Photo
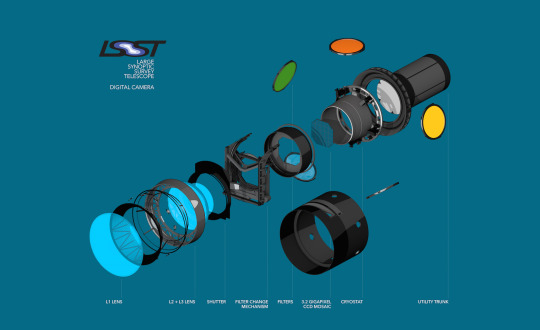
World’s Most Powerful Digital Camera Sees Construction Green Light
This exploded view of the LSST’s digital camera highlights its various components, including lenses, shutter and filters.
The Department of Energy has approved the start of construction for a 3.2-gigapixel digital camera – the world’s largest – at the heart of the Large Synoptic Survey Telescope (LSST). Assembled at the DOE's SLAC National Accelerator Laboratory, the camera will be the eye of LSST, revealing unprecedented details of the universe and helping unravel some of its greatest mysteries.
The construction milestone, known as Critical Decision 3, is the last major approval decision before the acceptance of the finished camera, said LSST Director Steven Kahn: “Now we can go ahead and procure components and start building it.”
Starting in 2022, LSST will take digital images of the entire visible southern sky every few nights from atop a mountain called Cerro Pachón in Chile. It will produce a wide, deep and fast survey of the night sky, cataloguing by far the largest number of stars and galaxies ever observed. During a 10-year time frame, LSST will detect tens of billions of objects—the first time a telescope will observe more galaxies than there are people on Earth – and will create movies of the sky with unprecedented details.
The telescope’s camera – the size of a small car and weighing more than three tons – will capture full-sky images at such high resolution that it would take 1,500 high-definition television screens to display just one of them.
The LSST’s camera will include a filter-changing mechanism and shutter, which allows the camera to view different wavelengths; the camera is capable of viewing light from near-ultraviolet to near-infrared (0.3-1 μm) wavelengths.
This has already been a busy year for the LSST Project. Its dual-surface primary/tertiary mirror – the first of its kind for a major telescope – was completed; a traditional stone-laying ceremony in northern Chile marked the beginning of on-site construction of the facility; and a nearly 2,000-square-foot, 2-story-tall clean room was completed at SLAC to accommodate fabrication of the camera.
LSST will generate a vast public archive of data—approximately 6 million gigabytes per year, or the equivalent of shooting roughly 800,000 images with a regular 8-megapixel digital camera every night, albeit of much higher quality and scientific value. This data will help researchers study the formation of galaxies, track potentially hazardous asteroids, observe exploding stars and better understand dark matter and dark energy, which together make up 95 percent of the universe but whose natures remain unknown.
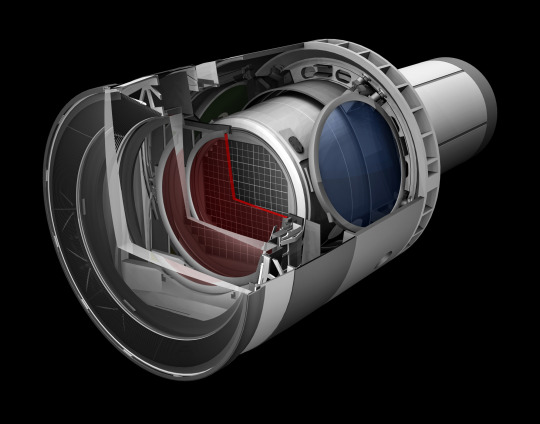
Rendering of the LSST camera.
1 note
·
View note
Photo
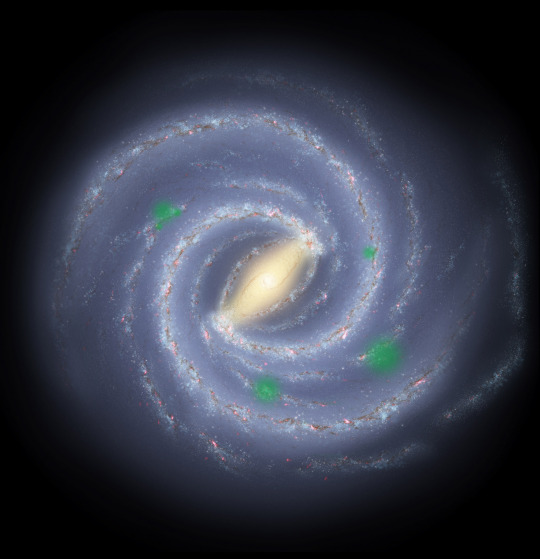
Interstellar Seeds Could Create Oases of Life
We only have one example of a planet with life: Earth. But within the next generation, it should become possible to detect signs of life on planets orbiting distant stars. If we find alien life, new questions will arise. For example, did that life arise spontaneously? Or could it have spread from elsewhere? If life crossed the vast gulf of interstellar space long ago, how would we tell?
New research by Harvard astrophysicists shows that if life can travel between the stars (a process called panspermia), it would spread in a characteristic pattern that we could potentially identify.
"In our theory clusters of life form, grow, and overlap like bubbles in a pot of boiling water," says lead author Henry Lin of the Harvard-Smithsonian Center for Astrophysics (CfA).
There are two basic ways for life to spread beyond its host star. The first would be via natural processes such as gravitational slingshotting of asteroids or comets. The second would be for intelligent life to deliberately travel outward. The paper does not deal with how panspermia occurs. It simply asks: if it does occur, could we detect it? In principle, the answer is yes.
The model assumes that seeds from one living planet spread outward in all directions. If a seed reaches a habitable planet orbiting a neighboring star, it can take root. Over time, the result of this process would be a series of life-bearing oases dotting the galactic landscape.
"Life could spread from host star to host star in a pattern similar to the outbreak of an epidemic. In a sense, the Milky Way galaxy would become infected with pockets of life," explains CfA co-author Avi Loeb.
If we detect signs of life in the atmospheres of alien worlds, the next step will be to look for a pattern. For example, in an ideal case where the Earth is on the edge of a "bubble" of life, all the nearby life-hosting worlds we find will be in one half of the sky, while the other half will be barren.
Lin and Loeb caution that a pattern will only be discernible if life spreads somewhat rapidly. Since stars in the Milky Way drift relative to each other, stars that are neighbors now won't be neighbors in a few million years. In other words, stellar drift would smear out the bubbles.
This research has been accepted for publication in The Astrophysical Journal Letters.
1 note
·
View note
Photo
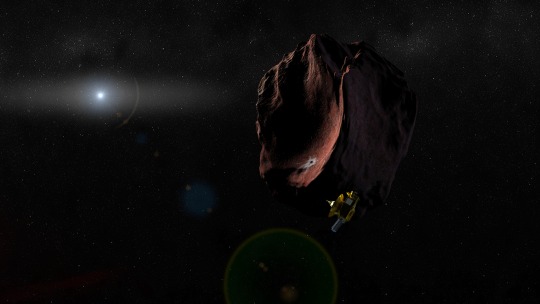
New Horizons Team Selects Potential Kuiper Belt Flyby Target
Artist's impression of NASA's New Horizons spacecraft encountering a Pluto-like object in the distant Kuiper Belt.
NASA has selected the potential next destination for the New Horizons mission to visit after its historic July 14 flyby of the Pluto system. The destination is a small Kuiper Belt object (KBO) known as 2014 MU69 that orbits nearly a billion miles beyond Pluto.
This remote KBO was one of two identified as potential destinations and the one recommended to NASA by the New Horizons team. Although NASA has selected 2014 MU69 as the target, as part of its normal review process the agency will conduct a detailed assessment before officially approving the mission extension to conduct additional science.
Like all NASA missions that have finished their main objective but seek to do more exploration, the New Horizons team must write a proposal to the agency to fund a KBO mission. That proposal – due in 2016 – will be evaluated by an independent team of experts before NASA can decide about the go-ahead.
Early target selection was important; the team needs to direct New Horizons toward the object this year in order to perform any extended mission with healthy fuel margins. New Horizons will perform a series of four maneuvers in late October and early November to set its course toward 2014 MU69 – nicknamed “PT1” (for “Potential Target 1”) – which it expects to reach on January 1, 2019. Any delays from those dates would cost precious fuel and add mission risk.
