mechanical description is necessary to fully characterize the behaviour of the relevant subsystem. While quantum effects are difficult to observe on macroscopic time and length scales, processes necessary for the overall function and therefore survival of the organism seem to rely on dynamical quantum-mechanical effects at the intermolecular scale. It is precisely the interplay between these time and length scales that quantum biology investigates with the aim to build a consistent physical picture.
Grand hopes for quantum biology may include a contribution to a definition and understanding of life, or to an understanding of the brain and consciousness. However, these problems are as old as science itself, and a better approach is to ask whether quantum biology can contribute to a framework in which we can repose these questions in such a way as to get new answers. The study of biological processes operating efficiently at the boundary between the realms of quantum and classical physics is already contributing to improved physical descriptions of this quantum-to-classical transition.
More immediately, quantum biology promises to give rise to design principles for biologically inspired quantum nanotechnologies, with the ability to perform efficiently at a fundamental level in noisy environments at room temperature and even make use of these ‘noisy environments’ to preserve or even enhance the quantum properties [19,20]. Through engineering such systems, it may be possible to test and quantify the extent to which quantum effects can enhance processes and functions found in biology, and ultimately answer whether these quantum effects may have been purposefully selected in the design of the systems. Importantly, however, quantum bioinspired technologies can also be intrinsically useful independently from the organisms that inspired them.
3. Quantum mechanics: an introduction for biologists
At the beginning of the twentieth century, the success of classical physics in describing all observable phenomena had begun to be challenged in certain respects. In 1900, as a means to explain the spectral energy distribution of blackbody radiation, Planck introduced the idea that interactions between matter and electromagnetic radiation of frequency ν are quantized, occurring only in integer multiples of hν, where h is the fundamental Planck constant. Five years later, Einstein further developed the notion of energy quantization by extending it to include the photon, a quantum of light. This concept is illustrated by the photoelectric effect where light incident on a material leads to the emission of electrons. It is, however, not the intensity of the light that determines this emission but rather its frequency. Even low-intensity light of a suitable frequency will lead to electrons being emitted whereas high-intensity light below this threshold frequency will have no effect. Einstein explained this by proposing that in this instance light behaves as a particle rather than a wave, with discrete energies hν that can be transferred to the electrons in a material. Bohr’s 1913 model of the hydrogen atom, with its discrete energy states, and Compton’s 1923 work with X-rays all contributed to the beginning of a new era in modern physics. These ways of explaining blackbody radiation and the photoelectric effect, as well as atomic stability and spectroscopy, led to the development of quantum mechanics, a theory that has proved extremely successful in predicting and describing microphysical systems [21,22].
Whereas Planck and Einstein began the quantum revolution by postulating that radiation also demonstrates particle-like behaviour, de Broglie, in 1923, made the complementary suggestion that matter itself has wave-like properties, with a wavelength related to its momentum through Planck’s constant. This hypothesis suggested that matter waves should undergo diffraction, which was subsequently proved by experiments that demonstrated that particles such as electrons showed interference patterns. Schrödinger built on this observation in his formulation of quantum mechanics, which describes the dynamics of microscopic systems through the use of wave mechanics. The formulation of quantum mechanics allows for the investigation of a number of important facets of a quantum state: its mathematical description at any time t, how to calculate different physical quantities associated with this state and how to describe the evolution of the state in time [21,22].
87 notes
·
View notes
Text

Autofluorescence of the tip of the stamens of flowers at 405 nm, 488 nm, and 561 nm, emitting a greenish blue and emerald green color like peacock feathers.
By Mei Yu (China)
Light Microscopy Awards
#mei yu#photographer#china#light microscopy awards#micro photography#autofluorescence of the tip of the stamens of flowers#nature
52 notes
·
View notes
Photo

This cute little fuzzling is a colony of the freshwater cyanobacteria, Gloeotrichia. 😍 Central nitrogen-fixing hetrocyte cells attach to a protruding trichome filament (the part we can see) covered by a mucilage matrix. The bacteria play an important role in nitrogen and phosphorus cycles. Some species can be harmful to humans, but others are being investigated for their potential roles as a renewable energy source. This micrograph was created using a darkfield illumination technique, and placed 6th in @olympuslifescience's 2005 Global Life Science Light Microscopy Award. 📷 : Spike Walker
111 notes
·
View notes
Photo

Microbial Multiverse
Diversity is everywhere, from animal and plant populations down to the mix of microbes in a single glass of seawater. Each of these green blobs is a highly diverse microscopic community of bacteria grown from a single seawater sample, feeding on nutrient patches in the lab. Even though they all came from the same starting liquid, the individual colonies have gone their own way, each creating a distinctive pattern of growth. By using high-powered microscopy and genetic analysis to study these miniature ecosystems up close, researchers can start to unpick how individual groups of diverse bacteria interact with each other as their communities develop and grow. Understanding more about the rules governing the formation and evolution of these collectives could shed light on microbial societies in the wider world, including bacterial colonies that are important for the natural environment and those that may be harmful – or helpful – to human health.
