#Hot Filament Chemical Vapor Deposition
Explore tagged Tumblr posts
Text

Advanced HFCVD technology for high-quality thin-film applications
Hot filament chemical vapor deposition (HFCVD) offers a high-efficiency method for producing durable, uniform thin films, essential for various applications in semiconductors, electronics, and optics. This technology utilizes a heated filament to create precise coatings that enhance material properties and ensure consistent surface quality. HFCVD is widely applied in industries that demand reliable deposition methods, supporting innovation with effective, precision-driven thin-film solutions.
0 notes
Text
The Allure of CVD Diamonds: The Future of Gemstone Technology
In the dazzling world of gemstones, CVD diamonds are emerging as a game-changer. These lab-grown diamonds, created through the Chemical Vapor Deposition (CVD) process, offer an ethical and innovative alternative to traditionally mined diamonds. This article explores what makes CVD diamonds special, their manufacturing process, and the benefits they bring to both consumers and the environment.
What are CVD Diamonds?
CVD diamonds are synthetic diamonds produced using the Chemical Vapor Deposition method. Unlike natural diamonds, which take millions of years to form deep within the Earth under high pressure and temperature, CVD diamonds are created in a laboratory over weeks. This process involves breaking down carbon-rich gases and depositing the carbon atoms onto a substrate, building up a diamond layer by layer.
The CVD Diamond Manufacturing Process
Substrate Preparation
The first step in the CVD diamond manufacturing process is preparing the substrate. This is often a small diamond seed or silicon wafer, meticulously cleaned to ensure it is free from contaminants. The substrate serves as the base upon which the diamond will grow.
Gas Introduction and Plasma Activation
The clean substrate is placed in a vacuum chamber, and gases like methane and hydrogen are introduced. The chamber is heated to high temperatures, typically between 800 to 1200 degrees Celsius. This extreme heat causes the gases to break down into reactive carbon and hydrogen atoms. Plasma, created using microwaves or a hot filament, activates these gases, allowing carbon atoms to bond to the substrate and begin forming a diamond crystal.
Diamond Growth
Diamond growth occurs layer by layer as carbon atoms adhere to the substrate. This growth phase can last from several hours to several days, depending on the desired size and quality of the diamond. Throughout this period, the chamber's conditions—such as temperature, pressure, and gas composition—are carefully controlled to ensure optimal growth.
Post-Growth Treatment
After the diamond reaches the desired size, it undergoes post-growth treatments to enhance its properties. These treatments can include annealing, which involves heating the diamond to remove internal stresses and improve its clarity. Additionally, the diamond may be polished and cut to prepare it for use in various applications, from fine jewelry to industrial tools.
Advantages of CVD Diamond Manufacture
Ethical and Environmental Benefits
One of the most significant advantages of CVD diamonds is their ethical and environmental impact. Traditional diamond mining often involves significant environmental degradation and human rights abuses. In contrast, CVD diamonds are grown in a controlled laboratory setting, eliminating the need for destructive mining practices and ensuring ethical labor conditions.
Superior Quality and Purity
CVD diamonds are known for their exceptional quality and purity. The controlled manufacturing process results in diamonds with fewer impurities and defects compared to natural diamonds. This makes CVD diamonds highly desirable for both industrial applications and fine jewelry.
Customization
The ability to customize CVD diamonds is another notable advantage. Manufacturers can adjust the growth conditions to create diamonds with specific colors, shapes, and sizes. This level of customization is particularly appealing to the jewelry industry, where unique and personalized designs are highly valued.
Applications of CVD Diamonds
Jewelry
CVD diamonds have made a significant impact on the jewelry industry. Their high quality, ethical production, and ability to be customized make them a popular choice for engagement rings, earrings, necklaces, and other fine jewelry pieces. Consumers are increasingly drawn to CVD diamonds for their brilliance and ethical appeal.
Industrial Uses
Beyond jewelry, CVD diamonds have a wide range of industrial applications. Their hardness and thermal conductivity make them ideal for cutting tools, heat sinks, and electronic devices. CVD diamonds are utilized in various industries, demonstrating their versatility and superior performance.
