#Metal Oxide Semiconductor Field Effect Transistor
Explore tagged Tumblr posts
Text
https://www.futureelectronics.com/p/semiconductors--discretes--transistors--mosfets/2n7002p-215-nexperia-4004769
Nexperia, 2N7002P,215, Transistors, Mosfets
2N7002P Series N-Channel 60 V 1.6 Ohm 350 mW 0.8 nC SMT TrenchMOS FET - SOT-23
#Nexperia#2N7002P#215#Transistors#Mosfets#Mosfet transistor#High voltage mosfet#N Channel Power MOSFET#gate#n-channel mosfet#Mosfet circuits#Field Effect Transistor#Metal Oxide Semiconductor Field Effect Transistor
1 note
·
View note
Text
Metal Oxide Semiconductor, Field Effect Transistor, Power MOSFET
N-Channel 20 V 1 A 0.45 Ω Surface Mount Enhancement Mode Power MosFet - SOT-323
#Diodes Incorporated#DMG1012UW-7#Transistors#Mosfets#Metal Oxide Semiconductor#Field Effect Transistor#Power MOSFET#Ultra-Small MOSFET Transistors#MOSFET types#MOSFET as a switch#Mosfet transistor#mosfet gate#power mosfet#mosfet vs transistor
1 note
·
View note
Text
She applies Silicon Oxynitride to my Polycrystalline Silicon till I Metal-Oxide-Semiconductor Field-Effect Transistor
23 notes
·
View notes
Photo

Beyond Moore's Law: Innovations in solid-state physics include ultra-thin 2D materials and more
In the ceaseless pursuit of energy-efficient computing, new devices designed at UC Santa Barbara show promise for enhancements in information processing and data storage.
Researchers in the lab of Kaustav Banerjee, a professor of electrical and computer engineering, have published a new paper describing several of these devices, "Quantum-engineered devices based on 2D materials for next-generation information processing and storage," in the journal Advanced Materials. Arnab Pal, who recently received his doctorate, is the lead author.
Each device is intended to address challenges associated with conventional computing in a new way. All four operate at very low voltages and are characterized as being low leakage, as opposed to the conventional metal-oxide semiconductor field-effect transistors (MOSFETs) found in smartphones that drain power even when turned off. But because they are based on processing steps similar to those used to make MOSFETs, the new devices could be produced at scale using existing industry-standard manufacturing processes for semiconductors.
Read more.
19 notes
·
View notes
Text
sorry my metal-oxide-semiconductor field-effect transistor doesnt support spotify wrapped. yeah i live like this. sorry
2 notes
·
View notes
Text
Tesla Cybertruck hit with sixth recall this year, this time over a bad inverter
Image: Cath Virginia / The Verge, Getty Images The Tesla Cybertruck was recalled for the sixth time this year — and the fix won’t involve an easy software update. Tesla voluntarily issued a recall for approximately 2,431 Cybertrucks manufactured between November 6, 2023 and July 30, 2024. The issue is related to something called the metal-oxide-semiconductor field-effect transistors, or MOSFET,…
0 notes
Text
Understanding MOS Relays: The Future of Switching Technology - MANGOFY
In the world of electronics, the need for efficient, reliable, and fast switching is ever-growing. Traditional mechanical relays, while useful in many applications, have limitations like slower response times, mechanical wear, and the need for regular maintenance. This is where MOS relays, a solid-state alternative, come into play.
What is a MOS Relay?
A MOS relay (also known as a MOSFET relay) is a type of solid-state relay that uses a MOSFET (Metal-Oxide-Semiconductor Field-Effect Transistor) to perform switching functions. Unlike mechanical relays, MOS relays do not have moving parts, making them more durable, faster, and more efficient in many applications.
Key features of MOS relays include:
No Mechanical Wear: Since there are no moving parts, MOS relays do not suffer from the wear and tear associated with mechanical relays.
Fast Switching Times: MOS relays can switch on and off rapidly, which is essential in applications requiring high-speed operations.
Low Power Consumption: Because MOS relays use semiconductor technology, they consume significantly less power compared to traditional relays.
Noise-Free Operation: MOS relays operate silently, which is a key advantage in noise-sensitive applications.
How Do MOS Relays Work?
MOS relays utilize a photovoltaic coupler to control a MOSFET. When a small control signal (usually a low-voltage signal like from a microcontroller) is applied to the input, it activates the LED within the relay. The light from the LED generates a voltage in the photovoltaic element, which in turn controls the MOSFET, allowing or blocking current flow in the output circuit.
This method of switching provides:
Electrical Isolation: The control signal is isolated from the switched circuit, which enhances safety and reduces the risk of interference.
Precision Control: MOS relays offer fine control over switching, ideal for sensitive applications.
Applications of MOS Relays
MOS relays have a wide range of applications, particularly in situations where high precision, reliability, and fast switching are required. Here are a few areas where MOS relays are commonly used:
Test Equipment: In precision measurement and testing equipment, MOS relays are used for switching signals without introducing noise or signal degradation. Their high speed and long lifespan make them ideal for automated test systems.
Telecommunications: In telecommunications infrastructure, MOS relays are employed for switching high-speed data signals. Their ability to switch quickly and without noise is crucial for maintaining signal integrity in high-frequency applications.
