#Aircraft Cooling Turbines
Explore tagged Tumblr posts
Text
Among car enthusiasts of a certain persuasion, there exists a yearning that cannot be satisfied by regular automakers. The hoi polloi are perfectly happy with their normal, pedestrian automobiles. The elites opt for penis-shaped zoom-zooms that cost more than a house. Those of us in the middle, who have an eternal love for going very fast for very little money, are abandoned. And as we all know, being in the self-described middle is the same thing as being morally correct at all times.
Back in the 50s, people really wanted to go fast for no money. It's what started the whole world of hot rodding. And they had lots of good options, thanks to the government suddenly having a ton of warplanes that weren't currently engaged in a war. Cool plane superchargers, engines, belly tanks – anything that weird nerds could get their hands on – got shoved into cars in the quest to go fast. And automakers were run by those weird nerds, back then.
Sure, a lot of them were putatively "run" by big-dollar, humanity-crushing fascists, but the real fun, in the research and development divisions? That was happening with the same hot rodder nutjobs who would go down to the beach after work and do skids in a car mostly made out of a bathtub, until the cops showed up. And in the late 50s, what those very same nutjobs were excited about were turbines.
See, turbine engines were getting exciting then. It was the jet age. Clean, efficient, very loud, screaming jets. Not inefficient, old clangy pistons with their oiled bearings and pitiful triple-digit horsepower. No, it was time to go fast, and so they dutifully started cramming turbines into street cars. Did it make sense? No. Were any of these cars even close to being practical? Absolutely not. Was it completely bad-ass? Yes.
Unfortunately, it was at this time that the nascent development of "management science" began to metastasize in the Western world. A lot of bosses came down and saw a screaming, shrieking demon burning nineteen litres of gasoline per minute, bolted loosely into a Ford Deluxe Coupe, and they asked: how many cupholders this got? Not having a sufficient answer that didn't start with "fuck you," these same bosses then began dismantling the apparatus that held a promise of a glorious, high-pitched-whining future of thirty-thousand-rpm engines.
There is still hope. For instance, things containing turbines get crashed all the time. Once the FAA is done looking at them to figure out what they fucked up (usually: aircraft contacted the earth too soon,) they don't really pay too much attention to what happens to the carcass. If you're quick, you can cut through the fence and get ahold of your very own helicopter turbine with which to start the project. And what do you use to slice through that fence and retrieve your futurist prize? A thirty-thousand-rpm battery-operated cut-off wheel, of course. Thanks, weird nerds.
740 notes
·
View notes
Note
hey are you the pilot guy?? can you tell us some cool things about airplanes?
I’m the pilot guy, and here’s some lame things about airplanes instead:
According to FAR Part 25, Section 795, any plane which seats more than 60 people or has a MTOW exceeding 100,000 lbs must have a “least bomb risk location”.
Outer space is a Class Echo airspace. If you can manage to get past FL600 without entering the great big Class Alpha in the sky, you only need a student cert to fly in space.
Pilots are considered a part of an aircraft’s structural weight during the design process.
Sometimes a ram air turbine looks like the plane is holding a little toy windmill, and this makes me happy.
Weeeee
23 notes
·
View notes
Text
An idea to generate electricity (that requires electricity)
I got this idea from Turbines(Turbos) and Jet Engines.
In airplanes; Engines not only make the Jet go vroom; they supply superheated air into the aircraft. This air goes through various methods for cooling and is used to pressurize the cabin.
The Jet Engines *also* drive a power shaft connected to an alternator(generator) that powers the aircraft's electronic systems.
But here's the part I'm interested in; the Super Heated air turned into Super Cooled air.
This works through several mechanisms; Ram Air being a primary provider of cooling to the super heated air directly off the engine.
But that Super Heated air then drives a Turbine, and gets super cooled through a regenerative process. That process is connected between Liquid Coolant systems and the Super Cooled air.
The Excessively hot air air gets super cooled at the turbine and through liquid cool systems; and then comes out of the other side of the turbo *super cooled*.
Not all air systems care about the liquid coolant, as cabin air (sometimes termed crew air) may be its own system that is focused on the air conditioning inside the cabin (so that the people inside the Jet are super affected if something else goes haywire. Like an Overclocked GPU or something).
This process which takes in the super heated air and turns into super cooled air; can also be used to drive air generated current.
But likely isn't used because of the oil/grease needed to maintain fans of that high caliber *all the time*.
This is very similar to refrigeration units; the main difference is that there isn't a high pressure of air to work with.
Which may make this idea moot, as flying super sonic speeds isn't in the realm of possibility for your average person.
But then I started considering Tornados and Hurricanes. The eye of each of these is *super heated*, and they move like air does in any other facet, high to low.
How do these supernatural phenomenon work? Id theorize that it's the sun that gets one started. Creating a super heated zone, that pushes strong winds outward in a cyclonic fashion.
Which means there needs to be enough *heat* to generate it *and* keep it contained. With the supplemental above-storm pressure feeding in air like a jet engine or a vacuum.
Oh dear God... I'm on par with evil genius thesis' now.
The question I'm trying to answer now; how can we identify and measure micro-high-pressure systems; and then use them for regenerative energy purposes?
Because trying to control the weather is a NATO violation...
7 notes
·
View notes
Text

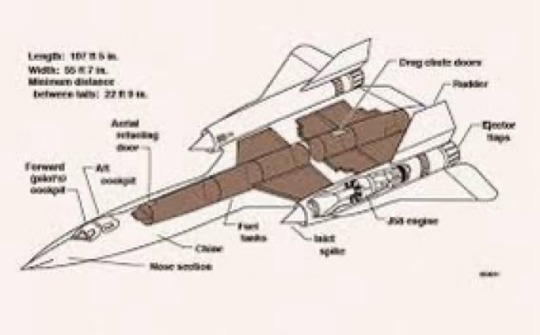

Where is the doghouse?
Not only JP-7: the SR-71 could use JP-4 and JP-5 as emergency fuels but they both limited the Blackbird's top speed to Mach 1.5..
The SR-71 Blackbird was the first aircraft to use its own fuel for hydraulic fluid. It was called the fuel hydraulic system.
The legendary SR-71 Blackbird Mach 3+ spy plane was powered by two 34,000 lbf (151,240 N) thrust-class J58 afterburning turbojet engines. Each engine contained a nine-stage compressor driven by a two-stage turbine. The main burner used an eight-can combustor and the afterburner is fully modulating. The primary nozzle area was variable. Above Mach 2.2, some of the airflow was bled from the fourth stage of the compressor and dumped into the augmentor inlet through six bleed-bypass tubes, circumventing the core of the engine and transitioning the propulsive cycle from a pure turbojet to a turbo-ramjet.
The SR-71 was the first aircraft to use its own fuel for hydraulic fluid. It was called the fuel hydraulic system.
An engine-driven pump provided 1800 psi of recirculating fuel to accurate various engine components and then returned it back to the aircraft fuel system to be burned. Fuel was used in the actuators to control the afterburner nozzles, which maintain the proper exhaust gas temperature and control the thrust output. The fuel was also used in the engine actuators to shift the two-position inlet guide veins from their axial position to the cambered position and back again. This was just another of the many first-ever inventions of the-SR-71.
The J58 engine was hydromechanically controlled and burned a special low volatility jet fuel mixture known as JP-7.
Emergency fuels could be used in the SR-71 if the crew was low on fuel and had to use ANY tanker (as already explained the Blackbird relied on KC-135Q tankers [that could simultaneously carry a maximum of 74,490lb of JP-7 and 110,000lb of JP-4 for their own engines] but the SR-71 could also be refueled by standard Stratotankers in the event KC-135Qs were not available or if the Blackbird crew had to deal with an emergency situation) they could find to avoid the loss of the aircraft. The emergency fuels were JP-4 or JP-5 but they limited the Blackbird top speed to Mach 1.5. There were six main fuselage tanks. All 80,285 pounds of JP-7 fuel were carried in six main fuselage tanks. The tanks numbered one through six moved forward to aft (back) tank 6B It could hold 7,020 pounds of gravity-fed fuel and two tanks sumps. This was also called the “doghouse” and was located in the extreme back portion of the fuselage.
Fuel was the lifeblood of this fastest-manned airplane in the world. I found the following in a declassified CIA brief.
There it would first be used as hydraulic fluid at 600 F to control the afterburner exit flaps before being fed into the burner cans of the powerplant and the afterburner itself.