“2014 MU69 is a great choice because it is just the kind of ancient KBO, formed where it orbits now, that the Decadal Survey desired us to fly by,” said New Horizons Principal Investigator Alan Stern, of the Southwest Research Institute (SwRI) in Boulder, Colorado. “Moreover, this KBO costs less fuel to reach [than other candidate targets], leaving more fuel for the flyby, for ancillary science, and greater fuel reserves to protect against the unforeseen.”
New Horizons was originally designed to fly beyond the Pluto system and explore additional Kuiper Belt objects. The spacecraft carries extra hydrazine fuel for a KBO flyby; its communications system is designed to work from far beyond Pluto; its power system is designed to operate for many more years; and its scientific instruments were designed to operate in light levels much lower than it will experience during the 2014 MU69 flyby.
Finding a suitable KBO flyby target was no easy task. Starting a search in 2011 using some of the largest ground-based telescopes on Earth, the New Horizons team found several dozen KBOs, but none were reachable within the fuel supply aboard the spacecraft.
The powerful Hubble Space Telescope came to the rescue in summer 2014, discovering five objects, since narrowed to two, within New Horizons’ flight path. Scientists estimate that PT1 is just under 30 miles (about 45 kilometers) across; that’s more than 10 times larger and 1,000 times more massive than typical comets, like the one the Rosetta mission is now orbiting, but only about 0.5 to 1 percent of the size (and about 1/10,000th the mass) of Pluto. As such, PT1 is thought to be like the building blocks of Kuiper Belt planets such as Pluto.
Unlike asteroids, KBOs have been heated only slightly by the Sun, and are thought to represent a well preserved, deep-freeze sample of what the outer solar system was like following its birth 4.6 billion years ago.
“There’s so much that we can learn from close-up spacecraft observations that we’ll never learn from Earth, as the Pluto flyby demonstrated so spectacularly,” said New Horizons science team member John Spencer, also of SwRI. “The detailed images and other data that New Horizons could obtain from a KBO flyby will revolutionize our understanding of the Kuiper Belt and KBOs.” The New Horizons spacecraft – currently 3 billion miles [4.9 billion kilometers] from Earth – is just starting to transmit the bulk of the images and other data, stored on its digital recorders, from its historic July encounter with the Pluto system. The spacecraft is healthy and operating normally.
0 notes
Photo
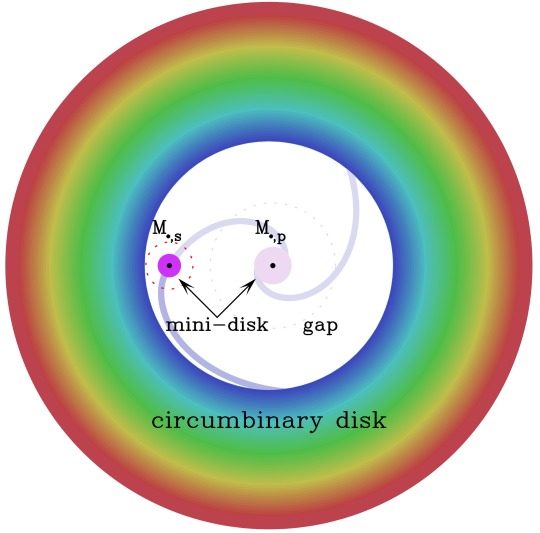
A binary black hole at the heart of the nearest quasar Markarian 231
Schematic diagram for a BBH–disk (BBH = Binary Black Hole) accretion system. The BBH is assumed to be on circular orbits. The BBH is surrounded by a circumbinary disk, connecting with the mini-disk around each component of the BBH by streams. In between the circumbinary disk and the inner mini-disks, a gap or hole is opened by the secondary SMBH (= SuperMassive Black Hole).
Conclusions from the study “A probable Milli-Parsec Supermassive Binary Black Hole in the Nearest Quasar Mrk 231”:
In this paper, we show that various unique features in the optical-to-UV spectrum and the intrinsic X-ray weakness of Mrk 231 can all be well explained, if a pair of SMBHs exists in the core of Mrk 231, with the masses of the primary and the secondary SMBHs as ∼ 1.5 × 108M⊙ and 4.5 × 106M⊙, respectively. The existence of a BBH in Mrk 231 is compatible with its disturbed morphology and tidal features, which indicates a merger event in the past. (Note that the secondary SMBH is rather low in mass; however, it should be able to sink down to the center because the stars initially associated with it enhance the dynamical friction.) The semimajor axis of this BBH is ∼ 590 AU, about 190 times of the Schwarzschild radius of the primary SMBH, and its orbital period is just ∼ 1.2 year, relatively short among the few known BBH candidates, which makes it an ideal system to study the dynamics of BBH systems. Such a BBH emits gravitational wave on tens of nanohertz, and the change rate of its orbital period due to gravitational wave radiation is about 40 seconds per orbit. This BBH might be a target for gravitational wave studies in future.
The orbit of such a BBH system decays on a timescale of a few times of 105 year due to gravitational wave radiation and the torque of the circumbinary disk, which is not too small compared with the lifetime of quasars (a few times 107 to 108 year). The majority of quasars are believed to be triggered by mergers of galaxies and consequently involve mergers of SMBHs, and those BBH systems with mass ratio in the range of a few percent to 1 may lead to a notch in the optical- to-UV continuum emission if their semimajor axes are in the range of a few hundreds to about one thousands gravitational radii, which correspond to orbital decay timescales of 105–106 year. Therefore, the occurrence rate of active BBH systems, with deficits in the optical-to-UV emission, may be roughly a few thousandths to about one percent among quasars. Our analysis of Mrk 231 demonstrates the feasibility of finding BBH systems by searching for the deficits in the optical-to-UV emission among the spectra of quasars, a new method proposed by a number of authors.
0 notes
Photo
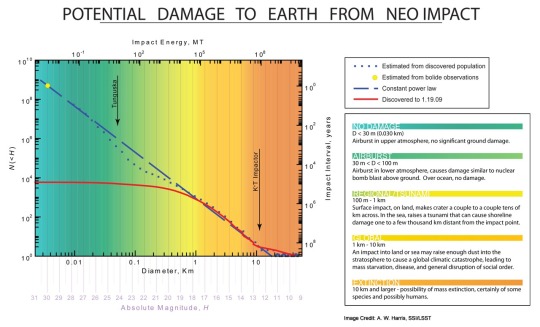
How big would an asteroid or comet have to be to end life on Earth?
NEO=Near Earth Object. The Absolute Magnitude (H) on the bottom axes is a measure of a body's brightness and is correlated with size. The larger an asteroid is, the brighter it will appear, assuming that all asteroids have the same surface composition (which they don't, but the assumption is likely good enough to give a rough estimate of the body's size).
This is an excellent diagram by astronomer Alan Harris that provides a lot of information on the relationship between asteroid size, damage, and frequency of impact.
The K-T impact event that caused the extinction of about 3/4 of the Earth's animal and plant species was likely caused by an impact from a body with a diameter of about 10 km. The good news is that there's an inverse relationship between the size of the impactor and the number of years between events of similar magnitude (in this case about a 100 million years for a mass-extinction event). The bad news is that we're likely due for another event fairly soon (The K-T event was 66 million years ago.)
To end all life on Earth, an impactor with a diameter between 10-100 km would likely do the job.
0 notes
Photo
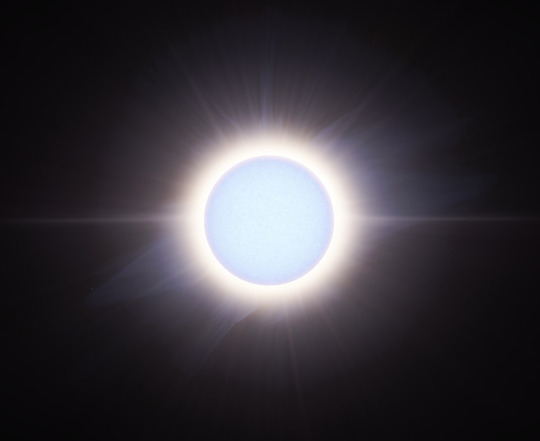
Dying star suffers ‘irregular heartbeats’
Some dying stars suffer from ‘irregular heartbeats’, research led by astronomers at the University of Warwick has discovered.