Written by Kat Arney
Microbial Multiverse: Dropping in on Diversity; A winner in the 2020 Koch Institute Image Awards
Image by Rachel Soble; The Parsons Laboratory for Environmental Science and Engineering in the Department of Civil and Environmental Engineering MIT
Image courtesy of the Koch Institute Image Awards
You can also follow BPoD on Instagram, Twitter and Facebook
15 notes
·
View notes
Photo

New Method for Detecting the Invisible Properties of Nano-Structured Light Fields
Researchers combine nano-optics and organic chemistry to measure complex light landscapes in the tight focus of a laser beam in a study published in Nature Communications.
Structured laser light has already opened up various different applications: it allows for precise material machining, trapping, manipulating or defined movement of small particles or cell compartments, as well as increasing the bandwidth for next-generation intelligent computing.
If these light structures are tightly focused by a lens, like a magnifying glass used as burning glass, highly intense three-dimensional light landscapes will be shaped, facilitating a significantly enhanced resolution in named applications. These kinds of light landscapes have paved the way to pioneering applications as Nobel prize awarded STED microscopy.
However, these nano-fields itself could not be measured yet, since components are formed by tight focusing which are invisible for typical measurement techniques. Up to now, this lack of appropriate metrological methods has impeded the breakthrough of nano-structured light landscapes as a tool for material machining, optical tweezers, or high-resolution imaging.
Read more.
35 notes
·
View notes
Text
Colorful Image Lights Up Microscopic Guts of 'Water Bear'
https://sciencespies.com/news/colorful-image-lights-up-microscopic-guts-of-water-bear/
Colorful Image Lights Up Microscopic Guts of 'Water Bear'
Tardigrades—eight-legged, microscopic “water bears” known for surviving in extreme conditions—can be pretty photogenic, given the right lighting. Last month, an image of a tardigrade’s insides lit up by fluorescent stains was named an Olympus “Global Image of the Year” award winner for 2019, reports Mindy Weisberger for Live Science.
Biologist Tagide deCarvalho, manager of the Keith Porter Imaging Facility at the University of Maryland Baltimore County (UMBC), created the award-winning photo of the microscopic animal, the University announced in a statement. Since tardigrades are mostly colorless, deCarvalho used fluorescent dye molecules to stain the tardigrade’s internal structures, revealing an eye-popping look inside a creature that typically grows no longer than one millimeter in length.
“I knew the moment I saw this colorful specimen that it was going to be a remarkable image,” deCarvalho says in a statement. “I love sharing the fascinating things I see in the microscope with other people.”
Tardigrades, also known as “moss piglets,” have earned a bit of a cult status over the years for being difficult to kill and “endearingly tubby,” reports Weisberger. In 2012, Smithsonian magazine reported that while the water bears generally live on moist pieces of moss or in the sediment on the bottom of lakes, they can also survive at both 212 degrees Fahrenheit and 459 degrees below zero. They’ve also been found enduring intense pressure at the bottom of the ocean floor.
To protect themselves, the animals curl their bodies into a compact pill shape, become completely dehydrated and secrete chemicals that form a protective shell called a tun. This process, known as “vitrification,” effectively mummifies the animal and allows it to survive even in outer space, reports Brian Resnick for Vox.
As Jenny O’Grady writes for UMBC Magazine, deCarvalho calls the specialized process of creating microscopic images with fluorescent molecules “Sci-Art.”
“I’m able to produce so much color in my images by using multiple fluorescent stains and capitalizing on the natural fluorescence of the samples,” deCarvalho says in a statement. “I’m excited about this image because the fluorescent dyes I used allow you to see the tardigrade digestive tract, including the mouthparts and stomach filled with food.”
Her image earned first place in the Americans region of the Olympus Global Life Science Light Microscopy contest, which “aim[s] to celebrate both the artistic and scientific value of microscopy images,” per a statement. The top pick for Global Image of the Year went to a brightly colored image of a slice of mouse’s brain, created by Ainara Pintor, a doctoral candidate at the Basque Center for Biophysics in Spain.