Cooling the cockpit and crew turned out to be seven times as difficult as on the X-15 research airplane which flew as much as twice as fast as the SR-71 but only for a few minutes per flight. The wheels and tires of the landing gear had to be protected from the heat by burying them in the fuselage fuel tanks for radiation cooling to save the rubber and other systems attached thereto. Special attention had to be given to the crew escape system to allow safe ejection from the aircraft over a speed and altitude range of zero miles per hour at sea level to Mach numbers up to 4.0 at over 100,000 feet.
Written by Linda Sheffield Sanitized Copy Approved for Release 2011/09/27: CIA-RDP90B00170R000100080001-5 -4- The problems of taking, pictures through
Be sure to check out Linda Sheffield Miller (Col Richard (Butch) Sheffield’s daughter, Col. Sheffield was an SR-71 Reconnaissance Systems Officer) Facebook Pages Habubrats SR-71 and Born into the Wilde Blue Yonder for awesome Blackbird’s photos and stories.
Written by Habubrat
@Habubrats71 via X
83 notes
·
View notes
Note
Hello Great Wizard of Quirks! I recently found this anime, Azur Lane and Kantai Collection, so I’m asking you to make a Quirk out of those so called “warship/aircraft carrier physiology”, I know you already did your Quirk “Tank” and that Turbine-like Quirk of yours too but please hear me out! The design and power of that were so cool so please grant me this!
Okay, so, I know that you were expecting something like the user being a living war ship. I am fine doing that. You are free to ask about that again if you want. However, I saw "Aircraft Carrier Physiology", got an idea, and really wanted to go with that. I hope you still like it.
I see it working as a Transformation type Quirk that allows the user to make a series of platforms around their arms and legs resembling the deck of an aircraft carrier. From these, the user may launch small planes that resemble jet fighters. The user can control these jets over long ranges and may use up to twenty of them at a time. These jets are equipped with machines, missiles, and torpedoes. While the attacks are not powerful, the sheer speed and number of the jets can make them a real problem for anyone they are sent against. The planes come equipped with various lock-on, radar technology, and cameras, feeding them information via the decks on their body. This gives the user a good idea of who or what may be around their jets. This gives the user a good mix of options, able to dive bomb their enemies with their squadron of fighters. They can harass their enemies with the jets, use the jets as spies, carefully blast apart debris for rescue work, use the sensors to trace their opponents, or just use them to do some cool flight shows. Though the planes are small and pretty easy to destroy. The planes will need to return to the user in order to restock and repair themselves. Damaging the landing pads can prevent the user from launching their fighters. A possible name for the Quirk could be "Aircraft".
14 notes
·
View notes
Text
In relation to that anonymous ask:
I spent the last half of September studying for, then training for my Airline Transport Pilot checkride. The ATP is the highest pilot certification level the FAA provides and it’s required for flying large, turbine aircraft for hire…. Commercial airlines. My 2200 total flights hours, 730 combat hours, 430 instrument hours etc mean nothing without this certificate.
It’s also the only certificate that the military doesn’t have equivalency for, so no matter what I train to as a pilot in the military, I have to go get this certification on my own… hence this training course.
I passed the checkride and the associated ground evaluation (1 on 1 interview-style exam). The evaluator pilot was super cool, also prior Air Force. I goofed one of the three landings… apparently not enough to jeopardize the checkride, but I was still relieved when he shook my hand after engine shutdown. I was worried for a moment.
I have a soft employment offer from a major US carrier. This certification was the last item standing in my way, so I consider this a big win, but that’s also where I’ve been for the last few weeks.
12 notes
·
View notes
Note
Hii, can I request a Soobin + The Avengers (first movie) + Fluff and Suggestive.
Btw, congrats for your achievement ❤️❤️❤️
NOW SHOWING...
pairing: choi soobin x fem!reader
genre: fluff, suggestive
wc: 1.7k
details + warnings: mdni, soobin + fem!reader don't represent any particular characters (though some references are made), a lil makeout sesh 😵💫
note: thank you!! ♡♡
you can't sleep.
not that you ever slept much in the first place, but since joining s.h.i.e.l.d. in their efforts of recovering the tesseract and locating kai's sister who happens to be in possession of it — even worse, kai's sister who aims to take over the planet with it — you've all but given up on proper rest. this mission is far bigger than yourself, far bigger than anyone on your ragtag team of (what you guess you could call) superheroes, for that matter. you may be a trained assassin and a master of close-range combat, but you cannot deny the traces of fear that gnaw at your nerves.
sitting up in your bed, you rub at your eyes until they feel raw. your room is too warm to be comfortable, and all you can think about is the sheer amount of unpredictability that plagues this situation. you're used to set dates, locations, people to eliminate…this situation entails none of those things. no one knows where bahiyyih is, no one knows where the tesseract is, and no one has a goddamn clue what she's planning. your chest tightens for a moment. breathe. breathe.
you strip the covers from your body once your eyes blink back into focus, swinging your legs over the side of the bed. the floor is cool beneath the soles of your feet as you move to stand; the sensation brings you back to yourself, bars your mind from spiraling any further. you need to get out of this room right now. with haste, you slip a pair of slides on and make your way out of your room. the helicarrier is eerily quiet as you walk down the sprawling hallways, the only sounds reaching your ears being the wind rushing by and the rumbling of the aircraft carrier's turbines. dim lights illuminate your path and create ominous shadows along the walls; you pay them little mind — you'd know if someone was following you. your shoes make little noise against the walkway, your featherlight steps a result of your intensive training. your heartbeat slowly returns to normal.
eventually, the hallway leads to a large room, a lookout platform lying opposite of where you stand. the windows jut out from the floor at an angle, supported by thick, floor-to-ceiling metal beams. you stride over to the platform, staring out at the clouds that rush by; far below, the ocean has opened its gaping maw, the darkness endless yet oddly comforting.
you're unsure how long you stand there, vision blurred as you stare out at the nothingness, before your ears perk up at the sound of footsteps sounding from behind. they are trying to be quiet, you can tell, but they lack finesse. it causes you to immediately raise your guard. the footsteps slowly grow closer, and your muscles tense in response. they're right behind you now, a hand reaching out to grab your shoulder—
in a flash, you have them twisted the other way, one arm pinned between their shoulder blades and the other clasped in your other hand's unrelenting grip. your foot moves on autopilot as it kicks the back of their knee. their knees collide with the platform with a dull thump!
“it’s me! it’s me!” the person whispers frantically, their unmistakably male tone bordering on panicked. it’s then that you recognize the platinum blond head of hair, the long limbs, a voice you know all too well…
soobin.
“oh my god, i’m so sorry,” you whisper back while you release his limbs. he stands, rubbing at where your fingers pressed a little too hard into his flesh with a soft “ow.” you level him with a raised eyebrow and downturned lips. “c’mon, it’s your fault. you shouldn’t have tried to sneak up on me.”
“yeah, i guess you’re right,” soobin sighs, rotating his shoulder with a pained wince. “jesus, remind me not to get on your bad side.”
this draws a chuckle from you. “what’re you doing up, anyway? it’s late.”
“couldn’t sleep, so i decided to get out and patrol,” he simply replies. it’s then that you notice that he’s wearing a simplified, more casual version of his field uniform — a tight black tank top, tactical pants of a similar color and fit, and his normal pair of thick gloves adorning his hands. you realize that you’ve never seen his bare arms before, only ever through his suit. though his build is thin and lithe, you can see the defined muscles that push against the skin of his biceps. the sight causes your mouth to run dry.
you’re feeling oddly bare in your sleep attire, your arms crossing over your chest. as your eyes meet his again, you find him staring at you, questions dancing his dark eyes. he continues, “y’know, i could ask you the same.”
“same reason as you, but my first thought wasn’t to patrol,” you laugh, refusing to let your eyes wander any more. “you work too hard.”
“ah, it’s nothing— force of habit, really,” he says, heart-shaped lips curving into a sheepish grin.
sure, you might be a little attracted to soobin, but you respect him more than anything. he didn't join this team just to flaunt his homemade tech like yeonjun, he is not here out of obligation like kai — rather, he's on this team because he wants to help people. his unrelenting drive to keep the world safe and lead your team to success is admirable, even if he does butt heads with yeonjun more often than not.
after a brief bout of silence, an idea pops into your head. your eyes trail back up to meet his own. “do you want to go spar, maybe?”
his eyes widen almost imperceptibly before his bunny-like smile returns, a teasing lilt to his following words.
