#Dissolved Oxygen Meter Parameters Concentration
Explore tagged Tumblr posts
Text
Labnic Dissolved Oxygen Meter is a desktop instrument that measures dissolved oxygen in mg/L, ppm, or % saturation with a water-resistant membrane keypad and a simultaneous temperature display. The Dissolved Oxygen Meter measures parameters including concentration, saturation, and temperature. The Dissolved Oxygen Meter features a wireless Bluetooth connection.
#Dissolved Oxygen Meter Parameters Concentration#Saturation#Temperature#dissolved oxygen electrode#portable dissolved oxygen meter
0 notes
Text
Benchtop Meters: Definition, Function, How They Work and Maintenance
Benchtop meters are essential analytical instruments used in laboratories, research facilities, and industrial settings. These devices play a crucial role in measuring various parameters, including pH, conductivity, ion concentration, and dissolved oxygen. In this article, we’ll explore the basics of benchtop meters, their functions, working principles, and maintenance requirements. What Is…
View On WordPress
0 notes
Text
Bench Top pH Meter
Bench Top pH Meter offers high accuracy, premium performance and ultimate flexibility to meet challenging applications with ease. The device is capable of monitoring pH, mV, relative mV and temperature parameters when an exact probe is attached. Calibrations can be performed up to 5 points and due alarm prompts user to calibrate the meter often. Automatic temperature compensation ensures accurate readings with Bench Top pH Meter has an expanded memory storage up to 1000 data sets.
Benchtop pH meters represent crucial analytical tools utilized in laboratory settings. These devices serve the purpose of precisely determining pH values, alongside other vital parameters like conductivity, ion concentration, ORP (oxidation-reduction potential), and dissolved oxygen levels. Their user-friendly nature streamlines the process of recording and documenting measurement results, promoting efficiency and accuracy in scientific tasks.
0 notes
Text
AirX tube Latest technology in aeration hoses now in India
Vannamei shrimp farms need a very efficient aeration system. The bubbles of a diffused aeration system need to be very small. Especially for large farms – more than 2 acres in size, the aeration required is quite intense. Farmers usually provide aeration only on the periphery using paddle wheel aerators or diffused aeration using blowers and aeration tube. But for large farms of 2 acres and more, aeration needs to be much more uniform and there cannot be any dark areas where DO is low, so providing aeration only in the corners with a paddle wheel aeration system or with a regular aeration is not sufficient.
Such issues are faced in cage farms also, where the distance of the floating service area is very far from the actual cages and fish. Cage farming in India is a relatively nascent industry. Due to the natural flow of water in reservoir and ocean, most water parameters are balanced naturally. There is no sludge or waste to worry about and most of the time the dissolved oxygen, ammonia, pH etc are within the limits because natural flow of water and the eco system ensures they are suitable for marine life.
However there are still times when the dissolved oxygen levels within the cage go very low. And at such times a suitable aeration system is needed. In cage farms submersible or paddle wheel aerators cannot be used. And diffused aeration with aeration tubes and blower is not efficient since the depth of cages is around 10 feet or 3 meters. Hence low pressure fine bubble diffusers along with blowers are needed in such situations.
Identifying the aeration needs of farmers, AirOxi is proud to offer the AirX tube. It is a special double walled EPDM tube which has very fine bubbles. The special 2 perforated wall structure ensures that that bubbles and pressure are proper throughout the length of the tube.
Any standard aeration tube can be used upto a maximum of 3 meter length because the pressure drop is quite high. But with the AirX tubes, the pressure drop is so low that it can be easily installed upto 20 meter length and still get uniform fine bubbles all along the length of the tube.
The material of construction EPDM is inert and this tube can be used in salt water and fresh water as well as RAS (recirculating Aquaculture System).
The tubes have very fine bubble that release at very low pressure so this product is ideal for grow out shrimp farms, RAS and transportation. However the water movement provided is very low so they are not very suitable for nurseries or biofloc.
Since the bubbles are very fine, the SOTR (Standard Oxygen Transfer Rate) is quite high. This makes them the ideal diffuser to use with oxygen cylinder and oxygen concentrators / generators.
The technical details for AirX tube are given below.
AirX tube technical details
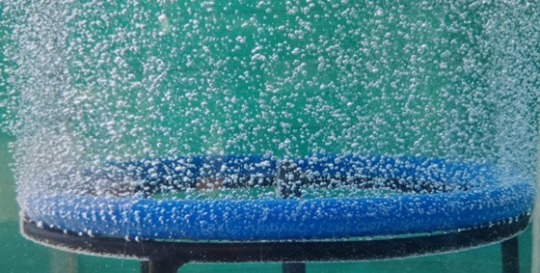
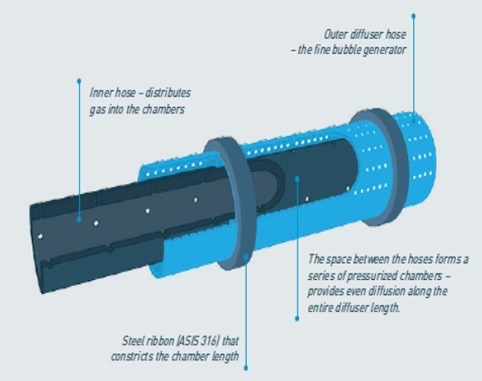
Material – EPDM double walled tube. Inert and safe to use with most liquids
Dimensions – Inside diameter – 12 mm. Outside Diameter – 20 mm.
Compatible fittings - Fittings of ½”/ 16 mm
Standard roll length – 100 mtr
Usage - Compatible for wide range of industrial, commercial, aquaculture in fresh, brackish or salt water, as well as effluent and sewage applications
Air Flow – Optimum – 5 LPM / 0.3 m3/hr. Maximum – 15 LPM / 0.9 m3/h3
Holes – Micro holes all around periphery and in both inner and outer tubes. Hole size is 0.2 mm
Usage length – Flexible and can be made into any grid or shape. Maximum length with single injection of air will depend on depth of installation and air pressure. Due to double wall holes, long lengths are possible with minimum reduction in pressure along the length unlike in single wall diffuser.
To know more call us at:
+91 – 9652122060
Email: [email protected]
www.buy.airoxi.com
#envirment #everyone #airoxitube #airoxi #airoxidiffuser #airoxiproducts #aquarium #aquaculture #fishing #fishingfarm #shrimp #shrimptank #fishtank #tank #RAS #aeration #aerationsystem #bubble #post #postoftheday #oxygenation #hatcheries #shrimpfarm #aqualife #blower #microbubblegenerator #transportationaeration #turbineblower #submersibleaerator #biofloc #growoutponds #nurseries #oxygenconcentrator #diffuser
#fish farming#aeration solution#shrimp farming#airoxi tube#aquaculture#airoxitube#aeration tubes#airoxi#fish#aeration
0 notes
Text
Water quality monitoring analyzers are devices used to assess and analyze water's chemical, physical, and biological characteristics. These analyzers help in determining the overall quality of water and identifying any potential contaminants or pollutants present. Here are some commonly used water quality monitoring analyzers:
pH Meters: pH meters measure the acidity or alkalinity of water. They provide information about the water's pH level, which is an important parameter in assessing water quality.
Turbidity Meters: Turbidity meters measure the cloudiness or haziness of water caused by suspended particles. High turbidity levels can indicate the presence of pollutants or sedimentation.
Conductivity Meters: Conductivity meters measure the ability of water to conduct an electrical current. They provide information about the dissolved solids or salts present in the water, which can indicate pollution or changes in water composition.
Dissolved Oxygen Meters: Dissolved oxygen meters measure the amount of oxygen dissolved in water. Oxygen is crucial for aquatic life, and low levels can indicate pollution or the presence of organic matter.
Total Organic Carbon (TOC) Analyzers: TOC analyzers measure the concentration of organic carbon compounds in water. They help assess the level of organic pollution and are commonly used in industrial and wastewater treatment applications.
Nitrate and Nitrite Analyzers: These analyzers measure the concentration of nitrate and nitrite ions in water. Elevated levels of these compounds can indicate agricultural runoff or contamination from industrial sources.
Ammonia Analyzers: Ammonia analyzers measure the concentration of ammonia in water. High levels of ammonia can be harmful to aquatic life and indicate pollution from agricultural or wastewater sources.
Chlorine Analyzers: Chlorine analyzers measure the concentration of chlorine and its derivatives in water. Chlorine is commonly used as a disinfectant in water treatment, and its levels need to be carefully monitored to ensure safe drinking water.
Heavy Metal Analyzers: These analyzers measure the concentration of heavy metals such as lead, mercury, arsenic, and cadmium in water. Heavy metals can be toxic and pose a risk to human health and the environment.
Biological Oxygen Demand (BOD) Analyzers: BOD analyzers measure the amount of oxygen consumed by microorganisms during the breakdown of organic matter in water. They provide an indication of water pollution and the presence of organic contaminants.
These are just a few examples of water quality monitoring analyzers available in the market. The selection of analyzers depends on the specific monitoring requirements and the parameters of interest in a given water system.
0 notes
Text
What Is Turbidity in Water? (A Water Doctor Explains)

Turbidity is one of the common methods of measuring water quality. If you get a Water Quality Report from your local drinking water utility, you should see "turbidity" listed alongside other chemical and physical water parameters, like pH, temperature, and total dissolved solids (TDS). In this guide, we've answered the question, "What is turbidity in drinking water?" 📌 Key Takeaways: - Turbidity in water is a measure of a water's clarity or cloudiness. - Organic matter, clay, sediment, phytoplankton, algae, and other microscopic organisms all cause turbidity of a water body. - There are numerous methods of turbidity measurement, including with a secchi disk, turbidity meters, and transparency tubes. 🤔 What Is Turbidity? Turbidity is a measure of how much light can pass through a water body. Another way to look at turbidity is a measure of the clarity or cloudiness of a water source. The more scientific definition of turbidity is water's “relative clarity” – or the amount of light refracting off the suspended particles in the liquid. When measuring turbidity, higher levels of light refraction indicate a higher level of turbidity. 🔎 What Causes Turbidity? Turbidity is caused by any materials that have the ability to block a light beam from traveling straight through the water. There are a number of suspended particles and impurities that can cause turbidity. These include: - Organic and inorganic matter - Clay - Silt - Sediments from erosion - Phytoplankton - Other microscopic organisms How does turbidity get into water sources? Turbidity may increase naturally over time in a surface water supply. Other ways that turbidity may enter a water supply include from waste discharge, urban stormwater runoff (from impervious surfaces, construction sites, etc.), and soil erosion. Human activity can influence the concentration of suspended solids in a water supply. Farming activities and the use of phosphorous in wastewater treatment processes may increase turbidity due to increased algae growth in the water body. The type of water source often determines the source of turbidity. For instance, a shallow pond or lake in a warm climate is most likely to be affected by algae growth, while a fast-flowing river is more likely to be affected by sediment, decomposing organic matter, and other suspended material. 📐 How Do You Measure Turbidity In Water? Turbidity in water is measured in Nephelometric Turbidity Units (NTU). Some experts measure turbidity in Formazin Nephelometric Units, or FNU. The best way to take an accurate turbidity reading is with a turbidity sensor or turbidity meter (nephelometer). This turbidity measurement method measures scattered light in the water. There are other, cheaper methods of measuring turbidity, such as transparency tubes and secchi disks, which are often used in lake and stream monitoring programs. A laboratory analysis can also be used to measure total suspended solids in water, which are linked to turbidity (see below). 👨🔧 We've shared an in-depth guide on how to measure turbidity in water if you're looking for more information. source: United States Geological Survey (usgs.gov) 🆚 Turbidity vs Total Suspended Solids Turbidity and total suspended solids (TSS) are similar, but not the same. Turbidity refers to the concentration of material suspended in a water sample, and how this affects light penetration. On the other hand, total suspended solids (or suspended particles) are the materials in the water that cause turbidity. So, turbidity and TSS are linked because the higher the concentration of suspended solids, the more turbid the water. 🚱 What's Wrong With High Turbidity? High turbidity has an environmental impact and affects the quality of water that is used for drinking. In natural water bodies, high turbidity readings may harm aquatic life by raising the water temperature and reducing dissolved oxygen. This could affect the function of fish gills, reduce food supplies, and damage spawning beds. Very turbid water may even kill fish or reduce their growth rate, or make them less tolerant to disease. The treatment processes for turbid water used for drinking water and food processing are more complex and expensive. Water treatment facilities must work harder to make water with a high turbidity level clean and safe for drinking, compared to water with a low suspended particles count. Drinking water with low turbidity is usually safe, but excess turbidity could be dangerous for human ingestion, depending on what is causing the turbidity. Algae and bacteria in water could make you sick and increase the risk of waterborne disease outbreaks, while high sediment levels could damage your home's plumbing system. 📈 What Is The Maximum Allowed Turbidity In Drinking Water? There is no EPA MCL (Maximum Contaminant Level) for turbidity because turbidity isn't a single contaminant, and high turbidity levels in one water source could look very different than another. However, some of the individual solids that are found in turbid water are regulated by the Environmental Protection Agency. The World Health Organization (WHO) says that the maximum amount of turbidity that should be present in water is 5 NTU, but ideally, turbidity measured in water should be 1 NTU at most. 👨🔬 Should You Reduce Turbidity In Water? So, should you reduce turbidity in water? There's actually no straight "yes" or "no" answer to this question. Whether or not you should reduce turbidity in your water depends on the concentration of the turbidity, the contaminants causing the turbidity, and your own personal tastes. - Turbidity concentration - If your water turbidity exceeds 5 NTU (nephelometric turbidity units), you should look at methods to reduce turbidity because high levels of turbidity could affect water quality, safety, and taste. - Contaminants causing the turbidity - Certain sediments in your water might not have a big effect on your water quality, while turbidity caused largely by algae or impurities from waste discharge might be unsafe to drink. - Your own personal tastes - For health reasons or to improve the taste of your drinking water, you might personally decide to reduce your water's turbidity concentration even if it isn't higher than the safe level. To decide whether or not you should reduce turbidity in your water, test your water to see what you're dealing with. From there, you can decide on a suitable method of water treatment, if necessary. 📉 How To Reduce Water Turbidity There are a few different water filtration systems and treatment methods that can be used to reduce turbidity. In the water treatment plant, coagulation and flocculation is used to encourage suspended particulate matter to clump together, making them easier to remove from water. For at-home use, there are two popular methods of reducing turbidity: - Reverse osmosis - Ultrafiltration Both these systems use a membrane filtration process that traps the majority of total dissolved solids, effectively lowering water's turbidity. However, high turbidity concentrations may foul or damage the membrane. ❔ What Is Turbidity? FAQs What is the normal range for turbidity in water? The normal range for turbidity in water is 0.1 to 1 NTU (nephelometric turbidity units). Ideally, municipal water suppliers should aim to reduce turbidity levels to an average of 0.2 or less in treated drinking water, regardless of the initial water source or quality. Is high turbidity good or bad? High turbidity in drinking water is bad because it means there's a high concentration of sediments, algae, phytoplankton, and contaminants from urban runoff, which reduces water's aesthetic quality and potentially makes it unsafe to drink. Even in natural water sources, high turbidity is considered a bad thing because it could affect aquatic life, recreation, and tourism. How does turbidity affect water quality? Turbidity affects water quality because generally, the higher the turbidity levels, the higher the likelihood of poor water quality. That's because many of the causes of turbidity, such as algae and phytoplankton, influence water quality. Can you drink high turbidity water? No, you shouldn't drink high-turbidity water because certain contributors to turbidity (such as algae and waste discharge) may have health effects. Plus, high concentrations of turbidity often indicate the presence of disease-causing microorganisms, which have definite health effects. What level of turbidity is safe to drink? The level of turbidity that's considered safe to drink is 5 NTU or lower. Ideally, water should contain less than 1 NTU of turbidity for it to be guaranteed safe to drink. If you're drinking from an unknown water source, test its turbidity first, and avoid cloudy or dirty-looking water. Read the full article
0 notes
Text
Juniper Publishers-Open Access Journal of Environmental Sciences & Natural Resources
Impact of Sustained Discharge of Treated Wastewater Effluent on Wetland Water Quality
Authored by Roger Saint Fort
Abstract
This study investigated the impact on water quality of sustained discharge of treated wastewater effluent on the wetland commonly known as Weed Lake. A field sampling program was conducted during the months of May through September. Various physical, chemical, and biological analyses were performed on retrieved water samples. EC and TDS values increased both spatially and temporally from the south to the north quadrant of the wetland. Similar patterns were also observed for HPC and coliforms CFU enumeration. Turbidity and TSS decreased from May to June and then surged slightly while typically remaining constant from June to September. COD and TKN were found to decrease from May to July and to increase marginally from August to September. Potentially mineralizable nitrogen and phosphorous were ascertained as indices of the wetland sediment capacity for mineralizing both nutrients. Batch isotherms of PO4-3 were conducted for interaction determination with the wetland sediments.