Automotive Electronics: MOS relays are increasingly used in automotive applications where reliability and durability are paramount. With the rise of electric and hybrid vehicles, MOS relays are used in systems like battery management, electric motor control, and various safety systems.
Industrial Automation: In factories and automated environments, MOS relays are employed for controlling motors, actuators, and sensors. Their durability and speed make them ideal for applications where fast switching is necessary to keep up with automated processes.
Renewable Energy Systems: Solar power systems, for example, often use MOS relays in their inverter circuits and battery management systems. The low power consumption and fast switching times help improve the efficiency and longevity of these systems.
MOS Relays vs. Mechanical Relays
Advantages of MOS Relays:
Durability: Without moving parts, MOS relays have a much longer operational life.
Speed: Mechanical relays are slower due to the physical movement required to make or break a connection. MOS relays, being solid-state, can switch in microseconds.
Power Efficiency: MOS relays consume less power, making them ideal for energy-conscious applications like battery-powered devices.
Noise-Free Operation: Since MOS relays do not physically move, they operate silently, which is critical in applications like medical devices and audio equipment.
Disadvantages of MOS Relays:
Cost: MOS relays tend to be more expensive than mechanical relays due to their advanced technology.
Current Rating: Mechanical relays can handle higher currents compared to MOS relays, which may have limitations in high-power applications.
Why MOS Relays are Crucial in Modern Electronics
As electronics continue to evolve toward more compact, efficient, and reliable systems, MOS relays are becoming an essential component. Their solid-state nature makes them more suitable for modern applications, where mechanical parts may be prone to failure and inefficiencies. Whether it's in consumer electronics, industrial automation, or renewable energy, MOS relays offer a compelling alternative to traditional relays, providing faster, quieter, and longer-lasting performance.
Conclusion:
With the increasing demand for more reliable and efficient switching technology, MOS relays are proving to be the future of electronics. Their fast switching times, low power consumption, and long operational life make them ideal for a wide range of applications—from high-speed data communication systems to advanced industrial automation. As industries continue to innovate and push the boundaries of technology, MOS relays will play a crucial role in ensuring high-performance, precision, and efficiency.
1 note
·
View note
Text
Le transistor MOSFET
Le transistor MOSFET, ou "Metal-Oxide-Semiconductor Field-Effect Transistor", est un type de transistor largement utilisé dans l'électronique moderne. Il joue un rôle crucial dans l'amplification et la commutation des signaux électriques. Grâce à sa capacité à contrôler le courant avec une tension très faible, le transistor MOSFET est devenu un composant essentiel dans les circuits intégrés, les alimentations à découpage, et de nombreux autres dispositifs électroniques.
Le principe de Fonctionnement
Le MOSFET fonctionne selon le principe du champ électrique. Il possède trois électrodes : la source (S), le drain (D) et la grille (G). La grille est séparée de la zone de conduction par une fine couche d'oxyde, ce qui lui confère une très haute impédance. Lorsqu'une tension est appliquée à la grille, elle crée un champ électrique qui modifie la conductivité entre la source et le drain. Cela permet au transistor MOSFET de fonctionner comme un interrupteur ou un amplificateur.
Les différents types de MOSFET
Il existe principalement deux types de MOSFET :
MOSFET à canal N : Dans ce type, le courant circule principalement à travers des électrons. Ils sont généralement utilisés pour des applications nécessitant une vitesse élevée et une faible résistance.
MOSFET à canal P : Dans ce cas, le courant est transporté par des "trous" (lacunes d'électrons). Ils sont souvent utilisés en complément des MOSFET à canal N pour former des circuits complémentaires (CMOS).
Plus de détails : electro-robot.com/electronique/composants/le-transistor-mosfet
Vous chercher à étudier les transistors en électronique : electro-robot.com/electronique/composants/le-transistor
et pour apprendre l’électronique et la robotique : electro-robot.com
0 notes
Text
RF Transistor Market is Booming Worldwide Growth Prospects, Incredible Demand, and Business Strategies by 2032
Allied Market Research, titled, “RF Transistor Market, By type, application and region: Global Opportunity Analysis And Industry Forecast, 2023-2032," the RF transistor market was valued at $2.1 billion in 2022, and is estimated to reach $3.8 billion by 2032, growing at a CAGR of 6.2% from 2023 to 2032.
A RF transistor is designed with precision to handle high-power radio frequency (RF) signals commonly present in devices such as amplifiers, radio transmitters, and television monitors. These transistors employ materials like germanium (Ge) or silicon (Si) that undergo doping with impurities to modify their electrical properties. They are used to amplify or switch electronic signals and are characterized by two types of parameters: DC and functional. RF power amplifiers use solid-state devices, predominantly metal–oxide–semiconductor field-effect transistors (MOSFETs) and Laterally- double diffused MOSFET (LDMOS) transistors, as the standard technology for RF power amplifiers.
These transistors play a pivotal role in numerous communique structures, along with Wi-Fi networks, cell telephones, radios, and televisions. Their layout is tailor-made to fulfill the demands of RF applications, such as high advantage, minimal noise, and effective power handling skills. The capacity to amplify alerts whilst minimizing distortion is important for preserving the integrity of alerts in verbal exchange systems. RF transistors have diverse configurations, encompassing commonplace types such as bipolar junction transistors (BJTs) and field-effect transistors (FETs). Each configuration incorporates its very own set of advantages, enabling designers to pick out the most appropriate kind for precise packages. RF transistors serve as crucial additives in RF amplifiers, oscillators, mixers, and different circuits fundamental for the processing and transmission of alerts.