The Future of CVD Diamonds
Technological Advancements
The future of CVD diamonds looks promising, with ongoing technological advancements paving the way for even greater innovations. Researchers are continually exploring new methods to enhance the quality and efficiency of the CVD process, promising exciting developments in the years to come.
Market Growth
As awareness of the benefits of CVD Diamond Supplier grows, so too does the market for these remarkable gemstones. Consumers are increasingly seeking out ethical and sustainable options, driving demand for CVD diamonds. Companies specializing in CVD diamond production are well-positioned to capitalize on this trend, continuing to expand their market presence and influence.
Conclusion
CVD diamonds represent a revolutionary approach to gemstone production, offering a sustainable and ethical alternative to traditional mining. With their superior quality, purity, and customization options, CVD Diamonds are poised to become the new standard in the gemstone industry. As technological advancements continue to enhance the CVD process, the future of these lab-grown diamonds looks incredibly bright with Harsha Diamond.
0 notes
Link
0 notes
Photo

Researchers design novel antibiofouling biomimetic diamond film
Biofouling is a worldwide problem that leads to severe deterioration after a substrate comes into contact with seawater. Traditional polymers and other antifouling coatings suffer from poor mechanical and chemical stability, which diminishes the antibacterial and antibiofouling performance upon progression of usage time.
In a study published in ACS Applied Materials & Interfaces, researchers report on the development of a biomimetic diamond film that simultaneously achieved superhydrophobicity, self-cleaning, antibacterial efficacy, antibiofouling, mechanical robustness and chemical stability. This study was conducted by Prof. TANG Yongbing and his collaborators from the Shenzhen Institutes of Advanced Technology (SIAT) of the Chinese Academy of Sciences.
Inspired by the micro- and nano-structures on the taro and lotus leaves, the researchers creatively designed the hierarchically structured diamond film. They synthesized it via a novel bottom-up strategy based on hot-filament chemical vapor deposition (HFCVD) and two-step self-assembly seeding processes.
Read more.
#Materials Science#Science#Biofouling#Biomimicry#Diamonds#Carbon#Coatings#Superhydrophobic#Self cleaning materials#Structures
18 notes
·
View notes
Text
Diamonds are forever – whether made in a lab or mined from the earth

Are you in the market for some sparkle? clearviewstock/Shutterstock.com
Joshua Wilhide, University of Maryland, Baltimore County and William LaCourse, University of Maryland, Baltimore County
It's diamond season. Almost 40 percent of American engagements happen between Thanksgiving and Valentine's Day, with Christmas the most popular day to pop the question – and hand over a sparkly piece of ice. Jewelry stores do at least double their usual monthly sales in December.
Since at least the late 1800s, with the discovery of huge diamond mines in South Africa, people have treasured these dazzling gems. The beauty and splendor of diamonds goes well beyond the surface. Like a diamond hunter digging in an underground mine, one must look deeper to their atomic characteristics to understand what sets these stones apart – and what makes them valuable not just for romantics but also for scientists.
On the atomic level

A literal diamond in the rough, before it's been removed from the matrix within which it formed. USGS, CC BY
When mined from the earth, diamonds look like cloudy rocks before they're cut and polished. Their chemical nature and structure were unknown for centuries. It was Isaac Newton's experiments in the 1600s that first suggested diamonds are made up of the fourth-most abundant element, carbon.
People doubted Newton's discovery, which is understandable considering how different diamonds look from other common forms of carbon, like the graphite in pencils or the ash left over in a wood-burning fireplace. But in 1797, English scientist Smithson Tennant confirmed the composition of diamonds.

Diamond and graphite are both made of carbon atoms, but organized in different structures. Materialscientist/Wikimedia Commons, CC BY-SA
It turns out that carbon takes two common forms that have crystalline structures on the atomic level. Graphite is a repeating two-dimensional, honeycomb-like shape, with layers stacking on top of each other. Alternatively, carbon can form a repeating three-dimensional shape, a tetrahedron – and that's your diamond.
Where do they come from?
There are two sources of the precious gemstone: natural mining or synthesis within a laboratory.