The research confirms rapid brightening events in otherwise normal pulsating white dwarfs, which are stars in the final stage of their life cycles.
In addition to the regular rhythm from pulsations they expected on the white dwarf PG1149+057, which cause the star to get a few percent brighter and fainter every few minutes, the researchers also observed something completely unexpected every few days: arrhythmic, massive outbursts, which broke the star's regular pulse and significantly heated up its surface for many hours.
The discovery was made possible by using the planet-hunting spacecraft Kepler, which stares unblinkingly at a small patch of sky, uninterrupted by clouds or sunrises.
Led by Dr JJ Hermes of the University of Warwick’s Astrophysics Group, the astronomers targeted the Kepler spacecraft on a specific star in the constellation Virgo, PG1149+057, which is roughly 120 light years from Earth.
Dr Hermes explains:
"We have essentially found rogue waves in a pulsating star, akin to ‘irregular heartbeats’. These were truly a surprise to see: we have been watching pulsating white dwarfs for more than 50 years now from the ground, and only by being able to stare uninterrupted for months from space have we been able to catch these events.”
The star with the irregular beat, PG1149+057, is a pulsating white dwarf, which is the burnt-out core of an evolved star, an extremely dense star which is almost entirely made up of carbon and oxygen. Our Sun will eventually become a white dwarf in more than six billion years, after it runs out of its nuclear fuel.
White dwarfs have been known to pulsate for decades, and some are exceptional clocks, with pulsations that have kept nearly perfect time for more than 40 years. Pulsations are believed to be a naturally occurring stage when a white dwarf reaches the right temperature to generate a mix of partially ionized hydrogen atoms at its surface.
That mix of excited atoms can store up and then release energy, causing the star to resonate with pulsations characteristically every few minutes. Astronomers can use the regular periods of these pulsations just like seismologists use earthquakes on Earth, to see below the surface of the star into its exotic interior. This was why astronomers targeted PG1149+057 with Kepler, hoping to learn more about its dense core. In the process, they caught a new glimpse at these unexpected outbursts.
“These are highly energetic events, which can raise the star's overall brightness by more than 15% and its overall temperature by more than 750 degrees in a matter of an hour,” said Dr Hermes. “For context, the Sun will only increase in overall brightness by about 1% over the next 100 million years.”
Interestingly, this is not the only white dwarf to show an irregular pulse. Recently, the Kepler spacecraft witnessed the first example of these strange outbursts while studying another white dwarf, KIC 4552982, which was observed from space for more than 2.5 years.
There is a narrow range of surface temperatures where pulsations can be excited in white dwarfs, and so far irregularities have only been seen in the coolest of those that pulsate. Thus, these irregular outbursts may not be just an oddity; they have the potential to change the way astronomers understand how pulsations, the regular heartbeats, ultimately cease in white dwarfs.
“The theory of stellar pulsations has long failed to explain why pulsations in white dwarfs stop at the temperature we observe them to,” argues Keaton Bell of the University of Texas at Austin, who analysed the first pulsating white dwarf to show an irregular heartbeat, KIC 4552982. "That both stars exhibiting this new outburst phenomenon are right at the temperature where pulsations shut down suggests that the outbursts could be the key to revealing the missing physics in our pulsation theory."
Astronomers are still trying to settle on an explanation for these never-before-seen outbursts. Given the similarity between the first two stars to show this behaviour, they suspect it might have to do with how the pulsation waves interact with themselves, perhaps via a resonance.
"Ultimately, this may be a new type of nonlinear behaviour that is triggered when the amplitude of a pulsation passes a certain threshold, perhaps similar to rogue waves on the open seas here on Earth, which are massive, spontaneous waves that can be many times larger than average surface waves," said Dr Hermes. "Still, this is a fresh discovery from observations, and there may be more to these irregular stellar heartbeats than we can imagine yet."
3 notes
·
View notes
Photo
Discovering Dust-Obscured Active Galaxies as They Grow
The number density of DOGs (Dust Obscured Galaxies) as a function of infrared luminosity. Data represented by the red star is the HSC result (Hyper Suprime-Cam, or HSC, is a new wide-field camera mounted at the prime focus of the Subaru Telescope). The research team found that (i) their infrared luminosity exceeds 10 trillion suns, and (ii) their number density is about 300 per cubic gigaparsecs (1 gigaparsec is about 3×10^25 meter).
How did galaxies form and evolve during the 13.8-billion-year history of the universe? This question has been the subject of intense observational and theoretical investigation. Recent studies have revealed that almost all massive galaxies harbor a supermassive black hole whose mass reaches up to a hundred thousand or even a billion times the mass of the sun, and their masses are tightly correlated with those of their host galaxies. This correlation suggests that supermassive black holes and their host galaxies have evolved together, closely interacting as they grow.
The group of researchers, lead by Dr. Yoshiki Toba (Ehime University), focused on the Dust Obscured Galaxies (DOGs) as a key population to tackle the mystery of the co-evolution of galaxies and black holes. DOGs are very faint in visible light, because of the large quantity of obscuring dust, but are bright in the infrared. The brightest infrared DOGs in particular are expected to harbor the most actively growing black hole. In addition, most DOGs are seen in the epoch when the star formation activity of galaxies reached its peak, 8-10 billion years ago. Thus both DOGs and their black holes are rapidly growing, at an early phase of their co-evolution. However, since DOGs are rare and are hidden behind significant amount of dust, previous visible light surveys have found very few such objects.
Hyper Suprime-Cam (HSC) is a new instrument installed on the 8.2 meter Subaru Telescope in 2012. It is a wide-field camera with a field of view nine times the size of the full moon. An ambitious legacy survey with HSC started in March 2014 as a "Subaru strategic program"; total of 300 nights have been allocated for a five year period. The Subaru strategic program with HSC started to deliver large quantities of excellent imaging data.
The research team selected DOGs from early data from the HSC Subaru Strategic Program (SSP). DOGs are thousand times brighter in the infrared than the optical and the team selected their targets using the HSC and NASA's Wide-field Infrared Survey Explorer. They also utilized the data from the VISTA Kilo-degree Infrared Galaxy survey (VIKING). The all-sky survey data with WISE are crucial to discover spatially rare DOG while the VIKING data are useful to identify the DOGs more precisely.
Consequently, 48 DOGs were discovered. Each of these is 10 trillion times more luminous in the infrared than the sun. The number density of these luminous DOGs is about 300 per cubic gigaparsecs. It is theoretically predicted that these DOGs harbor an actively evolving supermassive black hole. This result provides researchers new insights into the mysteries of the co-evolution of galaxies and supermassive black holes from the unique observational prospects.
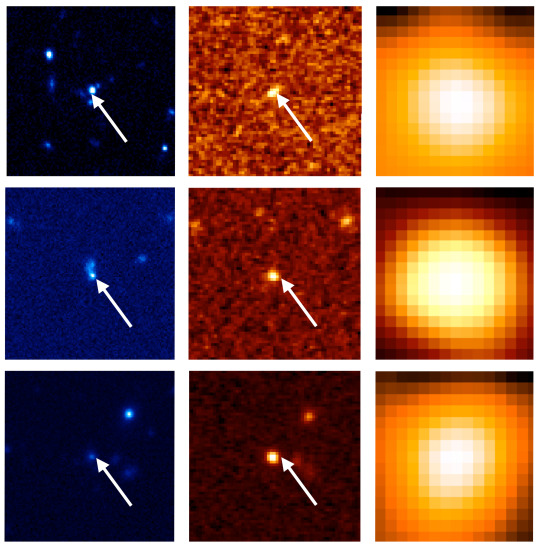
Images of 3 DOG's. The left, middle, and right panels show optical image from HSC, near-infrared image from VIKING, and mid-infrared image from WISE, respectively. The image size is 20 square arcsecond (1 arcsecond is 1/3600 degree). It is clear that DOGs are faint in the optical, but are extremely bright in the infrared.
In this research, the research team discovered 48 Dust Obscured Galaxies and revealed their statistical properties of infrared luminous DOGs in particular, for the first time.
0 notes
Video
youtube
Huygens’ view of the 1st landing on Titan
Ten years ago, ESA's Huygens probe descended to the surface of Titan, Saturn's largest moon.
This movie, built with data collected during ESA Huygens' mission at Titan on 14 January 2005, shows the operation of the DISR camera during its descent up to touch-down. The almost 4-hour long operation of DISR is shown in less than five minutes - 40 times the actual speed up to landing and 100 times the actual speed thereafter.