In addition, RF transistors represent essential additives within the realm of excessive-frequency communication, facilitating the reliable amplification and manipulation of radio frequency alerts across a variety of digital devices and systems. The growing request for advanced communication technologies is a significant factor pushing the expansion of the RF transistor market. The telecommunications sector is experiencing a rising need for RF transistors, propelled by advancements in wireless networks, the evolution of 5G infrastructure, satellite communication, and the growing Internet of Things (IoT). The industry's growth is further fueled by ongoing initiatives to boost data transfer speeds and enhance signal processing capabilities in mobile devices like smartphones and tablets. Additionally, the momentum in the market is amplified by the automotive industry's incorporation of advanced connectivity features and the escalating embrace of smart technologies in vehicles. Defense applications, such as radar and satellite communication systems, also fuel demand for RF transistors in the aerospace and defense sector. The ongoing development of smart cities and the expanding use of IoT in industrial automation further drive the RF transistor market demand.
The RF transistor industry sees broad application across various sectors, fueled by the growing demand for advanced communication technologies. In telecommunications, RF transistors are crucial for wireless networks, 5G infrastructure, satellite communication, and IoT devices. They are essential for amplifying and processing radio frequency signals, ensuring efficient data transfer, and improving signal quality in mobile devices such as smartphones and tablets. In addition, the automotive industry relies on RF transistors for integrating advanced connectivity features, and the aerospace and defense sector utilizes them in radar and satellite communication systems. The ongoing progress of smart cities and the increasing use of IoT in industrial automation further expand the utility of RF transistors, showcasing their versatile role in facilitating seamless and dependable communication across a range of electronic devices and systems. Many challenges can hamper the expansion of the RF transistor market growth.
One notable challenge is the growing intricacy of RF systems and the demand for more sophisticated and expensive RF transistors to meet heightened performance expectations. The ongoing trend toward smaller electronic devices poses another hurdle, necessitating RF transistors to be more compact, energy-efficient, and still capable of delivering superior performance. Stringent regulatory frameworks, encompassing spectrum allocation and licensing complexities, present a barrier that can impact the development and deployment of RF-enabled technologies. In addition, disruptions, and shortages in the global semiconductor supply chain, as witnessed recently, have the potential to impede the production and availability of RF transistors. Furthermore, the dynamic nature of communication standards and technologies, such as the shift to 5G, requires swift adaptation and innovation. This poses a challenge for RF transistor manufacturers to keep pace with these advancements. Addressing these collective challenges demands continuous research, development, and strategic planning within the RF transistor market to overcome obstacles and promote sustained growth.
The RF transistor market analysis is segmented into type, application, and region. By type, the market is analyzed across bipolar RF transistor, MOSFETs, and others. By application, the market is segmented into communication infrastructure, automotive, consumer electronics, industrial, and others. Region-wise, it is analyzed across North America, Europe, Asia-Pacific, and LAMEA.
Competitive analysis and profiles of the major RF transistor market trends players, such as Infineon Technologies AG, NXP semiconductors, Microchip Technology Inc., Analog devices, MACOM, Renesas Electronics, Semiconductor Components Industries, LLC., Skyworks Solutions Inc., Qorvo, In. and Wolfspeed, Inc. are provided in this report. Product launch and acquisition business strategies were adopted by the major market players in 2022.
KEY FINDINGS OF THE STUDY
The RF transistor market share is poised for substantial expansion in the foreseeable future, fueled by the rising demand for wireless communication technologies.
The increasing demand for RF transistors within the communication infrastructure sector is projected to influence the RF transistor market size.
The market exhibits high competitiveness, marked by the active participation of several major players vying for market share. Expectations include an intensification of competition in the coming years with the entry of new players into the market.
The Asia-Pacific region is expected to be a major market for RF transistor market owing to��rapid adoption of 5G technology development inside the country.
0 notes
Text
Finfet vs Mosfet: What are Differences
FinFET and MOSFET, two integral components in semiconductor technology, embody distinct approaches to transistor design. FinFET, with its innovative three-dimensional structure, has emerged as a transformative technology, promising improved efficiency and performance. Meanwhile, MOSFET, with its well-established planar structure, remains a stalwart in electronic applications. This comprehensive exploration delves into the structures, functionalities, advantages, disadvantages, and future trends of FinFET and MOSFET, providing insights into the dynamic landscape of semiconductor devices.
What is Finfet
A FinFET, short for Fin Field-Effect Transistor, represents an innovative type of semiconductor transistor, specifically a complementary metal-oxide-semiconductor (CMOS) transistor. It departs from traditional planar transistor structures by introducing a unique 3D design with a fin-shaped channel. This fin-like structure allows for enhanced control over the flow of current.
In contrast to standard transistors where the gate influences current on only one side, FinFET's gate features a three-dimensional, fork-shaped design, enabling control on both sides of the channel. This architectural advancement improves circuit control, minimizes leakage current, and significantly reduces the gate length of the transistor.