The average value and standard deviation of potentially mineralizable nitrogen for the sediment samples was 15±12.92 mg/kg. Potentially mineralizable P was estimated at 29.67±11.96 mg/kg. The nitrogen pool of NH3-N in the wetland ecosystem was double the NO3-N pool. Langmuir data indicated an average maximum sorbing capacity of 190mg of PO4-3per kg of substrate. The value constants of maximum sorbing capacity ranged from 100 to 294mg of PO4-3 per kg of substrate. Total phosphorus concentration increased significantly from June to July and then decreased in September, these concentration changes were in parallel with algal and plankton blooms. Mass balance analysis indicated that 40 to 80% of phosphorous in Weed Lake is in various complex forms and not readily bioavailable. Labile-P and soluble-P represent a smaller fraction of the total-P. It appears that uncontrollable natural factors will have episodic and direct influence from year to year on the speciation of phosphorous and nitrogen in the wetland and influence also the spatial-temporal relationship status of its water quality. As a natural dynamic ecosystem, implementation and evaluation of best management practices should be continued together with subsequent evaluation.
Keywords: Water quality; Wastewater; Nutrient; Isotherm; Mass balance; Algae
Introduction
Weed Lake is a 6 km2 surface area of historic wetland and highly valued as an important natural feature within the community. Hereafter referred to as Weed Lake or wetland, its orientation is a south-north direction. The subject site is located 20 km east of the City of Calgary and northeast of the Hamlet of Langdon within the Municipality District (MD) of Rocky View No. 44 (Figure 1). Glenmore Trail and TransCanada Highway represent important access roads along the southeastern corner and the northwestern boundary of the Hamlet. Weed Lake was previously a healthy functioning wetland ecosystem characterized by important waterfowl production, breeding habitat and staging area for a large number of shorebirds in transit during migration. The wetland was drained in 1971. However, because of a variety of soil fertility problems, the expected agricultural benefits of draining the original wetland were never achieved.
A Restoration and Rehabilitation Program were initiated in 2006 to restore Weed Lake as a dynamic and fully functioning wetland ecosystem. Currently, Weed Lake is a productive ecological aquatic environment that hosts a variety of aquatic plants, namely: emergent aquatic vegetation, floating leaved plants, submergent aquatic plants and free floating plants. The climate of the area is described as being largely sub-continental characterized by short and moderately warm summers, brief spring and fall seasons. The winter is rather long. The ambient temperature typically ranges between -350C and 250C. Average annual precipitation is approximately 55 cm. The surrounding land uses around the wetland is primarily agricultural comprising mainly of farmstead parcels and un-fragmented quarter sections [1].
The soils of Weed Lake were classified as Rego Gleysols and the major portion of the upland as Solonetznic soils [2]. These soils, while high in soluble salts and sodium, are predominantly heavy glacial tills to clay loam. Soils in the dipressional areas are classified as Rego Gleysols and were developed in fine textured, lacustrine sediments. Weed Lake area is generally flat consisting of sections sloping gently from the west toward the lake at an approximate rate of 0.25%. The upland landscape is gently undulating. Two major depressional areas exist in the center of each SE and NW portions of Weed Lake TP 23 R27 W4. In some areas, the banks of Weed Lake provide a sudden and discernible drop in surface topography. Weed Lake received a constant source of tertiary wastewater effluent influxes from the Hamlet's wastewater treatment facility during the spring, summer and fall seasons. The wetland shore line is relatively flat with the lake depth being less than 1.20 meter over much of its area. Typical annual water level varies from 76 to 55 cm.
Storm water from the Hamlet flows into the lake through a network of major and minor ditch systems. Influx of storm water from the Balzac area also flows into Weed Lake and is routed through the main drainage ditch through the Hamlet. The former may carry considerable amount of sediments and salts, especially in the spring season. Irrigation water is in some instances supplemented to stabilize the wetland water level during periods of drought. Flows out of the wetland are controlled by an outlet located east of the wetland and is mainly drained by Rosebud Creek. The main objectives of this study were to ascertain Weed Lake water quality through the months of May to September, and to gain insights into the relationship between basic physicochemical and biological processes. Hence, the impact on water quality of sustained discharge of treated effluent in Weed Lake can be scientifically evaluated.
Materials and Methods
Weed Lake Surface Water Sampling
The wetland was sampled once a month through the months of May to September. Representative grab samples were retrieved from five sampling locations and their respective global positioning system (GPS) coordinates recorded. Subsequent sampling of the wetland was achieved based on each sampling location precise and unique identifier. All water samples were collected in one liter sterilized glass bottle at depth of 12 cm below the surface water level. The process involved lowering the capped bottle into the water column at the desired depth. The cap was then removed to allow water collection. Once filled, the bottle was capped, brought to surface and appropriately labelled. The water samples were then transported in Calgary and kept refrigerated at 70C for subsequent chemical and physical analysis. Field measurements of water temperature, dissolved oxygen (DO), and water level were performed at each sampling location at sampling time.
The remaining physical, chemical, and biological parameters were performed in the laboratory. Water samples for bacteriological analyses were collected from each sampling location in labeled, 50 mL sterile containers and brought back to Calgary. The sterile containers were transferred into a cooler for transportation. The cooler temperature was maintained at 50C. The samples were then serially diluted and incubated within 3 hours after sampling.
Weed Lake Potentially Mineralizable -Nitrogen (PMN) and -Phosphorous (PMP)
The process involved placing 10 g of air-dry substrate into a 150 mL acid washed Pyrex flask to which 25mL of 0.01mL CaCl2 was added [3]. The suspension in the flask was gently mixed, weighed, capped with Al foil and placed in the autoclave overnight at 1210C and 1500 kPa. Then, the flask and its contents were allowed to cool to room temperature, weighed, and quantitatively adjusted with deionized water. The mixture was transferred in centrifuge tubes and spun for 4 minutes at 4500 RPM and the liquid filtered through Whatman No. 2 filter paper. The difference between the autoclave treatment and the NH3-N, NO3-N and PO43 initially present in the substrate indicates the NH3-N, NO3-N and PO4-3 produced by autoclaving.
Weed Lake Batch Isotherm of Orthophosphate
To carry out the isotherm study, six sediment samples were collected from the bottom of the wetland using a hand Dutch auger. The substrates were transferred in one liter wide mouth glass jar, accordingly labeled and stored in the fridge at 40C. Prior to initiate the batch isotherm study, the samples were allowed to equilibrate with the room temperature. Batch testing was used to obtain the equilibrium sorption capacity of a given sediment sample for a corresponding concentration of phosphorous (PO43). This was achieved by developing equilibrium isotherms that describe the sorption capacity of the sediment exposed to various concentrations of aqueous solutions of PO43. Batch studies were conducted by gently mixing a specific amount of the sediment substrate with a specific volume of PO4'3 solution. The latter was prepared with deionized water. Each sorbate/sorbent system was then allowed to equilibrate overnight at room temperature and 80C, respectively. Following equilibration, the suspension was centrifuged at 5000 RPM for 4 minutes.
The supernatant from each system was retrieved and analyzed for the construction of the isotherms. The extent of PO4'3 sorption was estimated as the difference between initial concentration of PO4'3 and final concentration at equilibrium. Corrections were made for PO43 present in the original substrate.
Weed Lake Material Balance Analysis
Several assumptions were made in assessing Weed Lake, being a dynamic system with finite capacity to cope with anthropogenic inputs which can affect its intrinsic quality. A steady state equilibrium conditions exists between precipitation and evaporation,
a) A steady state equilibrium conditions exists between upward capillary groundwater movement and seeping water,
b) Outgoing water released through the gate doesn’t represent a significant enough volume of water to disturb the overall equilibrium status of Weed Lake under current water management,
c) Incoming water from any tributary doesn’t represent a significant enough volume of water to disturb the overall equilibrium status of Weed Lake,
d) The rate of organic matter decay in relation to nutrient mineralization are in equilibrium interaction with uptake by the aquatic vegetation and growth dynamics,
e) Lateral averaging assumes longitudinal and vertical hydrodynamics variations in velocities, constituents, water density, and temperature are negligible,
f) Sediment oxygen demand (SOD) is coupled to the water column dissolved oxygen.
Water quality interactions are by necessity simplified for descriptions of most aquatic ecosystems that are intrinsically complex. Hence, Weed Lake can be scientifically analyzed with considerable flexibility as a steady-state conservative system without any potential technical limitations of great significance. Based on Weed lake geometry and boundary, the major perturbations affecting the Lake hydrodynamics and quality during the investigated months of May through September are created primarily by wind and temperature induced circulation. In that regard, to a lesser extent by hydraulically induced turbulence and circulation. Wind causes Weed lake water column to be mixed through circulatory motion that extends to the bottom. This echoes the technical sentiment that complete water mixing occurs in the wetland boundary within a timeframe of 10 days. As a result, temperature differential between surface and bottom heat exchange is therefore considered conservative.
Analytical Program
*Not applicable; “Chemical oxygen demand; bTotal suspended solids; cTotal dissolved solids; "Dissolved oxygen; eSodium absorption ratio = {[Na/([Ca + Mg]/2)1/2}
The analytical testing program along with the associated quality assurance and control (QA/QC) for the study are depicted in Table 1. Standard recommended holding times were followed for the samples. Bacteriological analyses were performed on water samples collected in sterile jars. Serial dilutions were accordingly performed on the water samples. Subsequently, the diluted samples were incubated using the recommended Millipore media pad for heterotrophic plate count (HPC) and Escherichia coli (E. Coli) & Coliform organisms. The number of colony forming units (CFU) were enumerated and recorded.
Results and Discussions
Weed Lake Potentially Mineralizable Nitrogen (PMN) and Phosphorous (PMP)
The results of PMN as an index of potential mineralization of organic nitrogen to inorganic nitrogen forms of NH3-N or NO3-N are reported in Table 2. Reactive P initial present in these substrates were also evaluated and are reported in Table 3. The data indicate that the substrate found at the bottom of Weed Lake has the potential to contribute to the inorganic pools of N that is present in the water column. Total Kjeldahl nitrogen (TKN) which refers to the combination of ammonia and organic nitrogen ranged from 30 to 1.97mg/L likely contributes significantly to the mineralizable N pool. The average value and standard deviation of PMN for the six samples were 15±12.92 mg/kg while potentially mineralizable P was estimated at 29.67± 11.96mg/kg. Ratio of NH3-N/NO3-N was calculated for each autoclaved substrate and was found to oscillate around a ratio value of 2.
NA=Not applicable.
Therefore, the potential reserve of NH3-N in the wetland pool of PMN and PMP will vary with temperature, plants density, ecosystem is twice more than NO3-N. The dynamic and fate ofthat dissolved oxygen level, water level. Degree of hydrodynamicmixing of mineralized N is expected to be primarily driven by wind conditions. The results further indicate the presence of a pool of PO4'3 in the bottom boundary of the wetland. That pool is relatively bio-available for uptake by the aquatic plants that comprise Weed Lake ecosystem.
Weed Lake Batch Isotherm Study of Orthophosphate
Sorption isotherms are normally obtained by measuring the amount of solute sorbed for a number of different concentrations of sorbate under specific conditions. They often can be described by the Freundlich or Langmuir equations. The Freundlich isotherm, a commonly used curvilinear model has no upper limit to the amount of sorbate that could be sorbed by a system. The Freundlich equation, Equation (1) can be written as:
where X/M is the quantity of PO4'3 sorbed per unit mass of sediment (mg/kg), Ceq is the equilibrium concentration of PO4'3 in the solution (mg/L) and where Kd and n are the constants. The logarithmic form of the Freundlich equation to plot the data is shown in Equation (2):
The logarithm of the concentration of the solute in the sorbate state, X/M in mg/kg, is plotted as a function of the logarithm of the residual solute concentration, Ceq in mg/L. Linear regression of the data points yields a best-fit line with a slope of 1/n and intercept of log [Kd]. The slope, 1/n, is a measure of sorption intensity, and the Kd value, which must be determined by taking the antilog of the intercept, is the partition coefficient, an indicator of the sorptive capacity of the system. A Langmuir plot models a system where there are a finite number sorption sites. The Langmuir equation, Equation (3) may be expressed as follows:
Where X/M and C are the same units as defined above, bis a constant related to the binding energy (L/kg), and a is the maximum amount of solute that can be sorbed by the sorbate, is the soil uptake quantity (mg/Kg). The Langmuir equation can be rewritten in the following linear form shown in Equation (4):
[Ceq]/[X/M] can be plotted as a function of Ceq. The linear regression of the data points yields a best-fit line with a slope of 1/a and 1/ab as the intercept. The maximum amount of PO4'3 that can be sorbed onto the samples can be calculated from the Langmuir linear equation.
The isotherm models coefficients for the representative equilibrium studies are summarized in Table 3. The regression equations and the corresponding coefficient of determination (R2) are given in Table 3. It appears that the P sorption data of solution could be best described mathematically by the Langmuir equation. In this current case, the Langmuir data indicate that the Weed Lake sediment has an average maximum sorbing capacity of 190 mg of PO43 per kg of substrate. The value constants of maximum sorbing capacity ranged from 100 to 294 of PO4'3 per kg of substrate which reflect the heterogeneity in the composition of the sediment substrate. Therefore, the sediment substrate serves as a significant sink for PO4'3 as long as the sorbing capacity is not exceeded nor the pH becomes basic. The latter case has not been observed throughout the duration of the monitoring program.
Weed Lake Water Monitoring
The trend in water quality status of the wetland was compiled in Table 4 in a comparative summary as average values for the parameters tested for years 2009 to 2016, respectively. Under the objectives of this research project, the parameters were compared to current Surface Water Quality Guidelines for Use in Alberta with respect to Freshwater Aquatic Life for long term [4]. The guidelines are numerical concentrations or narrative statements recommended to support and maintain a designated water body. The wetland was scientifically analyzed with flexibility as a steady-state conservative system with limited potential technical limitations of meaningful significance. Based on its geometry and boundary, it is deduced that the major perturbations affecting the hydrodynamics and water quality during the investigated months are created primarily by wind and temperature-induced circulation and to a lesser extent by hydraulically induced turbulence and circulation.
aTurbidity Guideline; bTSS Guideline; cDO Guideline; dLake Temperature Guideline.
Wind causes water column mixing through circulatory motion that likely extends to the lower boundary of the water column. Complete mixing of the wetland water is conceived to occur within a time frame of ten days. As a result, temperature differential between surface and bottom heat exchange is considered conservative as well as spatially throughout the water mass. Observed temperature difference, AT, is less than 20C, both in the water column and spatially, irrespective of the sampling month. Temperature differential between surface and bottom heat exchange is considered conservative [5]. As expected, the importance of hydraulic, thermally, and wind- induced circulation in active interactions and combinations in bringing about dynamic changes in Weed Lake water quality varies depending on the specific parameters. Analysis of water quality indicators show that some parameters are more affected in varying degree in their distribution pattern with respect to spatial and seasonal variations.
Data spatial variability within a sampling event appears not to be significantly different from one sampling point to the next. Annual recorded chemical and physical parameters for grab samples tend to marginally increase spatially and temporally with corresponding standard errors ranging from 1.3% to 15%. This supports the assumption that complete mixing of Weed Lake water column is enhanced through wind induced circulation patterns which negate the geometry effects in inducing intrinsic spatial variability. Biological parameters of HPC-total and E. coli & Coliform colony forming unit (CFU) enumeration tend to decrease from the south to the north quadrant of the wetland. The south quadrant is where the pipe discharges treated effluent from the wastewater plant into the wetland.
Enumeration of CFU for both biological parameters spatially appear to vary randomly, hence no discernible pattern could be denoted. However, the level of CFU enumeration tends to increase with temperature. The lowest level of bacteria and coliform organisms are typically denoted for the months of May and September. CEC and TDS values tend to increase both spatially and temporally from the south to the north quadrant. This could be best attributed to influxes of storm water diverted into the wetland. Turbidity and TSS generally decreased from May to June and then marginally increased to remain constant from June to September. COD and TKN typically decreased from May to July but slightly increased from August to September. The trend observed in relation to turbidity, TSS, COD and TKN clearly reflects the combining effects of hydrodynamic mixing with a high degree of photosynthesis and metabolic synergistic relationships between algae and bacteria.