Natural diamonds are formed under intense pressure and heat in the Earth's crust over millions of years. Natural deposits have been found all over the world, from Northern Canada to Western Australia, even underwater in Namibia.
Mines were the only source of the gemstone until 1955, when General Electric produced the first synthetic diamond using what's called the high-pressure, high-temperature process. This process works by applying hundreds of thousands pounds of pressure to graphite at 2,700 degrees Fahrenheit to force the carbon into the correct crystalline structure. It's sort of like an artificial version of the extreme conditions that produce diamonds deep within the earth.
In the 1970s, labs started to use the chemical vapor deposition method to grow diamonds at lower pressures. At the time, the HPHT technique couldn't produce a gem-quality stone. This improved method converts a hydrocarbon gas mixture by breaking it down to its components, carbon and hydrogen molecules, with an intense heated filament or plasma and deposits it onto a substrate, ultimately forming a solid diamond. Originally, this process had a very slow growth rate, but it's now optimized to grow quality diamonds within days.
Together these techniques are largely responsible for human-made diamonds – upwards of 4 billion carats worldwide annually.
There's a common misconception that a natural diamond must be inherently different than a synthetic diamond. To the contrary, they are chemically identical and share the same physical properties. Even the most sophisticated techniques can not detect a difference between a flawless mined diamond and a flawless human-made diamond – both are “real” diamonds. However, truly flawless diamonds of either type are extremely scarce.
Assessing a diamond
No matter its origin, a diamond can be assessed by the “four Cs” of cut, color, clarity and carat weight. Specialized laboratories grade each category, as created by the Gemological Institute of America.

Diamond cutters choose the shape of the finished stone. SPbPhoto/Shutterstock.com
The cut of a diamond is defined in two ways. There's “the general shape of the cut stone,” with shapes including round brilliant (most common), oval, emerald, pear, princess, trilliant, triangle, heart and radiant. And there's “the degree of perfection achieved by the cutting and polishing process” as rated on a scale ranging from excellent to poor. The type and quality of the cut ultimately determines the way light reflects in the stone, contributing to its “brilliance.”
The color of a diamond is graded on a scale from “D,” being perfectly colorless, to “Z” having the most color. Originally, the color of the stone was a huge hint about how it was formed because until 2007 about 90 percent of the high-pressure, high-temperature synthetic stones were yellow orange or yellow. Almost no stones from that process were colorless, so a colorless stone was almost certainly natural. But the HPHT growing process has greatly improved and as of 2016, 43 percent of synthetic diamonds were colorless.
Diamond clarity indicates the presence of inclusions, or tiny imperfections, in the stone. Inclusions make every diamond unique and provide strong clues to whether a diamond is natural or synthetic. The HPHT process uses metal flux, or a hot metal liquid, which acts as a solvent to dissolve the carbon source, graphite, to be rearranged and grown into a diamond. Diamonds grown this way can have inclusions of metals. The resulting stones may be magnetic – if a diamond reacts with a magnet, it is certainly synthetic. Additionally, most synthetic diamonds receive high clarity grades, while natural diamonds contain larger inclusions.
Many consumers focus on carat weight – that is, diamond size. The stone is weighed on a scale where one carat is 200 milligrams (0.007 ounces). Diamonds larger than four carats are almost guaranteed to be natural because that's the limit for the size of the diamonds that the synthetic processes can grow.
Although the “four Cs” of diamonds ultimately define retail value, sentimental value can be even greater. Buyers must decide if a natural or synthetic stone fits the bill for them, based on factors that might include the ecological and ethical ramifications of diamond mining as well as the lower price tag for synthetic rocks.
Diamonds found beyond your ring finger
Although diamonds are well known for their place in the jewelry industry, they play other valuable roles, too.
Their physical properties, especially hardness, are ideal for abrasive applications. Small diamonds can be found coating cutting wheels, drill bits and grinding wheels, which are used for cutting concrete or brickwork.
Diamonds also have certain optical properties that make them suitable for various spectroscopy techniques, or measurements involving the electromagnetic spectrum. Scientific researchers use these tests to help identify the composition of materials they're investigating.