FinFET technology has become integral in various electronic devices, including home computers, laptops, tablets, smartphones, wearables, high-end networks, and automotive applications. Its 3D structure and superior control over current flow make it a pivotal component for achieving improved performance, energy efficiency, and scalability in semiconductor technology.
Finfet Structure
FinFET is a three-dimensional transistor structure characterized by vertically arranged fins. Its basic configuration includes the Fin (fin), Gate (gate), Drain (drain), Source (source), and Substrate. The Fin serves as the channel, the Gate controls the flow of electric current, while the Drain and Source play roles akin to traditional MOSFETs, managing the input and output of current. The Substrate acts as the foundation for the entire structure, aiding in device isolation within the chip. FinFET's distinctive design enhances current control, providing superior performance in semiconductor technology.
How Does Finfet Work?
A FinFET is a type of transistor that operates similarly to a traditional MOS transistor but with a unique 3D structure. The name "FinFET" comes from its fin-shaped design. In a FinFET, the transistor structure is folded along the gate length, allowing the gate to control the conducting channel on both sides of the fin. This feature enhances conduction efficiency.
To visualize a FinFET, imagine folding a square piece of paper to create a tent-like structure, representing the gate and oxide. This folded structure is filled with silicon, and folded source and drain contacts are added on either side, forming the fin. This 3D design improves the transistor's performance, and its representation on paper involves a cross-section in the x-z plane, differing from the planar MOSFET.
In summary, a FinFET's distinctive 3D structure enhances conduction control, making it a more efficient and high-performance transistor.
Advantages and Disadvanatages of Finfet
Advantages of FinFET:
Better Control over the Channel:
FinFETs provide improved control over the channel due to their 3D fin-shaped structure, allowing for more effective regulation of current flow.
Suppressed Short-Channel Effects:
The unique design of FinFETs helps mitigate short-channel effects, enhancing the transistor's performance and reliability.
Lower Static Leakage Current:
FinFETs exhibit reduced static leakage current, contributing to enhanced energy efficiency and lower power consumption.
Faster Switching Speed:
The 3D architecture of FinFETs enables faster switching speeds, improving overall transistor performance.
Higher Drain Current (More Drive-Current per Footprint):
FinFETs offer a higher drain current, allowing for increased drive-current per unit area, which is beneficial for high-performance applications.
Lower Switching Voltage:
FinFETs typically operate at lower switching voltages, reducing power requirements and contributing to energy efficiency.
Low Power Consumption:
The combination of lower static leakage current and efficient switching contributes to overall lower power consumption in FinFET-based devices.
Disadvantages of FinFET:
Difficult to Control Dynamic Vth:
Dynamic threshold voltage (Vth) control can be challenging in FinFETs, posing complexities in certain operational scenarios.
Quantized Device-Width:
FinFETs have a quantized device-width, making it impossible to specify dimensions in fractions of fins. Designers must adhere to multiples of whole fins.
Higher Parasitics Due to 3-D Profile:
The 3D profile of FinFETs introduces higher parasitic capacitances and resistances, potentially impacting overall performance.
Very High Capacitances:
FinFETs can exhibit very high capacitances, affecting their ability to maintain low power consumption in certain applications.
Corner Effect:
The corner effect in FinFETs results in an amplified electric field at the corners compared to the sidewalls, which can be mitigated using specific design techniques such as nitrate layers.
High Fabrication Cost:
The complex 3D structure and additional fabrication steps contribute to higher manufacturing costs for FinFETs compared to traditional transistor technologies.
In conclusion, while FinFETs offer several advantages, including improved control and lower power consumption, they come with challenges such as quantized dimensions and higher parasitics, making careful consideration necessary in their application and design.
What is Mosfet
A Metal-Oxide-Semiconductor Field-Effect Transistor (MOSFET, MOS-FET, or MOS FET) represents a type of field-effect transistor (FET) featuring an insulated gate, where voltage governs the device's conductivity. Primarily employed for signal switching and amplification, its capability to alter conductivity based on applied voltage is instrumental in electronic signal modulation.
In MOSFETs, silicon dioxide constitutes the gate, providing isolation by impeding direct charge flow from the gate to the conducting channel. This insulation is pivotal in the MOSFET's role as a voltage-controlled device.
Notably, MOSFETs have surpassed Bipolar Junction Transistors (BJTs) in prevalence within both digital and analog circuits. The abundance of MOSFETs in digital applications, such as memory chips and microprocessors, underscores their significance. Their versatility extends to complementary metal-oxide-semiconductor (CMOS) logic, where pairs of MOS transistors, fabricated with either p-type or n-type semiconductors, facilitate switching circuits characterized by exceptionally low power consumption.
The dominance of MOSFETs in digital circuits, owing to their scalability, efficiency, and low power requirements, underscores their indispensable role in modern electronics.
Mosfet Structure
MOSFETs, or Metal-Oxide-Semiconductor Field-Effect Transistors, exhibit a planar structure characterized by the integration of metal, oxide, and semiconductors. In the case of a planar n-channel MOSFET, the transistor is created by diffusing heavily doped n-type regions onto a p-substrate body. Atop this planar configuration, a layer of silicon dioxide is cultivated. Metallic terminals are selectively etched onto the insulating layer of silicon dioxide. The metallic terminals that establish contact with the n-type regions beneath assume the roles of drain and source. The gate terminal, positioned on the silicon dioxide layer without contact with the n-type regions, completes the basic structure of the planar MOSFET.