Furthermore, when ascertaining the trend in the data for the average parameters from years 2009 to 2016 (Table 5), a pronounced difference can be observed. Both spatial and temporal variations can be best corroborated to operational changes, natural factors, and seasonal variations. These factors will interact to accordingly create new episodic dynamic equilibrium in the wetland functional systems. According to Table 5, the trend in values for pH, DO, and water temperature remained the most relatively stable as denoted by their respective standard deviation and standard error. The overall stability of the pH is also a good indication of the wetland buffering capacity. Functionally, the ability to resist change in pH can be attributed to the presence of CO2, CO3-2, HCO3- and organic matter whose origins are primarily of natural sources and from operational activities (i.e., wastewater effluents) to a lesser degree. Given the presence of decomposable organic materials at the bottom of the wetland, the following reduction processes may be occurring. They may naturally further sulfate-enrich the water chemistry and contributing to the buffering capacity as depicted in Equation (5) and Equation (6):
The presence of dissolved oxygen is of fundamental importance in maintaining aquatic life and the aesthetic quality of Weed Lake. Because of this importance, oxygen can be regarded as one of the most important water quality parameters.The impact is measured as oxygen demand, a parameter that can be interpreted as a gross measure of the concentration of oxidizable materials present in the water column and as a status of potential organic load. Through the metabolic action of bacteria, organic material (OM) in the water column is oxidized to its lowest energy state through the following mechanisms, Equation (7):
Dissolved oxygen profile in the water column tends to increase from the months of May to June (representative average 8.94 mg/L) and then drastically decrease during July and August (representative average 4.07 mg/L) to subsequently route to a marginal increase in September (representative average 6.42 mg/L). No stratification of dissolved oxygen was denoted in the water column during any of the monitoring programs. Dissolved oxygen at the bottom-water interface was totally lacking, therefore making it an anaerobic, organic matter rich boundary in the wetland. Above the anaerobic substrate layer, an intermediate transition zone was not identified which contained dissolved oxygen. Following episodic discharge of treated wastewater effluent, its mixing through the water column, and after some time has elapsed, several changes occurred.
The various inter and intra relationships between wind action, temperature, precipitation, and sunlight are germane to the wetland intrinsic stabilization processes. In that regard, these factors are natural and not controllable. Based on dissolved oxygen readings, stabilization reactions appear to be aerobically dominated. During the stabilization processes, much of the biodegradable organic matter pool is transformed by bacteria into living organic matter. As a by-product of their metabolism, they release through mineralization into the water column CO2, nitrates, phosphates, sulfates, and other mineral salts. A conceptual framework depicted in Figure 2 provides a fundamental representation of physicochemical and biological dynamics occurring in the wetland. Such conceptual model would aid in long-term nutrient mass balance monitoring and developing a better understanding of nutrient systems behavior.
Aerobic stabilization is highly influenced by the hydrodynamic mixing created by wind action, temperature, and sunlight. However, at lower temperature like during the months of April, May, and October, the overall biological activities and therefore, the stabilization processes in Weed Lake are slower. Consequently, the algae level remains relatively low. As temperature of the wetland water column increases, this triggers in response higher algae metabolic activity. Under the additional favorable natural conditions of peak solar radiation during the months of June and July, algae proliferate. They absorb light and use the mineralized by-products during this highly photosynthetic period. Their cellular material releases oxygen in the water column, often giving rise to supersaturated conditions in some instances. Furthermore, the combination of high temperature, dissolved oxygen and solar radiation as well as the quick stimulation of bacterial activity enhance oxygen consumption.
This therefore stimulates blue-green algae (Cyanophyceae) to grow exponentially relative to the more efficient green algae (Chlorophyceae). As a result, heavy algal blooms are observed in the wetland with thick green mats appearing on the water surface. From this point on, anaerobic conditions arise, the column water is typically turbid and objectionable anaerobic odours are produced and released. The month of August typically produces anaerobic conditions as evidenced by dissolved oxygen average concentration of 2.73 mg/L. Since algal oxygen production is a direct function of photosynthesis, a gradual decrease in water temperature will be accompanied by decreased algal activity and therefore lower oxygen production. Consequently, the floating green mats on the water surface impair light penetration. Consequently, decreased photosynthesis in deeper portions of the water column results in decreased oxygen production together with a decrease in algal activity.
Under these conditions, wind action doesn't mix the water mass sufficiently to transport oxygen from the surface to the lower layers. At this point, the algal mass starts dying off, decaying through induced microbial decomposition, which results in the settling of biomass to the bottom boundary. This leads to the development of an organic layer of substrate that works as an anaerobic digester. It is noteworthy that this chain of events represents the natural ecological order and stabilization processes in the wetland. The COD test was used to ascertain the organic carbon content. Unlike biological oxygen demand (BOD), the COD test does not differentiate between biologically oxidizable and inert organic matter. Based on the overall trend in the data analysis, COD values tend to decrease from May to June as well as July but the COD values then increase or decrease from June to July and tend to increase during the months of August to September.
Typically lower DO readings are observed in July and August because of the past presence of higher level of oxidizable materials in the water column. Minimal DO stratification was observed throughout the depth of Weed Lake indicating significant longitudinal and vertical hydrodynamics mixing in the water column. Anoxic characteristic of the wetland in May results in some degree of objectionable odor as a result of bacterial reduction of NO3-, SO42, NH3-N, and other products of bacterial growth. Algae growth was more abundant during the months of May to July but significantly decreased during the months of August and September. Depicted in Tables 6-10 is the ratio of total-P/NH3-N for the wetland water column and treated effluent as well as the ratio for PO43/NH3-N for selected representative years. The ratios can be used as an important determining factor in assessing on an ongoing basis the management of the water quality with respect to effluent influxes and episodic water release from Weed Lake.
Main Nutrients Budget Analysis
The wetland aquatic environment is the host for a variety of aquatic plants namely emergent aquatic vegetation, floating leaved plants, submergent aquatic plants and free floating plants. The months of May to September mark the periods of active microbial and aquatic growth in Weed Lake. The data indicate that in order to maintain proper water quality and managing algal bloom as well as potential offensive odor, particularly for the critical months of July and August, it would be prudent to maintain a treated effluent discharge with a ratio of total-P/ NH3-N around 0.55. However, an effluent ratio of total-P/ NH3-N between 0.55 and 1.45 should be effectively adequate in maintaining proper water quality of the wetland.
A significant improvement in the wastewater treated effluent quality has been observed from the data recorded between the years 2010 to 2014. The results indicate for the year 2014 a treated effluent ratio of total-P/NH3-N ranging from 0. 05 to 0.74 and a water column ratio of PO43/NH3-N varying from 0.14 to 0.23. With respect to year 2010, the treated effluent ratio of PO43/NH3-N oscillated between 0.13 and 1.22 while the water column ratio of PO4-3/NH3-N varying from 0.26 to 1.02.
Variations in those ratio values were the norm for the other comprising monitoring years. Consequently, the water column ratio of total-P/NH3-N and PO4-3/NH3-N significantly improved from 2010 to 2014. The water column ratio of total-P/NH3-N in 2014 was established to range from 0.30 to 1.88 while it was from 1.07 to 2.68 in 2010. Furthermore, in 2014, the water column ratio of PO43/NH3-N ranged from 0.14 to 0. 23 compared to year 2010 in which case it ranged from 0.26 to 1.02 (Table 7).
In a manner similar to nitrogen, phosphorous in the wetland is expected to cycle between organic and inorganic forms. However, phosphorous unlike nitrogen doesn't cycle as a gas. It has to be assumed that labile-P, the not so strongly sorbed intermediate form by the wetland sediment, represents a smaller fraction of total-P. It may enter in equilibrium with soluble-P to increase the level of orthophosphate in the wetland water column. Hence, the material balance constructed for phosphorous is orthophosphate and will be considered as the only form of phosphorus that is readily used by any aquatic plants and microorganisms in the wetland ecosystem. Therefore, significant amount of orthophosphate is removed through algal and macrophytes growth but more significantly during the months of May, June and July. As result keeping orthophosphate concentration relative low throughout the wetland ecosystem boundary.
Concurrently, conversion of dissolved forms of phosphorus into insoluble forms such as calcium phosphate Ca3(HPO4)2, magnesium phosphate Mg3(HPO4)2, and ferric phosphate FePO4 and AlPO4 complexes will contribute to the removal and control of PO43 concentration level as well as to controlling its buffering capacity. Additionally, phosphorous bioavailability may be significantly affected through organic complex formation with humic and fulvic acids. Given the water pH being greater than 8.50, assumptions must also be made regarding the activities and contribution of Al retention of PO43 onto the sediment as AlPO4 complex. The later complex will be closely related to the contents of acid-extractable Al+3 in the sediment. Total phosphorus (i.e., P-inorganic and P-organic) concentration increased significantly from June to July to decrease in September which parallels algal and plankton blooms (Table 8).
Weed Lake Material Balance Analysis
A simple water budget approach was used to analyze the water level dynamics in Weed Lake Equation (8):
A simple water budget approach was used to analyze the water level dynamics in Weed Lake Equation (8):
R = Volume of present in the wetland at any time,
Pr =Annual precipitation,
Inwt = Incoming water from the wastewater treatment plant, Tr = Tributaries feeding into the wetland,
Ca = Capillary groundwater feeding the wetland,
E = Evaporation of water from the wetland,
vap r '
Se = Seepage water from the wetland,
Out canal = Water released in the canal via the gate.
Therefore, the above water budget equation for the wetland can be simplified and rewritten as Equation (9):
Of special significance to the wetland water quality management program are nitrogen and phosphorous. The interrelationships that exist between these two nutrients are best manifested in their fundamental importance in eutrophication. A mass balance analysis was performed for phosphorous and nitrogen, both being the nutrients of greatest input from the wastewater effluent being regularly discharged into the wetland. The following assumptions were derived in formulating the mass balance analysis for nitrogen and phosphorous (Tables 9,10). Assumptions made for nitrogen:
i. Biological conversion of organic-N ---> NH3-N is not significant from November to April
ii. Mineralization of NH3-N ---> NO3-N is not significant from November to April
iii. Biological reduction of NH3-N ---> NH3 (g) is not significant from November to April and, assumptions for phosphorous:
iv. Biological conversion of organic-P --->PO43 is not significant from November to April
v. Formations of insoluble forms of Ca (HPO4)2 and Mg (HPO4)2 are nominal relative to the overall process of soluble PO4-3 removal by microorganisms, algae and plankton.
vi. Equilibrium reaction exists between soluble PO43 <---> sediment sorbed PO4'3
vii. The material balance equation for can be written Equation (10) for each nutrient of interest:
Total-P, PO4'3and NH3-N are expected to undergo significant biotic and abiotic interactions during the months of May to September. Their decay rate could be modeled as a first-order reaction Equation (11). That is
where K is the reaction rate coefficient with dimensions of 1/ time and C is the nutrient concentration. According to Equation (11), the rate of loss of any of these nutrients is proportional to the amount of available substance that is present in the water column. The integrated first order rate law yields Equation (12):
where C is the initial concentration of NO-N or PO'3 at first
The regression equations for rate constant, K, determination of NO3-N and PO43 for selected years were derived for the wetland most biologically active period (Table 11). Assuming that both nutrients are uniformly distributed throughout the wetland water column, hence the total amount of a nutrient is calculated as CV according to Equation (14). Thus,
where C represents the concentration of nutrient and it is uniformly distributed throughout the volume, V, of the wetland water column. The material balance becomes Equation (15):
Monthly phosphorous and nitrogen load (MLP or N) as a result of effluent discharges can be calculated Equation (16):
Where, EmV represents monthly volume of effluent (L) and CE, effluent concentration (mg/L). Therefore, average volume of water (LV) in the wetland at sampling time Equation (17):
The mass balance analysis reveals a decreasing trend in nutrients level in the wetland. In 2012 the water matrix consisted of 45% of PO4-3 which translated into a load of 1.32 x 106 g with a corresponding load of 8017g of NH3-N meaning a ratio of PO4-3/NH3-N of 165:1. Concurrently, 55% of phosphorus can be assumed to be in the forms of condensed-phosphates and organically bound-phosphates which represent a load of 1.63 x 106g. With respect to 2013, the wetland water matrix was made up of 35% PO43. Which translated into a load of 1.34 x 106 g with a corresponding load of 1.6 x 105 NH3-N. Hence 65% of phosphorous in the water mass can be assumed to be in complex forms. For year 2014, it was determined that 82% of phosphorous status was in various complex forms. The direct impact of various uncontrollable natural factors will influence the speciation of phosphorous and nitrogen in Weed lake from year to year.
Nonetheless, if too much nitrogen enters the wetland through effluent discharge to offset the current nutrient ratio PO4-3/NH3-N, the result will likely be rapid and significant growth of aquatic vegetation in nuisance quantities and eventually lowering DO content due to the death and decay of the aquatic vegetation. As a natural dynamic system, implementation and evaluation of best management practices of Weed Lake should be continued and accordingly evaluated in order to strive to achieve and maintain a healthy wetland ecosystem.
Conclusion
The results of the Weed Lake water quality monitoring program study indicate spatial and temporal variations in the concentrations of the parameters tested. EC and TDS values tend to increase both spatially and temporally from the south to the north quadrant. Similar trend has been observed as it relates to HPC and coliforms CFU enumeration. Mass balance analysis indicates that 40 to 80% of phosphorous in the wetland water column is in various complex forms. Uncontrollable natural factors will have a direct influence from year to year on the speciation of phosphorous and nitrogen in the wetland ecosystem. Any surges or significant departure from the current nitrate inputs will have the potential to adversely affect the water quality and health of the wetland.
To know more about Juniper Publishers please click on: https://juniperpublishers.com/manuscript-guidelines.php
For more articles in Open Access Journal of Environmental Sciences & Natural Resources please click on: https://juniperpublishers.com/ijesnr/index.php
#Juniper Publishers PubMed Indexed Journals#juniper publishers publons#Ecological psychology#Environmental Chemistry#Geo Morphology#Limnology
0 notes
Text
Effect of Different Water Sources on Survival Rate (%) Growth Performance, Feed Utilization, Fish Yield, and Economic Evaluation on Nile Tilapia (Oreochromis niloticus) Monosex Reared in Earthen Ponds- Juniper Publishers
Abstract
The aim of the present study was to investigate the effect of water source on survival rate %, growth performance, feed utilization, fish yield, economic evaluation and production of Nile tilapia (Oreochromis niloticus) monosex reared in earthen ponds. Nine earthen ponds were used and divided into three categories of three earthen ponds each. The average size of each pond was approximately 5200m2, 6000 monosex all male Nile tilapia were used in each pond and were stocked for 192 days. The fingerlings average weight was 4.38±0.03g/ fish, the fish were fed using a floating feed 25% crude protein, and were fed at a daily rate of 3% of their body weight. Results showed that body weight was increased significantly (P<0.05) with well water to 472.33g/fish. While were 354.17 and 320.17g/fish for fresh and agricultural drainage water, respectively. Specific growth rates (SGR%) increased with well water compared to both fresh and drainage water. Feed conversion ratio (FCR) and protein efficiency ratio (PER) were improved with Agriculture drainage water. Survival rates with fresh and well water were 98.53% and 98.31% respectively, however, was 95.05% with Agriculture drainage water. Total fish yield were affected significantly by treatments. It was 2128, 1921.8, and 2837.7kg at fresh, drainage and well water respectively. Net return arrived to 12996 for well water source when it was 6784LE for agricultural drainage water and 9158LE for fresh water.
Keywords: Water resources; Nile tilapia; Growth per
Introduction
Nile tilapia, Oreochromis niloticus (Linnaeus) is one of the most important freshwater fish in world aquaculture [1]. It is widely cultured in many tropical and subtropical countries of the world [2]. Rapid growth rates, high tolerance to adverse environmental conditions, efficient feed conversion, ease of spawning, resistance to disease and good consumer acceptance make it a suitable fish for culture [3]. Farmed tilapia production increased semi dramatically in recent years, increasing from 383,654mt in 1990 to 2,326,413mt in 2006 [4]. Tilapia has established a secure position in a number of water impoundments of India. But, its performance in open water ponds of the country has been discouraging over the years [5]. For tilapia aquaculture is excessive reproduction and the resulting small size of the fish produced.
Egypt has suitable natural conditions for desert aquaculture. Egypt has vast resources of groundwater [6]. Fresh groundwater resources in Egypt contribute 20% to the potential water resources in Egypt. One of the groundwater resources is the Nile Valley and Delta system with the storage capacities of 200 billion m3 and 300 billion m3, respectively. Oasis water in the west desert, Bahariya, Farafra, Dakhla, Kharga, and Siwa, were established from underground natural wells and springs.
With the prohibition of the establishment of fish farms on agricultural land, with the prohibition of the use of Nile water for fish farming, with increased competition for spaces adjacent to the lakes and sources of agricultural drainage water, despite its disadvantages, has caused the possession of new fish farm in the Nile [7]. Valley of the most difficult things and out of reach. Hence the search for an alternative to invade the desert, especially with the development of methods of fish farming and providing the requirements of education and with the provision of underground water of the highest purity with different salinity (fresh & brackish & marine) and where the trained professionals are available [8]. In the hope to produce clean fish with improved quality and cheaper than other animal proteins we conducted the present research in a private fish farm located in the desert belonging to Noubaria Agricultural Development company (Ragab Farms) aiming to study the effect of water source on survival rate (%) growth performances, feed conversion ratio, protein efficiency ratio, annul fish yield and profitability Nile tilapia (Oreochromis niloticus) monosex commercial farming.