“as long as you promise to go easy on me.”
after a brief stop at your room so that you could change into more fight-appropriate clothing, you and soobin navigate the hallways to the on-board training center. you have only been here a few times since you arrived on the helicarrier, usually taking out your energy on one of the punching bags that line the perimeter of the mat. it's odd to know that you are about to spar with an actual person; soobin is the only one who has accepted your offer thus far.
the two of you are quick to begin after a bout of stretching. the moment you're done bumping fists in the middle of the ring, his leg swings out from behind him, aiming for your side. you roll out of the way, rising to your feet to his left.
“cheap move,” you grin, closing in while intercepting his fist. you struggle for a moment to hold his arm back; while you prize agility, he favors strength. he mirrors your expression.
“just evening out the playing field.” his foot slips behind your ankle and pushes forward, but you slip out of the precarious position with ease.
the two of you go at it for awhile, exchanging punches and kicks, wearing each other down slowly — you're more evenly matched than he gave himself credit for. what you throw, he blocks, and when he swings, you easily sidestep out of the way, his limbs cutting through empty air. your stamina seems to trump his, however, his movements growing sloppier, more holes opening up in his defense.
like a machine, you exploit these weaknesses. the next time he throws a kick, you grab his calf and twist, his body rotating in the air before he crashes to the mat. the action knocks the breath from his lungs, and you take the opportunity to straddle his hips, your hands gathering his wrists and pinning them above his head.
“caught you,” you pant, a smirk playing on your lips as you lean over him, noses brushing against each other and mere centimeters separating your lips. it doesn’t take either of you long to realize just how close you are, frozen in place as you stare at each other in silence.
it's unclear who leans in first, but your lips are suddenly enveloped by his. your grip on his wrists loosens, and he takes the opportunity to wrap large his hands around your waist, pulling you closer to him. you lean down more, deepening the kiss, allowing his tongue to slip into your mouth. you resist the urge to grind down against him, your mind growing foggy with a lack of oxygen when neither of you pull away.
eventually, you are the first to pull away, pressing him back down as he chases your lips. you're back to staring at each other now, but there's something…different in the way he looks back at you — lightly veiled need swirls in his irises. you release a breath against his cheek. his hands do not move from their position on your waist.
soobin breaks the silence, but he doesn’t meet your gaze. “is…this a bad time to admit that i kinda like you?”
though your heart pounds, you try to act as if you’re not on the verge of freaking out. “i wouldn’t say so, no,” you smile, stomach fluttering. “‘cause i kinda like you too.”
leaning up, he kisses you again, slower this time, savoring the sensation of your soft lips against his. you find yourselves smiling into each other's mouths, and you lose yourself in him, ignorant of the way his hands wander down to your hips. suddenly, he rolls you over, turning the tables on you as he pins your hands down.
“rule number one,” he whispers against your lips, pupils blown out as he towers over you. “never let your guard down.”
“and rule number two?” you tease, tone light and breathy.
one side of his lips quirks up. “hm, guess we’ll have to figure that one out together.”
he's back to kissing you soon after, sparring be damned.
3k event masterlist | masterlist
© to agustdiv1ne. do not copy, repost, steal, and/or translate.
#txt fluff#soobin fluff#txt x reader#soobin x reader#txt suggestive#soobin suggestive#txt imagines#soobin imagines#txt smut#soobin smut#txt drabbles#3k milestone celebration#agust.nsfw#💌 — soob
128 notes
·
View notes
Text
Nuclear Power Renaissance with Molten Salts - Technology Org
New Post has been published on https://thedigitalinsider.com/nuclear-power-renaissance-with-molten-salts-technology-org/
Nuclear Power Renaissance with Molten Salts - Technology Org
A science team is reinventing nuclear energy systems via molten salt technologies.
A retro wonder gleaming white in the sun, propelled by six rear-facing rotors and four jet engines affixed to the longest wings ever produced for a combat aircraft, the Convair B-36 Peacemaker looks like it flew right out of a 1950s science fiction magazine.
Frozen uranium containing fuel salt (NaF-BeF2-UF4), inside a glovebox in Raluca Scarlat’s SALT lab. Illustration by Sasha Kennedy/UC Berkeley
One of these bombers, which flew over the American Southwest from 1955 to 1957, was unique. It bore the fan-like symbol for ionizing radiation on its tail. The NB-36H prototype was designed to be powered by a molten salt nuclear reactor — a lightweight alternative to a water-cooled reactor.
Nuclear-propelled aircraft like the NB-36H were intended to fly for weeks or months without stopping, landing only when the crew ran short of food and supplies. So what happened? Why weren’t the skies filled with these fantastical aircraft?
“The problem was that nuclear-powered airplanes are absolutely crazy,” says Per F. Peterson, the William S. Floyd and Jean McCallum Floyd Chair in Nuclear Engineering. “The program was canceled, but the large thermal power to low-weight ratio in molten salt reactors is the reason that they remain interesting today.”
Because of numerous concerns, including possible radioactive contamination in the event of a crash, the idea of nuclear-powered aircraft never took off. But nuclear submarines, using water as coolant, completely replaced their combustion-powered predecessors. Civilian reactors were built on the success of submarine systems, and as a result, most nuclear reactors today are cooled with water.
Professor Per Peterson holds a single fuel pebble, which can produce enough electricity to power a Tesla Model 3 for 44,000 miles. Illustration by Adam Lau / Berkeley Engineering
While most water-cooled reactors can safely and reliably generate carbon-free electricity for decades, they do present numerous challenges in terms of upfront cost and efficiency.
Molten salt reactors, like those first designed for nuclear-powered aircraft, address many of the inherent challenges with water-cooled reactors. The high-temperature reaction of such reactors could potentially generate much more energy than water-cooled reactors, hastening efforts to phase out fossil fuels.
Now, at the Department of Nuclear Engineering, multiple researchers, including Peterson, are working to revisit and reinvent molten salt technologies, paving the way for advanced nuclear energy systems that are safer, more efficient and cost-effective — and may be a key for realizing a carbon-free future.
Smaller, safer reactors
In the basement of Etcheverry Hall, there’s a two-inch-thick steel door that looks like it might belong on a bank vault. These days, the door is mostly left open, but for two decades it was the portal between the university and the Berkeley Research Reactor, used mainly for training. In 1966, the reactor first achieved a steady-state of nuclear fission.
Fission occurs when the nucleus of an atom absorbs a neutron and breaks apart, transforming itself into lighter elements. Radioactive elements like uranium naturally release neutrons, and a nuclear reactor harnesses that process.
Concentrated radioactive elements interact with neutrons, splitting themselves apart, shooting more neutrons around and splitting more atoms. This self-sustaining chain reaction releases immense amounts of energy in the form of radiation and heat. The heat is transferred to water that propels steam turbines that generate electricity.
The reactor in Etcheverry Hall is long gone, but the gymnasium-sized room now houses experiments designed to test cooling and control systems for molten salt reactors. Peterson demonstrated one of these experiments in August. The Compact Integral Effects Test (CIET) is a 30-foot-tall steel tower packed with twisting pipes.
The apparatus uses heat transfer oil to model the circulation of molten salt coolant between a reactor core and its heat exchange system. CIET is contributing extensively to the development of passive safety systems for nuclear reactors.
After a fission reaction is shut down, such systems allow for the removal of residual heat caused by radioactive decay of fission products without any electrical power — one of the main safety features of molten salt reactors.
The first molten salt reactor tested at Oak Ridge National Laboratory in the 1950s was small enough to fit in an airplane, and the new designs being developed today are not much larger.
Conventional water-cooled reactors are comparatively immense — the energy-generating portion of the Diablo Canyon Power Plant in San Luis Obispo County occupies approximately 12 acres, and containment of feedwater is not the only reason why.
The core temperature in this type of reactor is usually kept at some 300 degrees Celsius, which requires 140 atmospheres of pressure to keep the water liquid. This need to pressurize the coolant means that the reactor must be built with robust, thick-walled materials, increasing both size and cost. Molten salts don’t require pressurization because they boil at much higher temperatures.
In conventional reactors, water coolant can boil away in an accident, potentially causing the nuclear fuel to meltdown and damage the reactor. Because the boiling point of molten salts are higher than the operational temperature of the reactor, meltdowns are extremely unlikely.