The MOSFET is a four-terminal device with Source (S), Drain (D), Gate (G), and body (B) terminals. Often, the body (B) is connected to the source terminal, reducing the terminals to three. Its operation involves adjusting the width of a channel through which charge carriers (electrons or holes) flow.
Charge carriers enter the channel at the source and exit through the drain. The channel width is regulated by the voltage applied to the Gate electrode, positioned between the source and drain. This Gate is insulated from the channel by an extremely thin layer of metal oxide.
The MOSFET is alternatively referred to as a metal-insulator-semiconductor field-effect transistor (MISFET) or an insulated-gate field-effect transistor (IGFET).
Working Principle of Mosfet
The operational principle of a MOSFET hinges on the behavior of the MOS capacitor, a crucial component of the MOSFET structure. The semiconductor surface beneath the oxide layer, positioned between the source and drain terminals, plays a pivotal role and can transition from p-type to n-type based on applied gate voltages.
Upon the application of a positive gate voltage, holes beneath the oxide layer encounter a repulsive force, prompting their downward movement into the substrate. Simultaneously, the depletion region accumulates bound negative charges linked to acceptor atoms. Electrons migrate towards this region, forming a channel. The positive gate voltage also attracts electrons from the n+ source and drain regions into the channel.
Subsequently, if a voltage is introduced between the drain and source, current can flow freely between these terminals, and the gate voltage governs the behavior of electrons within the channel. Conversely, the application of a negative voltage results in the formation of a hole channel beneath the oxide layer. This dynamic interplay enables the MOSFET to regulate the flow of current based on the applied gate voltage, making it a versatile and controlled semiconductor device.
Advantages and Disadvanatages of Mosfet
Advantages:
Scalability: MOSFETs exhibit exceptional scalability, allowing for downsizing in dimensions. This characteristic facilitates increased component integration on the chip surface, a crucial feature in modern electronic devices.
Low Power Consumption: MOSFETs are known for their low power consumption, enabling the efficient incorporation of numerous components on a chip, contributing to energy-efficient electronic systems.
No Gate Diode: Unlike certain transistors, MOSFETs lack a gate diode. This unique feature allows them to operate seamlessly with both positive and negative gate voltages, enhancing their versatility in circuit design.
Direct Readability: MOSFETs offer direct readability with a very thin active area, streamlining their use in electronic applications where compact design and precision are essential.
High Drain Resistance: With higher drain resistance due to the lower resistance of the channel, MOSFETs contribute to improved signal control and integrity in electronic circuits.
Compact Physical Size: In packaged form, MOSFETs possess a physical size of less than 4 mm^2, allowing for the creation of smaller, space-efficient electronic devices.
Widespread Usage: MOSFETs find extensive applications in VLSI circuits, CMOS digital circuits, microprocessors, and memory devices, underlining their significance in modern electronics.
Enhancement Type for Digital Circuitry: The prevalence of enhancement-type MOSFETs in digital circuitry underscores their role in advancing digital technology.
High-Speed Operation: MOSFETs support high-speed operation, making them suitable for applications where rapid switching is essential, such as in digital signal processing.
High Input Impedance: With high input impedance, MOSFETs offer enhanced signal reception capabilities, making them versatile in various electronic circuits.
Ease of Fabrication: Fabricating MOSFETs is comparatively easier than certain transistor types, contributing to their widespread adoption in electronic manufacturing.
Manufacturing Convenience: MOSFETs are easy to manufacture, further contributing to their popularity in the semiconductor industry.
Disadvantages:
Limited Lifespan: MOSFETs have a finite lifespan, which may pose challenges in applications requiring prolonged device longevity.
Calibration Requirements: In certain applications, MOSFETs may require repeated calibration for accurate dose measurements, adding complexity to their implementation.
Susceptibility to Overload Voltage: MOSFETs are highly susceptible to overload voltage, necessitating special handling during installation to prevent potential damage and ensure reliable operation.
Finfet vs Mosfet: What are Differences
Aspect
MOSFET
FinFET
Structure
Planar structure
Three-dimensional or "fin" structure
Gate Control
Voltage-controlled conductivity
Voltage-controlled gate width
Leakage Current
Relatively higher leakage current
Lower leakage current
Power Efficiency
Relatively lower power efficiency
Improved power efficiency
Speed and Performance
Moderate speed
Faster switching speed
Transistor Capacitance
Higher transistor capacitance
Reduced transistor capacitance
Scalability
Limited scalability in smaller nodes
Better scalability in smaller nodes
Integration
Limited integration capability
Improved integration capability
Manufacturing Process
Relatively simpler manufacturing process
More complex manufacturing process
Cost
Lower manufacturing cost
Higher manufacturing cost
Applications
Wide range of applications
Similar range of applications as MOSFET
Future Trends
Limited potential for future improvements
Further potential for development
In summary, FinFET and MOSFET are two transistor technologies with distinct characteristics and applications. While MOSFET offers well-established performance and scalability, FinFET brings enhanced power efficiency and improved control over leakage current. Both technologies contribute to the continued advancement of the semiconductor industry and enable innovative electronic devices and systems.