The first part of the movie shows how Titan looked to DISR as it acquired more and more images during the probe's descent. Each DISR image has a small field of view, and dozens of images were made into mosaics of the whole scene.
The scientists analysed Huygens' speed, direction of motion, rotation and swinging during descent. The DISR movie includes sidebar graphics that show:
(Lower left corner) Huygens' trajectory views from the south, a scale bar for comparison to the height of Mount Everest, colored arrows that point to the sun and to the Cassini orbiter.
(Top left corner) A close-up view of the Huygens probe highlighting large and unexpected parachute movements, and a scale bar for comparison to human height.
(Lower right corner) A compass that shows the changing direction of view as Huygens rotates, along with the relative positions of the sun and Cassini.
(Upper right corner) A clock that shows Universal Time for Jan. 14, 2005 (Universal Time is two hours earlier with respect to Central European Summer Time). Above the clock, events are listed in Mission Time, which starts with the deployment of the first of the three parachutes.
Sounds from a left speaker trace Huygens' motion, with tones changing with rotational speed and the tilt of the parachute. There also are clicks that clock the rotational counter, as well as sounds for the probe's heat shield hitting Titan's atmosphere, parachute deployments, heat shield release, jettison of the DISR cover and touch-down.
Sounds from a right speaker go with DISR activity. There's a continuous tone that represents the strength of Huygens' signal to Cassini. Then there are 13 different chimes - one for each of DISR's 13 different science parts - that keep time with flashing-white-dot exposure counters.
During its descent, DISR took 3500 exposures.
0 notes
Photo
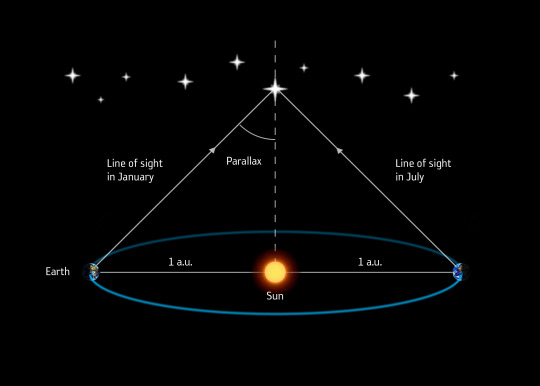
Gaia’s first year of scientific observations
Top image: Gaia's first Hertzsprung-Russell diagram; bottom: The parallax method of measuring a star's distance.
On 21 August 2015, ESA’s billion-star surveyor, Gaia, completed its first year of science observations in its main survey mode.
After launch on 19 December 2013 and a six-month long in-orbit commissioning period, the satellite started routine scientific operations on 25 July 2014. Located at the Lagrange point L2, 1.5 million km from Earth, Gaia surveys stars and many other astronomical objects as it spins, observing circular swathes of the sky. By repeatedly measuring the positions of the stars with extraordinary accuracy, Gaia can tease out their distances and motions through the Milky Way galaxy.
For the first 28 days, Gaia operated in a special scanning mode that sampled great circles on the sky, but always including the ecliptic poles. This meant that the satellite observed the stars in those regions many times, providing an invaluable database for Gaia’s initial calibration.
At the end of that phase, on 21 August 2014, Gaia commenced its main survey operation, employing a scanning law designed to achieve the best possible coverage of the whole sky.
Since the start of its routine phase, the satellite recorded 272 billion positional or astrometric measurements, 54.4 billion brightness or photometric data points, and 5.4 billion spectra.
The Gaia team have spent a busy year processing and analysing these data, en route towards the development of Gaia’s main scientific products, consisting of enormous public catalogues of the positions, distances, motions and other properties of more than a billion stars. Because of the immense volumes of data and their complex nature, this requires a huge effort from expert scientists and software developers distributed across Europe, combined in Gaia’s Data Processing and Analysis Consortium (DPAC).
As one example of the ongoing validation, the Gaia team has been able to measure the parallax for an initial sample of two million stars.
Parallax is the apparent motion of a star against a distant background observed over the period of a year and resulting from the Earth's real motion around the Sun; this is also observed by Gaia as it orbits the Sun alongside Earth. But parallax is not the only movement seen by Gaia: the stars are also really moving through space, which is called proper motion.
Gaia has made an average of roughly 14 measurements of each star on the sky thus far, but this is generally not enough to disentangle the parallax and proper motions.
To overcome this, the scientists have combined Gaia data with positions extracted from the Tycho-2 catalogue, based on data taken between 1989 and 1993 by Gaia's predecessor, the Hipparcos satellite.
This restricts the sample to just two million out of the more than one billion that Gaia has observed so far, but yields some useful early insights into the quality of its data.
The nearer a star is to the Sun, the larger its parallax, and thus the parallax measured for a star can be used to determine its distance. In turn, the distance can be used to convert the apparent brightness of the star into its true brightness or ‘absolute luminosity’.
Astronomers plot the absolute luminosities of stars against their temperatures – which are estimated from the stars' colours – to generate a ‘Hertzsprung-Russell diagram’, named for the two early 20th century scientists who recognised that such a diagram could be used as a tool to understand stellar evolution.
As Gaia has been conducting its repeated scans of the sky to measure the motions of stars, it has also been able to detect whether any of them have changed their brightness, and in doing so, has started to discover some very interesting astronomical objects.
Gaia has detected hundreds of transient sources so far, with a supernova being the very first on 30 August 2014. These detections are routinely shared with the community at large as soon as they are spotted in the form of ‘Science Alerts’, enabling rapid follow-up observations to be made using ground-based telescopes in order to determine their nature.
One transient source was seen undergoing a sudden and dramatic outburst that increased its brightness by a factor of five. It turned out that Gaia had discovered a so-called ‘cataclysmic variable’, a system of two stars in which one, a hot white dwarf, is devouring mass from a normal stellar companion, leading to outbursts of light as the material is swallowed. The system also turned out to be an eclipsing binary, in which the relatively larger normal star passes directly in front of the smaller, but brighter white dwarf, periodically obscuring the latter from view as seen from Earth.
Unusually, both stars in this system seem to have plenty of helium and little hydrogen.
Gaia has also discovered a multitude of stars whose brightness undergoes more regular changes over time. Many of these discoveries were made between July and August 2014, as Gaia performed many subsequent observations of a few patches of the sky close to the ecliptic poles. This closely sampled sequence of observations made it possible to find and study variable stars located in these regions.
Located close to the south ecliptic pole is the famous Large Magellanic Cloud (LMC), a dwarf galaxy and close companion of our own galaxy, the Milky Way. Gaia has delivered detailed light curves for dozens of RR Lyrae type variable stars in the LMC, and the fine details revealed in them testify to the very high quality of the data.
Another curious object covered during the same mission phase is the Cat’s Eye Nebula, a planetary nebula also known as NGC 6543, which lies close to the north ecliptic pole.
The Cat’s Eye Nebula.
Planetary nebulae are formed when the outer layers of an aging low-mass star are ejected and interact with the surrounding interstellar medium, leaving behind a compact white dwarf. Gaia made over 200 observations of the Cat’s Eye Nebula, and registered over 84 000 detections that accurately trace out the intricate gaseous filaments that such objects are famous for. As its observations continue, Gaia will be able to see the expansion of the nebular knots in this and other planetary nebulae.
Closer to home, Gaia has detected a wealth of asteroids, the small rocky bodies that populate our solar system, mainly between the orbits of Mars and Jupiter. Because they are relatively nearby and orbiting the Sun, asteroids appear to move against the stars in astronomical images, appearing in one snapshot of a given field, but not in images of the same field taken at later times.
Gaia scientists have developed special software to look for these ‘outliers’, matching them with the orbits of known asteroids in order to remove them from the data being used to study stars. But in turn, this information will be used to characterise known asteroids and to discover thousands of new ones.
Finally, in addition to the astrometric and photometric measurements being made by Gaia, it has been collecting spectra for many stars. The basic use of these data is to determine the motions of the stars along the line-of-sight by measuring slight shifts in the positions of absorption lines in their spectra due to the Doppler shift. But in the spectra of some hot stars, Gaia has also seen absorption lines from gas in foreground interstellar material, which will allow the scientists to measure its distribution.