Materials and Methods
Water Source
Three types of water sources: fresh water, agricultural drainage water, and well water were compared in the present experiment. Water supplies were replaced three times during the experimental period (192 days).
Experimental design
Nine earthen ponds (5200m2) were used in these experiment were divided into three categories of earthen ponds even three ponds represent one treatment (fresh water, drainage water and well water.
Stocking density
6000 monosex all male Nile tilapia (Oreochromis niloticus) fingerlings of average weight (4.38±0.03g/ fish) were stoked in each pond on April 11, 2007 and observed through October 19, 2007. The area of each pond 5200m2.
Experimental Fish
Fingerlings of all male Nile tilapia (Oreochromis niloticus) monosex were collected from Noubaria Agricultural Development Company (Ragab Fish Hatchery) and were over wintered in earthen ponds to provide suitable fingerlings for the beginning of the growing season. All ponds in this experiment were sampled monthly using a cast net method. Sample sizes were 1% of the stocked numbers and the average individual fish weight was calculated to determine growth rates. Then, with these calculations, the feed amounts were adjusted for the following month.
Experimental diet
The floating commercial diet used in this experiment was fed at a daily rate of 3% of the fish body weight by using self feeders The ingredients of the commercial diet used in the experiment is presented in (Table 1). The dietary composition of vitamin and mineral premix is listed in. Fish were fed a floating ration for 6 days per week. Feeding rate was adjusted monthly based upon the calculated biomass of fish obtained through the monthly sampling and assumption of 100% survival.
Water quality
Physical parameters: Water temperature °C was determined at every days in the experiment.
Chemical Parameters: Samples for determination of dissolved oxygen (DO) were immediately fixed after sampling and DO concentration was determined according to Winkler's technique. Methods described by Golterman et al. [9] were used in determination of ammonia. Also pH was measured by digital pH meter (Orion model 720 A, s /No 13602) in all experiments.
Chemical Analysis of the commercial of Diet: Chemical analysis of the commercial diet used in the experiment was done according to AOAC (2000) as shown in Table 1.
Growth parameters and Statistical analysis: Data on growth, feed utilization, survival rate and proximate and chemical composition of whole fish body were subjected to one-way ANOVA [10]. To locate significant differences between fish size within different water resources of pond. Duncan's multiple rang test [11] was done. All percentages and ratio were transformed to arcsine values prior to analysis [12].
Results and Discussion
Experimental diet
The commercial diet used in the present experiment contained 25% CP and 4.3kcal/g gross energy (Table 1). Although there are large variations in the data available about the optimum protein level for tilapias which range between 20 and 40% crude protein [13-15] practical diets as low as 25% protein was successfully used for rearing monosex tilapia [3].
Vit. A 8000 I.U. Vit. D3 4000 I.U.; vit. E 50mg; Vit. k3 19mg;
Vit. B1 40mg; vit. B2 25 mg; Vit. B6 125mg; vit B12 69mg;
Pantothenic acid 40mg; Nicotinic acid 125mg; Folic acid 400mg;
Water quality
Collected data on water temperature and dissolved oxygen (DO), pH and ammonia are summarized in Tables 2-4. Water temperature throughout the present experiments ranged between 24.13±0.53 and 30.26±0.45 °C in fresh water experiment, 24.23±0.53 and 30.65±0.53 °C in drainage water experiment and between 29.94±0.12 and 33.63±0.43 in well water experiment which was the high temperature and closely related to the average of optimal value for tilapia (28-30 °C). Our results were agreement with Broussard [16] reported that tilapia as a warm water fish that dominate African lakes, are known to grow well in high temperature. The fluctuation of water temperature are reached its maximum values during August, however its minimum were during April and November.
*Each value was on average of four sub samples
Biotin 20mg; cholin chloride 80 mg; copper 400mg; Iodine 40mg;
Iron 120mg; Manganese 220mg; Zink 22mg; Selenium 4mg
Means in the same column having different letters are significantly different (P<0.05).
Overall means of water dissolved oxygen (DO) throughout the present experiment were 7.20±0.37mg DO/I for fresh water, 7.19±0.36mg DO/I for drainage water and 6.33±0.36mg DO/I for well water. The fluctuation of water dissolved oxygen (DO) showed that the maximum values of DO were obtained in November for the fresh and drainage water and August in well water, however, the lowest values were in April. In general, dissolved oxygen levels were within the high standards and higher than cited by Boyd [18] for good production of tilapia (4.20 to 5.90mg DO/I) in aquaculture ponds. One of the most important environmental factors is dissolved oxygen. It is considered a limiting factor for success or failure in intensive culture. An excellent aquaculture attribute of tilapia is their tolerance to low dissolved oxygen concentration [16]. The dissolved oxygen content in earthen ponds depends on the pond water temperature, fish biomass and rate of water exchange [18]. Chervinski [19] reported that O. niloticus survived short term exposure to 0.1mg DO/ l. However, Collins [20] observed in a review on oxygen concentration of various studies, that growth rate of non-salmonid fish was increasingly depressed as dissolved oxygen fall below 50% saturation. Rappaport et al. [21] reported that growth of carp was reduced by predawn dissolved oxygen less that 25% saturation. Tichert-C & Green [22] compared the growth of tilapia monosex in earthen ponds aerated or unaerated at 10 or 30% saturation of dissolved oxygen. They found that tilapia production and final weight were significantly greater in aerated ponds than unaerated ponds.
The water pH values throughout the present experiments ranged between 8.00±0.13and 8.10±0.13 with an overall mean of 8.04±0.13 in fresh water and ranged between 8.01±0.13 and 8.10±0.13 with an overall mean of 8.05±0.13 in drainage water and ranged between 7.98±0.13and 8.01±0.13 with an overall mean of 8.00±0.13 in well water. The fluctuations of pH reach the highest value of 8.10+0.13 during August in fresh and drainage water and 8.01+0.13 in well water. The results showed that the present pH values are suitable. For rearing tilapia monosex in earthen ponds. Johnson [23] recommended the range of pH 6.5 to 9.0 for most of freshwater fish species.
The water un-ionized ammonia (NH3) throughout the present experiments ranged between 0.09±0.01 and 0.12±0.01 with an overall mean of 0.11±0.01 in fresh water and ranged between 0.10±0.01 and 0.13±0.01 with an overall mean of 0.11±0.01 in drainage water and ranged between 0.06±0.01 and 0.10±0.01 with an overall mean of 0.077±0.01 in well water. The fluctuations of un-ionized ammonia reach the highest values of 0.13mg/ l during August. Unionized ammonia concentrations in the experimental ponds generally remained below levels which would cause chronic toxicity problems in tilapia. Tilapia is more tolerant to elevated levels of ammonia than more other sensitive species such as salmonids [23]. Some tilapias have been shown to acclimate to higher levels of ammonia after chronic exposure to low levels [24]. Johnson [23] showed that levels of un-ionized ammonia which may adversely affect growth in tilapia range from 1mg/ l to 2mg/ l ammonia where temperature and pH are within normal range.
Growth performance of tilapia monosex
Mean weight: Results of the present study showed that the mean weights at all rearing intervals different significantly (P<0.05) during all the experimental periods (Table 5 & Figure 1). Averages of fish body weights for fresh water, drainage water and well water were found to be 23.16, 18.66 and 25.16g, respectively after the 1st month of stocking. The statistical evaluation of results indicated that live weights at this period increased significantly (P<0.05) with using well water. A similar trend was also observed in fish body weights during the other growing periods. At harvest average body weight of fish stocked at well water was significantly (P<0.05) higher than that of fish stocked in fresh or drainage water, which indicates that weights fish were decreased in fresh and drainage water with increasing for used well water at harvest were 354.17g, 320.17g and 472.33g for fresh, drainage and well water, respectively. This significant advancement in fish body weights with increasing at higher temperature of water advocated by Azaze et al. [25] reported that the final mean weight was significantly higher at 26 and 30 °C than at 22 and 34 °C. This finding agrees with our results.
Average daily gain (ADG g/day): Results presented in Table 5 revealed that water sources, affected significantly (P<0.05) ADG during all experimental periods tested (30, 60, 90.120.150.180 and 210 days after start). In general these results indicated that the well water favored significantly ADG of the tilapia monosex in intensive culture system. The results of this point were in agreement with those found by [17] who grew O. niloticus from 49g to 271g in 122 days (1.4%/day). Siddiqui et al. [15] found that ADG of tilapia O. niloticus reared for 98 days at different water exchange in outdoor concrete tanks was 1.06g / day at 30% dietary crude protein. In the present study the average daily gain was higher with 25% crude protein at all treatments. However, the optimal feeding rate depends on fish size and Specific Growth Rate (SGR %): Results presented in Table 5, revealed that water sources, affected significantly (P<0.05) SGR% during all experimental periods tested (30, 60, 90.120.150.180 and 205 days after start). In general these results indicated that the well water, favored significantly (P<0.05) SGR% of the tilapia monosex in intensive culture system.
During all tested experimental periods tested (30, 60, 90.120.150.180 and 205 days after start) SGR% increased significantly (P<0.05) in almost linear manner in the well water than fresh and drainage water In the present study SGR% values in case of well water continuously higher than fresh or drainage water in all experimental periods. This may be due to the higher temperature of the well water (average 31.94 °C) compared to 27.47 and 27.81 °C for fresh and drainage water, respectively. The results obtained in SGR% are in agreement with those found by Eid & El Denasoury [27] who indicated that increasing temperature from 16 °C to 27 °C improve growth rate of Nile tilapia, which using well water.
Feed conversion ratio (FCR): Results presented in Table 5, show that there were significant (P<0.05) effects of water sources on FCR, feed conversion ratio was observed at harvest was 2.87 at fresh water, followed by 2.83 at well water and 2.80 at drainage water and 2.94 for 1700m2 followed by 2.89 for 4000m2 followed by 2.75 for 5200m2 and was 2.57 for 6000 fish/ acre, 2.75 for 8000 fish/ acre and 2.78 for 10000 fish/ acre. The analyses of variance of the FCR values are presented in Table 5. The FCR is affected by the physiological state of the fish, environmental condition, [28]. Lovshin et al. [29] found that FCR for all male tilapia in earthen ponds was higher (4.3) than when compared with all male and female tilapia in earthen ponds (FCR=7.2). while, fish growth is affected by the amount of feed consumed and the efficiency of assimilation [30].
Protein efficiency ratio (PER): Results of protein efficiency ratio (PER) are presented in Table 5, There were significant (P<0.05) effects of water sources, on PER, it improved significantly (P<0.05) with each increase in pond sizes and decrease stocking density throughout the experimental periods. The best PER observed at harvest was 1.42 with drainage water, followed by 1.40 at well water and 1.38 at fresh water Nyina-W et al. [31] confirmed that when protein supply is appropriate (400500g protein/kg feed for percid fish), different lipid contents in feeds do not have an impact on the rearing results of pikeperch.
Fish survival rate: Results in Table 6 showed that survival rates were changed significantly (P<0.05) by water resources, in fresh and well water were insignificantly (P<0.05) different but survival of the fish in drainage water was 95.05% which was less than survival rates in both fresh water and well water indicating the probable effect of some faction of water quality.
Fish yield: Results of Table 4 show fish yield (kg) per acre as affected by water sources,. Results revealed that total yield increased significantly (P<0.05) with well water. The total production was found to be 133.34% and 90.31% for well water and drainage water, respectively, while it was found to be 76.33% and 68.84% for 4000m2 and 5200m2.
The results of the present experiment were similar to those of Tal & Ziv [32] who showed that the net yield of tilapia monosex in earthen ponds was 16750Kg /ha (7035.0kg/ acre) after 100 days of stocking of 80.000 fish/ha, (33600 fish/acre, 8 fish/m2) on the other hand Eid & Denasoury [27] indicated that increasing temperature from 16 °C to 27 °C improved growth rate of Nile tilapia. Watanabe et al. [33] found that growth rates generally increase with increasing temperature and where markedly lower at 22 °C and well water is the best because the temperature constant through the year and the best quality of the water. [34] found the higher yield obtained in small pond sizes because the bigger ponds with greater surface area were more difficult to manage and often resulted in lower fish yields.
All fish species are characterized by an ideal range of temperature in which they show their maximum growth [3537]. Several studies have been reported that the specific water temperature range showed the faster growth in Pikeperch, Sander lucioperca at 20 °C to 25 °C [38-40]. Low temperature causes sluggishness by retarding the digestion speeding of fish [41]. Some researchers have found that the digestion rate has been increased as the temperature increases [42]. Environmental temperature is one of the most important ecological factor which also influence the behavior and physiological process of aquatic animals [43].
One of the major advantages of groundwater sources is their constant temperature throughout the year. Shallow sources of groundwater approximate the mean air temperature of the area. The chemistry of groundwater is directly dependent on the geology of the area surrounding the source. In limestone areas, groundwater is hard, and high in calcium and carbon dioxide [44]. In areas of granite formation, the groundwater tends to be soft, low in dissolved minerals and carbon dioxide. As will be discussed later, there are advantages and disadvantages to both, emphasizing the need for early extensive water quality testing.
Water temperature has substantial effect on fish metabolism. In response to decreasing of water temperature the enzyme activity of tissues have been increased [45]. Velmurugan et al. [46] have investigated that histopathological and tissue enzyme changes of C. gariepinus exposed to nitrite when water temperatures changes from 27 °C to 35 °C. In a stressful and unfavorable environmental condition GPT and GOT may increase in blood serum. In the present study serum GPT and GOT level were affected by different water temperature. Serum GPT and GOT amount in different fish fed at 20 °C are comparatively lower than those of fish fed at 16 °C and 24 °C experiments (Tables 1-3). These results indicated that 20 °C may be a favorable water temperature for better growth of 16g juvenile Korean rockfish [47,48].
For more about Juniper Publishers please click on: https://twitter.com/Juniper_publish
For more about Oceanography & Fisheries please click on: https://juniperpublishers.com/ofoaj/index.php
0 notes
Text
PORTABLE MULTIPARAMETER WATER QUALITY METER
Portable Multiparameter Water Quality Meter is equipped with a backlit LCD display. It is suitable for measuring the PH, ORP, ion concentration, TDS, salinity, conductivity, resistivity and dissolved oxygen. Automatic electrode recognition shows the pH slope and offset. Relative and absolute millivolt modes provide reliable ORP measurements. Data can be transferred easily to computer by using USB interphase. It is reliable, user friendly applicable for outdoor measurements. It is widely used for quality testing of water and also used in research and development laboratories and water resources
1-5 points push button calibration to automatically rectify pH buffer Reset function instinctive. It can store and recall up-to 500 sets of data Rely resumes all settings back to the factory defaults Automatic temperature compensation provides accurate readings over the entire range salinity's and barometric pressure compensations remove the measurement error Water quality meter contains 8 measurement modes.
portable multiparameter water quality meter is a versatile device designed to measure and monitor several key parameters of water quality in various field settings. These meters are commonly used in environmental monitoring, water quality assessment, research, and regulatory compliance across different industries. These meters can simultaneously measure several important water quality parameters, which may include pH, conductivity, turbidity, dissolved oxygen (DO), temperature, total dissolved solids (TDS), oxidation-reduction potential (ORP), ammonium, nitrate, and more. Scientists and researchers in various fields, such as ecology, biology, and geology, use these meters for fieldwork to study the environment, aquatic ecosystems, and aquatic species. Portable multiparameter meters are valuable tools in emergency response situations, helping to quickly assess water quality after natural disasters or environmental incidents. These meters are used in educational institutions to teach students about water quality testing and environmental science.
0 notes
Text
Water pollution in India
New Post has been published on https://sos.greenpeace.fund/water-pollution-in-india/
Water pollution in India
Water pollution is a major environmental issue in India. The largest source of water pollution in India is untreated sewage. Other sources of pollution include agricultural runoff and unregulated small-scale industry. Most rivers, lakes and surface water in India are polluted due to industries, untreated sewage and solid wastes.
The issues
Untreated Sewage
We found that discharge of untreated sewage is the single most important source of pollution of surface and ground water in India. There is a large gap between generation and treatment of domestic waste water in India. The problem is not only that India lacks sufficient treatment capacity but also that the sewage treatment plants that exist do not operate and are not maintained.
The majority of the government-owned sewage treatment plants remain closed most of the time due to improper design or poor maintenance or lack of reliable electricity supply to operate the plants, together with absentee employees and poor management. The waste water generated in these areas normally percolates into the soil or evaporates. The uncollected waste accumulates in the urban areas causing unhygienic conditions and releasing pollutants that leach into surface and groundwater.