A diamond needle is what's in contact with the grooves on a record. Michelle Hawkins-Thiel/Flickr, CC BY
A previously common place for diamonds was on record players, where to this day the needle that touches the record can be a very small diamond sliver.
Whether one appreciates the aesthetic or scientific characteristics of the gem more, diamonds can dazzle.
Joshua Wilhide, Manager of the Molecular Characterization and Analysis Complex, University of Maryland, Baltimore County and William LaCourse, Professor of Chemistry and Dean of the College of Natural and Mathematical Sciences, University of Maryland, Baltimore County
This article is republished from The Conversation under a Creative Commons license. Read the original article.
0 notes
Text
3D Printing in Ceramics
3D printing technology, also known as augmented material manufacturing, has the advantages of simple operation, rapid molding speed and high precision. With the continuous development of 3D printing technology, it has been gradually applied to various fields of the manufacturing industry. Compared with the traditional preparation process, 3D printing ceramic materials and advanced sintering technology can significantly reduce the processing cost, shorten the production cycle and save raw materials. The development potential of 3D printing ceramic technology is huge, which will promote the development of aerospace, medicine and industry. It is widely applied in other fields.
Overview of 3D printing ceramic technology
At present, 3D printing ceramic technology mainly includes inkjet printing technology, melting deposition molding technology, laser curing molding technology, layered solid manufacturing technology and laser selective sintering technology. These technologies can be classified according to different standards. Among them, the methods based on laser forming are light curing forming technology, layered solid manufacturing technology and laser selective sintering technology, and the other two are non-laser forming methods. Melting deposition molding and laser selective sintering technology are needed to set up supporting structures, while the other three do not need supporting structures. Finally, according to the process can be divided into direct molding method and layer-by-layer bonding method, inkjet printing technology is to mix ceramic powder and binder to prepare ceramic ink, through 3D printing direct molding, belongs to direct molding method, the other four technologies belong to layer-by-layer bonding method.
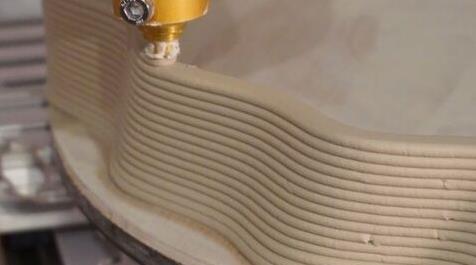
1. Inkjet printing technology
The working principle of 3D inkjet printing ceramic technology is that the ceramic ink in the capillary tube at the bottom of the nozzle is rapidly vaporized by heating the nozzle and bubbles are formed and expanded rapidly in a very short time. As the bubbles expand to a critical value to overcome the surface tension of the ink, the ink sprays from the top of the nozzle capillary. Ceramic ink draws patterns according to the pre-modeled data of the computer, and realizes the 3D printing process by stacking them. When the heating stops, the ink cools, the bubbles begin to condense and contract, the ceramic ink retracts, and the ceramic ink stops being supplied.
The advantages of 3D inkjet printing ceramics technology are: users design personalized ceramics according to their needs, the cost is greatly reduced, to a large extent, saving manpower and material resources, while the technology does not need the assistance of laser technology, has been widely developed and applied in daily life.
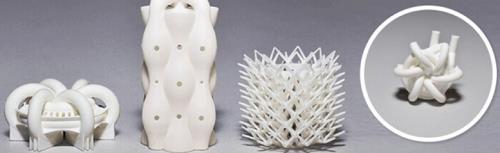
2. Melt deposition molding technology
Melting deposition molding technology is composed of three components: feeding roll, guide sleeve and sprinkler head. Its process engineering is that hot-melting filamentous material passes through feeding roll, enters guide sleeve with the cooperation of driven and active roll, and uses the low friction property of guide sleeve to make filamentous material enter the sprinkler accurately and continuously. The material is heated and melted in the sprinkler head, and 3D printing is done according to the original shape required.
The advantages of melt deposition molding technology are that it does not need the assistance of laser technology, the cost is lower, and the later maintenance is more convenient. However, the technology needs to set up supporting structure to ensure that the ceramic parts will not collapse in the printing process.