Gaia is an ESA mission to survey one billion stars in our galaxy and local galactic neighbourhood in order to build the most precise 3D map of the Milky Way and answer questions about its origin and evolution.
The mission’s primary scientific product will be a catalogue with the positions, motions, brightnesses, and colours of the surveyed stars. An intermediate version of the catalogue will be released in 2016.
0 notes
Photo
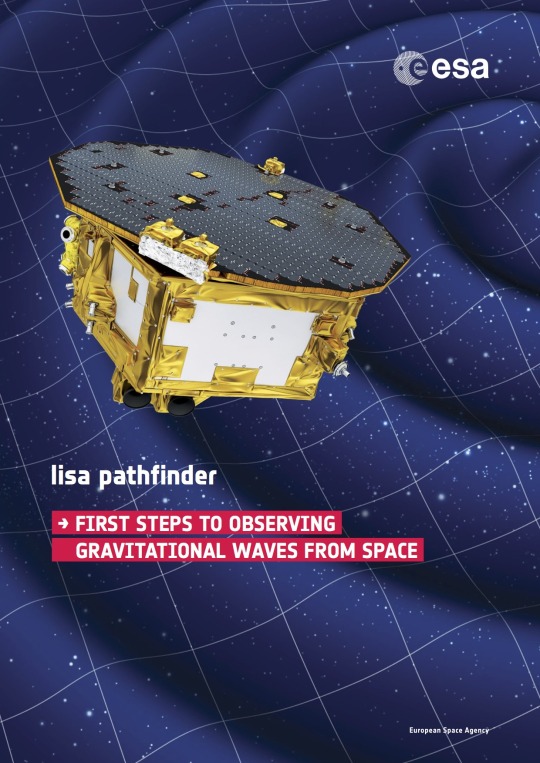
LISA Pathfinder - First steps to observing gravitational waves from space
The concept of gravitational-wave detection is based on monitoring two freely falling bodies. As long as all other disturbances can be sufficiently reduced and the two bodies are truly moving under the effect of gravity only, a gravitational wave passing between them would change their separation. LISA Pathfinder will test the underlying and most challenging condition for such experiments: whether it is possible to put two test masses into a near-perfect gravitational free-fall.
Even in space, realising a freely falling system is very complex. There are many non-gravitational forces at play, including radiation pressure from sunlight, charged particles from the solar wind and impacting micrometeoroids, as well as internal effects caused by the spacecraft and its instruments.
For this reason, LISA Pathfinder is a high-tech box that surrounds two freely falling test masses without touching them, shielding them from outside influence by constantly applying tiny adjustments to its position.
LISA Pathfinder is not aimed at the detection of gravitational waves themselves. Rather, its goal is to prove the innovative technologies needed to reduce external influences on two test masses and to measure their relative motion with unprecedented accuracy, tracking their free-fall by more than two orders of magnitude better than any past, present or planned mission.
LISA Pathfinder will create the most ‘silent’ place in the Solar System and measure how quiet it actually is.
0 notes
Photo
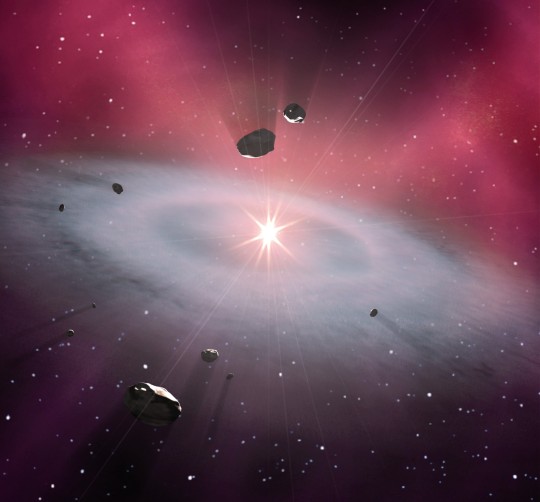
Why don't planets revolve around the sun vertically?
Answer by Robert Frost, Instructor and Flight Controller at NASA.
If we imagine a solar system as originating as a vast cloud that slowly condensed and coalesced into a star and orbiting planets, asteroids, and comets, it stands to reason that all of the bodies would orbit in the same general direction because they all are orbiting as part of the angular momentum of the original cloud.
Let's visualize such a cloud.
We know that angular momentum is conserved. We're all familiar with seeing an ice skater start rotating slowly with her arms outstretched and then she pulls in her limbs and starts to spin faster. The more she concentrates the space her body takes up, the faster she spins. The same is true for our stellar cloud.
We start with a vast cloud. It has some net angular momentum, causing it to rotate very very slowly in one direction. As gravity causes it to condense, that net momentum becomes more evident because the cloud starts to rotate faster.
The mass starts to concentrate at the center of the cloud, this center mass will become the Sun. The remaining mass orbits the central mass. A small mass orbiting a large mass orbits about the center (well the barycenter, but it's pretty close). That means we can't have the material orbiting the cloud like this:
It has to orbit like this:
Now we're kind of getting to the vertical scenario. This exact scenario isn't practical because the angular momentum in the pure vertical is zero. But what about out of plane towards vertical.
A system like this can exist - but it will not last - because, the two layers that are tilted have vertical motion components - they will cross through the center layer. That means there will be collisions. Over time these inelastic collisions will dampen out the vertical motion and the result will be every particle in a very thin plane like this:
And, over time the small particles in this plane will coalesce into larger bodies, forming the planets.
2 notes
·
View notes
Photo
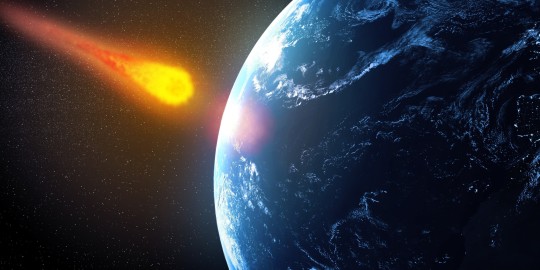
NASA: There is No Asteroid Threatening Earth
Numerous recent blogs and web postings are erroneously claiming that an asteroid will impact Earth, sometime between Sept. 15 and 28, 2015. On one of those dates, as rumors go, there will be an impact -- "evidently" near Puerto Rico -- causing wanton destruction to the Atlantic and Gulf coasts of the United States and Mexico, as well as Central and South America.
That's the rumor that has gone viral -- now here are the facts.
"There is no scientific basis -- not one shred of evidence -- that an asteroid or any other celestial object will impact Earth on those dates," said Paul Chodas, manager of NASA's Near-Earth Object office at the Jet Propulsion Laboratory in Pasadena, California.
In fact, NASA's Near-Earth Object Observations Program says there have been no asteroids or comets observed that would impact Earth anytime in the foreseeable future. All known Potentially Hazardous Asteroids have less than a 0.01% chance of impacting Earth in the next 100 years.
The Near-Earth Object office at JPL is a key group involved with the international collaboration of astronomers and scientists who keep watch on the sky with their telescopes, looking for asteroids that could do harm to our planet and predicting their paths through space for the foreseeable future. If there were any observations on anything headed our way, Chodas and his colleagues would know about it.
"If there were any object large enough to do that type of destruction in September, we would have seen something of it by now," he stated.
Another thing Chodas and his team do know -- this isn't the first time a wild, unsubstantiated claim of a celestial object about to impact Earth has been made, and unfortunately, it probably won't be the last. It seems to be a perennial favorite of the World Wide Web.
In 2011 there were rumors about the so-called "doomsday" comet Elenin, which never posed any danger of harming Earth and broke up into a stream of small debris out in space. Then there were Internet assertions surrounding the end of the Mayan calendar on Dec. 21, 2012, insisting the world would end with a large asteroid impact. And just this year, asteroids 2004 BL86 and 2014 YB35 were said to be on dangerous near-Earth trajectories, but their flybys of our planet in January and March went without incident -- just as NASA said they would.
"Again, there is no existing evidence that an asteroid or any other celestial object is on a trajectory that will impact Earth," said Chodas. "In fact, not a single one of the known objects has any credible chance of hitting our planet over the next century."
NASA detects, tracks and characterizes asteroids and comets passing 30 million miles of Earth using both ground- and space-based telescopes. The Near-Earth Object Observations Program, commonly called "Spaceguard," discovers these objects, characterizes the physical nature of a subset of them, and predicts their paths to determine if any could be potentially hazardous to our planet. There are no known credible impact threats to date -- only the continuous and harmless infall of meteoroids, tiny asteroids that burn up in the atmosphere.