Even in the event of an accident, the molten salt would continue to remove heat without any need for electrical power to cycle the coolant — a requirement in conventional reactors.
“Molten salts, because they can’t boil away, are intrinsically appealing, which is why they’re emerging as one of the most important technologies in the field of nuclear energy,” says Peterson.
The big prize: efficiency
Assistant professor Raluca Scarlat uses a glovebox in her Etcheverry Hall lab. Illustration by Adam Lau / Berkeley Engineering
To fully grasp the potential benefits of molten salts, one has to visit the labs of the SALT Research Group. Raluca O. Scarlat, assistant professor of nuclear engineering, is the principal investigator for the group’s many molten salt studies.
Scarlat’s lab is filled with transparent gloveboxes filled with argon gas. Inside these gloveboxes, Scarlat works with many types of molten salts, including FLiBe, a mixture of beryllium and lithium fluoride. Her team aims to understand exactly how this variety of salt might be altered by exposure to a nuclear reactor core.
On the same day that Peterson demonstrated the CIET test, researchers in the SALT lab were investigating how much tritium (a byproduct of fission) beryllium fluoride could absorb.
Salts are ionic compounds, meaning that they contain elements that have lost electrons and other elements that have gained electrons, resulting in a substance that carries no net electric charge. Ionic compounds are very complex and very stable. They can absorb a large range of radioactive elements.
This changes considerations around nuclear waste, especially if the radioactive fuel is dissolved into the molten salt. Waste products could be electrochemically separated from the molten salts, reducing waste volumes and conditioning the waste for geologic disposal.
Waste might not even be the proper term for some of these byproducts, as many are useful for other applications — like tritium, which is a fuel for fusion reactors.
Salts can also absorb a lot of heat. FLiBe remains liquid between approximately 460 degrees and 1460 degrees Celsius. The higher operating temperature of molten salt coolant means more steam generation and more electricity, greatly increasing the efficiency of the reactor, and for Scarlat, efficiency is the big prize.
“If we filled the Campanile with coal and burned it to create electricity, a corresponding volume of uranium fuel would be the size of a tennis ball,” says Scarlat. “Having hope that we can decarbonize and decrease some of the geopolitical issues that come from fossil fuel exploration is very exciting.”
“Finding good compromises”
Thermal efficiency refers to the amount of useful energy produced by a system as compared with the heat put into it. A combustion engine achieves about 20% thermal efficiency. A conventional water-cooled nuclear reactor generally achieves about 32%.
According to Massimiliano Fratoni, Xenel Distinguished Associate Professor in the Department of Nuclear Engineering, a high-temperature, molten salt reactor might achieve 45% thermal efficiency.
So, with all the potential benefits of molten salt reactors, why weren’t they widely adopted years ago? According to Peter Hosemann, Professor and Ernest S. Kuh Chair in Engineering, there’s a significant challenge inherent in molten salt reactors: identifying materials that can withstand contact with the salt.
Anyone who’s driven regularly in a region with icy roads has probably seen trucks and cars with ragged holes eaten in the metal around the wheel wells. Salt spread on roads to melt ice is highly corrosive to metal. A small amount of moisture in the salt coolant of a nuclear reactor could cause similar corrosion, and when combined with extreme heat and high radiation, getting the salt’s chemistry right is even more critical.
Hosemann, a materials scientist, uses electron microscopes to magnify metal samples by about a million times. The samples have been corroded and or irradiated, and Hosemann studies how such damage alters their structures and properties. These experiments may help reactor designers estimate how much corrosion to expect every year in a molten salt reactor housing.
Hosemann says molten salt reactors present special engineering challenges because the salt coolant freezes well above room-temperatures, meaning that repairs must either be done at high temperatures, or the coolant must first be drained.
Commercially successful molten salt reactors then will have to be very reliable, and that won’t be simple. For example, molten salt reactors with liquid fuel may be appealing in terms of waste management, but they also add impurities into the salt that make it more corrosive.
Liquid fuel designs will need to be more robust to counter corrosion, resulting in higher costs, and the radioactive coolant presents further maintenance challenges.
Nuclear engineering graduate students Sasha Kennedy and Nathanael Gardner, from left, work with molten salt. Illustration by Adam Lau/Berkeley Engineering
“Good engineering is always a process of finding good compromises. Even the molten salt reactor, as beautiful as it is, has to make compromises,” says Hosemann.
Peterson thinks the compromise is in making molten salt reactors modular. He was the principal investigator on the Department of Energy-funded Integrated Research Project that conducted molten salt reactor experiments from 2012 to 2018.
His research was spun off into Kairos Power, which he co-founded with Berkeley Engineering alums Edward Blandford (Ph.D.’10 NE) and Mike Laufer (Ph.D.’13 NE), and where Peterson serves as Chief Nuclear Officer.
The U.S. Nuclear Regulatory Commission just completed a review of Kairos Power’s application for a demonstration reactor, Hermes, as a proof of concept. Peterson says that high-temperature parts of Kairos Power’s reactors would likely last for 15 to 25 years before they’d need to be replaced, and because the replacement parts will be lighter than those of conventional reactors, they’ll consume fewer resources.
“As soon as you’re forced to make these high-temperature components replaceable, you’re systematically able to improve them. You’re building improvements, replacing the old parts and testing the new ones, iteratively getting better and better,” says Peterson.
Lowering energy costs
California is committed to reaching net zero carbon emissions by 2045. It’s tempting to assume that this goal can be reached with renewables alone, but electricity demand doesn’t follow peak energy generating times for renewables.
Natural gas power surges in the evenings as renewable energy wanes. Even optimistic studies on swift renewable energy adoption in California still assume that some 10% of energy requirements won’t be achieved with renewables and storage alone.
Considering the increasing risks to infrastructure in California from wildfires and intensifying storms, it’s likely that non-renewable energy sources will still be needed to meet the state’s energy needs.
Engineers in the Department of Nuclear Engineering expect that nuclear reactors will make more sense than natural gas for future non-renewable energy needs because they produce carbon-free energy at a lower cost. In 2022, the price of natural gas in the United States fluctuated from around $2 to $9 per million BTUs.
Peterson notes that energy from nuclear fuel currently costs about 50 cents per million BTUs. If new reactors can be designed with high intrinsic safety and lower construction and operating costs, nuclear energy might be even more affordable.
Molten salt sits on a microscope stage in professor Raluca Scarlat’s lab. Illustration by Adam Lau/Berkeley Engineering
Even if molten salt reactors do not end up replacing natural gas, Hosemann says the research will still prove valuable. He points to other large-scale scientific and engineering endeavors like fusion reactors, which in 60 years of development have never been used commercially but have led to other breakthroughs.
“Do I think we’ll have fusion-generated power in our homes in the next five years? Absolutely not. But it’s still valuable because it drives development of superconductors, plasmas and our understanding of materials in extreme environments, which today get used in MRI systems and semiconductor manufacturing,” says Hosemann. “Who knows what we’ll find as we study molten salt reactors?”
Source: UC Berkeley
You can offer your link to a page which is relevant to the topic of this post.
#000#2022#aircraft#airplanes#applications#atom#atoms#boiling#bombers#Building#carbon#carbon emissions#Cars#challenge#chemistry#Chemistry & materials science news#coal#construction#contamination#control systems#cooling#corrosion#crash#designers#development#effects#efficiency#electrical power#electricity#electron
4 notes
·
View notes
Text
For most of the history of civilisation we’ve exploited a pretty small selection of metals, including copper and tin for bronze-age tools, iron for steel, and lead, gold and silver. Our repertoire has begun to diversify over the past century or so, with the widespread use of aluminium and other new metals. But in the past few decades the number of different metals we wield in our technological society has absolutely exploded. A modern smartphone contains more than 30 different elements. These include carbon and hydrogen in the plastic casing, silicon for the microchip wafers, and copper wiring and gold contacts. But there are also small amounts of a large number of other metals, each exploited for its own particular electronic properties, or for the tiny, powerful magnets used in the speaker and vibration motor. This means that if you own a smartphone, you have in your pocket a substantial fraction of all the stable elements of the periodic table. And it’s not just modern electronics that demand a huge diversity of different metals. So too do the high-performance alloys used in the turbines of a power station or aircraft jet engine, or the reaction-accelerating catalysts that we use in industrial chemistry for refining oil, producing plastics or synthesising modern medicinal drugs. Yet most of us have never even heard of many of these critical metals – elements with exotic names like tantalum, yttrium or dysprosium.