Finfet vs Mosfet: Which is Better
The advent of FinFET technology has introduced significant advantages over traditional MOSFETs, primarily attributed to its three-dimensional structure. These desirable characteristics of FinFETs include:
Higher Integration Density: FinFET technology allows for the incorporation of a large number of transistors on a single chip, making it highly suitable for IC fabrication. This scalability advantage surpasses that of MOSFETs within a given footprint area.
Improved Short-Channel Behavior: As chips shrink in size, MOSFETs face challenges related to short-channel effects. The three-dimensional structure of FinFETs, with fins providing better short-channel behavior, addresses this issue effectively.
Elimination of Channel Doping Fluctuations: In planar MOSFETs, channel doping is common to improve short-channel behavior. However, FinFETs, with their wrap-around gate over a thin body, make channel doping optional, eliminating dopant-induced fluctuations.
Reduced Leakage Current and Power Consumption: FinFETs demonstrate reduced leakage current and voltage, contributing to lower power consumption compared to MOSFETs. The gate wrapping around the drain-source channel minimizes leakage current when the gate is not energized.
Enhanced Drive Strength and Current: FinFETs provide flexibility in increasing drive strength by incorporating multiple or longer fins, in contrast to planar MOSFETs, where drive strength depends on channel width.
Fast Switching Times: The higher drive current in FinFETs translates to faster switching times, making them high-speed devices compared to planar MOSFETs.
Ease of Fabrication for Multi-Gate Devices: Fabricating multi-gate devices is more straightforward with FinFET technology, while it poses challenges in planar MOSFETs.
Superior Subthreshold Slope and Voltage Gain: FinFETs exhibit excellent subthreshold slope and higher voltage gain compared to planar MOSFETs, enhancing their overall performance.
In the FinFET vs. MOSFET comparison, understanding the distinctive advantages of FinFETs underscores their appeal in contemporary semiconductor technology. The low leakage current, improved heat dissipation, reduced DIBL, and consistent threshold voltage make FinFETs a compelling choice for applications demanding advanced performance. Manufacturers have demonstrated the scalability of FinFET technology, showcasing its continued relevance in the evolution of semiconductor manufacturing.
Future Trends for FinFET and MOSFET
In the realm of transistor technology, the future holds promising advancements for both FinFET and MOSFET. For FinFET, ongoing research and development efforts focus on refining its three-dimensional structure, optimizing manufacturing processes, and exploring higher levels of transistor integration on a single chip. These trends aim to further enhance FinFET's scalability, efficiency, and cost-effectiveness, solidifying its role in the semiconductor landscape.
Simultaneously, MOSFETs are expected to evolve by persisting in scaling down to smaller process nodes and exploring hybrid integration with emerging technologies. As researchers delve into alternative transistor structures, including nanowire transistors and 2D materials, the future may witness the emergence of new transistor technologies beyond FinFET. Moreover, innovations in design and materials are anticipated to contribute to improved power efficiency and overall performance for both FinFET and MOSFET.
The integration of traditional transistors with emerging technologies, such as neuromorphic computing and quantum devices, could further shape the landscape. Additionally, customization for specific applications, such as AI, IoT, and edge computing, is likely to drive tailored solutions, ensuring that FinFET and MOSFET technologies continue to play pivotal roles in the ever-evolving field of semiconductor technology.
Conclusion
In the ever-evolving realm of semiconductor technology, FinFET and MOSFET stand as pillars, each contributing unique strengths to the field. While MOSFET showcases reliability, scalability, and versatility, FinFET introduces a paradigm shift with its three-dimensional prowess, promising enhanced efficiency and control. As research propels these technologies forward, the future holds promises of improved integration, scalability, and performance. Whether it be the refined structures of FinFET or the enduring legacy of MOSFET, both technologies continue to shape the landscape of modern electronics, paving the way for innovative applications and advancements.
1 note
·
View note
Text
JEE Main 2025 Syllabus: Your Complete Guide.
As the JEE Main 2025 exam approaches, eager engineers must first comprehend the syllabus. The Joint Entrance Examination (JEE) Main is a highly competitive exam administered by the National Testing Agency (NTA) for admission to several engineering institutes in India. A well-defined curriculum allows pupils to carefully arrange their preparation. This is an in-depth look at the JEE Main 2025 syllabus for Physics, Chemistry, and Mathematics.
Physics in JEE Main combines theoretical principles with practical tasks. The syllabus includes a wide range of topics that are essential for comprehending the physical world.
Section A covers theory and measurement, including units, dimensions, error analysis, and physical quantities.
Kinematics refers to one- and two-dimensional motion, vector algebra, and relative velocity.
Newton's Laws of Motion include friction and circular motion.
The work-energy theorem, conservative forces, and power are all related concepts.
Torque, angular momentum, and moment of inertia are all terms that describe rotational motion.
gravity includes the universal law of gravity, gravitational potential, and satellite motion.
Solid-liquid properties include elasticity, viscosity, surface tension, and fluid mechanics.
Thermodynamics: Laws of thermodynamics, heat engines, and Carnot cycle.
Gas kinetic theory includes molecules, degrees of freedom, and the Boltzmann constant.
Oscillations and waves include SHM, damped and forced oscillations, wave motion, and the Doppler effect.
Electrostatics includes Coulomb's law, electric field and potential, and capacitors.