0 notes
Video
youtube
How do we know that the earth is not the centre of the universe and other objects in the universe do not revolve around it?
We can observe directly about the solar system and the galaxy that the objects do not revolve around the earth. This has been observed for thousands of years in the retrograde motion of Mars, which appears to mysteriously turn around and go backwards for a while at certain points in its orbit.
For Mars to be orbiting Earth, it would have to have an extremely complicated and arbitrary orbit. By contrast, it is very easy to explain the motions of objects under gravity by one simple rule that applies to both apples on earth and planets in the sky.
That doesn't rule out beyond a shadow of a doubt that the universe could have been designed with some bizarre, arbitrary set of rules that just happen to mimic perfectly the operation of one very simple rule viewed from one particular location. But that's a very self-centered and inefficient way to go about trying to understand the universe.
It's far easier to construct a coherent, cogent model of the universe if you don't start with the assumption that you're in some special, select place. You could have been anywhere, and observed the same physical laws. That leads to very elegant and effective models of the universe that are far more useful than trying to preserve humanity's ego.
0 notes
Photo
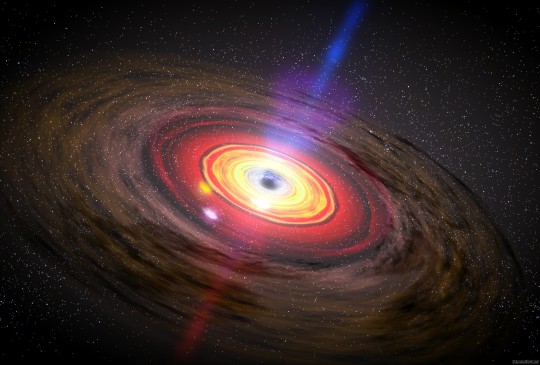
Bumpy Black Hole X-Rays May Push the Limits of Einstein's Relativity
In the century since Albert Einstein proposed his theory of general relativity physicists have put it through the wringer with extensive experimental tests—and it has withstood them all. But these experiments were conducted in environments of relatively weak gravity. Scientists have thus been left to wonder how well the theory describes the universe under more extreme conditions, like those found in the regions around black holes. To that end a new study suggests a means of testing the limits of the theory: Researchers have determined that if general relativity does break down near black holes, the effects may be detectable in x-rays blazing off the infalling matter. The study was published in Physical Review D on July 22.
According to general relativity, the phenomenon we experience as gravitational force is a result of spacetime (the combination of the three spatial dimensions and fourth dimension of time) curving around mass. The denser an object, the more severely it warps the fabric of spacetime and the stronger its gravitational field. Around objects like black holes—the remnants of exploded massive stars that are so compact not even light can escape their gravitational tug—spacetime is severely distorted. Physicists have used relativity, along with the so-called no-hair theorem, which states that black holes only have two defining characteristics (mass and rotation), to predict how spacetime curves around black holes. They call that curvature the Kerr solution.
Proving that the Kerr solution provides an accurate description of spacetime near a black hole would show that general relativity holds up even in environments of extreme gravity. But so far no one has been able to prove the Kerr solution’s correctness. Ideally, astrophysicists would record the motions of an object as it traveled through the region around a black hole to characterize the spacetime curvature. But some black holes are mere kilometers across, which is tiny on the cosmic scale. Scientists are not currently capable of tracking a single object moving in such a confined space from light-years away.
Even though no observational evidence yet suggests that relativity breaks down inside black holes, “that hasn’t stopped theorists from coming up with all sorts of alternative [theories of gravity],” says astrophysicist Jonathan Gair of the Institute of Astronomy at the University of Cambridge in England. These alternative theories produce their own descriptions of spacetime curvature that differ from the Kerr solution.
To help determine whether relativity or one of its competitors actually describes the warping of spacetime around black holes, Gair and fellow institute astrophysicist Christopher Moore used computer modeling to simulate several black holes with spacetime curves that somehow deviate from the topography described in the Kerr solution. They call these deviations “bumps.” The team wanted to know if Earthbound observers could detect the weird curvature around these bumpy black holes, so they looked for bump signatures in the radiation from the simulated black holes. As a black hole gobbles up surrounding matter, that material emits x-rays, which have slightly different characteristics depending on the black hole’s gravitational field.
Moore and Gair simulated black holes both with bumps described in specific alternative theories of gravity as well as a more general group of variously deformed black holes. “We wanted to do something more generic, because chances are, if general relativity is not right, we haven’t actually thought of the correct deviation,” Gair says. The researchers split their bumpy black holes into three categories, each with progressively smaller bumps: In the first category the spacetime curvature around the black hole is significantly different than the Kerr solution, even at long distances from the hole. In the second and third categories the bumps shrink more and more quickly, Moore explains.
By examining the x-rays produced by their simulated bumpy black holes, Moore and Gair determined which deformations scientists would be able to detect in the real thing, and which would blend in with the rest of the relativity-abiding universe. According to Moore, the team identified four bumps, all of which diminish in the slowest possible way, that scientists could feasibly detect. Knowing the x-ray signatures that correspond to particular black hole “bumps” provides a valuable reference for scientists taking x-ray observations of black holes in the future. According to Dimitrios Psaltis, an astrophysicist at the University of Arizona who was not involved with this study, the European Space Agency’s ATHENA x-ray observatory will conduct these observations when it launches in 2028.
Mike Kesden, an astrophysicist at The University of Texas at Dallas who was not involved with this study, notes that because only a few of Moore and Gair’s black hole bumps raise red flags in x-ray emission, this study provides further support for building gravitational wave detectors. Gravitational waves—ripples in spacetime—could encode information about the shape of spacetime around black holes. In fact, Moore and Gair plan to publish a follow-up study about the possibility of using gravitational waves to measure their simulated black hole bumps. So even if x-ray emission does not reveal the breakdown of general relativity near bumpy black holes, gravitational waves just might.
0 notes
Photo
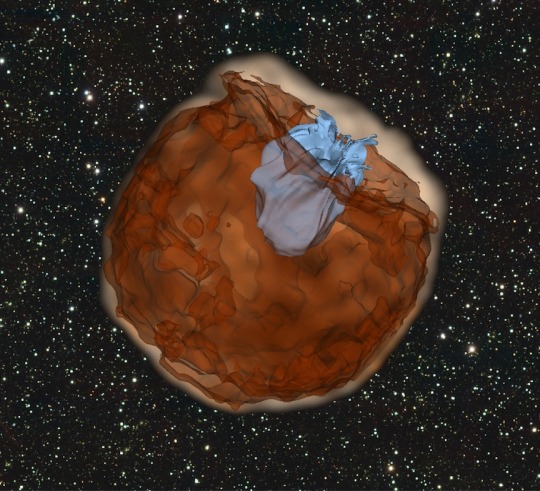
Captured at Last: The Tiny Stars that Spark Fierce Supernovae Explosions
Type 1a Supernova A visualization of a computer simulation of a Type Ia supernova. Ejected material (brown) from an exploding white dwarf star crashes into its companion star (blue), yielding an ultraviolet pulse of light streaming from the companion star's location.
A longstanding mystery about the tiny stars that let loose powerful explosions known as Type Ia supernovae might finally be solved. For brief periods, these cataclysmic blasts can outshine an entire galaxy of billions of stars. Astrophysicists want to understand their origins because they are integral to the evolution of galaxies and the study of dark energy.
Evidence has squarely pointed to "white dwarfs" as the source of these cosmic blow-ups. White dwarfs are the aged remnants of stars like our Sun that have contracted into a dense, compact balls about the size of Earth. Yet whether one white dwarf, or two, is needed to spark a Type Ia supernova inferno has remained frustratingly unclear to astrophysicists for decades now.
Two recent studies now offer perhaps the strongest evidence to date that both scenarios are valid, though the evidence hasn’t been easy to come by. Because supernovae are typically so far away, researchers must forensically work backwards from the explosion's aftermath to pinpoint its culprit star or stars. Now, armed with a new theory about Type Ia supernovae and aided by a fleet of modern telescopes, astronomers have shown that these stellar explosions can occur under more than one set of circumstances.