At present, there are two kinds of supporting materials: one is peeling supporting materials, which need manual peeling in the later treatment, and the other is water-soluble supporting materials, which can be removed conveniently and quickly by physical or chemical methods in the later treatment. Therefore, the latter is widely used in the market as a supporting material, to a certain extent, reducing the complexity of the post-treatment process.
3, laser curing molding technology
The basic principle of laser curing technology is to focus the ceramic-photosensitive resin mixture liquid in the working tank through the ultraviolet laser beam, according to the designed cross-section of the original layer, and curing point by point, from point to line, from line to surface. After curing the surface in the X-Y direction, the three-dimensional printing ceramic material is finished by the movement of the elevator in the z-axis direction.
The advantages of laser curing technology are: it is suitable for making parts with complex structure and high accuracy. It can be operated online, and remote control is conducive to full automation of production. In addition, the technology does not need to be sintered, and does not need to add sintering aids, so it can be completed at lower temperatures and pressures.
The shortcomings are: the working environment conditions are demanding, low efficiency, need to set up support structure, process is more complex.
4. Layered solid manufacturing technology
Laminated solid manufacturing technology is a thin sheet material superposition process. The process is to cut thin film materials directly by laser, move the lifting table, cut a new layer of thin film materials superimposed on the previous layer of materials, bond forming under the action of hot-bonded components, to achieve the transformation from layer to solid. In 3D ceramic printing, ceramic sheet materials for layered solid manufacturing can be prepared by tape casting.
The advantage of layered solid manufacturing technology is that the molding speed is fast and it is suitable for manufacturing laminated complex structural parts. The disadvantage is that the technology is not suitable for printing complex and hollow parts, there is a more obvious step effect between the layers, and the final product boundary needs to be polished and polished. 5. Laser selective sintering technology
Laser selective sintering technology is mainly realized by the combination of three structural components: roller, laser and worktable. The concrete principle is that the powder is laid on the worktable by the roller, the powder is scanned by the laser beam controlled by the computer, and the binder in the powder is melted by the laser scanning to form the layered structure. After the scanning, the worktable drops, the rollers are coated with a new layer of powder, and the rollers are re-scanned by laser. The worktable is bonded to the solidified sheet ceramics of the previous layer, and the finished product is printed after repeated operation.
Laser selective sintering technology has the advantage of being able to process a variety of materials, including metals, ceramics, coated sand and so on. In the process of laser sintering, there is no need to set up support structure. The final product has good precision and high strength. Compared with the previous several technologies, it has obvious advantages and is widely used.

Application of 3D printing ceramic materials technology
1. Alumina ceramics
The traditional process to prepare alumina ceramics is cumbersome and time-consuming. Compared with the traditional process, 3D printing ceramics have the advantages of a shorter production cycle, lower cost, convenient processing and strong operability. Therefore, using 3D printing technology to prepare alumina ceramics will become a new revolutionary development, further expanding the sales market of alumina ceramics, and will be widely used in construction, aerospace and electronic consumer goods.
The researchers first used the spray granulation technology to prepare alumina powder with a particle size controlled at 10-150 m, and then printed alumina ceramic with good mechanical properties by laser selective sintering technology.
2, tricalcium phosphate ceramics
The chemical composition of tricalcium phosphate is very similar to that of bone. It has the advantages of non-variability and good biocompatibility, and is widely used in the medical field. At present, 3D printing technology of tricalcium phosphate ceramics has been systematically studied abroad. The main technological process is to prepare high-quality tricalcium phosphate ceramics by mixing 100g tricalcium phosphate powder with ethanol and milling for 6h. The slurry is dried twice and then the green body is formed by inkjet deposition and printing technology.
3, porous silicon nitride ceramics
In the field of 3D printing porous silicon nitride ceramics, experts used high-purity silicon powder with particle size of 7.2 micron as raw material and dextrin as binder to prepare silicon nitride powder with particle size of less than 200 micron by granulation process. Porous silicon nitride ceramics were synthesized by 3D printing and step-heating sintering under nitrogen protection with purity greater than 99.999%.