The concern is that unlike widespread resources like iron or nitrogen, several of these elements crucial to the modern world may become prohibitively scarce. These have become known as the endangered elements. In response to the Mendeleev anniversary, the European Chemical Society (EuChemS) has released a version of the periodic table (see above) to highlight the elements that are most at risk over the coming decades.
Helium, for example is considered to be under serious threat in the next 100 years. It is the second most abundant element in the universe, but preciously rare on Earth because it is light enough to simply escape from the top of our atmosphere. The helium we do use is effectively mined from deep underground, usually along with natural gas, as it is produced as radiation particles from the decay of elements like uranium. Helium is very useful – as a cooling liquid for the superconducting magnets in hospital MRI scanners, for example, or as an extremely light gas for weather balloons and airships. But once it leaks into the air it is lost for ever, and there are concerns over meeting supply in the future. With this perspective, its frivolous use in party balloons seems almost painfully wasteful.
Many of these endangered elements are the sort of exotic metals used in modern electronics, and indeed the supply of 17 elements needed for smartphones may give cause for concern in years to come. Particularly worrying is the fact that many of those facing potential scarcity are exactly the elements we need for the green technologies to replace our reliance on fossil fuels – those used in rechargeable batteries, solar panels, and the powerful magnets within the motors of electric cars or generators in wind turbines. Gallium, for example, is needed for integrated circuits, solar panels, blue LEDs and laser diodes for Blu-ray Discs. Indium is used in everything from TVs to laptops, and in particular the touch-sensitive screens of modern smartphones and tablets. It is estimated that at current usage rates, available indium will be used up in 50 years and will become very expensive to collect and purify.
Except for helium, the problem isn’t that these scarce elements actually become lost to the planet, but that they become too expensive to mine or too dispersed to recycle effectively. “Rare earth elements”, such as yttrium, dysprosium, neodymium and scandium, are actually relatively plentiful in the Earth’s crust but aren’t geologically concentrated into rich ores. This means that they can’t be extracted economically in many areas of the world. And once they have been manufactured as tiny components within an electronic device, they can be even harder to reclaim and recycle. EuChemS calculates that 10m smartphones are discarded or replaced every month in the EU alone, and so serious action is needed to tackle these challenges of elemental scarcity.
#current events#environmentalism#capitalism#manufacturing#science#chemistry#mining#tantalum#yttrium#dysprosium#helium#indium#neodymium#scandium#dmitri mendeleev#periodic table
6 notes
·
View notes
Text
slight correction, they managed to get all four engines running but had to shut down one of them again cuz it was struggling.
furthermore, the aircraft did have onboard radar, but it didn't pick up the ash because doppler radar only works on water, and volcanic ash is bone dry. and they didn't see it because this whole event happened at night.
anyway, the engines cut out because the ash melted onto the turbine blades and choked them. the pilots knew none of this, nor why their windscreen looked like the old starfield screen saver, and could only try restarting the engines again. and again. and again. as fast as they could. they had the fuel, they knew the procedures, there wasn't anythiing to indicate that the engines were broken other then them not running. so for those twelve minutes they just kept trying to relight the engines. otherwise they would have to ditch and ditching over the ocean never ends well.
and eventually they caught and shuddered back to life. the melted ash had cooled enough to flake off the turbine blades and all four engines slowly came back online. one of them started struggling again so they shut it down to prevent damage or a flameout.
furthermore, the ILS was not inoperative otherwise they wouldn't have been able to land at all, only the part that told them how high they should be automatically.

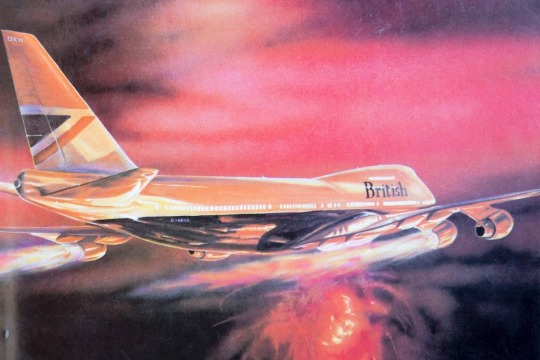
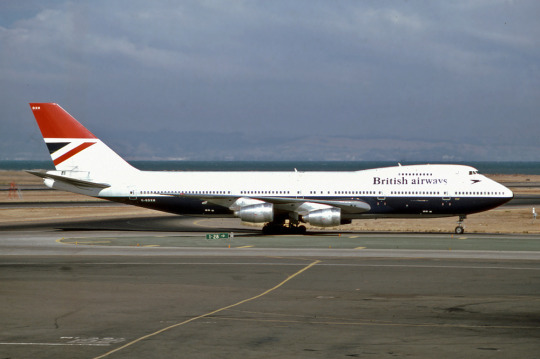
The story of British Airways Flight 009.
9K notes
·
View notes
Text
Special Hazards Fire Protection Safeguarding High-Risk Environments
What is Special Hazards Fire Protection?
Special hazards fire protection refers to the systems and practices used to detect, suppress, and control fires in areas where conventional sprinkler systems are inadequate or inappropriate. These areas typically include:
Power generation plants
Data centers and server rooms
Chemical processing facilities
Aircraft hangars
Marine environments
Clean rooms and laboratories
Museums and art storage
Industrial machinery enclosures
Each of these environments presents distinct fire risks due to the nature of the materials or equipment involved. For instance, water from a traditional sprinkler system can severely damage electronic equipment or react dangerously with certain chemicals. Special hazards systems are engineered to handle these challenges effectively.
Components of a Special Hazards Fire Protection System
A comprehensive special hazards fire protection system includes several key components:
1. Fire Detection and Alarm Systems
Rapid detection is crucial in special hazard environments. Advanced smoke, heat, and flame detectors are used, often employing technologies such as:
Aspirating Smoke Detection (ASD): These systems continuously sample air and are highly sensitive to even small amounts of smoke, making them ideal for clean rooms and server spaces.
Infrared and Ultraviolet Flame Detectors: These devices detect flames quickly and are often used in environments where fires can ignite and spread rapidly.
Linear Heat Detection: Useful for protecting cable trays and conveyor belts, this type of system detects heat along a wire or sensor cable.
2. Suppression Systems
Depending on the nature of the hazard, suppression agents can vary widely:
Clean Agents: Non-conductive, non-corrosive, and residue-free, clean agents like FM-200, Novec 1230, and CO₂ are used in data centers, telecommunication rooms, and control panels.
Foam Systems: Ideal for flammable liquid storage and aircraft hangars, foam forms a blanket over the fire, cutting off oxygen and preventing re-ignition.
Dry Chemical Systems: These are fast-acting systems used for Class B (flammable liquids and gases) and Class C (electrical) fires.
Water Mist Systems: They use high-pressure water to create a fine mist that cools the fire and displaces oxygen without damaging equipment like a traditional sprinkler might.
3. Control and Notification Panels
Centralized control panels monitor the fire protection systems and provide alerts. These panels are designed to integrate with building management systems and can initiate emergency shutdowns or notify first responders automatically.
4. Manual Release Stations and Alarms
In addition to automatic activation, most systems include manual pull stations for added safety, allowing personnel to activate suppression systems in case of a confirmed fire.
Applications and Industry Use Cases
Data Centers and Server Rooms
In environments where downtime equals significant financial loss, clean agent suppression systems are the go-to solution. These systems act quickly and leave no residue, ensuring minimal disruption to operations.
Power Generation Facilities
Turbine enclosures and transformer rooms are protected using CO₂ or water mist systems, which can suppress fires without harming expensive electrical equipment.
Chemical and Petrochemical Plants
These facilities often deal with volatile substances. Foam and dry chemical systems are commonly used, tailored to the specific flammable or reactive materials present.
Aviation and Marine Industries
Aircraft hangars and ship engine rooms face high fire risk. High-expansion foam and water mist systems are employed to rapidly suppress fires without damaging sensitive equipment or cargo.
Benefits of Special Hazards Fire Protection
1. Tailored Solutions
Each system is designed to meet the unique risks of a particular environment. This customization ensures that the protection is both effective and efficient.
2. Rapid Fire Suppression
Special hazards systems are designed for fast activation and response, minimizing fire damage and reducing business downtime.
3. Equipment and Asset Protection
Using appropriate suppression agents like clean gases or water mist helps avoid secondary damage that traditional water-based systems might cause.