Like this article? SIGN UP for our newsletter
#News
#04-2020 Science News#2020 Science News#Earth Environment#earth science#Environment and Nature#Nature Science#News Science Spies#Our Nature#outrageous acts of science#planetary science#Science#Science Channel#science documentary#Science News#Science Spies#Science Spies News#Space Physics & Nature#Space Science#News
1 note
·
View note
Text
Riding Sound Waves in the Brain - Technology Org
New Post has been published on https://thedigitalinsider.com/riding-sound-waves-in-the-brain-technology-org/
Riding Sound Waves in the Brain - Technology Org
ETH Zurich researchers have shown for the first time that microvehicles can be steered through blood vessels in the brains of mice using ultrasound. They hope this will eventually lead to treatments capable of precisely delivering drugs.
Neuroscience, brain – associative rendering. Image credit: Milad Fakurian via Unsplash, free license
Brain tumours, brain haemorrhages and neurological and psychological conditions are often hard to treat with medication. And even when effective drugs are available, these tend to have severe side effects because they circulate throughout the brain and not just the area they are meant to treat.
In light of this situation, researchers have high hopes of one day providing a more targeted approach to deliver medications to specific locations. To this end, they are in the process of developing mini-transporters that can be guided through the dense maze of blood vessels.
Researchers at ETH Zurich, the University of Zurich and the University Hospital Zurich have managed for the first time to guide microvehicles through the blood vessels in an animal’s brain using ultrasound.
Ultrasound instead of magnetism
Compared to alternative navigation technologies such as those based on magnetic fields, ultrasound offers certain benefits. Daniel Ahmed, Professor of Acoustic Robotics at ETH Zurich and supervisor of the study, explains: “In addition to being widely used in the medical field, ultrasound is safe and penetrates deep into the body.”
For their microvehicle, Ahmed and his colleagues used gas-filled microbubbles coated in lipids – the same substances that biological cell membranes are made of. The bubbles have a diameter of 1.5 micrometres and are currently used as contrast material in ultrasound imaging.
As the researchers have now shown, these microbubbles can be guided through blood vessels.
“Since these bubbles, or vesicles, are already approved for use in humans, it’s likely that our technology will be approved and used in treatments for humans more quickly than other types of microvehicles currently in development,” Ahmed says. He was awarded a Starting Grant by the European Research Council ERC in 2019 for his project to research and develop this technology.
Another benefit of the ultrasound-guided microbubbles is that they dissolve in the body once they’ve done their job. When using another approach, magnetic fields, the microvehicles have to be magnetic, and it’s not easy to develop biodegradable microvehicles. Moreover, the microbubbles developed by the ETH Zurich researchers are small and smooth.
“This makes it easy for us to guide them along narrow capillaries,” says Alexia Del Campo Fonseca, a doctoral student in Ahmed’s group and lead author of the study.
Going against the flow
Over the past few years, Ahmed and his group have been working in the lab to develop their method for guiding microbubbles through narrow vessels.
Now, in collaboration with researchers from the University of Zurich and University Hospital Zurich, they have tested this method on blood vessels in the brains of mice. The researchers injected the bubbles into the rodents’ circulatory system, where they are swept along in the bloodstream without any outside help.
However, the researchers managed to use ultrasound to hold the vesicles in place and guide them through the brain vessels against the direction of blood flow. The researchers were even able to guide the bubbles through convoluted blood vessels or get them to change direction multiple times in order to steer them into the narrowest branches of the bloodstream.
Blood vessels in the brain with clusters of microvehicles in orange (microscopy image). Image credit: Del Campo Fonseca et al., Nature Communications 2023
To control the microvehicles’ movements, the researchers also attached four small transducers to the outside of each mouse’s skull. These devices generate vibrations in the ultrasonic range, which spread through the brain as waves. At certain points in the brain, the waves emitted by two or more transducers can either amplify each other or cancel each other out.
The researchers guide the bubbles using a sophisticated method of adjusting the output of each individual transducer. Real-time imaging shows them what direction the bubbles are moving in.
To create the imaging for this study, the researchers used two-photon microscopy. In the future, they also want to use ultrasound itself for imaging and plan to enhance ultrasound technology for this purpose.
In this study, the microbubbles were not equipped with medications. The researchers first wanted to show that they could guide the microvehicles along blood vessels and that this technology is suitable for use in the brain. That’s where there are promising medical applications, including in treating cancer, stroke and psychological conditions.
The researchers’ next step will be to attach drug molecules to the outside of the bubble casing for transport. They want to enhance the entire method to the point at which it can be used in humans, hoping it will one day provide the basis for developing new treatments.
Source: ETH Zurich
You can offer your link to a page which is relevant to the topic of this post.
#2023#Aging news#applications#approach#biodegradable#Biotechnology news#blood#blood vessels#bloodstream#Brain#brains#bubbles#Cancer#cell#change#clusters#Collaboration#communications#development#devices#direction#drug#drug delivery#drugs#easy#effects#ETH Zurich#Future#gas#Health & medicine news
0 notes