0 notes
Text
Global Nanocrystalline Foils Market to Register Stable Expansion During 2018-2028
Global Nanocrystalline Foils Market Introduction
Technological advancements has led to evolution in the field of nanotechnology. The fine crystalline structures can be drawn into foils and other shapes to achieve superior properties such as mechanical strength, corrosion and wear resistance, protection from magnetic interference, higher energy and noise absorption and reduced weight as compared to conventionally used materials. The nanocrystalline foils are fabricated via electro deposition techniques. The strength of the material increase with decreasing the grain size. The nanocrystalline foils are in high temperature conditions by the process of hot filament chemical vapor deposition. The nanocrystalline foils can protect the surface of soft and less wear resistant materials and are also being used in machinery. These are being used for a number of applications demanding high resistance to external environmental conditions. The nanocrystalline foils have higher physical and mechanical properties as compared to microcrystalline materials. Significant investments are being made for the research and development in the field of nanotechnology. The nanocrystalline foils have the properties such as zero magnetostriction unlike ferrous material thus they can retain their original shape even during high magnetization. Thus they are being widely preferred for use in electronics and energy industry.
Global Nanocrystalline Foils Market Dynamics
Nanocrystalline Foils Market Drivers
The nanocrystalline foils are increasing being used for applications where the conventionally used materials where the conventionally used materials were restricted due to particle size, complexity and other parameters. The use of nanocrystalline foils has caused significant reduction in weight and has improved process efficiency. The global nanocrystalline foils market is driven by rising demand for from the high frequency power transformers. The nanocrystalline foils are suitable for noise suppression and electromagnetic shielding due to the high permeability properties. Nanocrystalline foils are also being used across a wide range of applications such as aerospace, energy, automotive and electronics owing to the properties such as selective permeability, high saturation and can be molded into small sized cores.
Request For Report Sample@ https://www.persistencemarketresearch.com/samples/24397
Nanocrystalline Foils Market Restraints
One of the major factor restraining the demand for nanocrystalline foils in the global market is the high cost associated with the production of such precision materials.
Another challenge faced by the nanocrystalline foils market is the lack of technological knowhow across many of the developing countries for the application of nanocrystalline foils.
Nanocrystalline Foils Market Trends
The manufacturers of nanocrystalline foils are entering into long term contracts with end users such as sensors, transducers, and other electronic devices manufacturers which requires coating and material highly resistant to magnetic interference.
Significant investments for the research and development for the technological advancements and the development of cost efficient methods of coating of components through nanocrystalline foils is being done and is projected to expand significantly over the forecast period. Manufacturers are focusing on the development of nanocrystalline foils made from alloys and other composite materials.
Global Nanocrystalline Foils Market Segmentation
The global Nanocrystalline Foils market can be segmented on the basis of thickness, application, form and region
On the basis of form, the global Nanocrystalline foils market can be segmented as:
Sheet
Roll
On the basis of thickness, the global
15-50 Micron
50-150 Micron
150-250 Micron
Request For Report Table of Content (TOC): https://www.persistencemarketresearch.com/toc/24397
On the basis of end use industry, the global nanocrystalline foils market can be segmented as:
Electronics
Medical
Energy
Automotive
Aerospace
Research and instrumentation
On the basis of material type, the global nanocrystalline foils can be segmented as:
Nickel
Diamond
Copper
Polymeric
Others
Global Nanocrystalline Foils Market: Regional Outlook
The global nanocrystalline foils market is anticipated to be dominated by North America and Europe. The growing investments for the research and development and use of such nano engineered products for use across number of applications including electronics, energy, aerospace and others is projected to boost the market demand. Other regions such as Asia Pacific is projected to witness moderate growth for nanocrystalline foils. China is projected to be relatively high growth country in the Asia Pacific market. Regions such as Middle East and Africa and Latin America are projected to witness sluggish growth in demand for nanocrystalline foils. Japan is also projected to witness significant rise in demand for nanocrystalline foils over the forecast period.
Global Nanocrystalline Foils Market Participants
Some of the market participants identified across the value chain of Nanocrystalline foils market are:
Metglas, Inc.