A 1992 World Health Organization study reported that out of India’s 3,119 towns and cities, just 209 have partial sewage treatment facilities, and only 8 have full wastewater treatment facilities. Downstream, the river water polluted by the untreated water is used for drinking, bathing, and washing. A 1995 report claimed 114 Indian cities were dumping untreated sewage and partially cremated bodies directly into the Ganges River. Lack of toilets and sanitation facilities causes open defecation in rural and urban pill areas of India, like many developing countries. This is a source of surface water pollution.
Sewage discharged from cities, towns and some villages is the predominant cause of water pollution in IndiaInvestment is needed to bridge the gap between sewage India generates and its treatment capacity of sewage per day. Major cities of India produce 38,354 million litres per day (MLD) of sewage, but the urban sewage treatment capacity is only 11,786 MLD.[8] A large number of Indian rivers are severely polluted as a result of discharge of domestic sewage.
The Central Pollution Control Board, a Ministry of Environment & Forests Government of India entity, has established a National Water Quality Monitoring Network comprising 1,429 monitoring stations in 28 states and 6 in Union Territories on various rivers and water bodies across the country. This effort monitors water quality year round. The monitoring network covers 293 rivers, 94 lakes, 9 tanks, 41 ponds, 8 creeks, 23 canals, 18 drains and 411 wells distributed across India.Water samples are routinely analysed for 28 parameters including dissolved oxygen, bacteriological and other internationally established parameters for water quality. Additionally 9 trace metals parameters and 28 pesticide residues are analysed. Biomonitoring is also carried out on specific locations.
The scientific analysis of water samples from 1995 to 2008 indicates that the organic and bacterial contamination is severe in water bodies of India. This is mainly due to discharge of domestic waste water in untreated form, mostly from the urban centers of India.
Organic matter
In 2010 the water quality monitoring found almost all rivers with high levels of BOD (a measure of pollution with organic matter). The worst pollution, in decreasing order, were found in river Markanda (490 mg/l BOD), followed by river Kali (364), river Amlakhadi (353), Yamuna canal (247), river Yamuna at Delhi (70) and river Betwa (58). For context, a water sample with a 5-day BOD between 1 and 2 mg O/L indicates a very clean water, 3 to 8 mg O/L indicates a moderately clean water, 8 to 20 indicates borderline water, and greater than 20 mg O/L indicates ecologically-unsafe, polluted water.
The levels of BOD are severe near the cities and major towns. In rural parts of India, the river BOD levels were sufficient to support aquatic life.
Coliform levels
Rivers Yamuna, Ganga, Gomti, Ghaghara River, Chambal, Mahi, Vardha and Godavari, are amongst the other most coliform polluted water bodies in India. For context, coliform must be below 104 MPN/100 ml, preferably absent from water for it to be considered safe for general human use, and for irrigation where coliform may cause disease outbreak from contaminated-water in agriculture.
In 2006, 47 percent of water quality monitoring reported coliform concentrations above 500 MPN/100 ml. During 2008, 33 percent of all water quality monitoring stations reported a total coliform levels exceeding those levels, suggesting recent effort to add pollution control infrastructure and upgrade treatment plants in India, may be reversing the water pollution trend.[3]
Treatment of domestic sewage and subsequent utilization of treated sewage for irrigation can prevent pollution of water bodies, reduce the demand for fresh water in the irrigation sector and become a resource for irrigation. Since 2005, Indian wastewater treatment plant market has been growing annually at the rate of 10 to 12 percent. The United States is the largest supplier of treatment equipment and supplies to India, with 40 percent market share of new installation. At this rate of expansion, and assuming the government of India continues on its path of reform, major investments in sewage treatment plants and electricity infrastructure development, India will nearly triple its water treatment capacity by 2015, and treatment capacity supply will match India’s daily sewage water treatment requirements by about 2020.
The Solution
Water conservation in India is gaining pace. The Ganga rejuvenation efforts by the union government, the Yamuna clean up are some of the government initiated efforts. The Chennai River Restoration trust’s efforts to clean the Cooum, Adyar rivers in Chennai and civil society efforts spearheaded by organizations like Environmentalist Foundation of India (E.F.I) to clean lakes and ponds in the country are seen as significant development towards water conservation.
Other problems
A joint study by PGIMER and the Punjab Pollution Control Board in 2008, revealed that in villages along the Nullah, fluoride, mercury, beta-endosulphan and heptachlor pesticide were more than permissible limit (MPL) in ground and tap water. Plus the water had high concentration of COD and BOD (chemical and biochemical oxygen demand), ammonia, phosphate, chloride, chromium, arsenic and chlorpyrifos pesticide. The ground water also contains nickel and selenium, while the tap water has high concentration of lead, nickel and cadmium.[16]
Flooding during monsoons worsens India’s water pollution problem, as it washes and moves solid waste and contaminated soils into its rivers and wetlands. The annual average precipitation in India is about 4000 billion cubic metres. From this, with the state of Indian infrastructure in 2005, the available water resource through the rivers is about 1869 billion cubic meters. Accounting to uneven distribution of rain over the country each year, water resources available for utilization, including ground water, is claimed to be about 1122 billion cubic meters. Much of this water is unsafe, because pollution degrades water quality. Water pollution severely limits the amount of water available to Indian consumers, its industry and its agriculture.
Specific rivers
The Ganges
The ghats of river Ganges are polluted.
More than 500 million people live along the Ganges River. An estimated 2,000,000 persons ritually bath daily in the river, which is considered holy by Hindus. Ganges river pollution is a major health risk.
NRGBA was established by the Central Government of India, on 20 February 2009 under Section 3(3) of the Environment Protection Act, 1986. It also declared Ganges as the “National River” of India. The chair includes the Prime Minister of India and Chief ministers of states through which the Ganges flows.
The Yamuna
The Oshiwara River is severely polluted with solid and liquid waste generated by Mumbai.
By an estimate by 2012, Delhi’s sacred Yamuna river contained 7,500 coliform bacteria per 100cc of water. A number of NGOs, pressure groups, eco-clubs, as well as citizens’ movements, have been active in their task to clean the river.
Even though India revised its National Water Policy in 2002 to encourage community participation and decentralize water management, the country’s complex bureaucracy ensures that it remains a “mere statement of intent.” Responsibility for managing water issues is fragmented among a dozen different ministries and departments without any coordination. The government bureaucracy and state-run project department has failed to solve the problem, despite having spent many years and $140 million on this project.
0 notes
Text
Selective Catalytic Reduction (SCR) Reactors For Ships – Types, Working Principle, Advantages And Disadvantages
What is NOx and where does it come from??
NOx pollution occurs when nitrogen oxides are released as a gas into the atmosphere during the high-temperature combustion of fossil fuels.
These nitrogen oxides consist mainly of two molecules, nitric oxide (NO) and nitrogen dioxide (NO2) along with a few others that occur in much lower concentrations. These molecules- Nitrous oxides are a significant greenhouse gas that plays an important role in Global Climate Change.
Nitrogen oxides form when oxygen and nitrogen from the air interact during a high-temperature combustion event. These conditions occur in Internal Combustion Engines and fossil fuel-powered electricity plants.
Environmental and Health Concerns
NOx gases play an important role in the formation of smog. When exposed to the UV rays in sunlight, NOx molecules break apart and form ozone (O3). The problem is made worse by the presence of volatile organic compounds (VOC) in the atmosphere, which also interacts with NOx to form dangerous molecules. Ozone at the ground level is a serious pollutant, unlike the protective ozone layer much higher up in the stratosphere.
In the presence of rain, nitrogen oxides form nitric acid, contributing to the problem of acid rain.
Nitrogen oxides, nitric acid, and ozone can all readily enter the lungs, where they create serious damage to delicate lung tissue. Even short-term exposure can irritate the lungs of healthy people.
For those with medical conditions like asthma, just a short time spent breathing these pollutants have proved to be fatal. This air pollution can lead to respiratory diseases such as emphysema and bronchitis. NOx pollution can also worsen asthma and heart disease and is associated with elevated risks of premature death.
The International Shipping Industry is facing an increasingly tight regulatory environment, especially in terms of limits imposed upon emissions to air. And with the entry into force of the International Marine Organization’s (IMO) NOx Tier III limits on January 1st 2016, a part of MARPOL Annex VI, the regulations governing marine emissions became significantly tougher and stringent to follow.
As per MARPOL Annex VI, Regulation 13- Nitrogen Oxides (NOx), the Nitrogen oxides from diesel engines on board are to be controlled as follows
The NOx emission limits are set for diesel engines depending on the engine maximum operating speed (rpm), as shown in the above table, Tier I and Tier II limits are global, while the Tier III standards apply only in NOx Emission Control Areas.
There are two exceptions – engines used solely for emergencies and engines on ships operating solely within the waters of the state in which they are flagged. The latter exception only applies if these engines are subject to an alternative NOx control measure.
Emission Control Areas must comply with NOx “Tier III” emission limits which means that they must emit 80% less nitrous oxides than a “Tier I” complaint engine. Under these regulations, ships which are keel-laid after January 1st 2016 and operating in the United States/Canadian Emission Control Areas(ECAs) must comply with the new emission limits. These emission limits are applicable for engines with an output power of more than 130kW installed on ships with more than 5,000GT
Tier III standards are expected to require dedicated NOx emission control technologies. These mainly consist of two options.
1) Using Liquified Natural Gas (LNG) as a fuel in engines, using lean burn technology. e.g.- Win GD Engines-Winterthur Gas & Diesel Ltd. which burn LNG in their combustion chambers using a Low-Pressure LNG gas injection system for the reduction in NOx emissions.
2) Use of abatement technology such as various forms of water induction into the combustion process (with fuel, scavenging air(in-take air humidification), or in-cylinder), exhaust gas recirculation, or selective catalytic reduction.
This article throws light upon Selective Catalytic Reduction Reactors on Ships, it’s types, their basic working principle, components, its benefits, advantages and disadvantages.
Basic Working Principle
Selective Catalytic Reduction is a means of converting nitrous oxides in the exhaust with the help of a catalyst into diatomic nitrogen and water.
A reductant Anhydrous Ammonia (NH3), Aqueous Ammonia (Ammonium Hydroxide) or Urea (Carbamide) solution is added to a stream of exhaust gas and is adsorbed onto a catalyst. Carbon Dioxide (CO2) is a reaction product when urea is used as the reductant.
The chemical equation for the reaction using either anhydrous aqueous ammonia for the process is
4NO + 4NH3 + O2 = 4N2 + 6H2O 2NO2 + 4NH3 + O2 = 3N2 + 6H2O NO + NO2 + 2NH3 = 2N2 + 3H2O
The reaction for urea instead of anhydrous or aqueous ammonia is 4NO + 2(NH2)2CO + O2 = 4N2 + 4H2O + 2CO2 (in presence of catalyst)
Selective Catalytic Reduction
This exhaust gas after-treatment technology has a NOx abatement capability Of more than 80%. The SCR concept involves injecting a Urea-Water solution into the exhaust gas stream in combination with a special catalyst unit.
The SCR is considered as an additional and independent exhaust treatment system and as such does not interfere with the basic engine design or combustion process.
The process diagram below gives a better understanding of the SCR system wherein the urea interacts with nitrous oxides present in the incoming exhaust gas, in the presence of a catalyst, converting it into free nitrogen and water vapour.
The Maritime Environmental Protection Committee (MEPC) At The IMO has published guidelines for the certification of selective catalytic reduction (SCR) systems, referred to the “SCR Guideline”, namely IMO Resolution MEPC.198(62).
According to their configurations, SCRs can Be Classified into 2 Types- They can be either installed between The Exhaust Gas Manifold & The Turbocharger or between The Turbocharger and The Exhaust Gas Boiler.
1) High-Pressure SCR
In the High-Pressure SCR, the reactor is placed before the turbocharger. A sufficient exhaust
gas temperature is to be maintained between 300 to 400 deg Celsius, which might be challenging when the engine is running at low loads and manoeuvring.
Therefore, for two-stroke engines, the most likely location of the SCR unit is before the turbocharger in order to expand the active range of SCR operation. This has little to no effect on the engine combustion process.
It is possible to run high-pressure SCRs on Heavy Fuel Oil.
2) Low-Pressure SCR
In Low-Pressure SCRs, the reactor is placed after the turbine. Pre-heating of the exhaust gas stream may be necessary in order to achieve a sufficient temperature at the reactor inlet for the catalytic reaction. Some power generation may be needed for preheating.
Components of an SCR
Dosing Unit
The dosing unit consists of a compact external dosing system having a urea-water solution tank. The tank size depends upon how often the vessel enters NOx Tier III areas and how often the SCR is put in use. Urea Tank capacities range from 4 to 10 cub metres/MW for larger engines.
The area for marine use is usually dissolved in water having a concentration of 32%-40%. Urea is a non-toxic odourless solution considered safe to transport and store at ambient temperature & pressure. However special caution is required in winter temperatures in order to avoid crystallization.
The dosing handling system provides the reducing agent (urea solution) based on the dosing demand signal provided by the SCR and Engine control and monitoring system.
Vaporizer/ Mixing Unit
The urea from the dosing system is metered and injected into the vaporizer or mixing unit. The injected reducing agent (urea) will vaporise and mix with the incoming exhaust gas.
The mixing unit is in line with the exhaust manifold of the engine and its pipes are designed & constructed after complex flow calculations & intensive testing, to ensure a good mixture of the urea solution & hot exhaust gases. The mixing unit is usually 2 to 6 meters long and 500mm in diameter, however, size may vary as per Engine size.
Injection tubes from the dosing unit penetrate the vaporizer from the bottom, the top of the vaporizer is equipped with an electronic enclosure having a NOx measurement sensor to monitor nitrous oxides in the exhaust gas and Backpressure sensor.
SCR Reactor Chamber
This is where the conversion of NOx in exhaust gas into nitrogen and water takes place in the presence of catalyst material. The SCR reactor contains cassettes of the catalyst substrate material. The substrate elements work in limited temperatures, if exhaust gas temperature is too high, the elements get destroyed.
If the temperature is too low, SCR efficiency is reduced. Catalyst element contains Vanadium Pentoxide (V2O5) which helps the reaction process of converting the urea and exhaust gas into nitrogen and water vapour. The SCR reactor volume is usually 1.5-3 cub metres/MW installed power.
Fuel Oil Quality and SCR technology
The sulphur content in fuel oil and consequent SO2 concentration in the exhaust gas is a critical parameter which has to be observed while operating SCR systems. Urea temperature is to be controlled according to sulphur content in fuel.
A high sulphur content in presence of a low exhaust gas temperature (in case of manoeuvring) will require a higher temperature of urea solution to be injected as a condensation of exhaust gas could result in corrosion and catalyst substrate damage. A lesser content of sulphur in fuel will allow a lesser temperature of urea solution to be injected.
Condensation of water vapour in the presence of sulphur in the exhaust gas during low load operations can cause the formation of solid ammonium bisulphate. Thus, the exhaust inlet temperature is to be kept high enough to avoid condensation of ammonium bisulphate onto catalyst substrate elements.
Condensation would severely affect NOx reduction performance and cause clogging, increasing backpressure due to soot formation in the reactor.
Soot Blowing Unit
To prevent contamination of the reactor elements, a soot blowing system is installed. Soot blowing is done using compressed air of 7 bar.
SCR Control Sensor Unit
NOx sensors measure the NOx concentration before the SCR reactor and the turbocharger. The reactor chamber also contains outlet NOx sensors and outlet temperature sensors.
Venting System
The venting system vents the SCR reactor when the SCR is bypassed (i.e. when the engine is running in Tier-II mode) to avoid exhaust gas accumulation and soot formation in the reactor. The reactor is vented with Fresh Air during Tier II operation.
The Reactor Sealing Valve is used to seal the reactor during venting when the SCR is not in use.
Reactor Throttling Valve is located at the outlet of the reactor.
Reactor Bypass Valve is used to bypass the reactor for NOx Tier II operation or failure of the SCR.
The Cylinder Bypass Valve can be used while the engine runs at partial loads to bypass scavenge air towards the turbocharger to increase exhaust gas temperature.
The Cut-In Cut-Out settings of Auxiliary blowers are set slightly differently in case of engines fitted with SCRs. While cutting-in of auxiliary blowers at the time of reducing load, a slight delay is introduced so as to avoid a sudden drop in exhaust temperature.
Similarly, while cutting-out the auxiliary blowers, when engine load increases, the exhaust temperature tends to increase suddenly, to prevent this- the Cylinder Bypass Valve (CBV) opens first to gradually increase temperature, then the blowers cut out and the CBV later closes as per engine load.