Current electricity includes Ohm's law, Kirchhoff's laws, and electromotive force.
Magnetic effects of current and magnetism include the Biot-Savart law, Ampere's law, and electromagnetic induction
Faraday's laws, inductance, and AC circuits are all examples of electromagnetic induction and alternating current.
Optics includes reflection, refraction, interference, diffraction, and polarization.
The photoelectric effect and de Broglie waves demonstrate the dual nature of matter and radiation.
Atoms and Nuclei include atomic models, radioactivity, and nuclear processes.
Electronic devices include semiconductors, diodes, transistors, and logic gates.
Communication systems include modulation, wave propagation, and satellite communication.
Section B - Practical Skills
Vernier calipers, a screw gauge.
Calculating g with a simple pendulum.
Ohm's law verification and the resistance of a specific wire.
Young's modulus calculated using Searle's approach.
The specific heat capacity of a liquid.
The refractive index of a glass slab.
JEE Main Chemistry is separated into three sections: physical, inorganic, and organic.
Physical chemistry
Some basic concepts in chemistry: Stoichiometry and the mole notion.
The three states of matter are gases, liquids, and solids.
Atomic Structure: Quantum theory and atomic models.
Chemical Bonding and Molecular Structure: VSEPR Theory, Hybridization.
Chemical thermodynamics includes enthalpy, entropy, and Gibbs free energy.
Solutions include concentration terms, Raoult's law, and colligative features.
Equilibrium includes chemical and ionic equilibrium.
Redox processes: Oxidation numbers and balancing redox processes.
Chemical Kinetics: Rate laws and the Arrhenius equation.
Surface Chemistry: Adsorption and Catalysis.
Inorganic chemistry
Elements' classification and periodicity in properties: Periodic table trends.
General Principles and Processes for Metal Isolation: Metallurgy.
Hydrogen: isotopes, preparation, and characteristics.
Groups 1 and 2 of the s-block elements (alkali and alkaline earth metals).
p-Block Elements: Groups of 13 to 18 elements.
D and F-Block components include transition and inner transition elements.
Coordination compounds: nomenclature and bonding.
Environmental Chemistry: Pollution and ozone depletion.
Organic Chemistry: Fundamental Principles and Techniques Nomenclature and isomerism.
Hydrocarbons include alkanes, alkenes, alkynes, and aromatic hydrocarbons.
Haloalkanes and haloarenes: composition, characteristics, and reactions.
Alcohols, phenols, and ethers: definitions, synthesis, and reactions.
Aldehydes, ketones, and carboxylic acids: Synthesis, characteristics, and reactions.
Organic compounds Contains nitrogen: Amines are diazonium salts.
Biomolecules include carbohydrates, proteins, and vitamins.
Polymers: Classification and Properties.
Chemistry in everyday life includes drugs, detergents, and food.
Mathematics
Mathematics in JEE Main necessitates a thorough comprehension of ideas and the ability to apply them to solve problems.
Sets, Relations, and Functions: Set theory; kinds of relations and functions.
Complex numerical operations and quadratic equations.
Matrices and Determinants: Types, Properties, and Applications.
Permutations and combinations: The fundamental premise of counting.
Mathematical induction: principles and applications.
Binomial Theorem: Expansion in generic terms.
Sequences and series are arithmetic and geometric progressions.
Limits, continuity, and distinction.
Integral calculus includes indefinite and definite integrals, as well as area under curves.
Differential Equations: Forming and solving differential equations.
Coordinate Geometry includes straight lines, circles, and conic sections.
Three-dimensional geometry includes directional cosines, planes, and lines.
Vector algebra encompasses vectors, scalars, and vector products.
Statistics and probability include the mean, median, mode
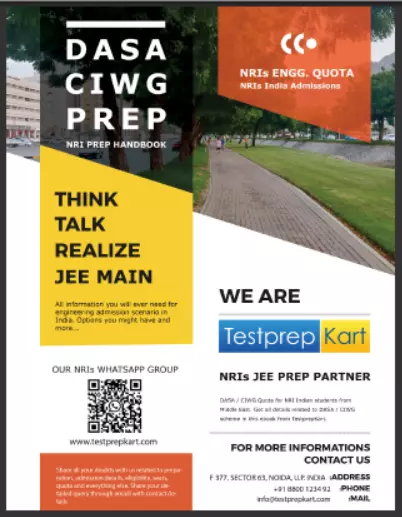
0 notes
Text
Electric Vehicle Power Inverter Market: Strategic Developments
Automotive manufacturers are progressively transitioning their production from conventional engine vehicles to hybrid and electric vehicles. This is attributed to increasingly stringent global emission standards. Concurrently, governments worldwide are implementing incentives to encourage the adoption of electric vehicles and stimulate sales in this sector. Subsequently, the electric vehicle demands are poised to surge the sales of critical components integral to electric vehicles. For instance, electric vehicle power inverters. As per Inkwood Research, the global electric vehicle power inverter market is expected to project a CAGR of 14.36% during 2024-2032 and is set to reach a revenue of $34868.78 million by 2032.
Furthermore, several manufacturers have committed to developments associated with electric vehicles and their components. This blog focuses on strategic product developments by key players regarding electric vehicle power inverters.