4. Regulatory Compliance
Many industries are required to meet specific fire protection standards. Special hazards systems ensure compliance with codes such as NFPA 2001 (Clean Agent Fire Extinguishing Systems), NFPA 12 (CO₂ Systems), and others.
Challenges in Special Hazards Fire Protection
Despite its benefits, special hazards fire protection presents several challenges:
Complex Design Requirements: Engineers must deeply understand the protected environment and tailor systems precisely.
Cost: These systems are more expensive to design and install compared to traditional fire suppression systems.
Training: Facility personnel need specific training to operate and maintain special hazard systems safely.
Regulatory Hurdles: Navigating the numerous fire protection codes and standards can be challenging without expert guidance.
Choosing the Right Fire Protection Partner
Implementing an effective special hazards fire protection system requires collaboration with experienced professionals. When choosing a fire protection partner, look for:
Proven industry experience
Compliance with NFPA and local fire codes
Capabilities in system design, installation, and maintenance
Strong track record of system reliability and customer support
Conclusion
Special hazards fire protection is a critical investment for industries operating in high-risk environments. It offers precise, efficient, and reliable fire suppression tailored to the specific challenges of sensitive or volatile settings. As technology and operational complexity increase, the demand for specialized fire protection solutions continues to grow. By adopting the right systems and working with knowledgeable experts, businesses can ensure safety, minimize downtime, and protect valuable assets from the devastating impact of fire.
0 notes
Text
High-Performance Gear Hobbing Machine for Your Business | Gear Hobbing Solutions
In today’s fast-paced industrial world, precision and efficiency are key to success. For manufacturers working with gears, choosing the right gear hobbing machine is crucial. Whether you're in the automotive, aerospace, heavy machinery, or renewable energy sector, the quality of your gears can make or break your product. At Gear Hobbing Solutions, we provide top-quality gear hobbing machines that guarantee accuracy, durability, and exceptional performance.
As a leading distributor and solution provider in the gear manufacturing industry, we are here to help you understand how our gear hobbing machines can boost your productivity, reduce operational costs, and ensure consistent quality.
What is a Gear Hobbing Machine?
A gear hobbing machine is a specialized tool used to cut gears, splines, and sprockets. It operates using a rotating cutter known as a "hob," which gradually cuts the gear teeth into the workpiece. The machine works on a continuous cutting process, making it ideal for mass production and ensuring uniformity in every piece.
Unlike other gear cutting methods, gear hobbing is highly accurate and efficient. It is the most preferred method in industries that demand tight tolerances and consistent output.
Why Gear Hobbing Machines Matter in Manufacturing
Gears are critical components in many mechanical systems. Whether it’s a car transmission or a wind turbine gearbox, gears need to be precisely made for the entire system to function properly. A reliable gear hobbing machine helps manufacturers create gears that are:
Strong and durable
Uniform in shape and size
Capable of handling high loads
Free from defects and inconsistencies
By using a high-quality gear hobbing machine, businesses can increase their production capacity and product reliability, all while reducing wastage and rework.
Gear Hobbing Solutions – Your Trusted Partner
At Gear Hobbing Solutions, we specialize in providing advanced gear manufacturing solutions. Our wide range of gear hobbing machines is designed to meet the needs of small workshops, mid-sized manufacturers, and large-scale industries.
Here’s why clients across India trust us:
We provide only high-quality, tested, and certified machines.
Our machines offer advanced features for ease of use and high output.
We deliver excellent after-sales service and technical support.
We are committed to helping our customers achieve their production goals.
If you're looking to upgrade your existing gear cutting process or set up a new production line, our gear hobbing machines are the perfect investment.
Key Features of Our Gear Hobbing Machines
Our machines are built with advanced technology and robust materials to ensure long-lasting performance. Some of the standout features include:
1. High Precision Cutting
Our gear hobbing machines are known for their precision. Whether you're producing helical gears, spur gears, or worm gears, you can expect perfect tooth profiles every time.
2. Sturdy Construction
Made with high-grade steel and engineered to endure high loads, our machines offer stability and durability even in demanding environments.
3. Ease of Operation
With user-friendly interfaces, our gear hobbing machines are easy to operate, even for less experienced technicians. CNC options are available for complete automation.
4. Flexible Setup
You can easily change tooling and set up the machine for different gear sizes and types. This flexibility helps in handling small and large batch productions with ease.
5. Efficient Cooling and Lubrication
Our machines are equipped with proper coolant and lubrication systems that help reduce wear, enhance tool life, and improve surface finish.
Industries We Serve
Our gear hobbing machines are trusted by companies in various industries:
Automotive: For manufacturing gears used in engines, gearboxes, and drive systems
Aerospace: Precision gears for aircraft components
Agricultural: Robust gears for tractors and farming equipment
Energy: Gear systems used in wind turbines and solar trackers
Machine Tools: Gears used in heavy-duty industrial equipment
No matter the size of your operation, we have a gear hobbing solution that fits your exact needs.
How Gear Hobbing Machines Improve Productivity
When you invest in a premium gear hobbing machine, you gain more than just a tool – you gain a competitive edge. Here's how:
1. Faster Production
Hobbing is a continuous cutting process, making it much faster than other gear manufacturing methods like shaping or milling.
2. Consistent Quality
Every gear produced on a hobbing machine is virtually identical, reducing the risk of defective parts and customer complaints.
3. Lower Operating Costs
With automation and high precision, you’ll save on labor, reduce material waste, and minimize rework.
4. Scalability
Whether you need to make 10 gears or 10,000, a gear hobbing machine can easily scale to your needs without compromising quality.
Our Gear Hobbing Machine Range
At Gear Hobbing Solutions, we offer different types of gear hobbing machines based on your requirements:
Manual Gear Hobbing Machines – Ideal for low-volume production and custom jobs
Semi-Automatic Machines – Perfect balance between automation and cost-effectiveness
CNC Gear Hobbing Machines – Fully automated and high-speed production systems
Horizontal and Vertical Gear Hobbing Machines – Depending on your workspace and gear dimensions
If you're not sure which one is right for you, our experts are just a call away. We'll help you choose the best machine for your business.
Why Choose Gear Hobbing Solutions?
Here’s what sets us apart from other suppliers:
Trusted by hundreds of clients across India
Competitive pricing without compromising quality
On-time delivery and installation support
Local presence and quick service in Coimbatore and other regions
Customized solutions for your unique production goals
As one of the top names in the gear machinery industry, we’re proud to supply world-class gear hobbing machines to both small manufacturers and large corporations.
Customer Support You Can Count On
At Gear Hobbing Solutions, our relationship with customers doesn’t end at the sale. We offer:
Installation assistance
Operator training
Regular servicing and maintenance
24/7 technical support
We believe in building long-term partnerships, and that’s why our customers keep coming back for more.
Conclusion
A high-quality gear hobbing machine is an essential asset for any gear manufacturer. It ensures precision, speed, and consistent output — all crucial factors in staying ahead of your competition. At Gear Hobbing Solutions, we take pride in offering advanced gear hobbing machines that help our clients succeed.
If you are looking to invest in a reliable, efficient, and long-lasting gear hobbing machine, get in touch with us today. Our team will guide you through the entire process — from selection to setup — and ensure your production never misses a beat.
0 notes
Text
Vibration Damping Materials Market: Unveiling Trends, Strategic Drivers, and Future Growth

In a world where machines run everything from cars to skyscrapers, the importance of vibration damping materials often goes unnoticed—yet their impact is everywhere. Whether it’s a smoother ride in your car, quieter appliances, or stable industrial equipment, these materials play a crucial role in reducing noise, controlling vibrations, and enhancing product durability.
What Are Vibration Damping Materials?
Vibration damping materials are engineered to reduce or absorb mechanical energy generated by vibrations. These materials are commonly used in automotive, aerospace, electronics, industrial machinery, and construction sectors. Their job is simple but vital—minimize the transmission of vibration from one surface to another, thereby improving performance, reducing wear and tear, and ensuring a quieter, safer environment.
Why Is the Market Growing?
The vibration damping materials market is on a steady rise, and for good reason. As industries continue to innovate and automate, the need for materials that improve machine longevity and user comfort is higher than ever. The vibration damping materials market is estimated to grow USD 11.20 billion in 2025 to USD 14.20 billion by 2030, at a CAGR of 4.8%.