Pros & Cons Of The SCR
To assure continuous NOx removal and avoid clogging, special considerations have to be observed with regard to exhaust temperatures.
Pros
● Tier III NOx compliance is achievable. ● Very efficient NOx removal for most engine loads (60-90%) ● SCRs have a widely growing reference base with over 300 installations
Cons
● Rather investment intensive ● Limited NOx removal at low engine loads ● In comparison to other solutions for NOx Tier III emissions, the use of urea requires the installation of urea tanks which may have to be replenished often (a costly affair) ● Can result in excess consumption of fuel (about 1%) ● Additional cost of Urea in ECA areas.
Maintenance
● Replacing NOx sensors- sensor life is approx. 2000hours ● Replacing the substrate catalyst elements in the SCR reactor (Lifetime-approx. 1000hours) The lifetime of the catalyst elements strongly depends upon the sulphur content of the fuel. For NOx Tier III compliance, NOx efficiency is to verified once a year. If NOx efficiency reduction < 70% all catalyst elements must be renewed as per manufacturer’s instructions. ● The Soot Blower consists of a compressed air bottle, soot blower diaphragm valves and pressure switches. All pressure hoses are to be checked and diaphragm valves to be maintained. ● The Dosing Unit consists of a urea solution tank, liquid filters, dosing pumps, nozzle assemblies, flowmeters, valves and pressure switches. Weekly maintenance includes cleaning of filters, monthly includes inspection of injectors. Proper inspection and working of all individual components are to be checked every 6 months.
Benefits For The Ship Owner/Operator
Installing NOx Tier III-compliant technology has been beneficial beyond just achieving compliance with emissions regulations. Demonstrating a company’s commitment to ensuring sustainable operations has become increasingly important. Some additional advantages include direct financial benefits, as major ports offer substantial discounts in harbour fees. One frequently used performance indicator for the environmental impact of shipping is the Environmental Shipping Index (ESI), which is used by major ports to calculate harbour fees. Installing Tier III-compliant technology, instead of Tier II-compliant technology, adds approximately five points on the ESI scale.
For example, the following reductions in harbour fees for operating on Tier III are given by these ports:
Los Angeles: $2,500 per call (ESI higher than 50) Hamburg: €1,500 per call (ESI higher than 50) Rotterdam: 20% reduction for Tier III Antwerp: 10% ESI higher than 31
Image credit: Cat Marine
Disclaimer: The authors’ views expressed in this article do not necessarily reflect the views of Marine Insight. Data and charts, if used, in the article have been sourced from available information and have not been authenticated by any statutory authority. The author and Marine Insight do not claim it to be accurate nor accept any responsibility for the same. The views constitute only the opinions and do not constitute any guidelines or recommendation on any course of action to be followed by the reader.
The article or images cannot be reproduced, copied, shared or used in any form without the permission of the author and Marine Insight.
Report an Error
from Storage Containers https://www.marineinsight.com/tech/selective-catalytic-reduction-scr-reactors-for-ships-types-working-principle-advantages-and-disadvantages/ via http://www.rssmix.com/
0 notes
Text
Hydrazine dosing device
JY metering pump dosing device product features:
The whole hydrazine dosing device is composed of solution tank, mixer, metering pump, electrical control cabinet, valve, pipeline, etc. It can be controlled manually or automatically on the spot: automatic control is based on the frequency conversion device to receive hydrazine or oxygen meter Of the signal. Function introduction of automatic control system: Under the state of automatic control, the system automatically adjusts the speed of the metering pump according to the signal of the hydrazine meter or oxygen meter, processed by PLC, host computer or DCS, the metering pump flow adjustment range is 0100, the speed control of the inverter The input signal is a standard 420mA signal, and the feedback signal is also a 420mA signal. The status and fault signals of all metering pumps and mixers can be remotely transmitted to the DCS or host computer as needed. The DCS or host computer can remotely start and stop the metering pump as required. In addition to manual preparation, the preparation of hydrazine can also be automatically prepared: the system completes the automatic dispensing process according to the control of the dosage of the hydrazine delivery pump. This device is a control system composed of basic units such as PLC controller, Chinese man-machine dialogue interface and follow-up proportional controller composed of single-chip microcomputer. It has high automation, stable performance, reliable work, intuitive Chinese man-machine interface and menu The operation is simple and clear.
The flocculant automatic dissolving and dosing device is composed of the following parts
water supply system
Dry powder dosing system
Liquid medicine dosing system
Dry powder premixing system
Integrated three-slot combination box
Mixing system
Automatic control and detection system
?Device features
Highly automated, the system is controlled by PLC and uses Chinese man-machine dialogue interface. Operate and display the running status of each unit on the interface, with an intuitive man-machine interface, simple and convenient operation.
The system is stable and reliable. When there is a fault, the related equipment will automatically stop as required, and the sound and light alarm will be issued at the same time.
The double-spiral feeder is used to feed the powder, and the speed is closed-loop controlled to ensure that the feeding is uniform, dispersed, and accurate within 1.
Under the specified production volume and continuous liquid injection conditions, the maturation time of the prepared solution is guaranteed to be more than 1 hour. Its preparation concentration is 0.050.5.
Using stainless steel material, good corrosion resistance and beautiful appearance.
The three-slot overflow solution operating system is adopted to make the equipment simple in structure, small in size and easy to install. Suitable for dissolving and continuously adding medicament
?Process flow
The agitator adopts a specially designed multi-stage paddle structure.
The premixer accelerates the hydration of the powder, and the water flow state can be adjusted.
The dry powder dosage will automatically follow the change of water flow to keep the concentration constant.
Optional., Vacuum feeder
?
JY metering pump dosing device uses materials:
Metering pump head: SUS304, SUS316 Ambient temperature: 30 90 Metering valve: SUS304, SUS316 Motor: Two-phase, three-phase standard motor or explosion-proof motor Valve ball: Silicon dioxide, SUS304, SUS316, ceramic, zirconia KW 1.5KW seal: PTFE protection grade: IP55
Application scope of JY metering pump dosing device:
With system design as the main body, it overcomes the shortcomings of poor systemicity of traditional products with a single device or component as the main design body.
Absorb the essence of domestic and foreign dosing technology, with a new design concept to make the process more optimized.
Modular design of product structure, integrated combination, can easily expand capabilities and functions.
According to different water quality and different requirements of users, flexible configuration scheme.
The equipment and components of various brands, different materials and various grades at home and abroad can be selected by users.
Choose manual, automatic and other control methods to achieve different control requirements
The main purpose of JY metering pump dosing device:
Furnace water treatment system, circulating cooling system, raw water pretreatment and wastewater treatment system in thermal power generation industry;
Furnace water treatment system, circulating cooling system, chemical additive dosing system, wastewater treatment system in the petroleum and chemical industries;
Municipal water supply and sewage treatment system;
Liquid dosing system for other related industries.
The JY metering pump dosing device has the characteristics of no leakage and high pressure, and is widely used in the transportation of flammable, explosive, volatile, strong corrosion, radioactive, highly toxic, suspension and expensive liquid media; to achieve high pressure liquid purpose.
Technical structure of JY metering pump dosing device:
?
Metering pump accessories list
Discharge tube 2 meters Suction tube 1.5 meters Injection valve Bottom valve filter
Optional accessories: back pressure valve
JY type metering pump dosing device performance parameters:
System diagram
Technical Parameters
Kind
Flow rate (L / h)
Pressure (Mpa)
Hydrazine deoxygenation dosing device
Two tanks and two pumps
1.0
1.5
2.0
Plunger
Mechanical diaphragm
Hydraulic diaphragm
30
40
50
60
80
100
200
16
twenty four
40
63
16
20
twenty four
Manual
Automatic
Two tanks and three pumps
Ammonia adding device for adjusting PH value
Two tanks and two pumps
Two tanks and three pumps
Phosphate dosing device
Two tanks and two pumps
Two tanks and three pumps
Coagulant (coagulant) dosing device
Two tanks and two pumps
1.0
1.5
2.0
3.0
Plunger
Mechanical diaphragm
Hydraulic diaphragm
40
60
90
160
330
06
10
14
Manual
Automatic
Two tanks and three pumps
Corrosion inhibitor (scale inhibitor) dosing device
Two tanks and two pumps
Two tanks and three pumps
Acid adjustment (alkali addition) device for adjusting PH value
Two tanks and two pumps
Two tanks and three pumps
Ferrous sulfate coating dosing device
One tank and one pump
Centrifugal
0.831 m3 / h
Around 0.4
Manual
Two tanks and two pumps
Rotor pump
Device use
Quantity sets
Operating parameters
Media Name
Medium temperature
Preparation concentration
Solution density / m3
Dosing points
Dosing flow per point l / h
Dosing point back pressure Mpa
Throwing distance m
status of use
Intermittent
continuous
Structural parameters
Dissolving box volume m3
Material
Stainless steel carbon steel carbon steel lining rubber non-metallic other
Metering pump type
Mechanical diaphragm hydraulic diaphragm plunger pump
amount
Metering pump flow l / h
Metering pump pressure Mpa
control method
Manual adjustment Automatic adjustment
Pipe material
Stainless steel Carbon steel UPVC other
Technical curve of JZR plunger metering pump:
JZR plunger metering pump connection method:
Installation dimensions of JZR plunger metering pump:
Matters needing attention for JY metering pump dosing device:
It is suitable for normal water at normal temperature until it has strong corrosion, volatile, crystallized, flammable, explosive, highly toxic, foul odor, strong corrosive, large specific gravity, medium viscosity, radioactive or other valuable liquids. At the same time, it can also transport suspended liquids. For some to prevent accidents caused by the mixing of the medium and hydraulic oil after the diaphragm ruptures, for flammable, explosive, highly toxic and precious liquids, a double diaphragm metering pump with diaphragm rupture alarm device can be selected.
Precautions
1 Before using the metering pump, add 220 worm gear oil or 50 gear oil to the oil mark in the gear box, and add LHM3246 hydraulic oil to the oil mark in the hydraulic oil sump.
?
JY type metering pump dosing device installation and commissioning:
Preparation before pump operation
1 After installation and acceptance, the bolts and nuts at each connection should not be loosened. The new pump should be cleaned of anti-corrosion or dirt on the pump. Kerosene should be used for cleaning and scraped with a shovel.
2Inject appropriate amount of LHM46 hydraulic oil into the hydraulic oil tank, the oil volume is subject to the horizontal center line of the oil standard.
3 Inject appropriate amount of LCKE460 worm gear oil (SH / T0094199) or LCKE460 industrial closed gear oil (GB59031995) into the gearbox. The oil volume shall be subject to the horizontal center line of the oil standard.
4 Use a manual coupling or motor to move the plunger back and forth several times throughout the stroke without any jamming.
5 Connect the power supply according to each brand of the motor, start the motor, and check whether the rotation direction of the motor is consistent with the steering sign. (Note: At this time, the inlet and outlet of the pipeline should be completely open, otherwise the consequences will be at your own risk)
Metering pump flow regulation
1 According to the flow requirements of the process flow, turn the adjustment meter or dial to the corresponding indication value. The regulation of flow should be from small to large. If you need to adjust from large to small (flow meter), observe that the pointer moves with the rotation of the adjustment handwheel. When the pointer reaches the required position and exceeds a few grids, then move the pointer to the direction of large flow to adjust the required value. After adjustment, the handwheel must be locked.
2 Flow adjustment can be carried out during parking or operation. After adjustment, the flow of the pump takes about 12 minutes to stabilize. The larger the flow, the longer the stability time.
3 The adjustment of overload and overload has been set according to the rated discharge pressure before leaving the factory. If the actual discharge pressure is different from the rated discharge pressure, adjust according to the table (1).
Table (1) Unit: MPa
Wide opening pressure%
0.15P
0.3P
0.2P
0.1P
Note: P is the rated pressure. For safety, it is recommended that non-professionals should not be arbitrarily transferred.
4 After completing the above work, confirm that it is correct before it can be put into operation.
Before shutting down, the outlet throttle should be fully opened and the discharge pressure should be reduced to normal pressure. Only then can the power supply be cut off and stopped.
Preparation before operation
1 The metering pump should be installed on a strong special foundation, and the anchor bolts should be tightened after correction. If it is installed on a concrete foundation, it should be more than 100150 mm above the ground.
2 The connecting pipeline should be equipped with measuring instruments. (For example, pressure gauge, etc.)
3 Filtration device should be installed at the end of the suction line. (User-supplied can also be ordered like our company)
4Electrical control equipment should be installed in the pump work place as much as possible according to the use situation (under the premise of not involving safety), electrical control equipment should be equipped with control and protection devices corresponding to the transport medium to ensure safety.
5 The suction and discharge pipe diameter should be no less than the inlet and outlet pipe diameter, and the suction pipe should be as short as possible. If the suction line is too long or there are too many elbow joints, NPSHa accounting should be performed. To meet the requirements of the net positive suction head.
6 The discharge pipeline should be equipped with safety relief or safety relief relief and back pressure relief (user-supplied). If it is necessary to reduce the outlet pressure pulse, a voltage stabilizing device can be installed on the nearest pump discharge path. (User-supplied)
7 Other technical requirements for the installation of the pump shall comply with the relevant provisions in the 'Code for Installation and Acceptance of Mechanical Equipment' TJ231 (5) 78.
Pump installation
1 Install the pump on a sturdy foundation higher than 200,300 dishes in the medicine tank, and install it horizontally.
2 The pump should be installed on the ground with a height of 300,500 mm above the ground, and the pump should be corrected in a horizontal state; the installation of the multi-pump should pay attention to the calibration of the interphase coupling of the pump, and the deviation of the concentricity should not exceed 0.15 mm. ), The steel coupling should be within 0.05 mm;
3 There should be no sharp elbows on the suction and discharge pipelines that are not greater than 90), and the components that bend and increase resistance in the pipeline should be minimized.
4 Do not install the pump and medicine tank in direct sunlight.
5 The medicine tank should be supplemented with liquid medicine and maintained for inspection. Please place it in a more spacious place.
6 Do not install in places where moisture or corrosive gas is easily touched.
7 The ambient temperature of the pump installation is 20 + 40, and the altitude is below 1000M.
Pipeline installation
1 The suction pipe diameter should not be less than the suction wide diameter of the pump, and the length of the suction pipe should be shortened as much as possible, generally 23 meters; if the length must be increased, it should be appropriately lengthened, but the length should not exceed 5 meters The time of liquid inhalation is relatively extended).
2 The pipes connected to the suction and discharge wide flanges cannot be forcibly combined to increase the load of the pump, and the weight of the pipes and wide doors must not be borne by the pump and the suction and discharge. After the pipeline is installed, the pipe fittings should be supported and fixed.
The increase of pipeline flux by special liquid
1 In order to ensure the safe operation of the pump and the safety of the pipeline system, the safety pipeline should be installed on the discharge pipe. If it is necessary to reduce the pulse of the liquid being conveyed, a buffer can be installed near the discharge pipe of the pump.
2 For the transportation of suspensions and media that are prone to precipitation, wide doors and tees should be added near the suction and discharge of the pump so that the cylinder can be <a href="https://www.sdaoqun.net/article/detail/heavy-duty-caster-running-wheels.html"></a> flushed without disassembling the pipeline when the pump operation is stopped.
Installation specifications (according to the installation drawing)
Other technical requirements for the installation of the pump shall comply with the relevant regulations for the installation of the TJ231 (V) 78 pump in the “Code for Construction and Acceptance of Mechanical Equipment Installation Work”.
instructions
JY metering pump dosing device failure maintenance:
Pump maintenance and use
maintain
1 The specified oil level should be maintained in the transmission box and hydraulic oil tank, not too much or too little.
2 After the lubricating oil and hydraulic oil are clean and free of impurities, replace them according to the cycle in the following table (2).
Table 2)
Lubricating oil starts 36 months, replaces lubricating <a href="https://www.sdaoqun.net/product/blow-molded-series">get more</a> oil every 3 months, after 6 months, replaces hydraulic oil every 6 months, starts 25 months, replaces hydraulic oil every 2 months, replaces every 5 months, every 5 months
3 After 20003000 hours of operation of the pump, the internal parts should be disassembled for inspection, repair and replacement of wearing parts.
4 If the pump is stopped for a long time, the medium in the liquid cylinder should be drained and the surface cleaned. The exposed processing surface needs anti-rust oil. The pump should be placed in a dry place during storage.
Disassembly and assembly
Disassembly of the hydraulic end of the hydraulic diaphragm metering pump. Unscrew the hexagon socket screw plug at the bottom of the hydraulic oil tank, release the hydraulic oil in the tank, turn the motor coupling, move the piston forward to the dead center, <a href="https://www.sdaoqun.net/product/detail/extruded-and-enhanced-products-03.html"></a> remove the piston from the crosshead, and remove the hydraulic end from the machine base Remove the upper part and then remove the hydraulic end parts in the following order:
1 Remove the deflation overload and compensation wide assembly;
2 Remove the inlet and outlet pressure flange, and then take out the wide sleeve, wide core, spring) wide seat;
3 Unscrew the fixing nut on the cylinder head, remove the cylinder head, and take the hydraulic diaphragm type in sequence;
4 Remove the bolts of the cylinder block and the fuel tank, remove the cylinder block, plunger, and liquid cylinder, and then remove the limit stop assembly.