The Kavli Foundation spoke with four astrophysicists about the significance of nailing down the causes of Type Ia supernovae for learning more about the dynamics of stars, galaxies and even the universe on its grandest scales.
Top: An artist's impression of the single white dwarf Type Ia supernova scenario. Bottom: An artist's impression of the double white dwarf Type Ia supernova scenario.
One white dwarf star or two: Which scenario leads to a Type Ia supernova, one of the biggest, brightest explosions in the universe? In the single white dwarf scenario, the dwarf star gravitationally draws matter off of a normal, nearby companion star. That matter piles up on the white dwarf, eventually triggering a runaway thermonuclear explosion. An alternative scenario does not require a normal companion star; instead, two merging white dwarfs sparks a Type Ia supernova.
A new theory, developed by the physicist Daniel Kasen, has offered a fresh way of finding out which scenario, or both, is correct. The theory predicts that the material hurled out from the supernova explosion of a single white dwarf should create an observable ultraviolet flash when it slams into a normal companion star. The absence of that flash, on the other hand, would lend support to the double white dwarf scenario. Recently, astronomers have found examples of Type Ia supernovae with and without Kasen’s ultraviolet flash, suggesting that there is more than one way to unleash this cosmic outburst.
0 notes
Photo
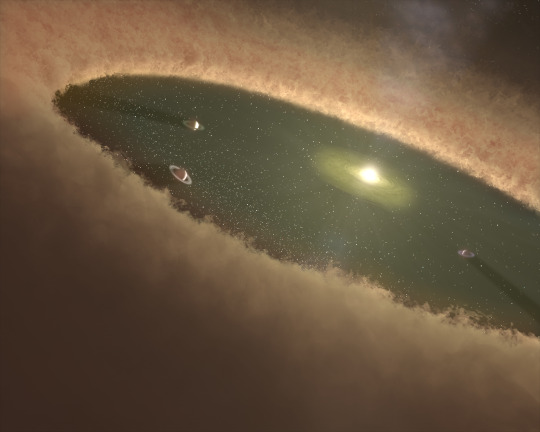
Scientists think “planetary pebbles” were the building blocks for the largest planets
This artist’s concept of a young star system shows gas giants forming first, while the gas nebula is present. Southwest Research Institute scientists used computer simulations to nail down how Jupiter and Saturn evolved in our own solar system. These new calculations show that the cores of gas giants likely formed by gradually accumulating a population of planetary pebbles – icy objects about a foot in diameter.
Researchers at Southwest Research Institute (SwRI) and Queen’s University in Canada have unraveled the mystery of how Jupiter and Saturn likely formed. This discovery, which changes our view of how all planets might have formed, is been published in the Aug. 20 issue of Nature.
Ironically, the largest planets in the solar system likely formed first. Jupiter and Saturn, which are mostly hydrogen and helium, presumably accumulated their gasses before the solar nebula dispersed. Observations of young star systems show that the gas disks that form planets usually have lifetimes of only 1 to 10 million years, which means the gas giant planets in our solar system probably formed within this time frame. In contrast, the Earth probably took at least 30 million years to form, and may have taken as long as 100 million years. So how could Jupiter and Saturn have formed so quickly?
The most widely accepted theory for gas giant formation is the so-called core accretion model. In this model, a planet-sized core of ice and rock forms first. Then, an inflow of interstellar gas and dust attaches itself to the growing planet. However, this model has an Achilles heel; specifically, the very first step in the process. To accumulate a massive atmosphere requires a solid core roughly 10 times the mass of Earth. Yet these large objects, which are akin to Uranus and Neptune, had to have formed in only a few million years.