Here are some of the key drivers behind the growth:
1. Booming Automotive Industry
Electric vehicles (EVs), hybrid cars, and next-gen combustion engine vehicles demand high-performance vibration damping solutions. With lightweight and compact components, reducing vibration and noise has become a design priority.
2. Demand in Electronics and Appliances
Miniaturization and increasing power density in electronics lead to more internal vibration and heat. Damping materials help maintain stability, prolong lifespan, and enhance user experience—think quieter dishwashers, washing machines, and cooling fans.
3. Industrial and Manufacturing Growth
Heavy-duty machines, turbines, pumps, and compressors need damping materials to prevent failures caused by constant mechanical stress. As global manufacturing scales up, especially in emerging economies, so does the demand for vibration damping solutions.
4. Aerospace & Defense Applications
Aircraft, drones, and defense systems rely on precision and performance. Vibration damping is not optional—it's a requirement for safety and system integrity in high-pressure environments.
Market Trends to Watch
Eco-friendly materials: Sustainability is driving the demand for non-toxic, recyclable, and bio-based damping materials.
Lightweight composites: Industries are turning to advanced composites that combine damping performance with reduced weight—especially critical in aerospace and automotive sectors.
Smart materials: Integration with IoT and sensor technologies is paving the way for intelligent damping systems that adapt to environmental conditions in real-time.
Regional Insights
Asia Pacific dominates the market, thanks to rapid industrialization, infrastructure development, and a strong automotive manufacturing base in countries like China, India, and Japan.
North America follows, with innovation-led demand from the aerospace, automotive, and electronics sectors.
Europe remains strong, focusing on sustainable materials and regulatory compliance.
Key Players in the Market
Some of the leading manufacturers shaping the future of the vibration damping materials market include:
3M
BASF SE
Henkel AG & Co. KGaA
Dow Inc.
Saint-Gobain
Trelleborg AB
These companies are investing in R&D, partnerships, and strategic expansions to cater to evolving industrial needs.
Download PDF Brochure to get deeper insights :
The vibration damping materials market is a backbone of modern design and engineering. As industries evolve toward quieter, smarter, and more efficient systems, the demand for advanced damping materials will only continue to grow. For manufacturers, engineers, and innovators, staying ahead in this market means keeping a close eye on materials innovation, application trends, and regional demand patterns.
#Vibration Damping Materials#Noise Reduction Solutions#Anti-Vibration Technology#Smart Materials#Industrial Materials Market
0 notes
Text

Did you know two Buick Nailhead V8 engines were used to start the SR-71 Mach 3+ plane because the Blackbird didn’t have a starter?
The Blackbird
The SR-71, unofficially known as the “Blackbird,” was a long-range, Mach 3+, strategic reconnaissance aircraft developed from the Lockheed A-12 and YF-12A aircraft.
The first flight of an SR-71 took place on Dec. 22, 1964, and the first SR-71 to enter service was delivered to the 4200th (later 9th) Strategic Reconnaissance Wing at Beale Air Force Base, Calif., in January 1966.
The Blackbird was in a different category from anything that had come before. “Everything had to be invented. Everything,” Skunk Works legendary aircraft designer Kelly Johnson recalled in an interesting article appeared on Lockheed Martin website.
Cool Video Explains how SR-71 Blackbird’s J58 Turbo-Ramjet Engine Works
The speed of the SR-71 exceeded 2,000 mph. Other planes of the era could, in theory, approximate that speed but only in short, after-burner-driven bursts. The Blackbird maintained a record-setting speed for hours at a time. At such velocity, friction with the atmosphere generates temperatures that would melt the conventional airframe.
Two Buick Nailhead V8 engines to start the SR-71 Mach 3+ plane
When Kelly Johnson was designing the A-12/YF-12 /M-21 and SR-71 he didn’t want the weight to be added for a starter on the airplane. He said the more it weights the more fuel it will need. It was decided that two Buick Nailhead V8 engines would be able to do the job.
Did you know two Buick Nailhead V8 engines were used to start the SR-71 Mach 3+ plane because the Blackbird didn’t have a starter?
SR-71 Blackbird AG330 start cart
Not only starting the Blackbird, the fastest airplane in the world, was exciting, but also sounded like the Indianapolis 500 was getting the SR-71 ready to fly thanks to the two V8 engines as you can hear in the following video.
youtube
According to Autoevolution, for this purpose alone, two of either above-mentioned Nailhead V8s were fused together via a common transmission and drive shaft to work in tandem, then placed inside a metal housing mounted on four wheels with a trailer hitch and dubbed the AG330 “start cart.” The resulting Chimera was attached directly to the Blackbird’s two engines. Using the combined drive shaft, the two V8s spun the turbines to the point they could sustain compression by themselves. Nailhead V8s served as impromptu starter motors for the SR-71 and its cousins, the A-12 Archangel and the YF-12 fighter, until at least 1970, when the bulk of them were replaced with Chevy 454 V8s.
Did you know two Buick Nailhead V8 engines were used to start the SR-71 Mach 3+ plane because the Blackbird didn’t have a starter?
Phased out
These were also phased out when a new, quieter pneumatic system was implemented to do the same job as the start cart at most airbases on US soil the Blackbird and company operated from. Some remained for longer at auxiliary bases abroad, including a handful with the original Buick Nailheads, until the Blackbird and all its variants was retired in 1999.
@Habubrats71 via X
19 notes
·
View notes
Text
Elevating Aviation Standards: The Best Aviation Parts Offered by Skyward Industries, LLC in Washington
The aviation industry demands unparalleled quality and precision when it comes to the parts that keep aircraft flying safely and efficiently. From commercial airliners to military jets, every component must meet stringent standards to ensure maximum performance, reliability, and safety. This is where Skyward Industries, LLC, a leading company based in Washington, stands out. Specializing in top-quality aviation parts, Skyward Industries provides aerospace manufacturers, maintenance facilities, and operators with the most reliable components that adhere to the highest safety and performance standards.
Why Choose Skyward Industries for Aviation Parts?
Skyward Industries, LLC has built a strong reputation in the aviation industry due to its unwavering commitment to quality and precision. The company offers a comprehensive selection of aviation parts designed to meet the needs of diverse aviation sectors, including commercial, private, and military aviation. Their products are meticulously engineered and tested to comply with rigorous aerospace specifications and regulations, ensuring they meet or exceed industry standards.
Skyward Industries' success is attributed to its focus on innovation, customer satisfaction, and long-term partnerships. The company’s dedicated team works closely with clients to provide tailored solutions that meet specific operational requirements. Whether you're in need of replacement parts, upgrades, or custom solutions, Skyward Industries offers expertise and high-performance products that enhance the functionality and longevity of any aircraft.
The Range of Aviation Parts Offered by Skyward Industries
Skyward Industries provides a vast range of aviation parts, all engineered to support the complex demands of modern aviation. Some of the key product categories include:
Airframe Components: Airframe parts are the backbone of any aircraft. Skyward Industries offers high-quality airframe components such as fuselage parts, wings, and control surfaces. These parts are designed for durability and are manufactured to handle the stresses of flight while maintaining structural integrity.
Landing Gear Systems: Skyward Industries manufactures and supplies critical landing gear components. These include wheels, tires, actuators, struts, and brake systems, all built to withstand the demanding conditions of takeoff, flight, and landing. Their products are engineered for longevity and reliability, ensuring the aircraft’s safe landing in all weather conditions.
Engine Parts: The engine is one of the most crucial components of any aircraft. Skyward Industries supplies high-quality engine parts, including turbine blades, exhaust systems, and cooling components. Each part is precision-engineered to ensure optimal engine performance and reliability, contributing to both fuel efficiency and overall safety.
Electrical and Avionics Parts: Electrical systems and avionics are the nervous system of any aircraft, controlling everything from navigation to communication. Skyward Industries provides a range of avionics components, such as cockpit displays, sensors, wiring harnesses, and power distribution systems. These parts are designed to meet rigorous standards for both functionality and safety.
Hydraulic and Fuel Systems: Hydraulic systems are critical for controlling various functions, including flight control surfaces, brakes, and landing gear. Fuel systems are equally important for ensuring the aircraft’s engines run smoothly and efficiently. Skyward Industries offers hydraulic pumps, valves, fuel pumps, and other key components that are built to perform under high pressure and extreme conditions.