Integran Technologies
Hitachi Metals, Ltd.
American Elements
Nanostructured & Amorphous Materials Inc.
Hill Technical Sales Corp.
Anhui Lizhi Magnetic Material Co., Ltd.
Shanghai Xianyu Electronics Co., Ltd
Know More About Report@ https://www.persistencemarketresearch.com/market-research/nanocrystalline-foils-market.asp
0 notes
Text
Crystalline tantalum carbide and ditungsten carbide formation via hot wire chemical vapor deposition using the precursor of 1-methylsilacyclobutane
Publication date: 15 October 2017 Source:Surface and Coatings Technology, Volume 326, Part A Author(s): Yujun Shi, Ismail Badran, Suresh Mulmi An efficient method for the preparation of crystalline tantalum carbide (TaC) was reported using a new methyl-substituted precursor, 1-methylsilacyclobutane (MSCB), in the hot-wire chemical vapor deposition process with a tantalum filament. The ability of MSCB to produce methyl radicals on heated Ta filament allows for the formation of crystalline TaC (cubic Fm-3m) in a wide temperature range of 1600–2200°C. This corroborates the key role of the radical species formed via the precursor's decomposition on the hot metal wire in the type of metal alloys formed on the wire surface. The growth rate of TaC achieved at 0.12μm/min and 0.35μm/min, respectively, for 1600°C and 2200°C is much higher than the value obtained from using CH4/H2 mixtures as the C-containing precursor. Crystalline ditungsten carbide (W2C) was formed by treating the tungsten filament with MSCB at the relatively high temperatures of 2200–2400°C, with a deposition rate of 2.1μm/min at 2400°C. The method reported in this work is a useful method to produce crystalline TaC or W2C without the use of the corrosive metal halide precursors.
Graphical abstract
Read more from Journal of Safety Research http://ift.tt/2tJyiLL
0 notes
Text
Innovative Hot Filament Chemical Vapor Deposition (HFCVD) System
Explore Blue Wave Semiconductors' innovative Hot Filament Chemical Vapor Deposition (HFCVD) system for advanced thin film synthesis. Trust our cutting-edge technology to elevate your research and development projects with reliable and precise HFCVD solutions.
0 notes
Text

Innovative Hot Filament Chemical Vapor Deposition (HFCVD) Technology
Explore the innovative Hot Filament Chemical Vapor Deposition (HFCVD) technology offered by Blue Wave Semiconductors. Discover advanced solutions for thin film synthesis, including diamond, graphene, and more. Trust our expertise to elevate your research and development with reliable and cutting-edge HFCVD technology.
0 notes
Link
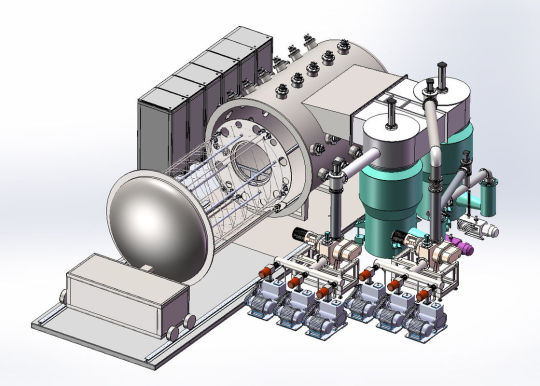
0 notes
Link

0 notes
Link
We offer best Hot Filament Chemical Vapor Deposition (HFCVD) System, Nanodiamond Coating machine, CVD diamond for Electronics at Blue Wave Semiconductor. For more info feel free to contact us at 1 (301) 7068833
0 notes
Link

We offer best Hot Filament Chemical Vapor Deposition (HFCVD) System, Nanodiamond Coating machine, CVD diamond for Electronics at Blue Wave Semiconductor. For more info feel free to contact us at 1 (301) 7068833
0 notes
Link
Chemical Vapor Deposition (CVD) is basically the process of coating a surface with a polymer material. It is used to obtain the desired properties such as hardness, luster or durability. Now CVD has replaced many conventional techniques of coatings as it provides significant advantages over other deposition techniques.
0 notes