In the standard model of planet formation, rocky cores grow as similarly sized objects accumulate and assimilate through a process called accretion. Rocks incorporate other rocks, creating mountains; then mountains merge with other mountains, leading to city-sized objects, and so on. However, this model is unable to produce planetary cores large enough, in a short enough period of time, to explain Saturn and Jupiter.
“The timescale problem has been sticking in our throats for some time,” said Dr. Hal Levison, an Institute scientist in the SwRI Planetary Science Directorate and lead author of the paper.
“It wasn’t clear how objects like Jupiter and Saturn could exist at all,” continued Levison. New calculations by the team show that the cores of Jupiter and Saturn could form well within the 10-million-year time frame if they grew by gradually accumulating a population of planetary pebbles – icy objects about a foot in diameter. Recent research has shown that gas can play a vital role in increasing the efficiency of accretion. So pebbles entering orbit can spiral onto the protoplanet and assimilate, assisted by a gaseous headwind.
In their article, Levison, Kretke, and Duncan show that pebble accretion can produce the observed structure of the solar system as long as the pebbles formed slowly enough that the growing planets have time to gravitationally interact with one another.
“If the pebbles form too quickly, pebble accretion would lead to the formation of hundreds of icy Earths,” said Kretke. “The growing cores need some time to fling their competitors away from the pebbles, effectively starving them. This is why only a couple of gas giants formed.”
“As far as I know, this is the first model to reproduce the structure of the outer solar system, with two gas giants, two ice giants (Uranus and Neptune), and a pristine Kuiper belt,” says Levison.
“After many years of performing computer simulations of the standard model without success, it is a relief to find a new model that is so successful,” adds Duncan.
0 notes
Photo
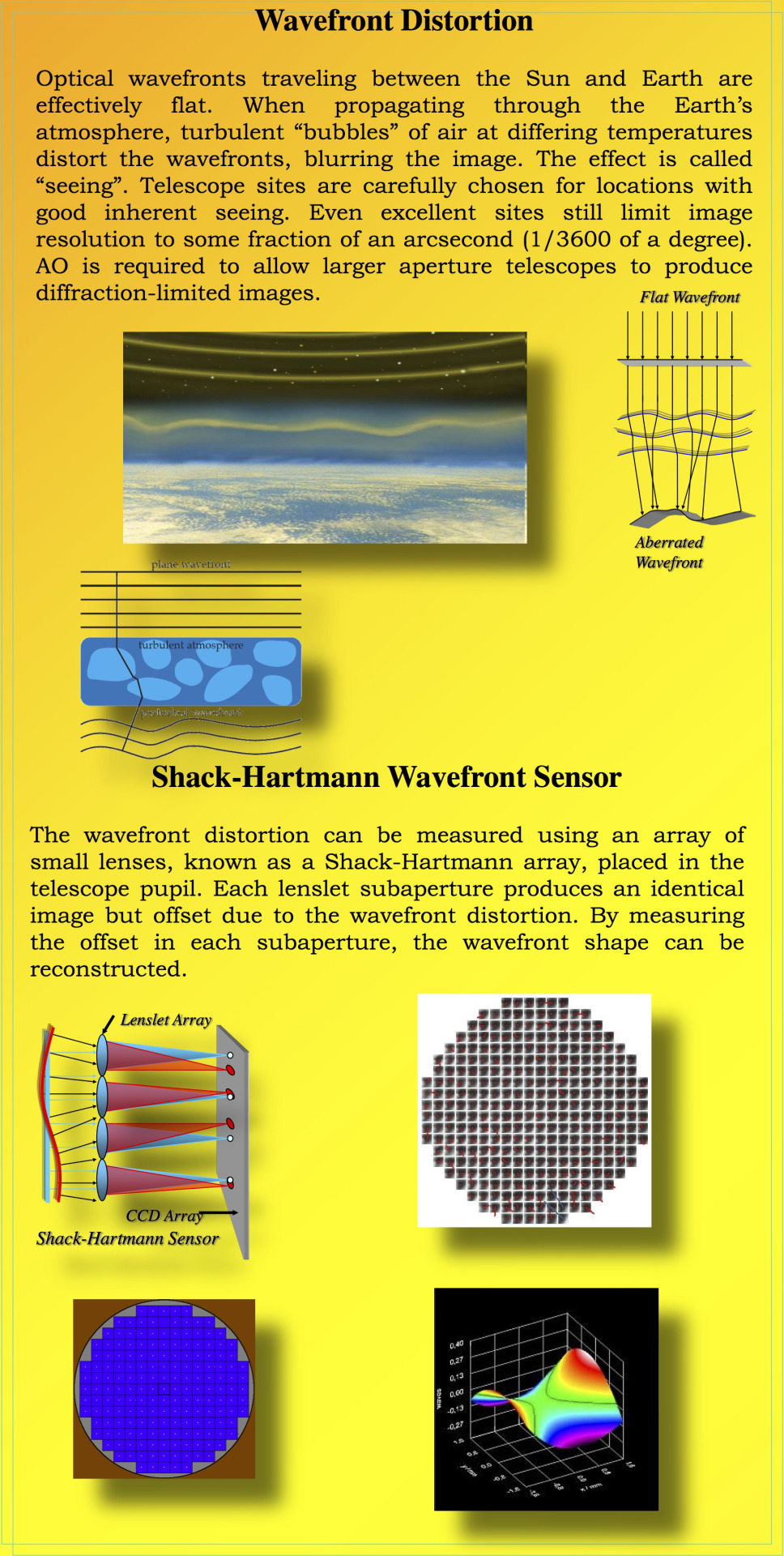
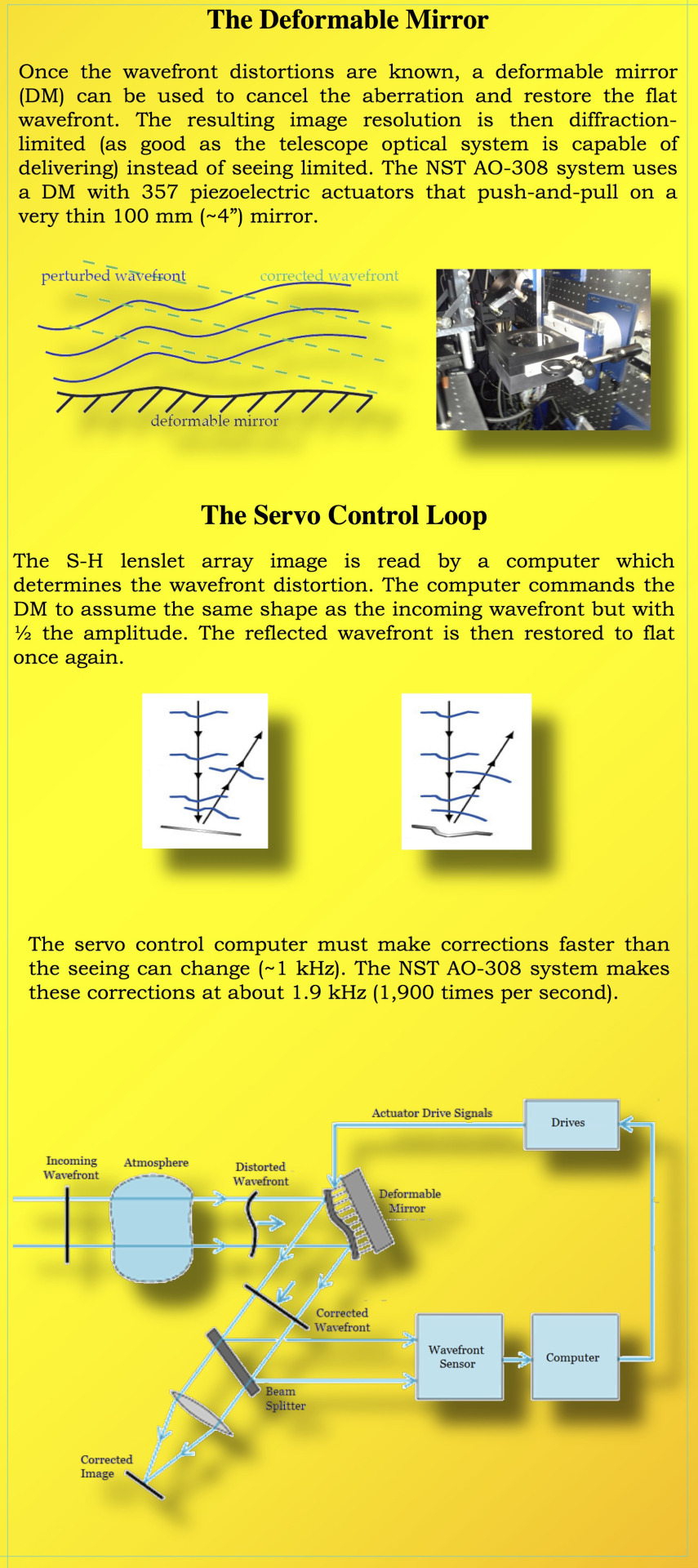
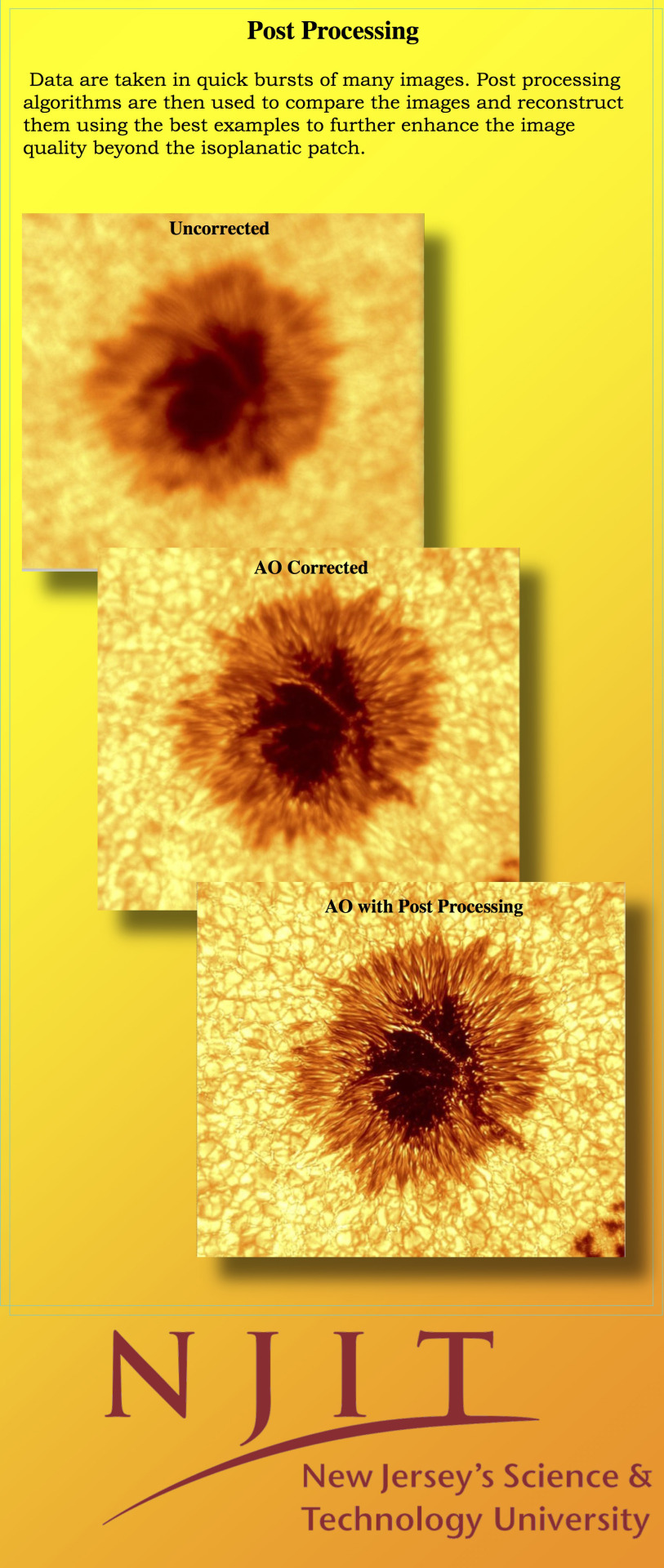
BBSO AO-308 Adaptive Optics
Adaptive optics (AO) is a technique that corrects in real time for image distortions caused by motions in the Earth’s atmosphere. The Big Bear Solar Observatory (BBSO) New Solar Telescope (NST) depends on a state-of-the-art AO system to enable diffraction-limited imaging (0.08 arc seconds in the visible). The current NST AO incorporates a Shack-Hartmann lens let array with 308 sub apertures for measuring wavefront error. The wavefront distortion is the corrected using a deformable mirror (DM) with 357 piezoelectric actuators.
AO systems, such as AO-308, can deliver diffraction-limited images over a relatively small angle on the sky known as isoplanatic patch. Image quality gradually deteriorates with increasing angle from the patch. The size of the patch varies with seeing conditions.
0 notes