Interior and Cabin Parts: The comfort and safety of passengers are just as important as the performance of the aircraft itself. Skyward Industries provides cabin and interior components such as seating systems, cabin lighting, emergency exits, and other vital interior parts. These components are designed with both passenger comfort and safety in mind.
The Skyward Industries Commitment to Quality
Skyward Industries, LLC is known for its dedication to quality control. Each aviation part undergoes rigorous testing and inspection to ensure it meets or exceeds the stringent regulatory requirements set by aviation authorities such as the FAA (Federal Aviation Administration). The company maintains strong relationships with certified manufacturers and suppliers, ensuring that every part meets the highest standards of performance and safety.
The company also invests in research and development to innovate and improve aviation technologies, making sure that their parts keep pace with industry advancements. This commitment to excellence has earned Skyward Industries a trusted name in the aviation community, making them a reliable partner for businesses seeking superior parts.
Why Skyward Industries is the Best Choice for Aviation Parts
Unmatched Quality: Skyward Industries provides only the highest-quality parts designed to perform under the most demanding conditions. Each part is carefully crafted and rigorously tested to meet industry standards, ensuring long-term reliability.
Expertise and Experience: With years of experience in the aviation industry, Skyward Industries has developed deep technical expertise. Their team understands the intricacies of aircraft maintenance and the importance of using top-quality parts to ensure optimal performance.
Customer-Centric Service: Skyward Industries places a strong emphasis on customer satisfaction. They offer personalized service, working closely with clients to understand their needs and provide tailored solutions that meet the specific requirements of each aircraft.
Timely Delivery: In the fast-paced world of aviation, time is critical. Skyward Industries understands the urgency of timely part delivery, and their efficient logistics ensure that clients receive the parts they need when they need them, minimizing downtime and optimizing operational efficiency.
Competitive Pricing: Despite offering premium-quality parts, Skyward Industries provides competitive pricing that makes it easier for customers to maintain their aircraft while staying within budget. The company offers both cost-effective options for routine maintenance as well as high-end components for specialized needs.
Conclusion
When it comes to aviation parts that ensure the safety, reliability, and efficiency of your aircraft, Skyward Industries, LLC is the trusted partner you need. Based in Washington, the company provides a wide range of high-quality parts that cater to every aspect of modern aviation, from airframes to avionics, engines, and interiors. With their commitment to quality, customer satisfaction, and innovation, Skyward Industries continues to play a crucial role in keeping aircraft flying safely and efficiently. Whether you're an operator, maintenance provider, or manufacturer, choosing Skyward Industries for your aviation parts needs guarantees top-tier performance and reliability for your fleet.
0 notes
Text
Computational Fluid Dynamics (CFD) Market Report 2034
Computational Fluid Dynamics (CFD) Market Outlook
The global computational fluid dynamics (CFD) market is gaining significant traction owing to its expanding use across diverse industries such as automotive, aerospace, energy, healthcare, and electronics. With the growing need for accurate simulation tools to design efficient and sustainable products, CFD solutions have become indispensable in modern engineering. The integration of high-performance computing (HPC), artificial intelligence (AI), and cloud-based services is transforming the way simulations are conducted, enabling more complex and precise modelling in shorter timeframes. Environmental challenges and the global focus on sustainability are further boosting the relevance of CFD technologies. These tools not only help in improving product performance but also play a pivotal role in reducing emissions and energy consumption, making the market outlook for the computational fluid dynamics market highly promising over the next decade.
In 2024, the global computational fluid dynamics market size attained a value of USD 2.52 billion. This figure encompasses both traditional on-premises software and modern cloud CFD market platforms used for fluid flow simulation, heat transfer, and related processes. The cfd market size reflects the widespread adoption of CFD solutions in various sectors, particularly automotive and aerospace, where fluid dynamics plays a crucial role in vehicle design and fuel efficiency. Furthermore, the growing use of simulation tools in HVAC systems, electronics cooling, and medical devices adds to the overall market volume. As industries shift towards digitised and simulation-based design approaches, the computational fluid dynamics market size is expected to see substantial growth.
Computational Fluid Dynamics (CFD) Market Growth
The computational fluid dynamics (CFD) market is projected to grow at a compound annual growth rate (CAGR) of 11.70% from 2025 to 2034, reaching USD 7.62 billion by 2034. This growth is driven by the increasing complexity of product designs, higher demand for virtual prototyping, and cost pressures associated with physical testing. Automotive companies, for instance, are using CFD to optimise aerodynamics and thermal management systems, while the aerospace sector is leveraging these tools for aircraft design and performance validation. Energy firms are also exploring CFD to improve wind turbine efficiency and cooling in power generation plants. Additionally, the Asia Pacific computational fluid dynamics market is emerging as a key growth driver, with countries like China, Japan, and India investing heavily in industrial automation and advanced engineering solutions. This regional expansion is a vital element in the overall cfd market growth.
Computational Fluid Dynamics (CFD) Market Trends
Current cfd market trends highlight a growing shift toward cloud-based CFD platforms that offer scalable computing resources and remote collaboration capabilities. Cloud CFD market solutions are particularly beneficial for small and medium enterprises that may not have access to high-end computing infrastructure. Another notable trend is the integration of machine learning and AI to enhance simulation speed, predictability, and accuracy. AI-driven CFD systems are able to reduce simulation time and assist in automated optimisation processes. The push for real-time CFD simulation and digital twin technologies is also transforming how engineers approach design and system monitoring. Furthermore, the apac computational fluid dynamics market is witnessing a rise in the adoption of open-source CFD tools, which are reducing entry barriers for new users and promoting innovation. These cfd market insights indicate a future of increased accessibility, accuracy, and efficiency in simulation workflows.
Receive a detailed report, including the Table of Contents: https://www.expertmarketresearch.com/reports/computational-fluid-dynamics-market/requestsample
Opportunities and Challenges
The computational fluid dynamic market presents significant opportunities across several verticals. In the automotive industry, increasing demand for electric vehicles (EVs) has opened new avenues for CFD applications in battery cooling, cabin airflow, and electric motor optimisation. The aerospace sector is focusing on fuel-efficient aircraft and unmanned aerial systems (UAS), where CFD plays a vital role in performance testing. In the energy domain, CFD is crucial for designing efficient wind turbines, cooling systems in nuclear reactors, and optimising combustion in thermal plants. The healthcare sector is also exploring CFD for blood flow simulation and respiratory device development.
Despite these opportunities, the cfd market faces challenges such as the high cost of advanced simulation tools, the need for skilled professionals, and the complexity of integrating CFD with other digital engineering solutions. Additionally, real-time simulation still requires substantial computational resources, which can limit adoption among smaller companies. Addressing these issues requires collaboration between software vendors, hardware providers, and research institutions to make CFD more user-friendly and cost-effective.
Computational Fluid Dynamics (CFD) Market Analysis
The global computational fluid dynamics market is highly competitive, with a mix of established players and emerging solution providers. Major companies such as ANSYS, Siemens Digital Industries Software, Dassault Systèmes, Altair Engineering, and Autodesk dominate the cfd market share, offering a wide range of tools tailored for various industries. These players continue to innovate by integrating cloud computing, automation, and AI capabilities into their offerings.
Regionally, the Asia Pacific computational fluid dynamics market is gaining ground due to industrialisation, smart manufacturing initiatives, and academic collaborations. The APAC computational fluid dynamics market is expected to see rapid adoption in automotive and electronics sectors, where simulation tools are used extensively to enhance product performance and reduce time-to-market. Europe and North America remain mature markets, focusing on sustainability, regulatory compliance, and innovation through digital twins.
In terms of application, the automotive and aerospace segments remain dominant contributors to the cfd market size, followed by energy, electronics, and healthcare. The increased availability of user-friendly CFD platforms and cloud integration is democratising access to simulation tools, expanding their use across startups, research institutions, and mid-sized firms.
The ongoing evolution of computational fluid dynamics tools, combined with growing environmental and performance demands, is positioning the computational fluid dynamics (CFD) market as a vital enabler of next-generation product development across industries. These comprehensive cfd market analysis findings suggest strong potential for continued innovation and global adoption in the years ahead.
Media Contact:
Company Name: Claight Corporation
Email: [email protected]
Toll Free Number: +1-415-325-5166 | +44-702-402-5790
Address: 30 North Gould Street, Sheridan, WY 82801, USA
Website: www.expertmarketresearch.com
Aus Site: https://www.expertmarketresearch.com.au
1 note
·
View note