5 Remove the coupling nut between the hydraulic oil tank and the transmission box, and remove the oil tank.
The assembly is carried out in the following order:
Installed on the transmission box in the reverse order of the disassembly order of the hydraulic end of the hydraulic diaphragm metering pump. Adjust the oil filling width and the bleed overload width. Before installing the bleed overload width, add enough hydraulic oil from the bleed overload wide oil hole to discharge the gas in the hydraulic chamber. After assembling, rotate the coupling, it should run freely, without jamming.
0 notes
Text
Simple ways to test Water Quality
With the help of modern chemistry we can detect thousands of chemicals in water even at extremely low concentrations. The ever-growing list of tests that are available can feel overwhelming and the vast majority of methods require state-of-the art lab facilities. Fortunately, we don’t need to test for everything! A much smaller and more practical set of tests can provide a good sense of Chemical Water Quality for monitoring purposes. The good news is that there are low-tech versions of these tests for situations when budgets are limited.
Test formats
Typical low-tech, portable, field test methods for chemical water quality monitoring fall into three categories:
Test strips – These are small, single-use strips that change colour to indicate the concentration of a specific chemical. Depending on the particular test, the user “activates” the paper or plastic strip by dipping it into the water sample and swishing it around, or by holding the strip in a stream of water. After waiting for a short time, the user compares the test strip colour with a color chart to read the concentration of the chemical. These Water Sample Test Kits are extremely simple, but they are less accurate than other methods, especially if users don’t follow the instructions.
Colour disk kits – Colour Disk Test Kits are available for a wide range of chemical tests. In a typical set-up, the user adds a powder packet or a few drops of a liquid reagent to a water sample in a reusable plastic tube. The user then places the sample tube in a small plastic viewing box. This viewing box contains a plastic disk with a colour gradient printed on it. The user rotates the colour disk to find the part that best matches the colour of the sample, and then reads the concentration of the chemical from the disk. Colour disk kits typically have multiple steps and often include prescribed wait times, so they’re a little more complicated and costly, but generally more accurate.
Hand-held digital instruments – Lightweight and portable Digital Meters, Colorimeters and Photometers are available for water testing. They provide the most accurate results of these three testing methods, but they are also more expensive and delicate than the previous options. These instruments require batteries and calibration. While Digital Instruments are helpful to field technicians and are an essential part of any continuous or remote monitoring network, they are unlikely to be suitable for “citizen science” or crowd sourced water quality testing.
Chemical Water Quality Parameters:
Having identified various test formats, the next question is: What do we test for? UNICEF recommends prioritizing fluoride, arsenic and nitrate for chemical monitoring. In areas where the earth is naturally rich in minerals that contain fluorine and arsenic levels in well water can be high enough that chronic exposure is dangerous to human health.
How can we test for these elements?
Fluoride: At least one colour disk test kit is available for fluoride. However, Portable Digital Colorimeters are often preferred because of concerns over accuracy.
Arsenic: Portable field testing options for arsenic are limited; this contaminant is best measured in a laboratory. Commercially available test kits do exist, but they are relatively complex and require several steps. Although the arsenic concentrations “measured” with these test kits may be inaccurate, the kits do detect arsenic in nearly all samples greater than 100 micrograms per litre (μg/L), as well as in most samples in the 50-99 μg /L range. UNICEF has therefore recommended reporting arsenic monitoring results from these portable tests as “present” or “absent” using a reference concentration of 50 μg /L—the drinking water standard in many countries that are affected by natural arsenic contamination.
Nitrate: Both test strips and colour disk test kits are available for Nitrate Testing. Nitrate can also be measured with a digital meter. High levels of nutrients are associated with agricultural pollution from fertilizers (nitrogen and phosphorous) and animal waste (nitrogen). Latrines, sewage, landfills and industrial pollution can also contribute nitrogen. Monitoring for nitrate is a simple way to assess the impacts of agricultural and human waste on water quality.
Resources permitting, UNICEF suggests adding three more chemical parameters to monitoring programs: the naturally-occurring metals iron and manganese, and the overall total dissolved solids (TDS). All three can cause taste and odour problems that might motivate consumers to seek out more appealing – and potentially unsafe – water sources.
Iron and Manganese: Both test strips and colour disk tests are available for these two metals which may also be measured using portable, digital instruments. Field testing with digital equipment is considered reliable for iron and manganese.
TDS: TDS includes a mixture of inorganic salts, mostly sodium, chloride, potassium, calcium, and magnesium. Rather than testing the particular components, TDS is monitored by measuring the conductivity of the water with a digital meter known as TDS Meter. There is no test strip or colour disk kit that can be used here, although at least one conductivity meter interfaces with a smartphone.
In chlorinated distribution systems, it is important to monitor two more chemical parameters: pH and chlorine residual.
pH: pH test strips and colour disk tests are widely available. More expensive, higher-tech options include electrode-based pH meters. pH is a measure of hydrogen ion activity, which means that it tells us how acidic or basic the water is. pH is not a pollutant, but it is a chemical master variable. It affects the behaviour of other chemical constituents, including the effectiveness of residual chlorine against microbial contamination. Sudden changes in pH can also reveal treatment plant failures or pollution events in natural water bodies (for example, illegal industrial discharge).
Chlorine: There are many easy ways to test residual chlorine including test strips, colour disks and even kits designed for testing swimming pools. Portable digital meters also exist that can provide reliable & quantitative measurements.
Depending on local conditions and on the focus of a water quality monitoring project, more chemical tests can be added. One might test for alkalinity or hardness (including calcium, magnesium, etc.; field kits are available), chloride (an indicator of road salt or seawater intrusion; test kits exist), dissolved oxygen,[2] organic carbon levels (BOD, COD, TOC), agrochemicals (specific pesticides or fertilizers), or mining/industrial contaminants (e.g., polychlorinated biphenyls, cyanide). Finally, heavy metals like lead, mercury, copper, chromium, etc. are often of local interest.
However, the vast majority of these additional tests are best performed in a laboratory given current technologies. Hydromo being one of such expertise in conducting the Water Tests, it also provides the entire Water Solutions for Homes and Businesses.
0 notes
Text
Iris Publishers
Iris Publishers
Welcome to IRIS Publishers! Here you can read from our vast archive of scientific knowledge that we’ve collected from all over the world.
Thursday, June 13, 2019
Iris Publishers- Open access Journal of Oceanography & Marine Biology | Influence of Types and Doses of Fertilizer on the Physicochemical Characteristics of Water, Composition and Structure of Zooplankton Populations in Ponds
Authored by Algrient Nana Towa
In order to improve fish productivity, an essay on the evaluation of the comparative effect of chicken and pig manure on the composition and structure of
Zooplankton
was conducted at the Application and Research farm from the University of Dschang (LN: 5º44’-5 °36 and LE: 10º06’-9 ° 85’). For this purpose, 15 ponds (5.7 x 5.7 x 1m), three doses of 0 (control); 800 and 1000kg/ha of chicken manure as well as pig dung were used. At each of the randomly selected ponds was administered one of the fertilizer doses every 7 days. Each of the doses and type of fertilizer representing a treatment was repeated three times. The physicochemical characteristics of the water and the
Zooplankton
population were measured every 14 days. With respect to the physicochemical characteristics of water, the values of nitrites (7.92 ± 0.05mg/l), nitrates (8.03 ± 0.24mg/l), phosphates (4.68 ± 0, 05mg/l) were significantly (p <0.05) higher in ponds fertilized at 1000kg/ha pig dung, the lowest being obtained in the control treatment. For
Zooplankton
, the highest specific (64.51% of total species), generics (87.87% of total genera) and families (82.35% of total)
Zooplankton
ic abundances were obtained in ponds fertilized at the rate of 1000kg/ha of chicken manure. The species of rotifers were the most diverse survived those of cladocerans whatever the type or the dose of fertilizer. The use of the 1000kg/ha dose of chicken droppings makes sense for a large production of
Zooplankton
diversity.
In Africa and more specifically in Cameroon, the role of aquaculture in the economy remains marginal. In fact, aquaculture accounts for less than 0.1% of total fish inputs, despite the water surface area covering nearly 3.5 million hectares, spread over 4 major river basins MINEPIA [1]. It should be noted that commercial fish farming is 90% from fertilized ponds. However, one of the constraints of development of pond production is attributable to the fertilization technique associated with the mastery of the operation of the interspecific, intraspecific biodiversity of the living prey of the pond ecosystem.
Fertilization is an activity that makes it possible to optimize fish production by improving the physicochemical parameters of water and the densities of
Zooplankton
organisms [2], which are the living prey for the most fishes.
Zooplankton
is by its specific diversity and nutritional value the best fish about the plasticity of the diet of fish species. Several studies have been carried out on the fertilization of ponds from animal waste [3-5], at different doses. But none of this work has focused on studying the diversity of
Zooplankton
populations.
The objective of this work is to contribute to the improvement of the production of
Zooplankton
intended for larval feeding through a better fertilization. More specifically, the aim is to evaluate the effect of pig manure doses on:
• The physicochemical characteristics of water
• The richness and distribution of
Zooplankton
populations
Area and period of the study
The trial was conducted from May 15 to November 15, 2016 at the Dschang University Applied Research Farm (FAR) (LN: 5° 44’-5° 36’ and LE: 10° 06’ -9° 85’, altitude: 1392-1396m) in the Sudano- Guinean agro-ecological zone characterized by a short dry season (mid-November to mid-March) and a long rainy season (mid-March to mid-March) November). The annual rainfall varies between 1500 and 2000mm and the temperatures oscillate between 14 °C (July- August) and 25 °C (February).
Fertilizer
The slurry and droppings used in hens came from a pork farm and egg layer layers fed a feed (feed). A sample of each fertilizer was taken to determine the total dry matter, nitrogen and phosphorus concentration. The average values of the characteristics of the droppings were: dry matter (80.2±3.33%), total nitrogen (2±0.14%) and total phosphorus (1.5±0.06%) and those of pig slurry: dry matter (30.5±2.21%), total nitrogen (33±1.11%), total phosphorus (9±2.30%).
Exp erimental ponds
The test was carried out in 15 identical by-pass ponds (5.7 x 5.7 x 1m and a water exchange rate of 0.05 l/s). The 15 ponds were fed in water from an artificial lake located 100m away. A 1.5mm mesh net was attached to the feed pipe of each pond to prevent intrusion of fish from the artificial lake.
Conduct of the test and data collection
At each randomly selected pond, one of the 0kg/ha (control), 800; 1000kg/ha for both chicken manure and pig dung (weighed with a 0.1g precision electronic balance) corresponding to T
0, F
800
, F
1000
, L
800
and L
1000
, respectively. Fertilization was done every 7 days on the surface of each pond according to Lacroix (2004).
Sampling
The determination of the physicochemical characteristics of the water was carried out parallel to that of
Zooplankton
every 14 days between 6 and 8 o’clock in the morning according to Agadjihouédé et al. [6] Dakwen et al. [7]. The sampling of
Zooplankton
was carried out at twenty different points of the water column of each pond using a calibrated polyethylene container of 1 liter capacity, ie a total volume of 20 liters/pond filtered by means of a plankton screen of 40μm mesh. A volume of 350ml
Zooplankton
concentrate was recovered, fixed by addition of 5% formalin (¼ volume of the concentrated sample) and stored in the plastic bottles for quantitative and qualitative analyzes.
Determination of the physicochemical characteristics of water
a. The transparency of the dry disk, temperature, pH, dissolved oxygen and electrical conductivity were directly measured in the field with the help of a weighed dry disk and attached to a graduated rope, Thermo-Conductivity meter, Thermo pH-meter, pH meter, thermo-oximeter and thermo-conductivity meter brand HANNA.
b. Total nitrite, nitrate and phosphate measurements were carried out by spectrophotometry (HACH DR/2000 spectrophotometer) using Alpha techniques Greenberg [8].
Determination of Zooplanktonic characteristics
Two types of analysis were carried out namely: qualitative analysis and quantitative analysis.
To read more about this article...
Oceanography & Marine biology
Please follow the URL to access more information about this article
https://irispublishers.com/aomb/fulltext/influence-of-types-and-doses-of-fertilizer-on-the-physicochemical-characteristics-of-water.ID.000516.php
To read more about our journals....
Iris Publishers
at
June 13, 2019
No comments:
Email This
BlogThis!
Share to Twitter
Share to Facebook
Share to Pinterest
Labels:
iris publishers
,
Iris Publishers LLC
,
journal of oceanography and marine biology
,
Journal of oceanography and marine research
,
journal of physical oceanography
Iris Publishers- Open access journal of Oceanography & Marine Biology | Mangrove and Its Health Benefits
Authored by Delianis Pringgenies
Mangrove, which in Indonesian is known as mangrove, is one of the many vegetations found on bays of shallow coasts, estuaries, deltas and protected coastal areas that are still affected by tides in Indonesia. This plant has striking features, with large and woody supporting roots, shoots in the form of tapered foliage leaves, and fruits that germinate and root while still in the tree. Mangroves provide various benefits, the wood is used as fuel and charcoal, tannins from the bark are used as dye, and are sometimes used as medicine in cases of hematuria (presence of blood in urine). Mangroves are also planted along the ponds to protect the embankments [1].
Based on the type of compound, waste can be categorized into two parts, namely organic waste and inorganic waste. Organic waste is a group of waste which consists of the constituent parts of plants and animals. Organic waste can be used to increase soil fertility, because organic matter can be decomposed by microbes which then become beneficial nutrients for plant growth. Today mangrove fruit can be used as food, for example mangrove crackers, mangrove syrup, mangrove pudding, and mangrove klepon, as presented in Figure 1. Furthermore, organic waste such as mangrove waste can be processed into natural fabric dye.
Pigment of mangrove waste for hypoallergenic natural batik dye
The use of mangrove waste as a natural dye pigment of batik is more environmentally friendly because the waste produced will not damage the land and sea environment and is more easily decomposed. In addition, the mangrove waste used is obtained directly from nature by taking fallen bark and leaves, at no cost at all because the principle used is to recycle organic “waste”. Research found 6 species of mangroves that can be used as natural fabric dyes, namely
Sorenasia alba, Rhizophora sp, Avecenia sp, Ceriops decandra, Lunicera sp
each leaf and stem are used, except
C. decandra,
which is only used for leaves. The mangrove species
Lunicera sp
only uses lotus fixer solution on leaves and stems, while combined fixers are also used as treatment for processing
Lunicera sp
leaves [2].
The color produced by each type of mangrove
Retention of fabric dyes from mangrove waste has not been widely studied and applied to batik cloth. The mangrove species of Rizhophora mucronata, which can produce light brown to maroon colors, has the potential to be applied as a unique fabric dye from this type of mangrove. The color content can be an asset that when utilized properly will be economically beneficial for the surrounding community. Phytochemical test results on the leaves and bark of mangrove trees showed a strong positive result on the tannin group with dark green color, while the mangrove bark showed positive results on the quinone with red orange color. The application of mangrove-based fabric dye pigments on batik is a different method than other batik production methods, because the basic ingredients of the dye are made from natural ingredients that are hypoallergenic [3].
Observation with a UV-VIS spectrophotometer on pigments in leaves and mangrove bark waste shows the presence of chlorophyll a and b. Mangrove leaves show the presence of chlorophyll a pigment, while mangrove bark waste has chlorophyll b pigment content [4]. The quality of fabric dyes used in batik cloth depends on the type of pigment used.
https://irispublisher.blogspot.com/
1 note
·
View note
Text
Juniper Publishers- Open Access Journal of Environmental Sciences & Natural Resources
Evaluation and Characterization of Tannery Waste Water in Each Process at Batu and Modjo Tannery, Ethiopia
Authored by Abdrie Seid Hassen
Abstract
The leather industry is suffering from the negative impact generated by the pollution it causes to the environment. Nearly 70% of the pollution loads of BOD, COD, and Total Dissolved Solids (TDS) are generated from soaking, liming, deliming, pickling and tanning and retanning processes. There is an enormous pressure from the various pollution control bodies to regulate and minimize the amount of pollution generated from the leather processing. The need for use of alternative to chemical methods to combat pollution problem have become necessary to protect the industry and to comply with the environmental norms. In the present study, effluent samples were collected from Batu and Modjoa tannery in Ethiopia. The effluent samples were collected from all stages of processing viz., soaking, liming, deliming, pickling, Chrome tanning and Retaining. The physicochemical parameters of the tannery effluent viz. pH, alkalinity, acidity, biochemical oxygen demand (BOD5), chemical oxygen demand (COD), total solids (TS), total dissolved solids (TDS), suspended solids (SS), chlorides and sulfides were determined. All the parameters included in this study are found to be higher than the prescribed discharge limits for tannery industries. The investigation of the tannery wastewater from different tanning processes gave a number of conclusions. The results indicate that the wastewaters from the tanneries do not satisfy the legal ranges of selected parameters discharge to inland water and to sewer.