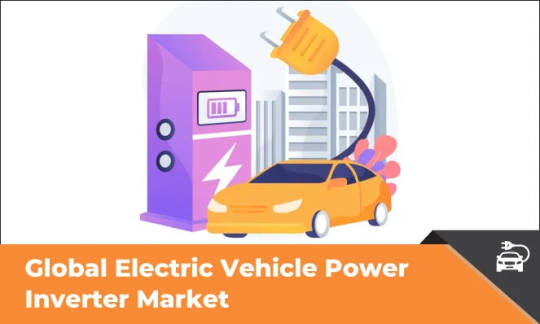
Request a FREE sample of the Electric Vehicle Power Inverter Market-
Global Electric Vehicle Power Inverter Market: Strategic Product Developments Prominent Players
· Tesla’s Offbeat Silicon Carbide Inverter
In 2018, with the introduction of the Model 3, Tesla pioneered the incorporation of SiC metal-oxide-semiconductor field-effect transistors (MOSFETs) from STMicroelectronics into an internally designed inverter. While the overall design boasts numerous innovations, the integration of SiC packages stands out as a primary advancement.
The utilization of SiC MOSFETs presents a breakthrough in inverter design and opens avenues for novel materials. Coping with increased areal power densities involves the use of a Cu lead frame, replacing conventional Al wire bonds. Moreover, the die-attach material has transitioned from conventional solders to Ag-sintered die-attach.
As with any nascent technology, cost has traditionally impeded the widespread adoption of SiC MOSFETs and related materials in power module packages. However, Tesla has effectively addressed this challenge, achieving a noteworthy cost reduction in its inverter within a mere three years.
The outcome is a Tesla inverter and permanent magnet motor combination that stands among, if not at the pinnacle of the market. With an impressive 97% efficiency, it delivers extended range without necessitating a costly increase in battery capacity. All this, while maintaining a comparable cost to the older technologies it is displacing.
· Diamond Foundry strikes Gold with its Latest Innovation
Diamond Foundry Inc has launched an electric car inverter utilizing diamond wafers. With this, the entity has reached a significant milestone in electric vehicle technology. This advanced inverter is six times smaller than the one in Tesla Model 3. Even outperforming in both efficiency and power delivery. The incorporation of diamond wafers is credited for this groundbreaking feat. This further significantly enhances thermal conductivity and electrical insulation – two crucial factors in semiconductor design. The progress in miniaturization represents a notable advancement in electric car efficiency, potentially reshaping power electronics in the industry.
Diamond Foundry’s innovation utilizes the unique properties of diamond wafers to address longstanding thermal challenges in power semiconductors. The company’s inventive approach enables robust voltage isolation while maintaining optimal thermal performance. Additionally, this creative use of diamond wafers overcomes past limitations in a cost-effective manner, paving the way for new designs in power electronics.
Get CUSTOMIZED market insights delivered right to your inbox!
· Hillcrest’s Pioneering Promise
The US and Canadian governments aim for net-zero emissions by 2050, necessitating substantial electrification. However, auto manufacturers face mineral shortages, especially lithium, crucial for EV batteries. With the surge in electric vehicle (EV) sales, battery demand may double by 2050.
Hillcrest Energy Technologies Ltd addresses this by introducing a groundbreaking ‘soft switching‘ inverter. Unlike conventional ‘hard switching’ inverters in EVs, Hillcrest’s inverter utilizes Zero Voltage Switching (ZVS) technology, eliminating trade-offs. This results in enhanced efficiency, performance, and reliability, allowing manufacturers to produce batteries up to 15% smaller while maintaining performance and reducing the need for lithium and other metals.
Hillcrest’s ZVS inverter marks a significant breakthrough, addressing challenges present in the industry since the 1990s.
Stay up-to-date with the Latest Global Electric Vehicle Power Inverter Market Trends- https://inkwoodresearch.com/global-electric-vehicle-power-inverter-market/
Electric Vehicle Power Inverter Market: Reassuring Inputs
The electric vehicle market’s rapid growth is driven by the imperative for decarbonization, aligning with sustainability goals. The electric vehicle power inverter, crucial in the drive system, converts DC battery voltage to AC, propelling the traction motor for vehicle torque.
Notably, the on-board charging system, serving as a rectifier for AC-to-DC conversion, significantly influences charging times. This system interfaces with Level 1 or Level 2 chargers, enhancing the AC-to-DC charging service when plugged into the vehicle.
Furthermore, combining the on-board charger and inverter in some instances leverages high-voltage architecture efficiencies. This integrated approach, coupled with effective thermal management, enhances power output, expediting the charging process. As a result, strategic product developments and integrations are expected to bode well for the global electric vehicle power inverter market.
FAQ
What role does the power inverter play in an electric vehicle?
The electric vehicle power inverter is a crucial component responsible for converting direct current (DC) from the vehicle’s battery into alternating current (AC) for the electric motor. This conversion is vital for powering the motor and ensuring the efficient operation of the electric vehicle.
How do advancements in electric vehicle power inverters contribute to overall vehicle performance?
Advancements in electric vehicle power inverters often result in improved energy efficiency, faster response times, and better thermal management. The evolution of power inverter technology contributes to extended battery life, increased driving range, and optimized electric drivetrain performance, thereby influencing the overall efficiency and capabilities of electric vehicles.
0 notes