Keywords: Alkalinity; Acidity; COD; BOD; Tannery Waste Water; Sulfides; Chlorides
Abbreviations: COD: Chemical Oxygen Demand; TS: Total Solids; TDS: Total Dissolved Solids; SS: Suspended Solids; BOD: Biochemical Oxygen Demand
Introduction
The tanning process aims to transform skins in stable and imputrescible products namely leather. There are four major groups of sub-processes required to make finished leather: beam house operation, tanyard processes, retanning and finishing [1-3]. However for each end product, the tanning process is different and the kind and amount of waste produced may vary in a wide range [2,4]. Traditionally most of tannery industries process all kind of leathers, thus starting from dehairing to retanning processes. However, in some cases only pre-pickled leather is processed with a retanning process. Acids, alkalis, chromium salts, tannins, solvents, sulfides, dyes, auxiliaries, and many others compounds which are used in the transformation of raw or semi-pickled skins into commercial goods, are not completely fixed by skins and remain in the effluent. For instance, the present commercial chrome tanning method gives rise to only about 50-70% chromium uptake [5]. During retanning procedures, synthetic tannins (Syntan), oilsand resins are added to form softer leather at varying doses [6]. One of the refractory groups of chemicals in tannery effluents derives mainly from tannins [7]. Syntans are characterized by complex chemical structures, because they are composed of an extended set of chemical such as phenol, naphthalene formaldehyde and melamine-based Syntans, and acrylic resins [8-10].
Among Syntans, the ones based on sulfonatednaphthalene's and their formaldehyde condensates play a primary role, for volumes and quantity used in leather tanning industry. The oils cover the greater COD equivalents compared to the resins and syntans. The BOD5/COD ratio of syntans was also lower than other compounds. A brief description about the wastes generated from a tannery and their impact on the environment would be appropriate to understand the problem associated with it. The beam house operations soaking, liming and deliming lead to discharge of high amount of sulfides, lime, and ammonium m salts, chlorides, sulphate, and protein in the effluent. Consequently, the wastewater is characterized with high amount of BOD and COD. Soak liquor contains, suspended solids, dirt, dung, blood adhering to hides and skins, and chloride etc. lime liquors are highly alkaline. This stream contains suspended solids, dissolved lime, sodium sulfide, high ammonia cal nitrogen and organic matter. Unhearing and fleshing effluent contains fatty fleshing matter in suspension. The spent deliming liquors carry significant BOD load. The spent bate liquors on account of presence of soluble skin proteins and ammonium salts containing high organic matter. Pickle liquors are acidic and contain high amount of salt.
The spent chrome liquors contain high concentration of chrome compounds and neutral salts. The wastewater from neutralization, retanning, dyeing and fat liquoring sections contribute little pollution load [11]. Solvents and this leads to the emission of volatile organic compounds (VOC) [12]. An average of 30-35m3 of wastewater is produced per ton of raw hide. However, wastewater production varies in wide range (10-100 m3 per ton hide) depending on the raw material, the finishing product and the production processes [2]. Organic pollutants (proteic and lipidic components) are originated from skins (it is calculated that the raw skin has 30% loss of organic material during the working cycle) or they are introduced during processes. The objectives of this study to evaluate thephysico-chemical properties of polluted water discharged from tannery, viz., pH, chloride, sulfide BOD5, COD, alka linity, T.S.S, TDS and evaluate of tannery wastewater in the different tanning processes viz. soaking, liming and unhairing, deliming and bating, pickling, tanning and retanning processes [13,14].
Materials and Methods
Materials
For the present study effluent samples were collected from tanneries in Batu and Modjo, Ethiopia. The effluent samples were collected from all stages of tanning processing viz., soaking, liming, deliming, pickling, Chrome tanning and Retanning. The effluent was collected in polythene containers of two litres capacity and were brought to the laboratory with due care and was stored at 4oC for further analysis. Chemicals used for the analysis of spent liquor were analytical grade reagents. The physical and chemical characteristics of tannery effluents parameters viz. pH, total alkalinity, COD, BOD5, total solids (TS), total dissolved (TDS); total suspended solids (TSS), chlorides, sulfide sand chromium were analyzed as per standard procedures [15].
Methods
Determination of pH: The pH is determined by measurement of the electro motive force (emf) of a cell comprising of an indicator electrode (an electrode responsive to hydrogen ions such as glass electrode) immersed in the test solution and a reference electrode (usually a calomel electrode). Contact is achieved by means of a liquid junction, which forms a part of the reference electrode. The emf of this cell is measured with pH meter.
Determination of total alkalinity: The alkalinity of sample can be determined by titrating the sample with sulphuric acid or hydrochloric acid of known value of pH, volume and concentrations. Based on stoichiometry of the reaction and number of moles of sulphuric acid or hydrochloric acid needed to reach the end point, the concentration of alkalinity in sample is calculated. A known volume of the sample (50 ml) is taken in a beaker and a pH probe was immersed in the sample. HCl or H2SO4 acid (0.1NHCl in 1000 ml distilled water) added drop by drop until the pH of the sample reached 3.7. The volume of the acid added was noted [15].
Calculation: Alkalinity as mg/l of CaCO3= (50000x N of HClx ml acid titrated value) /volume of sample taken.
Determination of chemical oxygen demand (COD): The chemical oxygen demand of an effluent means the quantity of oxygen, in milligram, required to oxidize or stabilize the oxidizable chemicals present in one litre of effluent under specific condition. 2.5 ml of the sample was taken in tube, 1.5 ml of 0.25 NK2Cr2O7(potassium dichromate), spatula of mercuric sulphate HgSO4 and 3.5 ml of COD acid were added and kept in COD reactor for 2hrs at 150oC. After cooling the sample titrated against FAS (standard ferrous ammonium sulfate 0.1N) and used ferrion as indicator. The end point is reddish brown color. In the blank tube 2.5 ml of distilled water was taken and then follow the same procedure in the sample [15].
Calculation: COD (mg/l) = (blank value-titrated value) xN of FASx8000/ volume of sample
8000 ill equivalent wt of O2x1000ml
Determination of biochemical oxygen demand (BOD): Biochemical oxygen demand (BOD) of an effluent is the milligram of oxygen required to biologically stabilize one litre of that effluent (by bio-degradation of organic compounds with the help of micro-organisms) in 5 days at 4oC.If the BOD value of an effluent is high, is high, then that effluent contains too much of bio-degradable organic compounds and so will pollute the receiving water highly.
A. Procedure
a) Take 5 litres of distilled water, aerated for 3.5 hours, added nutrients 1 ml nutrient for 1 litre aerated distilled water (FeCl, CaCl2, PO4, MgSO4, domestic water), aeration for 30 minutes.
b) BOD bottle (300 ml), add sample, fill the bottle with aerated water, put the lid (avoid air bubbles), keeping BOD incubator at 20oC for 5 days, after 5 days take the bottle and add 2 ml MnSO4, 2 ml alkaliazide iodide and 2 ml conc. H2SO4. Shake the bottle well (yellow colour) take 200 ml sample add starch solution as indicator (purple colour) titrated with 0.025 N sodium thiosulphateend point colour change from purple to colorless. In blank filled the bottle with aerated water without the sample and follow the procedure [15].
c) Calculation
BOD5= (blankvaluetitratedvalue) x300/volume of sample
Determination of Total solid: The term solid refers to the matter either filtrable or non-filtrable that remains as residue upon evaporation and subsequent drying at a defined temperature. Residue left after the evaporation and subsequent drying in oven at specific temperature 103-105°C o f a known volume of sample are total solids. Total solids include Total suspected solids (TSS) and Total dissolved solids (TDS).
A. Procedure
Dry weight of empty dish or crucible (initial weight), add 50 ml sample, keep it in water bath until dry, keep it in oven (103 to 105oC) for at least 1 hour, desiccators, and take final weight of dish [15].
a) Calculation
Total solid (mg/l) = (final weight-initial weight) x1000x1000 / volume of sample
Determination of total dissolved solid
A. Procedure
Dry weight of empty dish or crucible (initial weight) take sample and filter with What man No.1, add 50 ml filtrate sample, keep it in water bath until dry, keep it in oven (103 to 105oC) for at least 1 hour, desiccators, take final weight of dish [15].
a) Calculation
Total dissolved solid (mg/l) = (final weight-initial weight) x1000x1000 / volume of sample
Determination of total suspended solid: The difference between the total solids and total dissolved solids are suspended solids.
TSS = TS-TDS
Determination of chloride: Chloride is determined in a natural or slightly alkaline solution by titration with standard silver nitrate, using potassium chromate as an indicator. Silver chloride is quantitatively precipitated before red silver chromate is formed.
A. Procedure: Take sample (10 ml to 50 ml), add 2 ml of hydrogen peroxide (H2O2), add 2 ml K2CrO4 (potassium chromate indicator), titrate with silver nitrate (0.0141 N), end point formation of reddish yellow colour (yellow to orange). In blank trial take distilled water instead of sample and follow the same procedure above [15].
a) Calculation
Chloride (mg/l) = (A-B)xN. of silver nitratex35.45x1000/ volume of sample
A = ml titration for sample
B = ml titration for blank
N = normality of AgNO3
Determination of sulfide: The sulfides in the solution are oxidized with an excess of a standard iodine solution and the excess back titrated with a standard thiosulfate solution.
A. Procedure: Take sample (10ml) in conical flask, add 5 ml zinc acetate (5%), filter through filter paper, take the filter paper and put it in the same conical flask, add 100 ml distilled water. then add 20 ml, iodine solution and 4 ml 6N HCl, add 2 drops of starch as indicator (purple colour will form), titrate against sodium thiosulphate (0.025N), end point the colour change from blue colour to colorless. In the blank test take 100 ml distilled water instead of sample and follow the same procedure above for the sample [15].
a) Calculation:
Sulfide (mg/l) =(BV-TV) x N. thiox400/Volume of sample xN. Ioden
BV= blank value
TV= titrated value
Results and Discussion
Characteristics of tannery waste water
Wastewater of each tannery process consists of pollution of varying pH values. Similarly, a large variation exists in every parameter BOD, COD, Chloride, Sulphate, etc. Discharge of these chemicals into wastewater is hazardous for the environment. Analysis of physical and chemical characteristics of the tannery wastewater collected from different tanning processes viz. soaking, liming and unhairing, deliming and bating pickling, chrome tanning and retaining are listed in (Tables 1 & 2) respectively.
Determination of pH
The pH values of both tanneries are in the range 3.2512.64. Which was very higher value compare to limit set by EPA (6.0-9)? The extreme pH of wastewater is generally not acceptable, as lower pH cause problems to survival of aquatic life. It also interferes with the optimum operation of wastewater treatment facilities. Water with high or low pH is not suitable for irrigation. At low pH most of the metals become soluble in water and therefore could be hazardous in the environment. At high pH most of the metals become insoluble and accumulate in the sludge and sediments. The toxicity of heavy metals also gets enhanced at particular pH [6].
Determination of Biochemical Oxygen
a) Demand (BOD): BOD is measure of the content of organic substances in the waste water which are biologically degradable with consumption of oxygen. Usually indicated as 5-day Biochemical oxygen demand (BOD). This is the amount of oxygen in milligrams per litre (O2) (mg/l) that consumed by microorganisms in5 days at 20oC for oxidation of the biologically degradable substances contained in the water. The results of present study revealed that BOD level from different tanning processes viz. soaking, liming and unhairing, deliming and bating pickling, chrome tanning and retanning is given in (Figures 1-4) indicating high organic load surpassed legal limit set by EPA (200 mg/l). The presence of organic matter will promote anaerobic action leading to the accumulation of toxic compounds in the water bodies.
Determination of Chemical Oxygen Demand (COD)
Chemical oxygen demand (COD) is quantity of oxygen expressed in milligram consumed by the oxidisable matter contained in one litre of the sample. The test is performed by vigorous oxidation with chemicals and back-titrating the chemical consumed for oxidation. COD is system of measuring the content of organic impurities with oxidizing agents. The results of present study revealed that COD level from different tanning processes viz. soaking, liming and unhairing, deliming and bating pickling, chrome tanning and retanning is given in (Figures 5 & 6) exceeds the permissible COD level EPA (500mg/l).This indicates that the effluent is unsuitable for the existence of the aquatic organisms, due to the reduction in the dissolved
Determination of Total Solids (TS)
The results of present study revealed that TS level from different tanning processes viz. soaking, liming and unhairing,deliming and bating pickling, chrome tanning and retanning is given in (Figures 7 & 8) exceeds the permissible TS level of 110 mg/L. These solid impurities cause turbidity in the receiving streams. The composition of solids present in tannery effluent mainly depends upon the nature and quality of hides and skins processed in the tannery.
Determination of Total Suspended Solids (TSS)
The results of present study revealed that TSS level from different tanning processes viz. soaking, liming and unhairing, deliming and bating pickling, chrome tanning and retanning is given in (Figure 6) and it exceed the permissible TSS level of (20200) mg/ L. These suspended impurities cause turbidity in the receiving streams. The composition of solids present in tannery effluent mainly depends upon the nature and quality of hides and skins processed in the tannery. High level of total suspended solids present in the tannery effluent could be attributed to their accumulation during the processing of finished leather. Presence of total suspended solids in water leads to turbidity resulting in poor photosynthetic activity in the aquatic system [16-18] and clogging of gills and respiratory surfaces of fishes [19].
Determination of Chloride
The results of present study revealed that chloride level from soaking and pickling, are 19250 mg/ l, 23500 mg/l respectively (Table 2) and the levels exceed the permissible chloride level of 1000 mg/L of effluent discharge into inland surface waters. High levels of chlorides in the tannery effluent could be attributed to the soaking and pickling processes. The chloride content in water sample gives an idea of the salinity of water sample.
Determination of Sulfide
Sulfides are particularly objectionable because hydrogen sulfide will be liberated if they are exposed to a low pH environmental, and if they are discharged into stream containing iron, black precipitates will be formed. Sulfides may be toxic to stream organisms or to organisms employed in biological treatment systems. The results of present study revealed that sulfide level from liming and unhairing process is given in Table 2 and it exceed the permissible sulfide level of 2 mg/ L. of effluent discharge into inland surface waters [13].
Determination of Total Alkalinity
Alkalinity of water is its acid neutralizing capacity. It is the sum of all the bases. The alkalinity of natural water is due to the salt of carbonates, bicarbonates, borates silicates and phosphates along with hydroxyl ions indeliming & bating process is given in (Tables 1 & 2). The Free State. However the major portion the alkalinity is due to hydroxides, carbonates and bicarbonates. The results of present study revealed that alkalinity level from soaking, liming and unhairing, and deliming process are given in (Figures 9-16).
Determination of hexavalent chromium
Cr is one of the most important pollutants released from the tanning industries in the effluent. According to Saritha and Meikandaan (2013) chrome tanning processes originates toxic metals and regular treatment systems are not eligible for the elimination of it. The wastewater generated by tanneries is the major source of Chromium pollution. The chromium (Cr) is well-known to be toxic to living organisms due to their bioaccumulation and non-biodegradable properties. In Tables 1 & 2 Maximum Cr concentrations was show at both tanneriesrespectively.This indicates that the concentration is above permissible limit of EPA (0.1mg/l).
All value except pH are stated Mg/l
Conclusion
The processing of hides and skins into leather is carried out in an aqueous medium m and hence the discharged water from pits, drums or paddles containing several soluble and insoluble constitutes the effluents from the tannery. In the present study, investigation of the tannery wastewater from different tanning processes gave a number of conclusions. Results of the analysis showed that the tannery wastewater from different tanning processes viz., soaking, liming and unhairing, declaiming and bating, pickling, chrome tanning and retanning is highly With a disagreeable pH, alkalinity, acidity, total solids, total dissolved solids, suspended solid, chemical oxygen demand, biochemical oxygen demand, chlorides and sulfides. The results of the analysis indicate that the wastewaters from different units of the tannery do not satisfy the legal ranges of selected parameters.
For more articles in Juniper Publishers | Open Access Journal of Environmental Sciences & Natural Resources please click on: https://juniperpublishers.com/ijesnr/index.php
#Juniper Publishers#Juniper Publishers PubMed Indexed Journals#Hydrology#Molecular Ecology#Environmental Chemistry#Ecological psychology
0 notes